- Department of Chemistry, Yasouj University, Yasouj, Iran
In this study, magnetic cobalt oxide (Co3O4) nanoparticles (NPs) were synthesized through a new and green method using cobalt chloride hexahydrate (CoCl2.6H2O), pluronic P123 as a stabilizer, and sodium borohydride (NaBH4). The CO3O4 nanoparticles were characterized by diffuse reflectance infrared Fourier transform spectroscopy, powder X-ray diffraction, X-ray photoelectron spectroscopy, energy-dispersive X-ray spectroscopy, scanning electron microscopy, and vibrating sample magnetometer.
The magnetic Co3O4 NPs were used as a catalyst with high activity and stability in the synthesis of tetrahydrobenzo[b]pyran derivatives. This reaction was carried out in water, as it is an environmentally friendly solvent, using a low loading of Co3O4 NPs at room temperature. Various derivatives of aldehydes were used as substrates to obtain a high yield of the corresponding tetrahydrobenzo[b]pyrans in short times. In addition, the catalyst was recovered and reused several times with no notable decrease in its activity.
1 Introduction
In the last decade, magnetic nanoparticles (MNPs) have been noted by many researchers owing to their advantages of high stability, magnetic and electrical properties, high surface area, and special optical properties (Ichiyanagi et al., 2004; Zhang et al., 2006; Bisht and Rajeev, 2011; Ghasemzadeh et al., 2017; Ramamoorthy and Rajendran, 2017; Yin et al., 2017; Vennela et al., 2019). Among different magnetic nanoparticles, cobalt oxide (Co3O4) NPs are very interesting due to their high chemical stability, good reactivity, high surface area, excellent semiconductivity, easy synthesis, high catalytic performance, and superior magnetic properties. These nanoparticles have many applications, such as in energy storage, sensors, anodic supplies, drug delivery, and catalysis (Kumar et al., 2008; Koza et al., 2012; Wang et al., 2015; Agilandeswari and Rubankumar, 2016; Ivetić et al., 2016; Pourzare et al., 2017; Galini et al., 2018; Shi et al., 2019; Tahanpesar et al., 2019; Mohammadi et al., 2020; Tonelli et al., 2020; Al-Qasmi, 2022; Anele et al., 2022; Bilge et al., 2022; Farrag and Ali, 2022; Mohammadpour-Haratbar et al., 2022; Saeed et al., 2022). The chemical formula of these NPs is Co2+(Co3+)2O4 with a normal spinel structure in which Co2+ ions are in tetrahedral interstices and Co3+ ions are in the octahedral interstices of the cubic close-packed lattice of oxide anions (Salavati-Niasari et al., 2009; Thota et al., 2009). It is believed that the shape and size of NPs affect their properties; thus, the morphology of these nanoparticles must be controlled. Co3O4 NPs have been synthesized in various morphologies such as nanorods (Lou et al., 2007; Meng et al., 2015), nanotubes (Chen et al., 2015; Wang et al., 2019), nanowires (Mahmoud and Al-Agel, 2011; Yao et al., 2012), cubic (Ghiasi et al., 2016; Liu et al., 2020), spheres (Chen et al., 2007; Wang et al., 2017), and mesoporous (Qiu et al., 2014; Zhao et al., 2019). Since Co3O4 NPs and their nanocomposites are resistant to corrosion, they have been used as powerful catalysts in different chemical reactions. Some recent reports in this matter are Co3O4–SiO2 nanocomposites for CO oxidation (Jia et al., 2011), Co3O4@SiO2 NPs for the preparation of 3,4-dihydroquinoxalins (Ghasemzadeh et al., 2016), Co3O4 for the reduction of 4-nitrophenols (Al Nafiey et al., 2017), Co3O4@SiO2 for the preparation of indazoles (Ghasemzadeh et al., 2017), Co3O4 NPs as a photocatalyst for methylene blue degradation (Vennela et al., 2019), and Co3O4@SiO2 core/shell–nylon as an adsorbent for the removal of Congo red from wastewater (Mohammadi et al., 2020). There are also several methods for the synthesis of these NPs, including sol–gel, chemical pyrolysis, microemulsion, chemical vapor deposition (CVD), coprecipitation, microwave, decomposition of organic precursors, and hydrothermal methods (He et al., 2004; Yoshikawa et al., 2004; Ştefănescu et al., 2008; Ozkaya et al., 2009; Gupta et al., 2011; Alagiri et al., 2013; Salavati-Niasari and Khansari, 2014; Gopinath et al., 2016; Diallo et al., 2017; Izu et al., 2017; Jamil et al., 2018; Tan et al., 2018; Yetim, 2021). However, some of the aforementioned methods face problems of high reaction temperature, high pressure, and the use of expensive precursors. Therefore, designing a novel method to overcome the aforementioned limitations is an important subject in this matter.
On the other hand, multicomponent reactions are one of the very important chemical processes because of their key role in the synthesis of biologically active heterocyclic organic compounds (Fotouhi et al., 2007; Altass et al., 2021; Alshorifi et al., 2022; Altass et al., 2022; El-Yazeed et al., 2022). Tetrahydrobenzo[b]pyrans are one of these compounds that are synthesized via a three-component reaction. They have good biological activity and are used in the pharmaceutical field, cosmetics, agriculture, pigment, etc. (Hekmatshoar et al., 2008). To date, many catalysts have been reported for the preparation of tetrahydrobenzo[b]pyrans. Some of the recently developed catalysts are choline hydroxide-based ionic liquid [Ch][OH] (Hu et al., 2014), nano-structured diphosphate (Na2CaP2O7, DIPH) (Maleki et al., 2016), dihydrogen phosphate-supported silica-coated magnetic nanoparticles (H2PO4–SCMNPs) (Saadati-Moshtaghin and Zonoz, 2017), Fe3O4@Ph–SO3H (Elhamifar et al., 2018), Preyssler heteropoly acid on Ni0.5Zn0.5Fe2O4 magnetic nanoparticles (MNPs) (Javid and Moeinpour, 2018), nickel Schiff base complex immobilized on silica-coated Fe3O4 (Fe3O4@SiO2@NiSB) (Maleki et al., 2020), and Eu/IDA/CPTS@CoFe2O4 (Tamoradi et al., 2020).
In view of the aforementioned characteristics, herein, for the first time, a novel surfactant-assisted method is presented for the preparation of magnetic cobalt oxide nanoparticles. In this method, NaBH4 has been used as a reducing agent and the pluronic P123 surfactant has been employed as a stabilizer. Moreover, the reaction was performed in EtOH at room temperature. The Co3O4 NPs were characterized and employed as a powerful nanocatalyst in the synthesis of tetrahydrobenzo[b]pyrans.
2 Experimental
2.1 General
All chemicals were used as received with no further purification. Pluronic P123 (98%), CoCl2.6H2O (98%), malononitrile (≥99%), and dimedone (95%) were purchased from Sigma-Aldrich. Moreover, aldehydes (≥95%) and sodium borohydride (99%) were purchased from Merck. Powder X-ray diffraction (PXRD) was performed using a Bruker D8 ADVANCE diffractometer (Germany). The morphology of the particles was evaluated using the TESCAN-Vega3 scanning electron microscope (SEM) (Czech Republic). Energy-dispersive X-ray (EDX) spectroscopy was performed using the TESCAN-Vega3 apparatus (Czech Republic). Fourier transform infrared (FT-IR) spectroscopy was recorded on a Bruker Vector 22 spectrometer (Germany). The magnetic properties of the particles were investigated using a vibrating sample magnetometer (VSM) of Meghnatis Daghigh Kavir Co. (Iran). X-ray photoelectron spectroscopy (XPS) was performed using a Thermo Scientific K-Alpha + XPS spectrometer (United States).
2.2 Preparation of Co3O4 nanoparticles
Magnetic Co3O4 nanoparticles were synthesized through the following reduction procedure: CoCl2.6H2O (1.85 mmol; 0.440 g) was added in 15 mL of absolute EtOH while stirring at RT. Then, ethanol-dissolved pluronic P123 (0.2 g in 5 mL EtOH) was added to the aforementioned solution. After complete mixing, NaBH4 (12.9 mmol; 0.487 g) was added, and the resulting combination was stirred for 10 min at RT. The obtained material was magnetically separated and then washed completely with warm EtOH and water to remove pluronic P123 and other impurities. The magnetic Co3O4 NPs were obtained after drying the product at 65°C for 5 h.
2.3 Synthesis of tetrahydrobenzo[b]pyrans using Co3O4 NPs
For this, dimedone (1.0 mmol), aldehyde (1.0 mmol), malononitrile (1.2 mmol), and a Co3O4 catalyst (0.015 g) were mixed in water (8 mL) while stirring at RT. The reaction progress was monitored by TLC. After completing the process, the catalyst was separated using an external magnetic field. Then, hot EtOH was added, and the resulting mixture was put in an ice bath to precipitate the pure product.
2.4 Procedure for the recovery of the Co3O4 NPs in the synthesis of tetrahydrobenzo[b]pyrans
For this, the reaction was performed as explained previously. After finishing the process, monitored by TLC, the catalyst was separated using a magnet. Then, the recovered catalyst was washed with EtOH and reused in the next run under the same conditions as the first run. These steps were repeated, and it was found that the Co3O4 NPs can be recovered and reused several times with no significant decrease in their efficiency.
3 Result and discussion
The water- and air-stable magnetic Co3O4 nanoparticles were easily and rapidly prepared at RT through a novel reduction method in the presence of pluronic P123 as a stabilizer and NaBH4 as a reducing agent (Scheme 1).
The Co3O4 NPs were characterized using FT-IR, PXRD, VSM, EDX, XPS, and SEM techniques. In the FT-IR spectrum (Figure 1) of Co3O4 NPs, the stretching vibration of the Co–O bond was observed at 619 cm−1. The absorption peaks that appeared at 1,636 and 3,416 cm−1 are related to bending and stretching vibrations of OH, respectively. Furthermore, the band at 1,412 cm−1 is for the B–O bond, resulting from the hydrolysis of the borohydride ion (Medina et al., 2019).
The PXRD analysis shows nine peaks with low intensity at 2θ = 19, 32, 35, 37, 45, 56, 60, 65°, and 72° corresponding to the spinel crystalline structure of magnetic Co3O4 NPs, which is in agreement with the previous literature (Figure 2) (He et al., 2004; Merino et al., 2012; Mujtaba et al., 2016; Hu et al., 2018).
The XPS analysis of cobalt oxide nanoparticles clearly shows the presence of Co and O elements (Figure 3). The most significant peaks related to O 1s, Co 2p3/2, and Co 2p1/2 appeared at 529.1, 780.1, and 795.8 eV, respectively. The energy difference between the Co 2p3/2 and Co 2p1/2 splitting is 15.7 eV, indicating the presence of Co2+ and Co3+ in the prepared material. These results are in good agreement with the previous reports and confirm the Co3O4 structure for the prepared material (Nwanya et al., 2017; Qiu et al., 2017; Zhang et al., 2017).
The morphology of the catalyst was studied by SEM. This analysis showed sponge-like spherical particles for the designed material (Figure 4). According to the SEM images, the average size of the designed Co3O4 NPs was about 36 nm.
In the next study, the magnetic properties of Co3O4 NPs were investigated using a vibrating sample magnetometer (VSM). Importantly, herein, two other methods were used to prepare magnetic Co3O4 NPs, and the magnetic properties of the resulting products were compared with those of our method (Figure 5). These methods were a) the milling process (Medina et al., 2019), b) EtOH/NaBH4 without a stabilizer (our test), and c) ETOH/NaBH4 in the presence of pluronic P123 as a stabilizer agent (our method). The VSM analysis showed the products with a magnetization of 28, 33, and 56 for the a, b, and c methods, respectively (Figure 5). This confirms the very good efficiency of our novel procedure to prepare Co3O4 NPs with high magnetic properties. This result is attributed to the key role of pluronic P123 as a surfactant in the stabilization of magnetic NPs during their preparation. It is also important to note that the prepared black Co3O4 NPs were easily separated using an external magnet (Figure 5, inset figure).
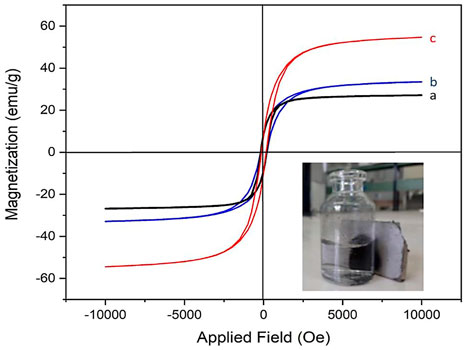
FIGURE 5. VSM diagrams of Co3O4 NPs prepared via (A) the milling process, (B) EtOH/NaBH4, and (C) EtOH/NaBH4/pluronic P123 methods. Magnetic separation of Co3O4 NPs (inset figure).
The EDX analysis was used to distinguish the elements in the structure of the Co3O4 material. This analysis showed the presence of O and Co in the Co3O4 structure (Figure 6). Moreover, the EDX mapping analysis also showed the uniform distribution of these elements in the material network (Figure 7).
After successful characterization, the Co3O4 NPs were used as an efficient catalyst in the synthesis of tetrahydrobenzo[b]pyrans. For this, the reaction between dimedone, benzaldehyde, and malononitrile was selected as a test model, and the effect of different parameters was studied (Table 1). In the absence of a catalyst, no product was found (Table 1, entry 1), while by adding the catalyst, the product yield was increased, and the best result was obtained using 0.015 g of Co3O4 NPs (Table 1, entries 2–4). Screening different solvents showed that a low yield in toluene and acetonitrile, a moderate yield in EtOH and THF, and the highest yield in water were obtained (Table 1, entries 3, 5–8). Increasing temperature from RT to 35°C and 50°C showed no significant change in the product yield (Table 1, entries 3, 9, 10). According to these results, 0.015 g of Co3O4, water solvent, and RT were chosen as optimum conditions (Table 1, entry 3).
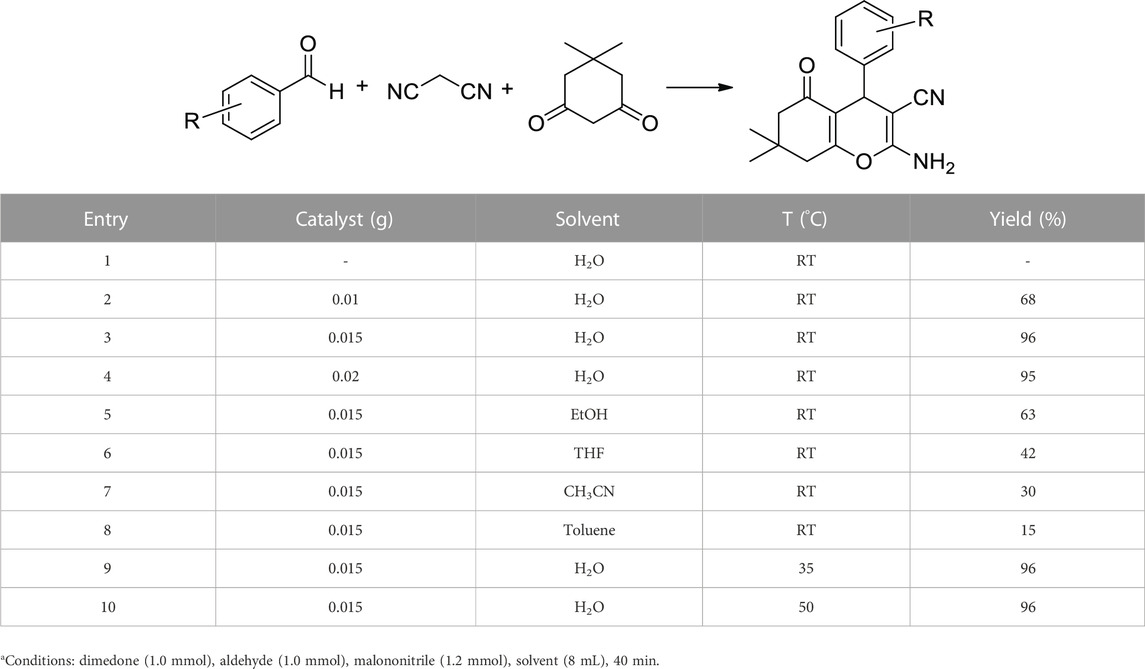
TABLE 1. Effect of catalyst loading, solvent, and temperature in the synthesis of tetrahydrobenzo[b]pyransa.
With the optimal conditions in hand, the catalytic activity of Co3O4 was investigated for different aldehyde substrates to produce the tetrahydrobenzo[b]pyran derivatives. As shown, benzaldehyde (Table 2, entry 1), electron-donating containing aldehydes (Table 2, entries 2–4), and electron-withdrawing bearing aldehydes (Table 2, entry 5) are converted to the corresponding products in high yields at short times. Halogen-substituted aldehydes also delivered a high yield of the corresponding adducts (Table 2, entries 6, 7). In addition, the reaction between 4-nitrobenzaldehyde, malononitrile, and coumarin in the presence of the Co3O4 nanocatalyst also gave a high yield of the corresponding product (Table 2, entry 8). These results confirm the high activity and efficiency of the Co3O4 catalyst for the preparation of a wide range of biologically active tetrahydrobenzo[b]pyrans.
The recoverability and reusability of the designed catalyst were investigated in the reaction model of benzaldehyde, dimedone, and malononitrile under optimal conditions. It was found that the Co3O4 nanocatalyst can be recovered and reused six times with no noticeable decrease in its activity (Figure 8).
Finally, the catalytic activity of the designed Co3O4 nanoparticles was compared with former heterogeneous catalysts in the synthesis of tetrahydrobenzo[b]pyrans (Table 3). It was found that the present catalyst has a high ability to synthesize desired products with higher efficiency and more recovery times than previous catalysts. These findings are attributed to the high surface area, excellent magnetic properties, and good stability of Co3O4 MNPs.
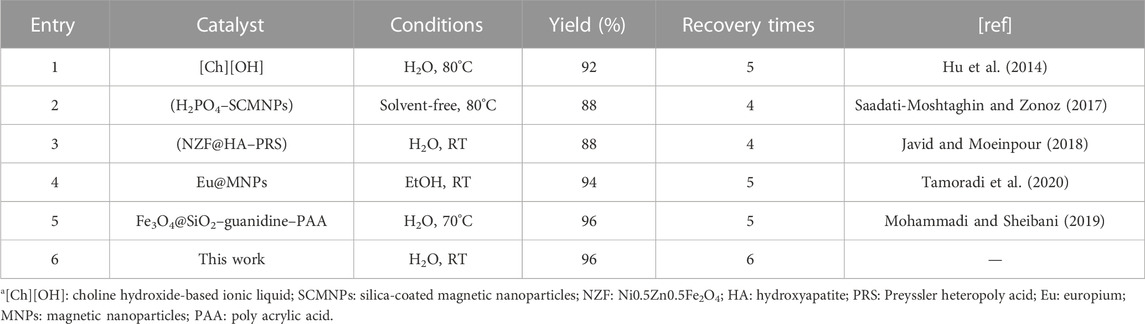
TABLE 3. Comparison of the efficiency of Co3O4 nanoparticles with former catalystsa.
4 Conclusion
In this study, a novel and interesting method for the preparation of magnetic cobalt oxide (Co3O4) NPs was developed. These NPs were synthesized under green conditions at RT in a short time. The FT-IR, PXRD, SEM, VSM, XPS, and EDX analyses confirmed the good preparation, high stability, and good magnetic properties of Co3O4 NPs. These magnetic NPs were used as a powerful and efficient nanocatalyst for the synthesis of tetrahydrobenzo[b]pyrans. The desired products were obtained in water as an environmentally friendly solvent in a short time. The Co3O4 nanocatalyst was separated easily using an external magnet and recovered at least six times with no significant decrease in its activity. Due to the simplicity and eco-friendliness of the designed method, the preparation of other magnetic metal oxide NPs using this strategy is underway in our laboratory.
Data availability statement
The original contributions presented in the study are included in the article/supplementary material; further inquiries can be directed to the corresponding author.
Author contributions
HA: writing—original draft, investigation, resources, and formal analysis. DE: conceptualization, writing—review and editing, supervision, and visualization. All authors listed made a substantial, direct, and intellectual contribution to the work and approved it for publication.
Acknowledgments
The authors acknowledge Yasouj University and the Iran National Science Foundation (INSF) for supporting this work.
Conflict of interest
The authors declare that the research was conducted in the absence of any commercial or financial relationships that could be construed as a potential conflict of interest.
Publisher’s note
All claims expressed in this article are solely those of the authors and do not necessarily represent those of their affiliated organizations, or those of the publisher, the editors, and the reviewers. Any product that may be evaluated in this article, or claim that may be made by its manufacturer, is not guaranteed or endorsed by the publisher.
References
Agilandeswari, K., and Rubankumar, A. (2016). Synthesis, characterization, optical, and magnetic properties of Co3O4 nanoparticles by quick precipitation. Synthesis React. Inorg. Metal-Organic, Nano-Metal Chem. 46, 502–506. doi:10.1080/15533174.2014.988807
Al Nafiey, A., Addad, A., Sieber, B., Chastanet, G., Barras, A., Szunerits, S., et al. (2017). Reduced graphene oxide decorated with Co3O4 nanoparticles (rGO-Co3O4) nanocomposite: A reusable catalyst for highly efficient reduction of 4-nitrophenol, and Cr (VI) and dye removal from aqueous solutions. Chem. Eng. Sci. 322, 375–384. doi:10.1016/j.cej.2017.04.039
Al-Qasmi, N. (2022). Sustainable and efficacy approach of green synthesized cobalt oxide (Co3O4) nanoparticles and evaluation of their cytotoxicity activity on cancerous cells. Molecules 27, 8163–8174. doi:10.3390/molecules27238163
Alagiri, M., Muthamizhchelvan, C., and Hamid, S. B. A. (2013). Synthesis of superparamagnetic cobalt nanoparticles through solvothermal process. J. Mat. Sci. Mat. Electron. 24, 4157–4160. doi:10.1007/s10854-013-1375-z
Alshorifi, F. T., Tobbala, D. E., El-Bahy, S. M., Nassan, M. A., and Salama, R. S. (2022). The role of phosphotungstic acid in enhancing the catalytic performance of UiO-66 (Zr) and its applications as an efficient solid acid catalyst for coumarins and dihydropyrimidinones synthesis. Catal. Commun. 169, 106479–106488. doi:10.1016/j.catcom.2022.106479
Altass, H. M., Khder, A. S., Ahmed, S. A., Morad, M., Alsabei, A. A., Jassas, R. S., et al. (2021). Highly efficient, recyclable cerium-phosphate solid acid catalysts for the synthesis of tetrahydrocarbazole derivatives by Borsche–Drechsel cyclization. React. Kinet. Mech. Catal. 134, 143–161. doi:10.1007/s11144-021-02050-4
Altass, H. M., Morad, M., Khder, A. S., Raafat, M., Alsantali, R. I., Khder, M. A., et al. (2022). Exploitation the unique acidity of novel cerium-tungstate catalysts in the preparation of indole derivatives under eco-friendly acid catalyzed Fischer indole reaction protocol. Arab. J. Chem. 15, 103670–103681. doi:10.1016/j.arabjc.2021.103670
Anele, A., Obare, S., and Wei, J. (2022). Recent trends and advances of Co3O4 nanoparticles in environmental remediation of bacteria in wastewater. Nanomater 12, 1129–1155. doi:10.3390/nano12071129
Bilge, S., Karadurmus, L., Atici, E. B., Sınağ, A., and Ozkan, S. A. (2022). A novel electrochemical sensor based on magnetic Co3O4 nanoparticles/carbon recycled from waste sponges for sensitive determination of anticancer drug ruxolitinib. Sens. Actuators B Chem. 367, 132127. doi:10.1016/j.snb.2022.132127
Bisht, V., and Rajeev, K. (2011). Non-equilibrium effects in the magnetic behavior of Co3O4 nanoparticles. Solid State Commun. 151, 1275–1279. doi:10.1016/j.ssc.2011.05.039
Chen, M., Xia, X., Yin, J., and Chen, Q. (2015). Construction of Co3O4 nanotubes as high-performance anode material for lithium ion batteries. Electrochim. Acta 160, 15–21. doi:10.1016/j.electacta.2015.02.055
Chen, Y., Zhang, Y., and Fu, S. (2007). Synthesis and characterization of Co3O4 hollow spheres. Mat. Lett. 61, 701–705. doi:10.1016/j.matlet.2006.05.046
Diallo, A., Doyle, T., Mothudi, B., Manikandan, E., Rajendran, V., and Maaza, M. (2017). Magnetic behavior of biosynthesized Co3O4 nanoparticles. J. Magn. Magn. 424, 251–255. doi:10.1016/j.jmmm.2016.10.063
El-Yazeed, W. S. A., El-Hakam, S. A., Salama, R. S., Ibrahim, A. A., and Ahmed, A. I. (2022). Ag-PMA supported on MCM-41: Surface acidity and catalytic activity. J. Solgel Sci. Technol. 102, 387–399. doi:10.1007/s10971-022-05755-7
Elhamifar, D., Ramazani, Z., Norouzi, M., and Mirbagheri, R. (2018). Magnetic iron oxide/phenylsulfonic acid: A novel, efficient and recoverable nanocatalyst for green synthesis of tetrahydrobenzo [b] pyrans under ultrasonic conditions. J. Colloid Interface Sci. 511, 392–401. doi:10.1016/j.jcis.2017.10.013
Farahi, M., Karami, B., Keshavarz, R., and Khosravian, F. (2017). Nano-Fe3O4@ SiO2-supported boron sulfonic acid as a novel magnetically heterogeneous catalyst for the synthesis of pyrano coumarins. RSC Adv. 7, 46644–46650. doi:10.1039/c7ra08253c
Farrag, M., and Ali, G. A. (2022). Hydrogen generation of single alloy Pd/Pt quantum dots over Co3O4 nanoparticles via the hydrolysis of sodium borohydride at room temperature. Sci. Rep. 12, 17040. doi:10.1038/s41598-022-21064-z
Fotouhi, L., Heravi, M. M., Fatehi, A., and Bakhtiari, K. (2007). Electrogenerated base-promoted synthesis of tetrahydrobenzo [b] pyran derivatives. Tetrahedron Lett. 48, 5379–5381. doi:10.1016/j.tetlet.2007.06.035
Galini, M., Salehi, M., and Behzad, M. (2018). Structural, magnetic and dielectric properties of pure and Dy-doped Co3O4 nanostructures for the electrochemical evolution of oxygen in alkaline media. J. Nanostructures 8, 391–403.
Ghasemzadeh, M. A., Mirhosseini-Eshkevari, B., and Abdollahi-Basr, M. (2016). Rapid and efficient one-pot synthesis of 3, 4-dihydroquinoxalin-2-amine derivatives catalyzed by Co3O4@ SiO2 core-shell nanoparticles under ultrasound irradiation. Comb. Chem. High. Throughput Screen 19, 592–601. doi:10.2174/1386207319666160524141831
Ghasemzadeh, M., Molaei, B., Abdollahi-Basir, M., and Zamani, F. (2017). Preparation and catalytic study on a novel amino-functionalized silica-coated cobalt oxide nanocomposite for the synthesis of some indazoles. Acta Chim. Slov. 64 (1), 73–82. doi:10.17344/acsi.2016.2823
Ghiasi, M., Malekzadeh, A., and Mardani, H. (2016). Synthesis and optical properties of cubic Co3O4 nanoparticles via thermal treatment of a trinuclear cobalt complex. Comb. Chem. High. Throughput Screen 42, 311–318. doi:10.1016/j.mssp.2015.10.019
Gopinath, S., Sivakumar, K., Karthikeyen, B., Ragupathi, C., and Sundaram, R. (2016). Structural, morphological, optical and magnetic properties of Co3O4 nanoparticles prepared by conventional method. Phys. E Low. Dimens. Syst. Nanostruct. 81, 66–70. doi:10.1016/j.physe.2016.02.006
Gupta, R., Sinha, A., Sekhar, B. R., Srivastava, A., Singh, G., and Deb, S. (2011). Synthesis and characterization of various phases of cobalt oxide nanoparticles using inorganic precursor. Appl. Phys. A . 103, 13–19. doi:10.1007/s00339-011-6311-6
He, T., Chen, D., and Jiao, X. (2004). Controlled synthesis of Co3O4 nanoparticles through oriented aggregation. Chem. Mat. 16, 737–743. doi:10.1021/cm0303033
Hekmatshoar, R., Majedi, S., and Bakhtiari, K. (2008). Sodium selenate catalyzed simple and efficient synthesis of tetrahydro benzo [b] pyran derivatives. Catal. Commun. 9, 307–310. doi:10.1016/j.catcom.2007.06.016
Hu, H., Qiu, F., Ying, A., Yang, J., and Meng, H. (2014). An environmentally benign protocol for aqueous synthesis of tetrahydrobenzo [b] pyrans catalyzed by cost-effective ionic liquid. Int. J. Mol. Sci. 15, 6897–6909. doi:10.3390/ijms15046897
Hu, L., Zhang, G., Liu, M., Wang, Q., and Wang, P. (2018). Enhanced degradation of bisphenol A (bpa) by peroxymonosulfate with Co3O4-Bi2O3 catalyst activation: Effects of pH, inorganic anions, and water matrix. Chem. Eng. J. 338, 300–310. doi:10.1016/j.cej.2018.01.016
Ichiyanagi, Y., Kimishima, Y., and Yamada, S. (2004). Magnetic study on Co3O4 nanoparticles. J. Magn. Magn. 272, E1245–E1246. doi:10.1016/j.jmmm.2003.12.377
Ivetić, T. B., Tadić, M., Jagodič, M., Gyergyek, S., Štrbac, G., and Lukić-Petrović, S. R. (2016). Structure and magnetic properties of Co3O4/SiO2 nanocomposite synthesized using combustion assisted sol-gel method. Ceram. Int. 42, 18312–18317. doi:10.1016/j.ceramint.2016.08.159
Izu, N., Matsubara, I., Uchida, T., Itoh, T., and Shin, W. (2017). Synthesis of spherical cobalt oxide nanoparticles by a polyol method. J. Ceram. Soc. Jpn. 125, 701–704. doi:10.2109/jcersj2.17114
Jamil, S., Janjua, M. R. S. A., and Khan, S. R. (2018). Synthesis and structural investigation of polyhedron Co3O4 nanoparticles: Catalytic application and as fuel additive. Mat. Chem. Phys. 216, 82–92. doi:10.1016/j.matchemphys.2018.05.051
Javid, A., and Moeinpour, F. (2018). Ni0 5Zn0 5Fe2O4@ HA-PRS nanoparticle: A recoverable green catalyst for the synthesis of tetrahydrobenzo [b] pyrans in water. Bull. Chem. Soc. Ethiop. 32, 501–511. doi:10.4314/bcse.v32i3.9
Jia, C.-J., Schwickardi, M., Weidenthaler, C., Schmidt, W., Korhonen, S., Weckhuysen, B. M., et al. (2011). Co3O4–SiO2 nanocomposite: A very active catalyst for CO oxidation with unusual catalytic behavior. J. Am. Chem. Soc. 133, 11279–11288. doi:10.1021/ja2028926
Jin, T.-S., Wang, A.-Q., Wang, X., Zhang, J.-S., and Li, T.-S. (2004). A clean one-pot synthesis of tetrahydrobenzo [b] pyran derivatives catalyzed by hexadecyltrimethyl ammonium bromide in aqueous media. Synlett 2004, 0871–0873. doi:10.1055/s-2004-820025
Koza, J. A., He, Z., Miller, A. S., and Switzer, J. A. (2012). Electrodeposition of crystalline Co3O4 A catalyst for the oxygen evolution reaction. Chem. Mat. 24, 3567–3573. doi:10.1021/cm3012205
Kumar, U., Shete, A., Harle, A. S., Kasyutich, O., Schwarzacher, W., Pundle, A., et al. (2008). Extracellular bacterial synthesis of protein-functionalized ferromagnetic Co3O4 nanocrystals and imaging of self-organization of bacterial cells under stress after exposure to metal ions. Chem. Mat. 20, 1484–1491. doi:10.1021/cm702727x
Liu, Y., Wan, H., Zhang, H., Chen, J., Fang, F., Jiang, N., et al. (2020). Engineering surface structure and defect chemistry of nanoscale cubic Co3O4 crystallites for enhanced lithium and sodium storage. ACS Appl. Nano Mat. 3, 3892–3903. doi:10.1021/acsanm.0c00614
Lou, X., Han, J., Chu, W., Wang, X., and Cheng, Q. (2007). Synthesis and photocatalytic property of Co3O4 nanorods. Mat. Sci. Eng. B 137, 268–271. doi:10.1016/j.mseb.2006.12.002
Mahmoud, W. E., and Al-Agel, F. (2011). A novel strategy to synthesize cobalt hydroxide and Co3O4 nanowires. J. Phys. Chem. Solids 72, 904–907. doi:10.1016/j.jpcs.2011.04.014
Maleki, B., Nasiri, N., Tayebee, R., Khojastehnezhad, A., and Akhlaghi, H. A. (2016). Green synthesis of tetrahydrobenzo [b] pyrans, pyrano [2, 3-c] pyrazoles and spiro [indoline-3, 4′-pyrano [2, 3-c] pyrazoles catalyzed by nano-structured diphosphate in water. RSC Adv. 6, 79128–79134. doi:10.1039/c6ra15800e
Maleki, H., Rakhtshah, J., and Shaabani, B. (2020). Effective one-pot synthesis of tetrahydrobenzo [b] pyran derivatives using nickel Schiff-base complex immobilized on iron oxide nanoparticles. Appl. Organomet. Chem. 34, e5683. doi:10.1002/aoc.5683
Medina, B., Fressati, M. G. V., Gonçalves, J. M., Bezerra, F. M., Scacchetti, F. a. P., Moisés, M. P., et al. (2019). Solventless preparation of Fe3O4 and Co3O4 nanoparticles: A mechanochemical approach. Mat. Chem. Phys. 226, 318–322. doi:10.1016/j.matchemphys.2019.01.043
Meng, T., Xu, Q.-Q., Wang, Z.-H., Li, Y.-T., Gao, Z.-M., Xing, X.-Y., et al. (2015). Co3O4 nanorods with self-assembled nanoparticles in queue for supercapacitor. Electrochim. Acta 180, 104–111. doi:10.1016/j.electacta.2015.08.085
Merino, M. G., Palermo, M., Belda, R., De Rapp, M. F., Lascalea, G., and Vázquez, P. (2012). Combustion synthesis of Co3O4 nanoparticles: Fuel ratio effect on the physical properties of the resulting powders. Procedia Mat. Sci. 1, 588–593. doi:10.1016/j.mspro.2012.06.079
Mohammadi, A. A., Asghariganjeh, M. R., and Hadadzahmatkesh, A. (2017). Synthesis of tetrahydrobenzo [b] pyran under catalysis of NH4Al (SO4) 2· 12H2O (Alum). Arab. J. Chem. 10, S2213–S2216. doi:10.1016/j.arabjc.2013.07.055
Mohammadi, P., and Sheibani, H. (2019). Synthesis and characterization of Fe3O4@ SiO2 guanidine-poly acrylic acid nanocatalyst and using it for one-pot synthesis of 4H-benzo [b] pyrans and dihydropyrano [c] chromenes in water. Mat. Chem. Phys. 228, 140–146. doi:10.1016/j.matchemphys.2018.11.058
Mohammadi, S. Z., Safari, Z., and Madady, N. (2020). A novel Co3O4@ SiO2 magnetic nanoparticle-nylon 6 for high efficient elimination of Pb (II) ions from wastewater. Appl. Surf. Sci. 514, 145873. doi:10.1016/j.apsusc.2020.145873
Mohammadpour-Haratbar, A., Mosallanejad, B., Zare, Y., Rhee, K. Y., and Park, S.-J. (2022). Co3O4 nanoparticles embedded in electrospun carbon nanofibers as free-standing nanocomposite electrodes as highly sensitive enzyme-free glucose biosensors. Rev. Adv. Mat. Sci. 61, 744–755. doi:10.1515/rams-2022-0251
Mujtaba, J., Sun, H., Huang, G., Mølhave, K., Liu, Y., Zhao, Y., et al. (2016). Nanoparticle decorated ultrathin porous nanosheets as hierarchical Co3O4 nanostructures for lithium ion battery anode materials. Sci. Rep. 6, 20592–20598. doi:10.1038/srep20592
Nwanya, A. C., Obi, D., Osuji, R. U., Bucher, R., Maaza, M., and Ezema, F. I. (2017). Simple chemical route for nanorod-like cobalt oxide films for electrochemical energy storage applications. J. Solid State Electrochem. 21, 2567–2576. doi:10.1007/s10008-017-3520-8
Ozkaya, T., Baykal, A., Toprak, M. S., Koseoğlu, Y., and Durmuş, Z. (2009). Reflux synthesis of Co3O4 nanoparticles and its magnetic characterization. J. Magn. Magn. 321, 2145–2149. doi:10.1016/j.jmmm.2009.01.003
Pourzare, K., Farhadi, S., and Mansourpanah, Y. (2017). Graphene oxide/Co3O4 nanocomposite: Synthesis, characterization, and its adsorption capacity for removal of organic dye pollutants from water. Acta Chim. Slov. 64, 945–958. doi:10.17344/acsi.2017.3642
Qiu, B., Guo, W., Liang, Z., Xia, W., Gao, S., Wang, Q., et al. (2017). Fabrication of Co 3 O 4 nanoparticles in thin porous carbon shells from metal–organic frameworks for enhanced electrochemical performance. RSC Adv. 7, 13340–13346. doi:10.1039/c6ra28296b
Qiu, D., Bu, G., Zhao, B., Lin, Z., Pu, L., Pan, L., et al. (2014). In situ growth of mesoporous Co3O4 nanoparticles on graphene as a high-performance anode material for lithium-ion batteries. Mat. Lett. 119, 12–15. doi:10.1016/j.matlet.2013.12.083
Ramamoorthy, C., and Rajendran, V. (2017). Effect of surfactants assisted Co3O4 nanoparticles and its structural, optical, magnetic and electrochemical properties. Optik 145, 330–335. doi:10.1016/j.ijleo.2017.07.058
Saadati-Moshtaghin, H. R., and Zonoz, F. M. (2017). Preparation and characterization of magnetite-dihydrogen phosphate as a novel catalyst in the synthesis of tetrahydrobenzo [b] pyrans. Mat. Chem. Phys. 199, 159–165. doi:10.1016/j.matchemphys.2017.06.066
Saeed, S. Y., Raees, L., Mukhtiar, A., Khan, F., Khan, M., Shah, S. K., et al. (2022). Green synthesis of cobalt oxide nanoparticles using roots extract of Ziziphus Oxyphylla Edgew its characterization and antibacterial activity. Mat. Res. Express. 9, 105001. doi:10.1088/2053-1591/ac9350
Salavati-Niasari, M., and Khansari, A. (2014). Synthesis and characterization of Co3O4 nanoparticles by a simple method. C. R. Chim. 17, 352–358. doi:10.1016/j.crci.2013.01.023
Salavati-Niasari, M., Mir, N., and Davar, F. (2009). Synthesis and characterization of Co3O4 nanorods by thermal decomposition of cobalt oxalate. J. Phys. Chem. Solids 70, 847–852. doi:10.1016/j.jpcs.2009.04.006
Salvi, P., Mandhare, A., Sartape, A., Pawar, D., Han, S., and Kolekar, S. (2011). An efficient protocol for synthesis of tetrahydrobenzo [b] pyrans using amino functionalized ionic liquid. C. R. Chim. 14, 878–882. doi:10.1016/j.crci.2011.02.007
Seifi, M., and Sheibani, H. (2008). High surface area MgO as a highly effective heterogeneous base catalyst for three-component synthesis of tetrahydrobenzopyran and 3, 4-dihydropyrano [c] chromene derivatives in aqueous media. Catal. Lett. 126, 275–279. doi:10.1007/s10562-008-9603-5
Shi, X., Quan, S., Yang, L., Shi, G., and Shi, F. (2019). Facile synthesis of magnetic Co3O4/BFO nanocomposite for effective reduction of nitrophenol isomers. Chemosphere 219, 914–922. doi:10.1016/j.chemosphere.2018.12.045
Shirini, F., Langarudi, M. S. N., and Daneshvar, N. (2017). Preparation of a new DABCO-based ionic liquid [H2-DABCO] [H2PO4] 2} and its application in the synthesis of tetrahydrobenzo [b] pyran and pyrano [2, 3-d] pyrimidinone derivatives. J. Mol. Liq. 234, 268–278. doi:10.1016/j.molliq.2017.03.063
Ştefănescu, M., Dippong, T., Stoia, M., and Ştefănescu, O. (2008). Study on the obtaining of cobalt oxides by thermal decomposition of some complex combinations, undispersed and dispersed in SiO2 matrix. J. Therm. Anal. Calorim. 94, 389–393. doi:10.1007/s10973-008-9111-2
Tahanpesar, E., Tavakkoli, H., and Hadikhani, S. (2019). Facile sol-gel synthesis of Co 3 O 4 nanoparticles—an efficient and recyclable catalyst for the synthesis of 1, 2-disubstituted benzimidazoles under solvent-free conditions. Russ. J. Org. Chem. 55, 1217–1222. doi:10.1134/s1070428019080220
Tamoradi, T., Karmakar, B., Kamalzare, M., Bayat, M., Kal-Koshvandi, A. T., and Maleki, A. (2020). Synthesis of Eu (III) fabricated spinel ferrite based surface modified hybrid nanocomposite: Study of catalytic activity towards the facile synthesis of tetrahydrobenzo [b] pyrans. J. Mol. Struct. 1219, 128598. doi:10.1016/j.molstruc.2020.128598
Tan, L., Liu, B., Siemensmeyer, K., Glebe, U., and Böker, A. (2018). Synthesis of polystyrene-coated superparamagnetic and ferromagnetic cobalt nanoparticles. Polymers 10, 1053. doi:10.3390/polym10101053
Thota, S., Kumar, A., and Kumar, J. (2009). Optical, electrical and magnetic properties of Co3O4 nanocrystallites obtained by thermal decomposition of sol–gel derived oxalates. Mat. Sci. Eng. B 164, 30–37. doi:10.1016/j.mseb.2009.06.002
Tonelli, A. M., Venturini, J., Arcaro, S., Henn, J. G., Moura, D. J., Viegas, A. D. C., et al. (2020). Novel core-shell nanocomposites based on TiO2-covered magnetic Co3O4 for biomedical applications. J. Biomed. Mat. Res. Part B Appl. Biomater. 108, 1879–1887. doi:10.1002/jbm.b.34529
Vennela, A., Mangalaraj, D., Muthukumarasamy, N., Agilan, S., and Hemalatha, K. (2019). Structural and optical properties of Co3O4 nanoparticles prepared by sol-gel technique for photocatalytic application. Int. J. Electrochem. Sci. 14, 3535–3552. doi:10.20964/2019.04.40
Wang, D., Yu, Y., He, H., Wang, J., Zhou, W., and Abruna, H. D. (2015). Template-free synthesis of hollow-structured Co3O4 nanoparticles as high-performance anodes for lithium-ion batteries. ACS Nano 9, 1775–1781. doi:10.1021/nn506624g
Wang, J., Wang, C., and Zhen, M. (2019). Template-free synthesis of multifunctional Co3O4 nanotubes as excellent performance electrode materials for superior energy storage. Chem. Eng. Sci. 356, 1–10. doi:10.1016/j.cej.2018.09.014
Wang, Z., Qu, S., Cheng, Y., Zheng, C., Chen, S., and Wu, H. (2017). Facile synthesis of Co3O4 spheres and their unexpected high specific discharge capacity for Lithium-ion batteries. Appl. Surf. Sci. 416, 338–343. doi:10.1016/j.apsusc.2017.04.194
Yao, X., Xin, X., Zhang, Y., Wang, J., Liu, Z., and Xu, X. (2012). Co3O4 nanowires as high capacity anode materials for lithium ion batteries. J. Alloys Compd. 521, 95–100. doi:10.1016/j.jallcom.2012.01.047
Yetim, N. K. (2021). Hydrothermal synthesis of Co3O4 with different morphology: Investigation of magnetic and electrochemical properties. J. Mol. Struct. 1226, 129414. doi:10.1016/j.molstruc.2020.129414
Yin, K., Ji, J., Shen, Y., Xiong, Y., Bi, H., Sun, J., et al. (2017). Magnetic properties of Co3O4 nanoparticles on graphene substrate. J. Alloys Compd. 720, 345–351. doi:10.1016/j.jallcom.2017.05.275
Yoshikawa, H., Hayashida, K., Kozuka, Y., Horiguchi, A., Awaga, K., Bandow, S., et al. (2004). Preparation and magnetic properties of hollow nano-spheres of cobalt and cobalt oxide: Drastic cooling-field effects on remnant magnetization of antiferromagnet. Appl. Phys. Lett. 85, 5287–5289. doi:10.1063/1.1827329
Zhang, T., He, C., Sun, F., Ding, Y., Wang, M., Peng, L., et al. (2017). Co3O4 nanoparticles anchored on nitrogen-doped reduced graphene oxide as a multifunctional catalyst for H2O2 reduction, oxygen reduction and evolution reaction. Sci. Rep. 7, 43638–43711. doi:10.1038/srep43638
Zhang, Z., Chen, X., Zhang, X., and Shi, C. (2006). Synthesis and magnetic properties of nickel and cobalt nanoparticles obtained in DMF solution. Solid State Commun. 139, 403–405. doi:10.1016/j.ssc.2006.06.040
Keywords: magnetic cobalt oxide, nanoparticles, tetrahydrobenzo[b]pyrans, recoverable nanocatalyst, Biologically active compounds
Citation: Ardeshirfard H and Elhamifar D (2023) An efficient method for the preparation of magnetic Co3O4 nanoparticles and the study of their catalytic application. Front. Catal. 3:1194977. doi: 10.3389/fctls.2023.1194977
Received: 27 March 2023; Accepted: 26 April 2023;
Published: 15 May 2023.
Edited by:
Rosa Maria Padilla Paz, Technical University of Denmark, DenmarkReviewed by:
M. P. Pachamuthu, Bannari Amman Institute of Technology (BIT), IndiaReda S. Salama, Delta University for Science and Technology, Egypt
Copyright © 2023 Ardeshirfard and Elhamifar. This is an open-access article distributed under the terms of the Creative Commons Attribution License (CC BY). The use, distribution or reproduction in other forums is permitted, provided the original author(s) and the copyright owner(s) are credited and that the original publication in this journal is cited, in accordance with accepted academic practice. No use, distribution or reproduction is permitted which does not comply with these terms.
*Correspondence: Dawood Elhamifar, d.elhamifar@yu.ac.ir