- 1Center for Cardiac Intensive Care, Beijing Anzhen Hospital, Capital Medical University, Beijing, China
- 2School of Public Health, Capital Medical University, Beijing, China
Objective: To investigate the feasibility of drainage from the superior vena cava (SVC) to improve upper body oxygenation in patients with cardiogenic shock undergoing femoral veno-arterial extracorporeal membrane oxygenation (VA ECMO).
Methods: Seventeen adult patients receiving peripheral femoral VA ECMO for circulatory support were enrolled. The femoral drainage cannula was shifted three times (from the inferior vena cava (IVC) level to the SVC level and then the IVC level again), all under ultrasound guidance, at an interval of 15 minutes. The blood gas levels of the right radial artery (RA) and SVC and cerebral oxygen saturation (ScO2) were measured and compared.
Results: Fifteen patients (88.2%) were successfully weaned from ECMO, and 12 patients (70.6%) survived to discharge. The oxygen saturation (SO2) and oxygen partial pressure (PO2) of the RA (97.0 ± 3.5% to 98.3 ± 1.5%, P < 0.05, SO2; 127.4 ± 58.2 mmHg to 153.1 ± 67.8 mmHg, P < 0.05, PO2) and SVC (69.5 ± 9.0% to 75.7 ± 8.5%, P < 0.05, SO2; 38.5 ± 5.6 mmHg to 43.6 ± 6.4 mmHg, P < 0.05, PO2) were increased; ScO2 was also increased on both sides (left: 50.6 ± 8.6% to 55.0 ± 9.0%, P < 0.05; right: 48.7 ± 9.2% to 52.3 ± 9.8%, P < 0.05) when the femoral drainage cannula was shifted from the IVC level to the SVC level. When the femoral drainage cannula was shifted from SVC level to the IVC level again, the SO2 and PO2 of RA (98.3 ± 1.5% to 96.9 ± 3.2%, P <0.05, SO2; 153.1 ± 67.8 mmHg to 125.8 ± 63.3 mmHg, P <0.05, PO2) and SVC (75.7 ± 38.5% to 70.4 ± 7.6%, P <0.05, SO2; 43.6 ± 6.4 mmHg to 38.9 ± 4.5 mmHg, P < 0.05, PO2) were decreased; ScO2 was also reduced on both sides (left: 55.0 ± 9.0% to 50.7 ± 8.2%, P < 0.05; right: 52.3 ± 9.8% to 48.7 ± 9.3%, P <0.05).
Conclusion: Drainage from the SVC by shifting the cannula upward could improve upper body oxygenation in patients with cardiogenic shock undergoing femoral VA ECMO. This cannulation strategy provides an alternative solution for differential hypoxia.
Introduction
Venous-arterial extracorporeal membrane oxygenation (VA ECMO) is a temporary mechanical circulatory support device that provides circulatory and respiratory support for patients with cardiogenic shock (1–4). However, complications, such as hemorrhage, infection, and acute kidney injury, may occur during VA ECMO support and are associated with poor outcomes (5).
Differential hypoxia is a severe complication that can lead to an insufficient oxygen supply to the heart and brain and affect the prognosis of patients (6–9). The incidence of differential hypoxia was 8.8% in patients receiving femo-femoral VA ECMO (10). Differential hypoxia occurs when femo-femoral VA ECMO patients have residual cardiac function and severely impaired pulmonary function (i.e., acute respiratory failure, redundant fluid expansion, and pulmonary edema) (11–13). In this situation, the blood to coronary arteries, cerebral blood vessels, and upper limbs is ejected from the left heart and the native lung and is poorly oxygenated. Additionally, the lower part of the body is supplied with blood from ECMO, which is well oxygenated (14, 15).
Raising the ventilator parameters and increasing ECMO flow were reported to increase oxygen delivery. However, the former may lead to lung injury (16), and the latter may cause blood cell destruction, coagulation dysfunction, and high left ventricular afterload (17–20). Differential hypoxia can also be solved by changing the position of the returning cannula, such as to the axillary artery or ascending aortic artery, or shifting to the veno-arterio-venous ECMO mode (21–25). However, additional invasive procedures are needed. A simpler alternative to relieve differential hypoxia is to place the femoral drainage cannula at the superior vena cava (SVC) level. Differential hypoxia makes blood oxygen saturations in the SVC and inferior vena cava (IVC) different. Blood in the SVC is oxygen-poor, and blood in the IVC is oxygen-rich. Therefore, drainage from the SVC makes oxygen-poor blood drain to the ECMO oxygenator, and oxygen-rich IVC blood returns to the native lung, increasing the oxygen content in the pulmonary circulation. However, that finding was only proven in animal models (26, 27).
This single-center prospective study aimed to verify the feasibility of drainage from the SVC to improve upper body oxygenation in patients with cardiogenic shock undergoing femoral VA ECMO.
Materials and Methods
Setting
This study was conducted between January 2021 and July 2021 at the Center for Cardiac Intensive Care, Beijing Anzhen Hospital, Capital Medical University, and was approved by the hospital's institutional review board (approved number: 2020187X). Informed consent was obtained from all the patients or their surrogates.
Patients
This study enrolled patients (aged ≥ 18 years) with cardiogenic shock or cardiac arrest. The patients received VA ECMO for circulatory support in the intensive care unit or intraoperatively in the operating room for circulatory instability during or immediately after weaning from cardiopulmonary bypass in the primary cardiac procedure. They also received VA ECMO for refractory cardiogenic shock, malignant arrhythmia or cardiac arrest occurring in the intensive care unit (ICU), ward, or catheterization room. Patients were excluded if any one of the following was present: inability to maintain stable ECMO flow or uncontrollable surgical bleeding or bleeding at the cannulation site.
ECMO Management
The patients had undergone ECMO implantation via peripheral cannulation through the femoral route using the cutdown method. A distal leg perfusion cannula was routinely introduced to prevent lower extremity ischemia. The ECMO flow settings were adjusted to maintain a mean arterial pressure (MAP) of 60–90 mmHg. Continuous intravenous heparin was administered to obtain an activated clotting time of 180–220 s during VA ECMO support. The 15 Fr or 17 Fr return cannula and 21 Fr femoral drainage cannula (Bio-Medicus Cannula; Medtronic Inc., Michigan, USA) were selected according to the patient's body weight. A protective lung ventilation strategy was adopted during the experiment, with a tidal volume of 6 mL/kg, a positive end-expiratory pressure of 6–8 cmH2O, a respiratory rate of 10–12 times/min, a FiO2 level of 40%, and unchanged ventilator parameters during the experiment.
Study Protocol
The experiment was performed when the cannula was adjusted after ECMO was successfully established, and the patient was provided with circulatory and respiratory support. During the experiment, the ECMO flow rate, return cannula position, drug type, and dose were kept unchanged. This experimental procedure shifted the femoral drainage cannula three times, all under ultrasound guidance (GE Vivid-i ultrasound system; GE Health care, Milwaukee, WI), at an interval of 15 min (Figure 1). The femoral drainage cannula position after each move was as follows: (1) IVC: the tip of the femoral drainage cannula was moved to the level of the opening of the hepatic vein in the IVC; (2) SVC: the tip of the femoral drainage cannula was moved to the level of the opening of the SVC; (3) IVC: the femoral drainage cannula position was the same as that in the first step. Fifteen min after each move, the blood gas levels of the right radial artery (RA) and SVC were analyzed (Stat Profile pHOx Ultra; Nova Biomedical, USA). Cerebral oxygen saturation (ScO2) and hemodynamic parameters (MAP, heart rate [HR], and velocity time integral [VTI] of the aortic artery) were also evaluated. Arterial and central venous catheters were placed in all the patients were placed after entering the ICU. The central venous catheter was positioned with the tip within 2 cm above the SVC. This position was verified by chest radiography and adjusted if necessary.
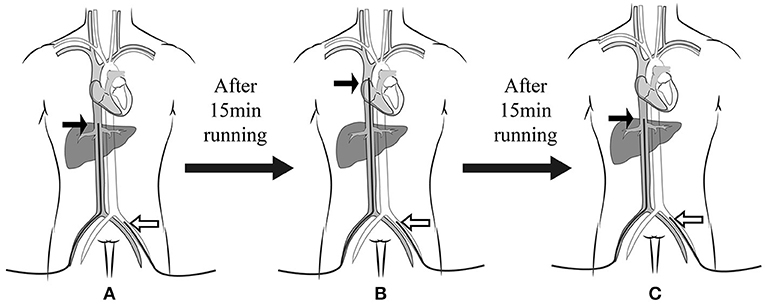
Figure 1. Experimental procedure. The femoral drainage cannula was shifted three times at an interval of 15 min. The femoral drainage cannula position after each move was (A) at the IVC level, (B) SVC level, and (C) IVC level again. The black arrow indicates the drainage cannula, and the white arrow indicates the return cannula. IVC, inferior vena cava; SVC, superior vena cava.
Cerebral Oxygen Saturation Monitoring
ScO2 was measured using a tissue oxygen saturation monitor (Cerebral Oximeter Mc-2030c; CAS Medical Systems, USA). Near-infrared spectroscopy (NIRS) probes were placed on the upper edge of the patient's bilateral eyebrow arch.
Statistical Analysis
Statistical analysis was performed using SPSS 26.0 software (SPSS Inc., Chicago, IL, USA). The data were presented as means (standard deviation) for continuous variables and numbers (percentages) for categorical variables. The differences before and after cannula shifting were analyzed using paired Student's t-test. A P value less than 0.05 was considered statistically significant.
Results
Patients
From January 2021 to July 2021, 17 patients had received peripheral femoral VA ECMO. The mean age was 58.2 ± 11.5 years, and 15 (88.2%) patients were male. All the patients had received circulatory support following the cardiac procedure, including coronary artery bypass grafting (CABG) (7), CABG combined with a valvular procedure (4), aortic operation (4), and a valvular procedure (2). Three patients (17.6%) had established ECMO through extracorporeal cardiopulmonary resuscitation (ECPR).
Fifteen patients (88.2%) were successfully weaned from ECMO, and 12 patients (70.6%) survived to discharge. Three patients died after ECMO weaning (2 because of multiorgan failure and 1 because of septic shock). The mean ECMO flow was 3.5 ± 0.5 L/min. The mean ICU length of stay was 14.4 ± 9.8 days. The detailed patient characteristics are shown in Table 1.
Hemodynamic Parameters
Seventeen data groups were collected during the study. No statistically significant differences were found in HR, MAP, or aortic VTI before and after the femoral drainage cannula was moved (P > 0.05) (Table 2).
Overall Result
Compared with the femoral drainage cannula at the IVC level, after shifting to the SVC level, the oxygen saturation (SO2) and oxygen partial pressure (PO2) of the RA (97.0 ± 3.5% to 98.3 ± 1.5%, P < 0.05, SO2; 127.4 ± 58.2 mmHg to 153.1 ± 67.8 mmHg, P < 0.05, PO2) and SVC (69.5 ± 9.0% to 75.7 ± 8.5%, P < 0.05, SO2; 38.5 ± 5.6 mmHg to 43.6 ± 6.4 mmHg, P < 0.05, PO2) were increased; ScO2 was also increased on both sides (left: 50.6 ± 8.6% to 55.0 ± 9.0%, P < 0.05; right: 48.7 ± 9.2% to 52.3 ± 9.8%, P < 0.05) (Figure 2).
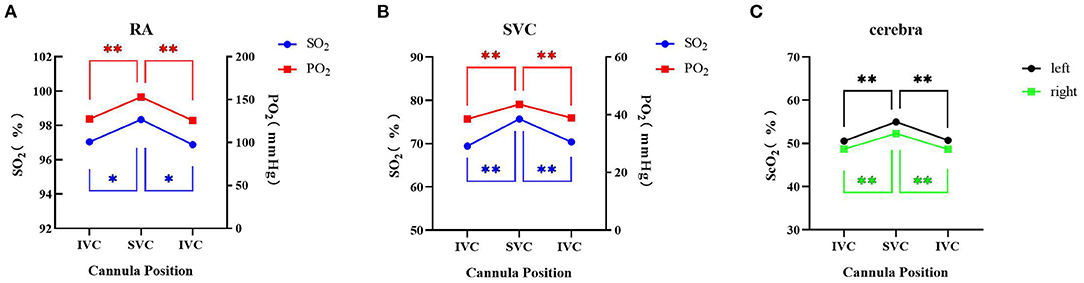
Figure 2. Overall blood gas analysis and cerebral oxygen saturation with different positions of the drainage cannula on femoral VA ECMO. (A) Right radial artery SO2 and PO2; (B) superior vena cava SO2 and PO2; (C) cerebral oxygen saturation. * Indicates P < 0.05 between IVC and SVC; ** Indicates P <0.01 between IVC and SVC. IVC, inferior vena cava; SVC: superior vena cava; RA, right radial artery; SO2, oxygen saturation; PO2, oxygen partial pressure; ScO2, cerebral oxygen saturation.
When the femoral drainage cannula was shifted from the SVC level to the IVC level again, the SO2 and PO2 of the RA (98.3 ± 1.5% to 96.9 ± 3.2%, P < 0.05, SO2; 153.1 ± 67.8 mmHg to 125.8 ± 63.3 mmHg, P < 0.05, PO2) and SVC (75.7 ± 38.5% to 70.4 ± 7.6%, P < 0.05, SO2; 43.6 ± 6.4 mmHg to 38.9 ± 4.5 mmHg, P < 0.05, PO2) were decreased; ScO2 was also reduced on both sides (left: 55.0 ± 9.0% to 50.7 ± 8.2%, P < 0.05; right: 52.3 ± 9.8% to 48.7 ± 9.3%, P < 0.05) (Figure 2).
Upper Body Hypoxia Group and Upper Body Normoxia Group
To determine whether similar changes occurred in patients with different degrees of upper body oxygen supply, we divided the patients into an upper body hypoxia group (n = 8) and an upper body normoxia group (n = 9) based on the initial central venous oxygen saturation (ScvO2) (measured during placement of the femoral drainage cannula on the IVC for the first time). Upper body hypoxia was defined as ScvO2 <70%, and normoxia was defined as ScvO2 ≥ 70%. The ScvO2 was 62.3 ± 8.2 in the upper body hypoxia group and 75.8 ± 2.5 in the upper body normoxia group (p < 0.01).
No significant differences were found in the hemodynamic parameters before and after the femoral drainage cannula was moved in either group (Table S1). The SO2 and PO2 of the SVC and ScO2 were higher with the femoral drainage cannula at the SVC level than at the IVC level in both groups (Tables 3, 4 and Figures S1, S2). However, no significant difference in the degree (SVC - IVC or SVC - IVC again) of improvement in the SO2 of the SVC between the groups (7.4 ± 8.5% in the upper body hypoxia group vs. 5.2 ± 4.6% in the upper body normoxia group, P = 0.51, SVC - IVC; 5.2 ± 5.7% in the upper body hypoxia group vs. 5.4 ± 6.6% in the upper body normoxia group, P = 0.963, SVC - IVC again). In the upper body hypoxia group, compared with the femoral drainage cannula at the IVC level, after shifting to the SVC level, the PO2 of the RA was increased, and SO2 showed an upward trend but no significant differences. No change was observed in the SO2 and PO2 of the RA in the upper body normoxia group (Table 3 and Figure S1).
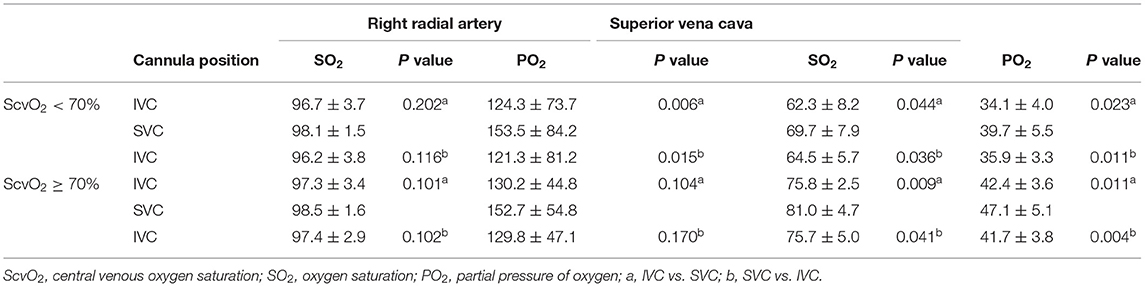
Table 3. Blood gas analysis in upper body hypoxia group (ScvO2 < 70%) and upper body normoxia group (ScvO2 ≥ 70%).
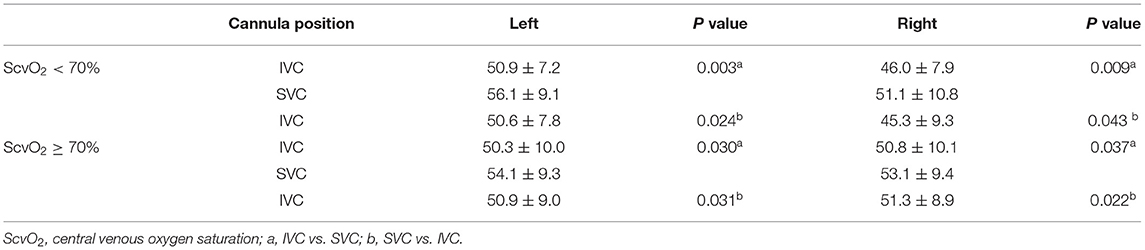
Table 4. Cerebral oxygen saturation in upper body hypoxia group (ScvO2 < 70%) and upper body normoxia group (ScvO2 ≥ 70%).
Discussion
Compared with drainage from the IVC, the present study suggested that the femoral cannula drained from the SVC achieved higher SO2 and PO2 of the right radial artery in peripheral VA ECMO, representing oxygenation of the upper body. This study is the first to validate this cannulation strategy in patients, providing an alternative solution for differential hypoxia.
The present study found a significant increase in the SO2 of the RA after shifting the femoral drainage cannula position to the SVC level. We consider the mechanism as follows: a dual circulatory system with both self-circulation and ECMO circulation exists in patients with differential hypoxia. Self-circulation refers to oxygen-poor blood oxygenated by impaired lung injection through the left heart into the proximal branches of the aorta. ECMO circulation indicates well-saturated ECMO blood perfusion of the descending aorta and its distal end. Therefore, when the femoral drainage cannula is located in the IVC (Figure 3A), oxygen-rich blood returning to the IVC is drained back to the ECMO circulation, and oxygen-poor blood returning to the SVC enters the right atrium and restarts self-circulation. Therefore, the oxygen supply to the upper body worsens. Placing the femoral drainage cannula on the SVC level breaks the dual circulatory system (Figure 3B). Thus, the oxygen-poor blood from the SVC in the self-circulation is drained out by the femoral drainage cannula into the ECMO circulation, and the oxygen-rich blood from the IVC in the ECMO circulation enters the right atrium to participate in the self-circulation, eventually improving upper body oxygenation.
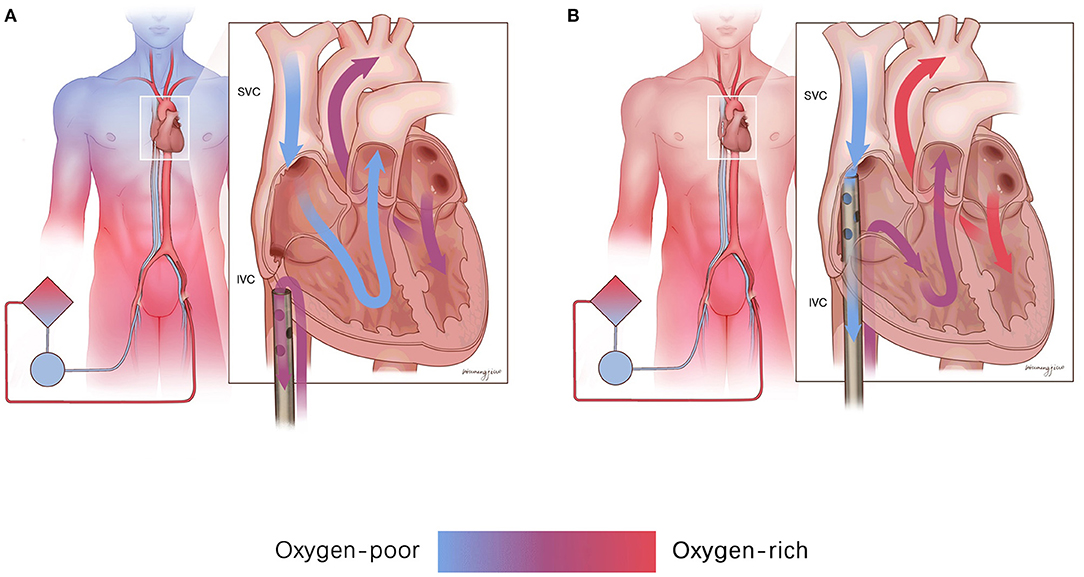
Figure 3. The mechanism of drainage from the SVC improves upper body oxygenation. (A) Drainage from the SVC made the oxygen-rich blood from the IVC drain back to the ECMO circulation, and the oxygen-poor blood from the SVC enters the right atrium and restarts the self-circulation. (B) Drainage from the SVC could drain out the oxygen-poor blood from the SVC into the ECMO circulation, and the oxygen-rich blood from the IVC could enter the right atrium to participate in the self-circulation. IVC, inferior vena cava; SVC, superior vena cava.
Lindfors et al. reached the same conclusion. They applied an artificial model to study the effect of different drainage cannula positions on upper body oxygenation in femoral VA ECMO patients. They found that the SO2 of RA was 64% (IVC), 75% (right atrium), and 81% (SVC) at different drainage cannula positions. They also reported a patient receiving VA ECMO using a double femoral vein drainage cannula (21 Fr, 18 and 50 cm inside the vein), and the patient developed respiratory failure with an RA SO2 of 57%. After moving the tip of the longer drainage cannula from the IVC to the right atrium, the SO2 of the RA increased by 5–10% (28).
With drainage from the SVC, upper body oxygenation could be improved. In the present study, we monitored the ScO2 of patients by near-infrared spectroscopy (NIRS), which was not available in the previous study. NIRS can monitor cerebral oxygen saturation, reflecting the microcirculation and tissue perfusion of patients (29). We found that placing the femoral drainage cannula on the SVC level increased ScO2 in femoral VA ECMO patients. The occurrence of neurological complications may be reduced theoretically. Previous experiments have also demonstrated that the animal model with differential hypoxia after receiving femoral VA ECMO shows reduced ScO2 (6). Choi (7) and Loftsgard (8) respectively reported a case of patients who developed differential hypoxia several days after receiving VA ECMO and developed neurological complications immediately thereafter. One of the patients presented with generalized tonic-clonic seizure, and an electroencephalogram showed delta waves suggesting diffuse cerebral cortical dysfunction (7). The other showed acute onset of neurogenic pulmonary edema, an uncommon complication of anoxic brain injury (8). However, their neurological symptoms were relieved after improving upper body oxygenation.
Previous studies have also suggested that in patients receiving femo-femoral VA ECMO with respiratory circulatory failure, myocardial hypoxia occurred as residual cardiac function increased (30). Coronary oxygen saturation was measured using proximal aortic oxygen saturation, and coronary oxygen insufficiency has been demonstrated in patients (9). Improving hypoxia of the upper body can make the myocardial oxygen supply sufficient.
Subgroup analysis was conducted. ScvO2 <70% reflects an insufficient oxygen supply in the upper body (31). To investigate whether this cannulation strategy shows a better oxygenation improvement effect in patients with upper body hypoxia, we classified patients with an initial measurement of ScvO2 <70% as the upper body hypoxia group and those with ScvO2 ≥ 70% as the upper body normoxia group. However, no significant difference was found in the degree of improvement in upper body oxygenation between the groups. The reason is likely that the degree of hypoxia in the upper body group was not very obvious.
Conversely, for both groups, placing the femoral drainage cannula on the SVC level increased patient ScvO2. Previous studies have shown that low postoperative ScvO2 is associated with an increased risk of complications in high-risk surgical patients, whereas elevated ScvO2 significantly reduces mortality in critically ill patients (32–34). However, studies on the effect of ScvO2 in patients receiving VA ECMO are lacking.
Limitations
The present study was conducted in patients without severely impaired pulmonary function undergoing peripheral VA ECMO. We did not investigate the effect of SVC drainage in patients with severe differential hypoxia. Additionally, the cannulation strategy in the present study requires shifting the drainage cannula upward. The cannula is limited in length and may not reach the SVC opening in taller patients. Thus, this approach may only work for some people.
Conclusion
Drainage from the SVC by shifting the cannula upward improved upper body oxygenation in patients with cardiogenic shock undergoing femoral VA ECMO. This cannulation strategy provides an alternative solution for differential hypoxia. The improvement of cardiac function, cerebral function and prognosis of patients using this cannulation strategy warrants further study for confirmation.
Data Availability Statement
The raw data supporting the conclusions of this article will be made available by the authors, without undue reservation.
Ethics Statement
The studies involving human participants were reviewed and approved by Beijing Anzhen Hospital (affiliated to Capital Medical University)'s Institutional Ethics Committee. The patients/participants provided their written informed consent to participate in this study.
Author Contributions
TC, CL, and QF manuscript writing. TC, BX, CL, XHo, and HW study design. TC, CL, BX, and LW data analysis. ZD, XHa, DG, CJ, ZX, MX, and PW data collection. LW, XHo, XHa, and HW manuscript revision. All authors read and approved the final manuscript.
Funding
This study was supported by grants from the Beijing Hospitals Authority Clinical Medicine Development of Special Funding Support, Code: ZYLX202111 and Beijing Hospitals Authority Ascent Plan, Code: FDL20190601.
Conflict of Interest
The authors declare that the research was conducted in the absence of any commercial or financial relationships that could be construed as a potential conflict of interest.
Publisher's Note
All claims expressed in this article are solely those of the authors and do not necessarily represent those of their affiliated organizations, or those of the publisher, the editors and the reviewers. Any product that may be evaluated in this article, or claim that may be made by its manufacturer, is not guaranteed or endorsed by the publisher.
Supplementary Material
The Supplementary Material for this article can be found online at: https://www.frontiersin.org/articles/10.3389/fcvm.2021.807663/full#supplementary-material
Abbreviations
VA ECMO, Veno-arterial extracorporeal membrane oxygenation; SVC, superior vena cava; IVC, inferior vena cava; RA, right radial artery; ICU, intensive care unit; SO2, oxygen saturation; PO2, oxygen partial pressure; ScO2, cerebral oxygen saturation; ScvO2, central venous oxygen saturation; VTI, velocity time integral; MAP, mean arterial pressure; HR, heart rate.
References
1. Conrad SA, Broman LM, Taccone FS, Lorusso R, Malfertheiner MV, Pappalardo F, et al. The extracorporeal life support organization maastricht treaty for nomenclature in extracorporeal life support. A position paper of the extracorporeal life support organization. Am J Respir Crit Care Med. (2018) 198:447–51. doi: 10.1164/rccm.201710-2130CP
2. Mosier JM, Kelsey M, Raz Y, Gunnerson KJ, Meyer R, Hypes CD, et al. Extracorporeal membrane oxygenation (ECMO) for critically ill adults in the emergency department: history, current applications, and future directions. Crit Care. (2015) 19:431. doi: 10.1186/s13054-015-1155-7
3. Lorusso R, Shekar K, MacLaren G, Schmidt M, Pellegrino V, Meyns B, et al. ELSO interim guidelines for venoarterial extracorporeal membrane oxygenation in adult cardiac patients. ASAIO J. (2021) 67:827–44. doi: 10.1097/MAT.0000000000001510
4. Miller PE, Solomon MA, McAreavey D. Advanced percutaneous mechanical circulatory support devices for cardiogenic shock. Crit Care Med. (2017) 45:1922–9. doi: 10.1097/CCM.0000000000002676
5. Bačić G, Tomulić V, Medved I, Zaputović L, Zaninović T, Jurjević, et al. Extracorporeal membrane oxygenation in adults cardiologia. Croatica. (2017) 12:216–25. doi: 10.15836/ccar2017.216
6. Wada H, Watari M, Sueda T, Kochi K, Sakai H, Shibamura H, et al. Cerebral tissue oxygen saturation during percutaneous cardiopulmonary support in a canine model of respiratory failure. Artif Organs. (2000) 24:640–3. doi: 10.1046/j.1525-1594.2000.06601.x
7. Choi JH, Kim SW, Kim YU, Kim SY, Kim KS, Joo SJ, et al. Application of veno-arterial-venous extracorporeal membrane oxygenation in differential hypoxia. Multidiscip Respir Med. (2014) 9:55. doi: 10.1186/2049-6958-9-55
8. Loftsgard TO, Newcome MD, Hanneman MR, Patch RK, Seelhammer TG. Management of neurogenic pulmonary edema and differential hypoxemia in an adult supported on venoarterial extracorporeal membrane oxygenation. J Cardiothorac Vasc Anesth. (2017) 31:2170–4. doi: 10.1053/j.jvca.2017.03.044
9. Lee JG, Kim N, Narm KS, Suh JW, Hwang J, Paik HC, et al. The effect of additional stepwise venous inflow on differential hypoxia of veno-arterial extracorporeal membrane oxygenation. ASAIO J. (2020) 66:803–8. doi: 10.1097/MAT.0000000000001052
10. Rupprecht L, Lunz D, Philipp A, Lubnow M, Schmid C. Pitfalls in percutaneous ECMO cannulation. Heart Lung Vessel. (2015) 7:320–6.
11. Falk L, Sallisalmi M, Lindholm JA, Lindfors M, Frenckner B, Broome M, et al. Differential hypoxemia during venoarterial extracorporeal membrane oxygenation. Perfusion. (2019) 34:22–9. doi: 10.1177/0267659119830513
12. Moller PW, Hana A, Heinisch PP, Liu S, Djafarzadeh S, Haenggi M, et al. The effects of vasoconstriction and volume expansion on veno-arterial ECMO flow. Shock. (2019) 51:650–8. doi: 10.1097/SHK.0000000000001197
13. Moller PW, Winkler B, Hurni S, Heinisch PP, Bloch A, Sondergaard S, et al. Right atrial pressure and venous return during cardiopulmonary bypass. Am J Physiol Heart Circ Physiol. (2017) 313:H408–H20. doi: 10.1152/ajpheart.00081.2017
14. Sidebotham D, McGeorge A, McGuinness S, Edwards M, Willcox T, Beca J. Extracorporeal membrane oxygenation for treating severe cardiac and respiratory failure in adults: part 2-technical considerations. J Cardiothorac Vasc Anesth. (2010) 24:164–72. doi: 10.1053/j.jvca.2009.08.002
15. Hoeper MM, Tudorache I, Kuhn C, Marsch G, Hartung D, Wiesner O, et al. Extracorporeal membrane oxygenation watershed. Circulation. (2014) 130:864–5. doi: 10.1161/CIRCULATIONAHA.114.011677
16. Matthay MA, Ware LB, Zimmerman GA. The acute respiratory distress syndrome. J Clin Invest. (2012) 122:2731–40. doi: 10.1172/JCI60331
17. Russo JJ, Aleksova N, Pitcher I, Couture E, Parlow S, Faraz M, et al. Left ventricular unloading during extracorporeal membrane oxygenation in patients with cardiogenic shock. J Am Coll Cardiol. (2019) 73:654–62. doi: 10.1016/j.jacc.2018.10.085
18. Schrage B, Burkhoff D, Rubsamen N, Becher PM, Schwarzl M, Bernhardt A, et al. Unloading of the left ventricle during venoarterial extracorporeal membrane oxygenation therapy in cardiogenic shock. JACC Heart Fail. (2018) 6:1035–43. doi: 10.1016/j.jchf.2018.09.009
19. Doyle AJ, Hunt BJ. Current understanding of how extracorporeal membrane oxygenators activate haemostasis and other blood components. Front Med. (2018) 5:352. doi: 10.3389/fmed.2018.00352
20. Olson SR, Murphree CR, Zonies D, Meyer AD, McCarty OJT, Deloughery TG, et al. Thrombosis and bleeding in extracorporeal membrane oxygenation (ECMO) without anticoagulation: a systematic review. ASAIO J. (2021) 67:290–6. doi: 10.1097/MAT.0000000000001230
21. Chan-Dominy AC, Anders M, Millar J, Horton S, Best D, Brizard C, et al. Extracorporeal membrane modality conversions. Perfusion. (2015) 30:291–4. doi: 10.1177/0267659114544486
22. Ushijima T, Sonoda H, Tanoue Y, Shiose A. A therapeutic concept to resolve a possible coronary desaturation under Ecpella support and maximize the potential for myocardial recovery: combination of veno-arteriovenous extracorporeal membrane oxygenation and Impella (VAVEcpella). Perfusion. (2021) 36:535–7. doi: 10.1177/0267659120956540
23. Blandino Ortiz A, Belliato M, Broman LM, Lheureux O, Malfertheiner MV, Xini A, et al. Early findings after implementation of veno-arteriovenous ECMO: a multicenter european experience. Membranes. (2021) 11:81. doi: 10.3390/membranes11020081
24. McGough EC, McGough S, Hawkins JA. Subclavian artery cannulation for infant extracorporeal membrane oxygenation. Ann Thorac Surg. (1993) 55:787–8. doi: 10.1016/0003-4975(93)90301-W
25. Wickline SA, Soeter JR, McNamara JJ. Oxygenation of the cerebral and coronary circulation with right axillary artery perfusion during venoarterial bypass in primates. Ann Thorac Surg. (1977) 24:560–5. doi: 10.1016/S0003-4975(10)63458-3
26. Hou X, Yang X, Du Z, Xing J, Li H, Jiang C, et al. Superior vena cava drainage improves upper body oxygenation during veno-arterial extracorporeal membrane oxygenation in sheep. Crit Care. (2015) 19:68. doi: 10.1186/s13054-015-0791-2
27. Kitamura M, Shibuya M, Kurihara H, Akimoto T, Endo M, Koyanagi H. Effective cross-circulation technique of venoarterial bypass for differential hypoxia condition. Artif Organs. (1997) 21:786–8. doi: 10.1111/j.1525-1594.1997.tb03743.x
28. Lindfors M, Frenckner B, Sartipy U, Bjallmark A, Broome M. Venous cannula positioning in arterial deoxygenation during veno-arterial extracorporeal membrane oxygenation-a simulation study and case report. Artif Organs. (2017) 41:75–81. doi: 10.1111/aor.12700
29. Huber W, Zanner R, Schneider G, Schmid R, Lahmer T. Assessment of regional perfusion and organ function: less and non-invasive techniques. Front Med (Lausanne). (2019) 6:50. doi: 10.3389/fmed.2019.00050
30. Prisco AR, Aguado-Sierra J, Butakoff C, Vazquez M, Houzeaux G, Eguzkitza B, et al. Concomitant respiratory failure can impair myocardial oxygenation in patients with acute cardiogenic shock supported by VA-ECMO. J Cardiovasc Transl Res. (2021). doi: 10.1007/s12265-021-10110-2. [Epub ahead of print].
31. Bloos F, Reinhart K. Venous oximetry. Intensive Care Med. (2005) 31:911–3. doi: 10.1007/s00134-005-2670-9
32. Rivers E, Nguyen B, Havstad S, Ressler J, Muzzin A, Knoblich B, et al. Early goal-directed therapy in the treatment of severe sepsis and septic shock. N Engl J Med. (2001) 345:1368–77. doi: 10.1056/NEJMoa010307
33. Collaborative Study Group on Perioperative Scv OM. Multicentre study on peri- and postoperative central venous oxygen saturation in high-risk surgical patients. Crit Care. (2006) 10:R158. doi: 10.1186/cc5094
Keywords: veno-arterial extracorporeal membrane oxygenation, differential hypoxia, upper body hypoxia, cannulation, oxygenation, cardiogenic shock
Citation: Cai T, Li C, Xu B, Wang L, Du Z, Hao X, Guo D, Xing Z, Jiang C, Xin M, Wang P, Fan Q, Wang H and Hou X (2022) Drainage From Superior Vena Cava Improves Upper Body Oxygenation in Patients on Femoral Veno-Arterial Extracorporeal Membrane Oxygenation. Front. Cardiovasc. Med. 8:807663. doi: 10.3389/fcvm.2021.807663
Received: 02 November 2021; Accepted: 27 December 2021;
Published: 15 February 2022.
Edited by:
Andrew Richard Kolodziej, University of Kentucky, United StatesReviewed by:
Paul Philipp Heinisch, German Heart, GermanyAntonino S. Rubino, University of Campania Luigi Vanvitelli, Italy
Copyright © 2022 Cai, Li, Xu, Wang, Du, Hao, Guo, Xing, Jiang, Xin, Wang, Fan, Wang and Hou. This is an open-access article distributed under the terms of the Creative Commons Attribution License (CC BY). The use, distribution or reproduction in other forums is permitted, provided the original author(s) and the copyright owner(s) are credited and that the original publication in this journal is cited, in accordance with accepted academic practice. No use, distribution or reproduction is permitted which does not comply with these terms.
*Correspondence: Xiaotong Hou, xt.hou@ccmu.edu.cn; Hong Wang, wanghong@mail.ccmu.edu.cn