- 1Laboratorio de Ciências Ambientais, Centro de Biociências e Biotecnologia, Universidade Estadual do Norte Fluminense Darcy Ribeiro, Rio de Janeiro, Brazil
- 2Programa de Pós-Graduação em Ciências Ambientais, Universidade do Estado de Mato Grosso, Centro de Pesquisa de Limnologia, Biodiversidade, Etnobiologia do Pantanal, Cáceres, Mato Grosso, Brazil
- 3Gerência de Biodiversidade e Caraterização Ambiental, Centro de Pesquisas e Desenvolvimento (CENPES), Petróleo Brasileiro (PeTROBRAs), Rio de Janeiro, SA, Brazil
One of the negative effects of linear infrastructures is the formation of barriers to the movements for arboreal wildlife resulting in the reduction in connectivity between populations and thus increasing the risk of extinction. One of the most widely used ways to mitigate these barrier effects is canopy bridges. There is a lack of knowledge about how behavioral differences between species may affect the efficacy of canopy bridges. The purpose of this study was to evaluate the hypothesis that Leontopithecus rosalia (an endangered endemic species) and Callithrix spp. hybrids (introduced invasive species) perceive canopy bridges as sites with risk of predation. We compared the behavior of these species during attempts to cross four canopy bridges installed over oil and gas pipeline right-of-way strips, and another 4 bridges installed inside the forest near the pipeline bridges. The behavior was recorded from 30-second videos obtained from two camera traps installed at each end of the bridges. Of the 1917 crossing events identified, 356 events were randomly chosen for behavioral data collection. Of these, 173 were social group crossing events and 183 individual crossing events. There were few crossings (7.9%) with the two species present. The differences between the species were more quantitative than qualitative and may be related to interspecific differences in vulnerability to predation and anti-predatory tactics. Both species exhibited significantly more behaviors related to perception of risk of predation (vigilance, hesitation, rapid passage) in the pipeline bridges than in the forest bridges. One vigilance measure showed higher occurrence during the first months after installation of the cameras, especially for Callithrix spp., but in general there was little waning of the risk-assessment response. Alarm calls, scent marking and social and feeding behaviors were rare or absent. Low-cost simple canopy bridges may be an effective strategy to overcome the barrier effect of right-of-way strips over gas and oil pipelines. However, the results on perceived risk of predation raise the hypothesis that there may be a cost-benefit ratio between distance to be traveled and risk perception that would lead to a reduction in effectiveness of bridges in greater distance. We discuss the implications for conservation of an endangered species and management of an invasive species.
Introduction
Linear infrastructures such as roads, railways, canals, pipeline right-of-way strips are among the main drivers of deforestation and fragmentation of tropical forests (Laurence et al., 2001; Geneletti, 2006). For arboreal mammals, the negative effects of these structures on population size and persistence are caused by direct mortality and by the barrier effect (Jaeger et al., 2005; Fahrig and Rytwinski, 2009; Dorsey et al., 2015; Santos et al., 2022; Barrientos et al., 2019). The first type is most often associated with collision with vehicles on roads and railways (Malo et al., 2004; Taylor and Goldingay, 2010; Ascensão et al., 2017), and the second is composed of a series of factors that restrict or divert the natural movement of animals over linear infrastructures or areas adjacent to them (Goosem et al., 2010; Barrientos et al., 2019). The combination of mortality and barrier effects can set off a series of negative consequences that can ultimately reduce population viability in fragmented landscapes. Constraints on animal movements could reduce dispersal among populations and consequently lower genetic diversity over time or could restrict animals to smaller home ranges leading to lack of resources or increased competition and consequently lower reproductive success or longevity (Russell et al., 2003; Fahrig, 2007; Taylor and Goldingay, 2012; Niebuhr et al., 2015). Different types of linear structures differ in the type, magnitude and driving factors of these effects (Jaeger et al., 2005; Yokochi and Bencini, 2015; Lucas et al., 2019) and understanding these differences is key to the design of appropriate effective mitigation measures.
One of the measures to mitigate these impacts of linear structures is the installation of canopy bridges (natural or artificial). Canopy bridges are used by a variety of arboreal mammals, with the potential to mitigate the barrier effect, even over small forest gaps (Teixeira et al., 2013; Gregory et al., 2017; Arias et al., 2023; Soanes et al., 2024). However, studies of primates indicate that there are species differences in the propensity to use canopy bridges: some use them readily, for others there is a time lag, and some species do not use them at all (Buss et al., 2022; Flatt et al., 2022; Gregory et al., 2022b). One variable that may affect usage is the design of the bridge. Canopy bridges made of multiple ropes or substrates show significantly more crossings of small mammals than single rope bridges, and those made of natural materials being more effective than artificial bridges (Mitchell et al., 2023; Gregory et al., 2022b; Soanes et al., 2015). The higher efficacy of multi-rope over single-rope canopy bridges has been attributed in part, and hypothetically, to offering more opportunities to avoid predators (Soanes et al., 2015; Gregory et al., 2022a; Mitchell et al., 2023).
Predation has long been considered a variable that can affect the efficacy of wildlife crossing structures (Little et al., 2002). Studies of patterns of temporal overlap among predators and prey on wildlife crossing structures or quantification of predation attempts at the structure (Little et al., 2002; Martinig et al., 2020; Soanes et al., 2015; Ford and Clevenger, 2010) reject the hypothesis that wildlife passages (in general) act as prey traps. However, predation could still be a factor affecting usage of these structures through specific attraction-avoidance relationships among predators and prey (Mata et al., 2020). Studies of predation of small mammals on canopy bridges indicate that the risk is present even though few events are observed (Soanes et al., 2015). The lack of detection of predator-prey interactions may be related to the methodological issues. Most studies with camera traps are not designed to study predation and studies differ widely in aspects of the sampling design (number of cameras, duration of video, inter-image interval) that could affect detection of such interactions. Also, for longer canopy bridges (> 50 meters) the camera traps may only detect a portion of the crossing event (Soanes et al., 2015).
One approach to understanding how predation may affect differential use of canopy bridges is to observe behavior in relation to usage and the perception of predation risk. Behavior of animals near linear infrastructure can change as a result of perceived predation-risk (Gavin and Komers, 2006). Pronghorn antelopes increase vigilance near roads and their risk-avoidance behavior varies with group size, gender, and presence of young. Perception of risk can be approached within the framework of landscapes of fear (Gaynor et al., 2019) if we consider that various ecological changes brought by human activities on and around the locations where canopy bridges are implemented. There are changes in vegetation structure and these changes can shift the perception of predation risk by changing either escape opportunities or the probability of encountering specific predators (Loggins et al., 2019; Dammhahn and Almeling, 2012; McKinney, 2009). For primates, perception of predation risk differs spatially according to forest structure and the probability of encountering a predator (Campos and Fedigan, 2014; Willems and Hill, 2009). Traversing a canopy bridge is tantamount to crossing from an area of dense vegetation (edge effect of fragmentation) to another, through an open canopy where raptors and ambush predators would be at an advantage. Increased human activities, including hunting, around these locations may also increase the perception of predation risk (Darimont et al., 2023; Suraci et al., 2019).
Wildlife crossing structures are new environments for forest animals and novelty in the environment can alter predator-prey interactions and risk-perception (Crane et al., 2024; Greggor et al., 2014). These ecological factors are likely to interact with variation in cognitive abilities among species to result in species differences in the usage of canopy bridges and other crossing structures. Understanding the role of the perception of predation risk in the use of canopy bridges can provide insights into the causes of observed differences in use of these structures by arboreal mammals and pave the way for better bridge design or site selection for implementation.
In this study we compared the behavior of two primate species, native endangered golden lion tamarins (Leontopithecus rosalia) and introduced common marmosets (Callithrix spp.), during crossings of bridges installed over the right-of-way strips over gas and oil pipelines in a highly fragmented watershed of the Atlantic Forest of Brazil. This study was part of a larger study of the effect of these right-of-way strips on arboreal mammals and the efficacy of canopy bridges as a mitigation measure (Ruiz-Miranda et al., 2023 unpublished report). These right-of-way strips have been shown to limit home range and daily movements (but not dispersal) of the endangered golden lion tamarin, albeit the effects are significantly less than those of roads and highways (Lucas et al., 2019; Ruiz-Miranda et al., 2023). We are also interested in the use canopy bridges by the introduced common marmosets, which considered a threat to the conservation of golden lion tamarins (Ruiz-Miranda et al., 2019).
We tested two hypotheses related to predation risk by comparing the behavior of the two species in bridges installed over the right-of-way strip with behavior over similar bridges installed in the interior of the forest: (1) small arboreal primates would perceive canopy bridges over cleared strips of land as places of high risk of predation, (2) that vulnerability to predation would affect the level of perceived risk and consequently in the behavior during crossing of the structure. The first hypothesis predicts that vigilance and other anti-predator behaviors (alarm calls, grouping and traversal speed) would be significantly more frequent when using canopy bridges over the right-of-way strips than when using similar bridges located within the forest, because the former would render individuals more vulnerable to predators by increasing long distance visibility of the moving animal, especially to aerial predators (Boinski et al., 2000).
The second hypothesis predicts more vulnerable species (smaller body size and/or those that use crypsis as an anti-predator strategy) would show behavior compatible with a higher perception of risk. The adults of both species are vulnerable to predation by a variety of medium to large sized raptors, carnivores (such as tayras (Eyra barbara), jaguarundis (Herpailurus yagouaoundi) and ocelots (Leopardus pardalis)) and snakes (Boa constrictors) (Ferrari, 2009; Hankerson and Dietz, 2014); the young are vulnerable to wider variety of raptors and other mammals (Stafford and Ferreira, 1995; Ferrari, 2009). Both species have evolved specific vocalizations and similar responses to aerial and terrestrial predators (Caesar and Zuberbuehler, 2012), but differences in body size and pelage coloration render the marmosets more vulnerable to predation (Stafford and Ferreira, 1995; Ferrari, 2009).
Materials and methods
The golden lion tamarin (Leontopithecus rosalia) is an endangered species endemic to the Atlantic Forest of the state of Rio de Janeiro (Kierulff et al., 2012; Ruiz-Miranda et al., 2019). The marmosets are populations of hybrids of two species of Callithrix (C. jacchus and C. penicillata) introduced to the region and considered a threat to the conservation of golden lion tamarins (Ruiz-Miranda et al., 2019). Golden lion tamarins and marmosets are strikingly different in pelage coloration and body size (Figure 1): tamarins are red orange in color and the adults weight on average 650 grams, whereas marmosets are gray-agouti colored and adults weight 350 grams. These differences likely affect their conspicuousness to visually guided predators such as raptors (Ferrari, 2009).
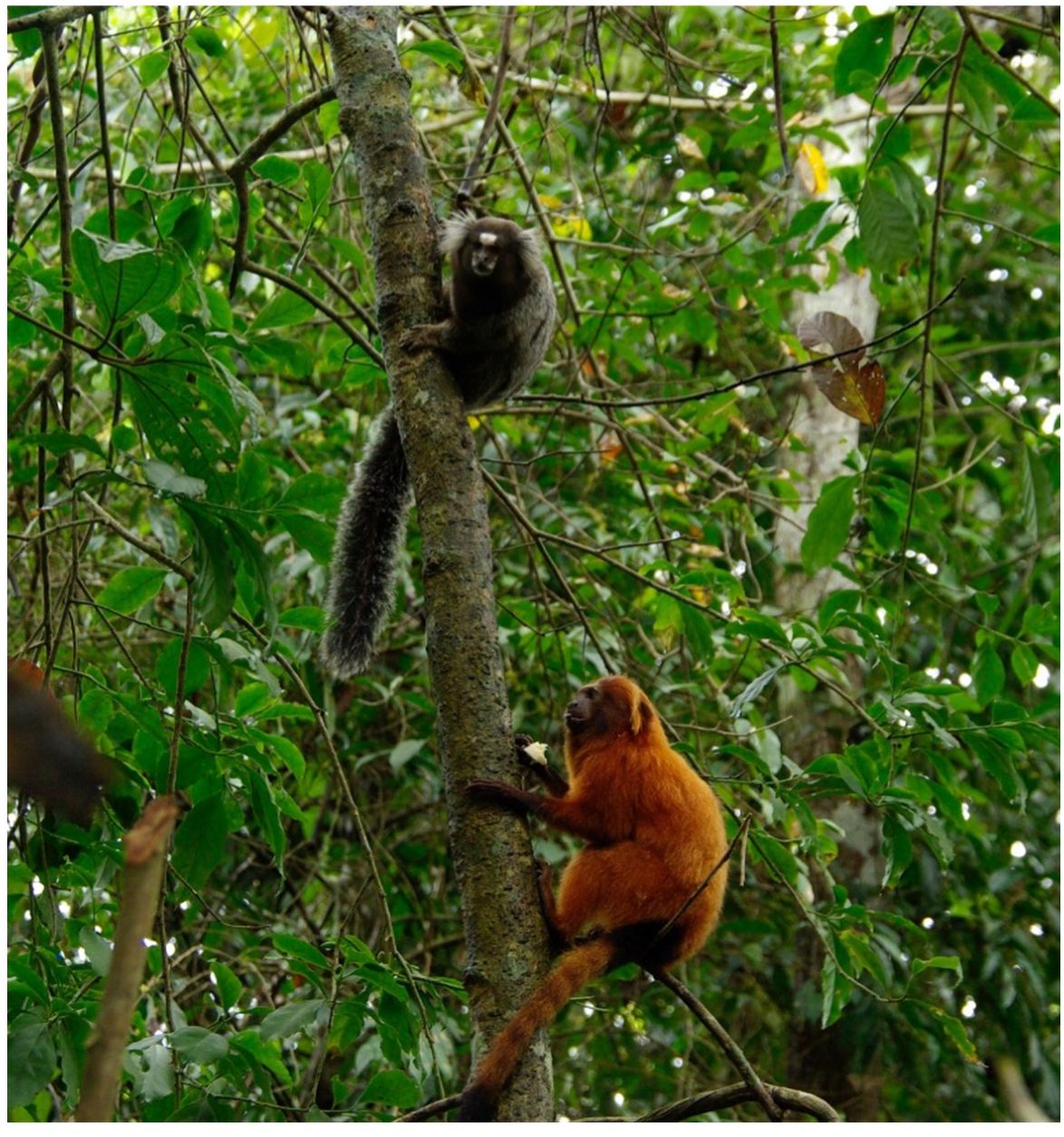
Figure 1. Common marmoset hybrid (top) and golden lion tamarin (bottom) moments before interacting while foraging in a forest fragment within the environmentally protected area of the São João river Basin, Brazil. Photo illustrates the differences in pelage coloration and body size between the species. Photo by Associação Mico Leão Dourado.
The study was conducted in forest fragments (30–200 ha) within the Environmental Protection Area of the São João River Basin/Golden Lion Tamarin (APABRSJ), in the state of Rio de Janeiro, Brazil (22°30´034”S and 42 ° 18´29” W) (Figure 2). The area has a high level of endemic flora and fauna and was decreed a protected area as part of the conservation efforts for the endangered golden lion tamarin (ICMBio, 2008). The climate in the region is humid tropical with a dry winter (Köppen, 1948). The forest vegetation is a mix of lowland and submontane late-stage secondary growth with varying degrees of perturbations (IBGE, 2012). Forests occur mostly on hill slopes and hilltops, whereas lowland areas have been deforested for activities such as agriculture, livestock and urban settlements (ICMBio, 2008). The APABRSJ is traversed by various types of linear infrastructure: high-voltage lines, oil-and-gas pipelines, paved and unpaved roads, a railway, and a federal highway, the BR-101 (Lucas et al., 2019).
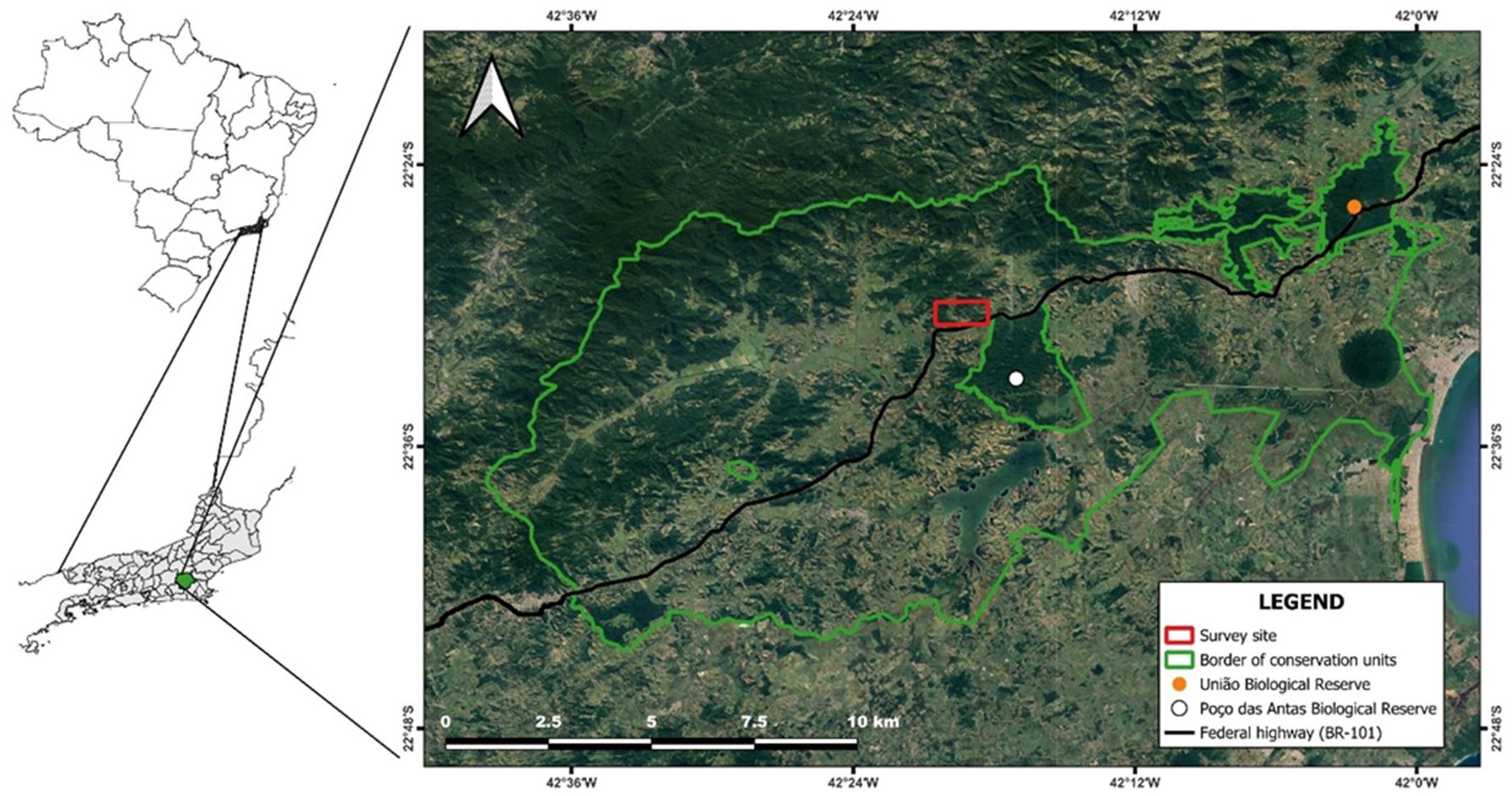
Figure 2. APA - Environmental Protection Area of the São João River Basin/Golden Lion Tamarin. The green line borders the area designated as the protected area. The red square borders the area where the study was conducted.
The oil-gas pipelines in the region are laid 1.5 meters below the surface within a 25-30 meter-wide right of way strip. The right of way area is a deforested strip whose vegetation is a mix of native and introduced grasses (Figure 3). These clearings cause an edge effect which alters vegetation of the forest for up to 10 meters (Arias et al., 2023). We selected four forest fragments within privately owned farms traversed by the pipeline right of way (Figure 4). The selected fragments had monitored groups of golden lion tamarins and unmonitored groups of hybrid marmosets. Most of the golden lion tamarins were individually marked with Nyanzol dye on the body and tail, and at least one animal per group had a radio telemetry collar. These body marks were visible from 20-30 meters. The marmosets had no markings, except for natural variation in pelage, ear tufts and facial characteristics; these natural markings can be used for individual identification (Schiel et al., 2008). Because the bridge in one location (Igarapé farm) was rarely used by both species we excluded it from the data analysis.
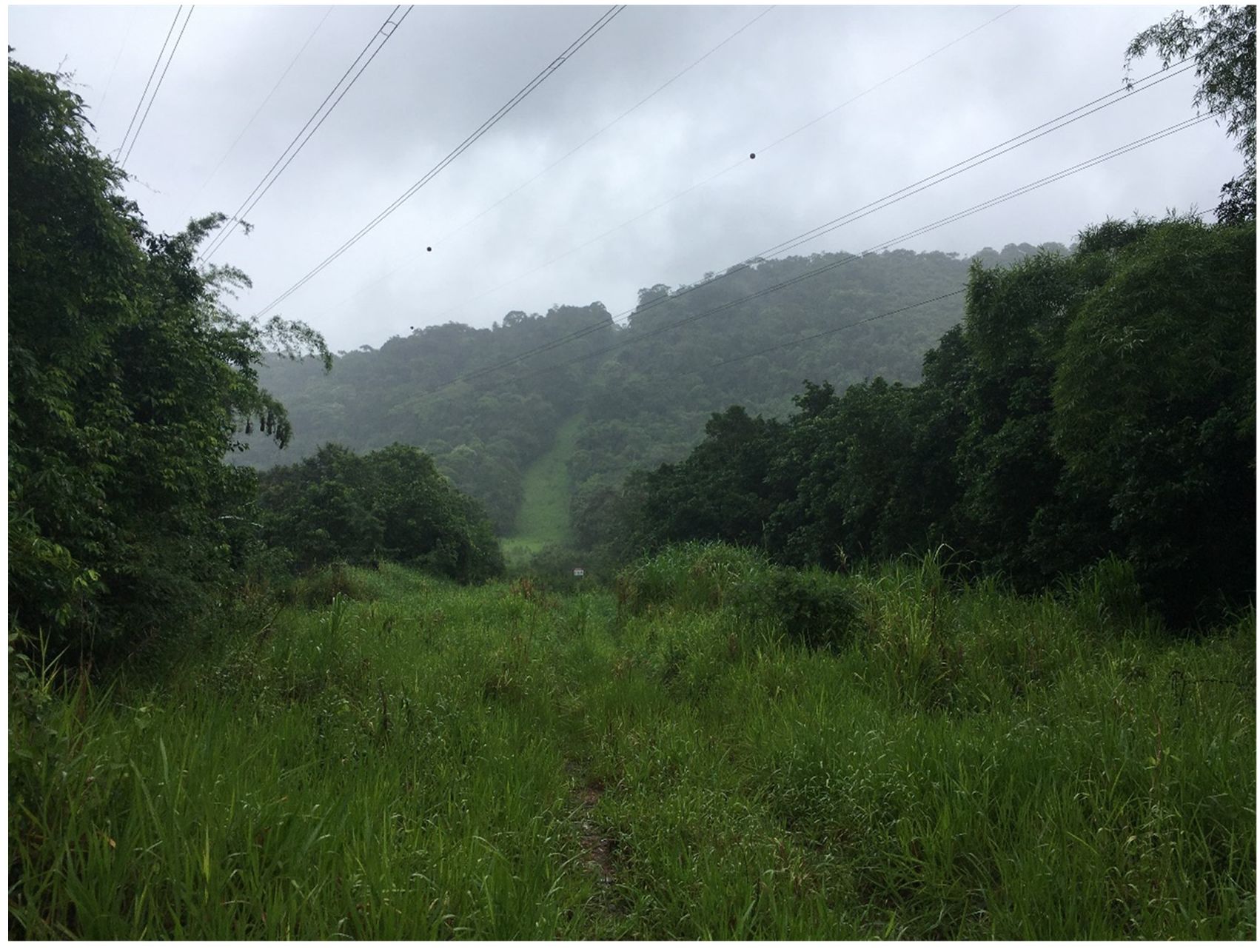
Figure 3. Photo of a right of way strip over the subterranean oil and gas pipeline traversing Haras de Flandria farm. The grassy vegetation over the strip was about 40 cm high at this time. High-tension electric power lines can be seen traversing over the strip.
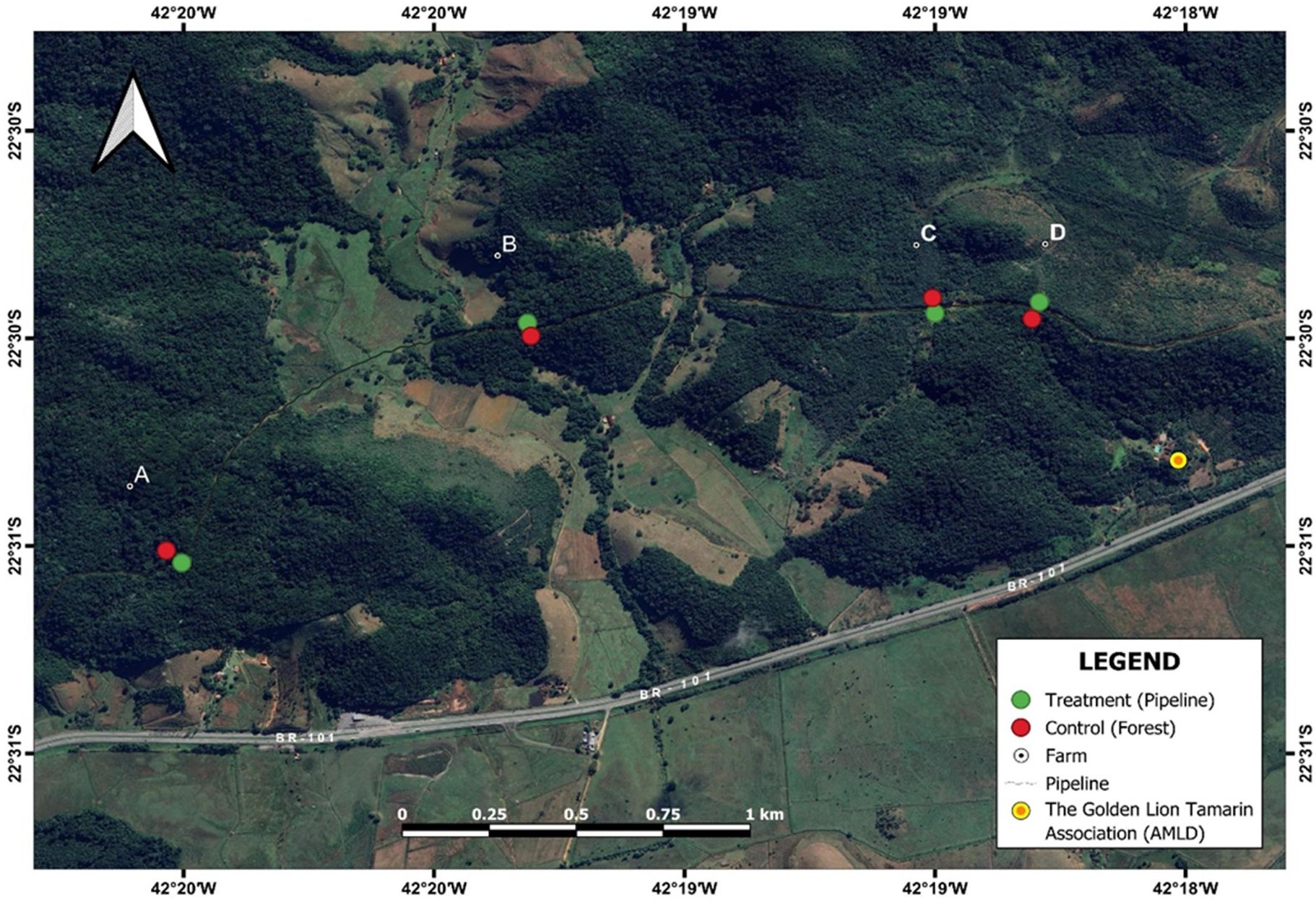
Figure 4. Location of the sites selected for the installation of canopy bridges distributed in four farms in the APA São João River Basin/Golden Lion Tamarin: (A) Dois Irmãos Farm, (B) Iguape Farm, (C) Haras de Flandria Farm, (D) Igarapé Farm.
At each location, we installed a bamboo bridge over the right of way strip (Pipeline Bridge) and another bamboo bridge inside the nearby forest (Forest Bridge) within 20 meters from the Pipeline bridge (Figure 5). The bridges were between 4 and 5 meters above ground level. In choosing the appropriate location for the installation of the bridges, we considered the type of forest and the presence of large trees in good condition near the forest edge to anchor the bridges. The installation was carried out in two phases (Table 1) from 16 September 2019 to 9 April 2020 (Initial Phase) and from 6 July 2020 to 12 November 2020 (Second Phase). In the first phase, four Pipeline bridges were installed over the right of way strip (Pipeline), and in the second phase, the Forest bridges were added to the locations. This timing difference was related to restrictions to enter private properties due to the covid-19 pandemic. As a result of these restrictions sampling effort (days cameras were deployed) varied among locations and experimental conditions (Table 1).
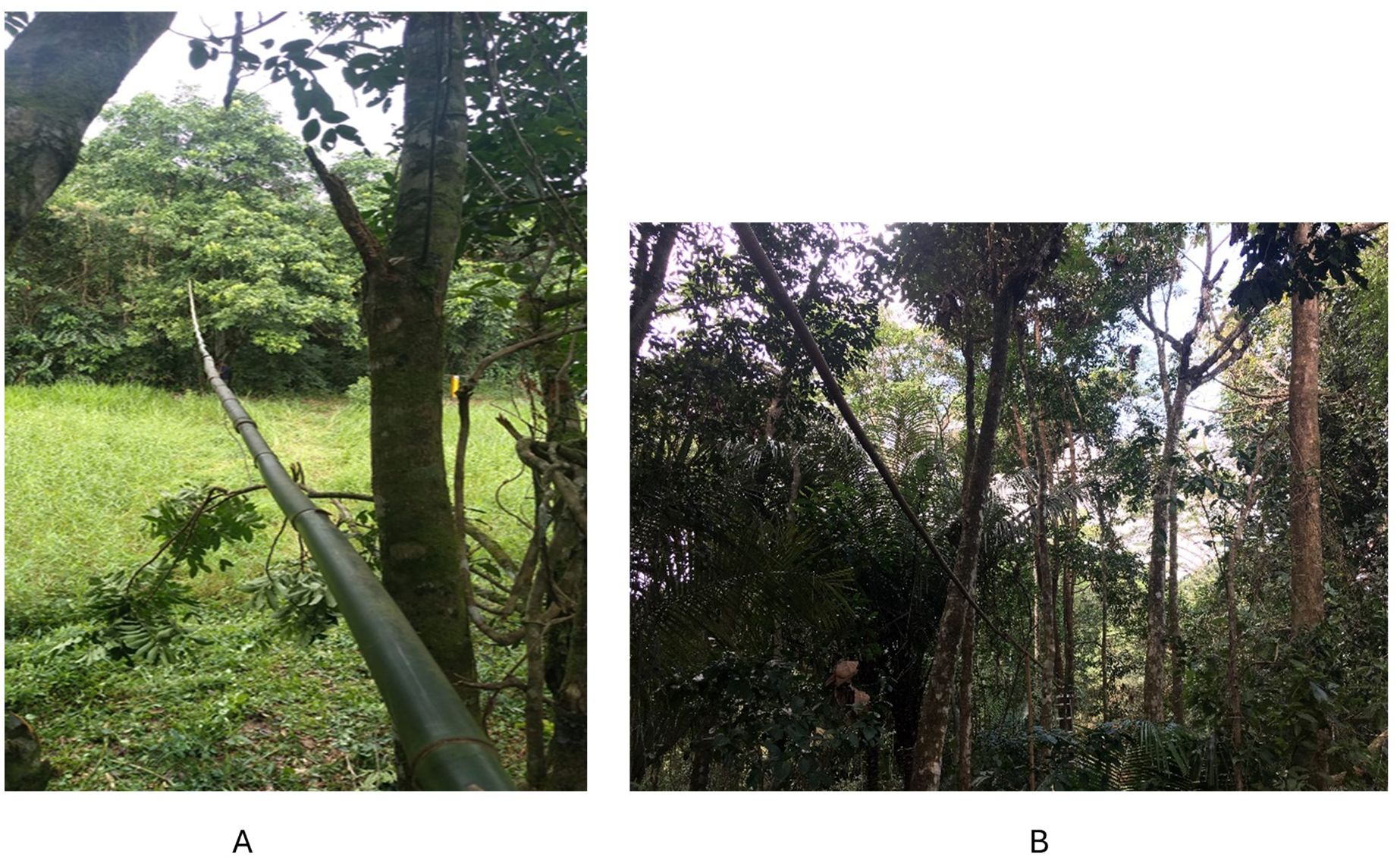
Figure 5. Artificial canopy bridges installed at Fazenda Haras de Flandria. (A) Bridge over the right-of-way strip. Grasses were about 5-10 cm high. (B) Bridge within the forest at the same location.
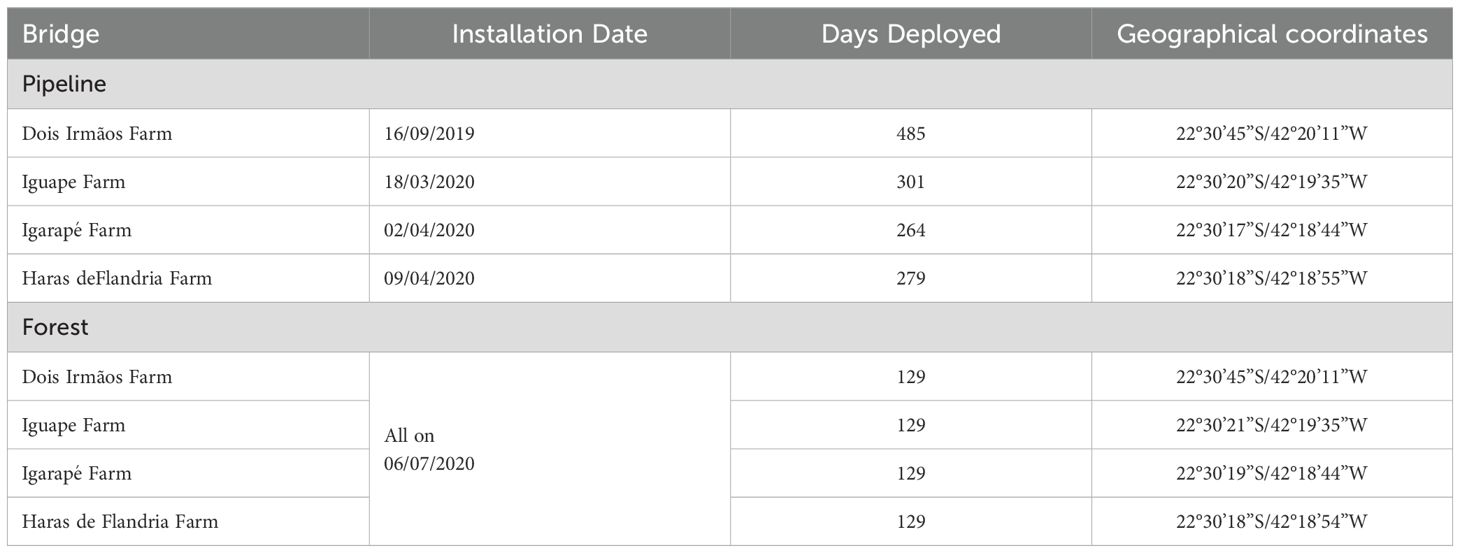
Table 1. Date of installation of the pipeline and forest bridges, and geographical coordinates of the bridges.
Bamboo was used as a natural substrate for the construction of the bridges. The bamboos used belong to the genus Guadua, which have a medium to large woody stem that can reach a height of 30 m and 15 cm in diameter (Figueiras and Viana, 2017). Because the right-of-way strips varied in width (22-27 meters), the bridges over them were made of one (N= 1) or more bamboo stems (N=3). The forest bridges were all made of one bamboo stem. To concatenate the bamboo stems and to tie the ends to the supporting trees, all bridges had 25 mm steel cables passed though the inner part of the bamboo, resulting in a total bridge weight around 30 kg (Figure 5). The ends of the stems were cut so as to fit into each other to form a continuous stem. All bridges were tied to trees with 3.40 mm galvanized wire at a height of 4 to 5 m.
In order to record the use of canopy bridges by primates and collect behavioral data, we positioned the camera traps (Bushnell HD Aggressor Low Glow model 119874) close to the connection point (Figure 6). Twenty motion-sensor-activated camera traps were used in two phases during the study. In the initial phase, 8 camera traps were installed and in the final phase, 12 traps were used, of which 8 were used for the Pipeline bridges and 4 for the Forest bridges. In the pipeline bridges the cameras were placed one on each side facing the bridge, and the control bridges were placed on the south side facing towards the bridge. The difference in the number of cameras in the two types of bridges was due to differences in vegetation and light at the edges between pipeline and forest: we needed cameras at each end for the pipeline, whereas one camera could record everything in the forest.
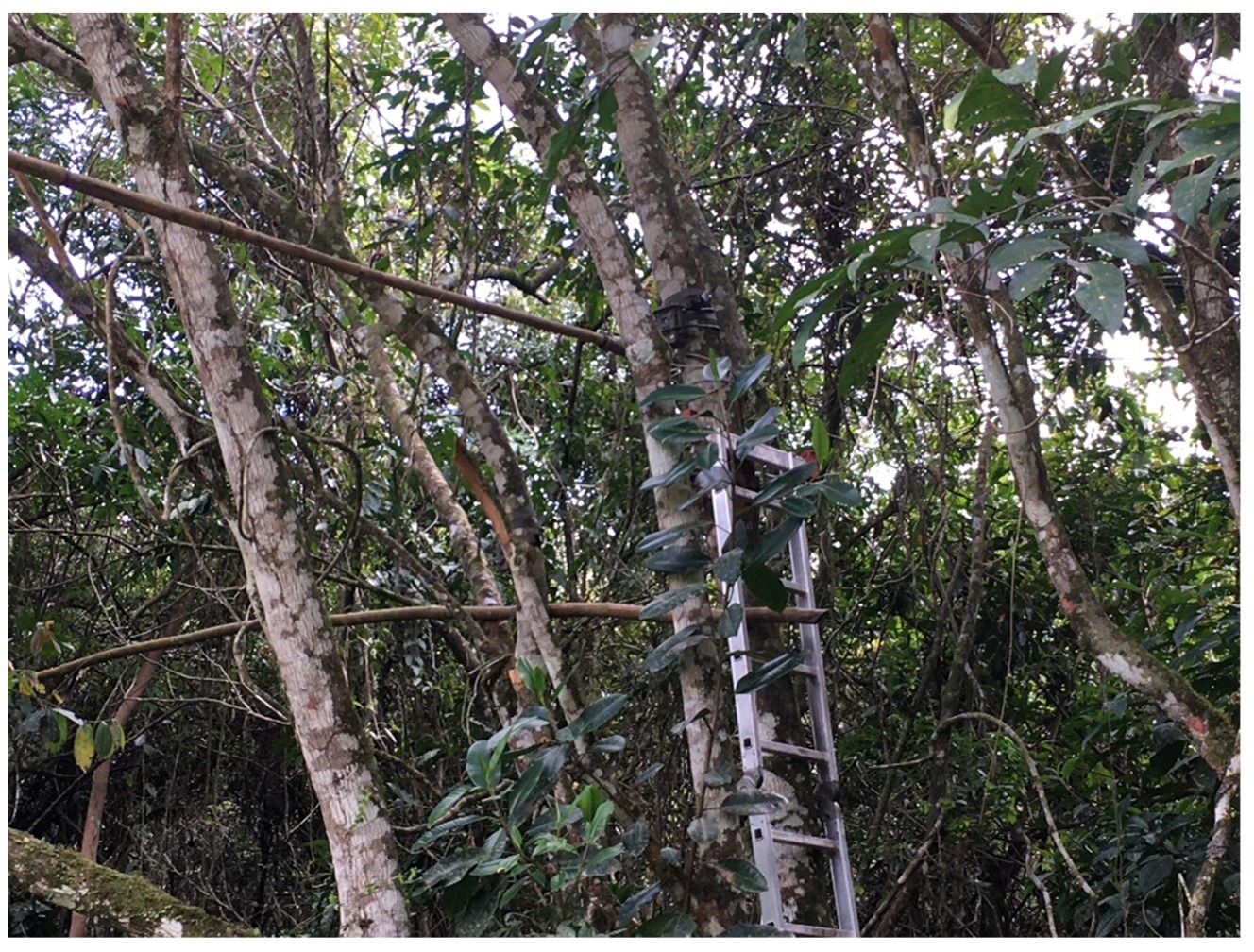
Figure 6. Photo showing the location of the positioning of the camera traps in relation to the bridge and forest.
Camera traps were set up to record videos with a duration of 30 seconds, with an interval of 2 seconds between videos. The cameras were inspected approximately every 20 days when we changed memory cards, checked battery life, and adjusted, if necessary, the positioning of the camera regarding the bridge. Three camera-traps were replaced during the study. Each memory card was identified by a code consisting of the name of the farm and the camera number that indicated the origin of the videos.
The camera traps recorded 5,237 videos with use of the bridges by tamarins and marmosets. Of these, 1,975 videos were from the First phase, and 3,262 were of the Second phase. These videos were compiled into crossing attempts and events. A crossing event was defined as at least one animal from the target species moving completely across the bridge to the opposite side from which it started. If none of the animals crossed completely it was deemed a crossing attempt. Because videos were 30 secs long (with a 2 sec interval before the next), a crossing event could encompass one or more videos of one or both cameras on the same bridge. To compile the videos that make up the crossing event, we considered the time stamps of each video, the size of the group, body size and the presence of a radio collar (in the case of L. rosalia) or other identified marks on the body of the individuals. In the first phase of the study, a total of 285 crossing attempts + events (groups and individuals) were compiled, of which 206 were marmosets and 79 tamarins. For the second phase, we compiled 1,632 crossing attempts+ events (1,382 of marmosets and 250 of tamarins).
These videos were reviewed to identify those from which we could record behavior during a crossing event, either in groups or individually. Videos were assigned a number and chosen at random, observing the criterion that the behavior of the individuals could be observed for the duration they appeared on the cameras on the bridge. We selected for behavioral data analysis a subset of videos from each location. For the first phase, we selected 41 crossing events from tamarins and 39 from marmosets. For the second phase, we selected 125 events for tamarins (42 forest, 83 pipeline) and 151 for marmosets (67 forest, 84 pipeline) from these three locations (Table 2).
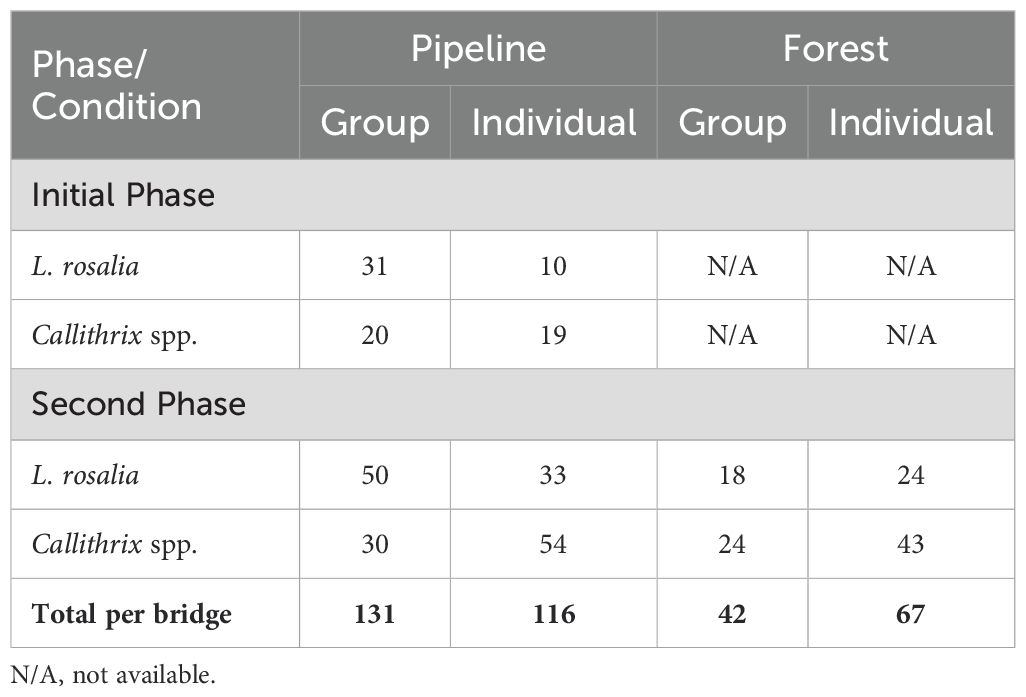
Table 2. Total bridge crossing events recorded by the cameras according to bridge location, species, study phase, and social grouping.
Data were collected from each of the group members from the moment they entered the bridges as recorded by the camera traps. From each crossing event we recorded the time of day and the total duration of the event, number of individuals crossing, their age categories (based on body size), group identify (in the case of L. rosalia) and presence of dependent offspring. We classified the crossing events as being either Group or Individual events, and Mixed when both species were crossing simultaneously or Single species when only one species was present in the crossing event.
For each individual in the videos, we recorded the following behavioral variables:
● Initial Vigilance: Presence or absence of vigilance behavior at the time of the start of the crossing. Vigilance was defined as animal stationary scanning the surroundings by following a visual arch above the horizon to the sides and front (between 120-180 degrees approximately) (Oliveira et al., 2003; Smith et al., 2004).
● Vigilance Events: Number of times each individual exhibited vigilance behavior during the crossing of the bridge. We chose this metric over duration and percent of time vigilant, because the vigilance bouts were of very short duration (events) and longer bouts were truncated often by the end of the 30 second video.
● Crossing speed: This pertains to a locomotion style that could be correlated with actual speed. Scored as Fast (when the animals went from one end to the other of the bridge, stopping no more than twice for less than two seconds each) or Slow/Moderate (when the animals walked or ran with less intensity and stopped to observe more than 3 times for more than 2 seconds each). Stops at or near the edges during the beginning and end of the crossing were not considered.
● Hesitation: An event was scored as showing Hesitation (Presence or Absent) when the leading animal in the video went back and forth between moving forward to cross and returning to the starting side of the bridge. One round of the back and forth was enough to score hesitation.
● Alarm: For each crossing event we scored if alarm vocalizations were Present or Absent. We considered the following to be alarm vocalizations; for tamarins (whine, aerial and terrestrial predator calls, clucks and combinations of clucks-whines, Ruiz-Miranda and Kleiman, 2002) and for marmosets (see, seep, tsak, ek, cough, Stevenson and Rylands, 1988).
● Crossing Order: Position of each individual in the group during the start of the crossing (the first 2 meters of the bridge). Scored as 1 (leading animal), 2 (second), and 3 to ith for the remaining of the animals.
● We also recorded the presence of behavior that could reflect a lack of stress or fear such as social grooming, resting, olfactory marking, or feeding (eating or foraging) (Stevenson and Rylands, 1988; Kleiman et al., 1988).
We sought to infer the strength, and how different explanatory variables affect the perceived risk variables measured in this study. We used a binomial distribution in GLMM (Generalized Linear Mixed Model) to model the response variables crossing speed, initial vigilance, and hesitation, as a function of selected biological and experimental explanatory measures such as species, bridge location (forest versus pipeline), phase (initial versus final) and group size (single versus group). For each response variable, we created different models ranging from reduced models (response variable ~ no explanatory variables) to global models that included a combination of all explanatory variables (variable ~ species + bridge location + phase + group size). We used a Poisson distribution in GLMM as well to model the response variable vigilance events as a function of the same measures above in the same fashion. For all models, the crossing event was assigned as the random effect variable. We built GLMM models using the glmmTMB package with functions in R Software version 4.1 (R Core Team, 2020; Brooks et al., 2017). We used Akaike Information Criterion (AIC) to select the best models among all the models we tested (Zuur et al., 2009). The models with the lowest AIC values have the best support.
In addition, we calculated pseudo R2 values to have a clue on how well adjusted and explicative our models were, since GLMM models do not have R2 metrics like OLS techniques have. We computed an index to calculate pseudo R2 (Efron, 1978) by using the package performance in R (Lüdecke et al., 2021) that is a package dedicated to assessing the performance and quality of regression models.
Chi square tests were calculated to test for significant associations between species, bridge location and behaviors reflecting low level of risk perception that occurred infrequently such as foraging, grooming and scent marking. A test of proportions using the Monte Carlo method was used to test differences in the proportion of crossings by primates that were either by marmoset or tamarins (Lumivero, 2024).
Results
Time lags from pipeline bridge installation to first crossing were between 1 and 30 days for tamarins and 2 and 21 days for marmosets. We compiled 455 pipeline bridge crossings for marmosets (176 during Phase 1 and 279 on Phase 2) and 257 for tamarins (119 for Phase 1 and 138 (Phase 2). Two locations showed similar proportions of the total number of crossing events for tamarins and marmosets (50% and 47 vs.53%), and one location showed a significantly higher proportion of marmoset crossings (75%; χ2 = 46.9, df=2, p<0.0001).
Behavioral analyses of the selected events (356) showed that study phase had little influence on the variables that represent risk perception during Pipeline bridge crossings (Table 3). Initial vigilance was higher during the initial phase of the study for both species (Marmosets Initial= 65.8%, Final 45.8%; Tamarins Initial=72.4%, Final=57.8%), all other variables were not significant for either species.
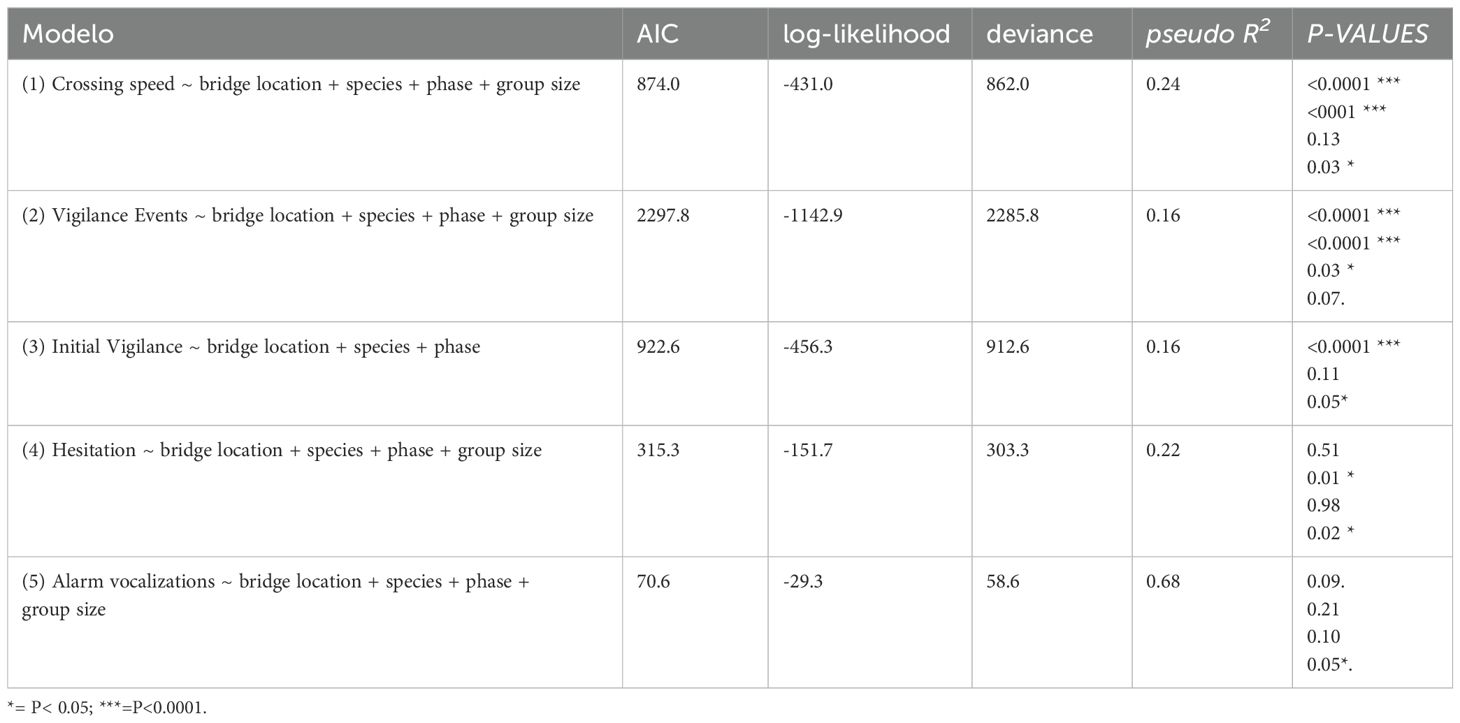
Table 3. Subset of the best models tested through the GLMM models for each response variable of risk perception in primates during fauna crossings in canopy-to-canopy bridges in the APA of the São João River Basin, RJ.
Bridge location had a significant effect on vigilance and crossing speed for both species (Table 3). Tamarins and the marmosets showed significantly more vigilance events (Figure 7), initial vigilance (Figure 8A) and fast speed behavior when crossing pipeline bridges than forest bridges. There were significant differences between species in vigilance events and crossing speed, but not in initial vigilance (Table 3). Tamarins showed significantly more vigilance events, whereas marmosets showed a higher proportion of fast speed crossings.
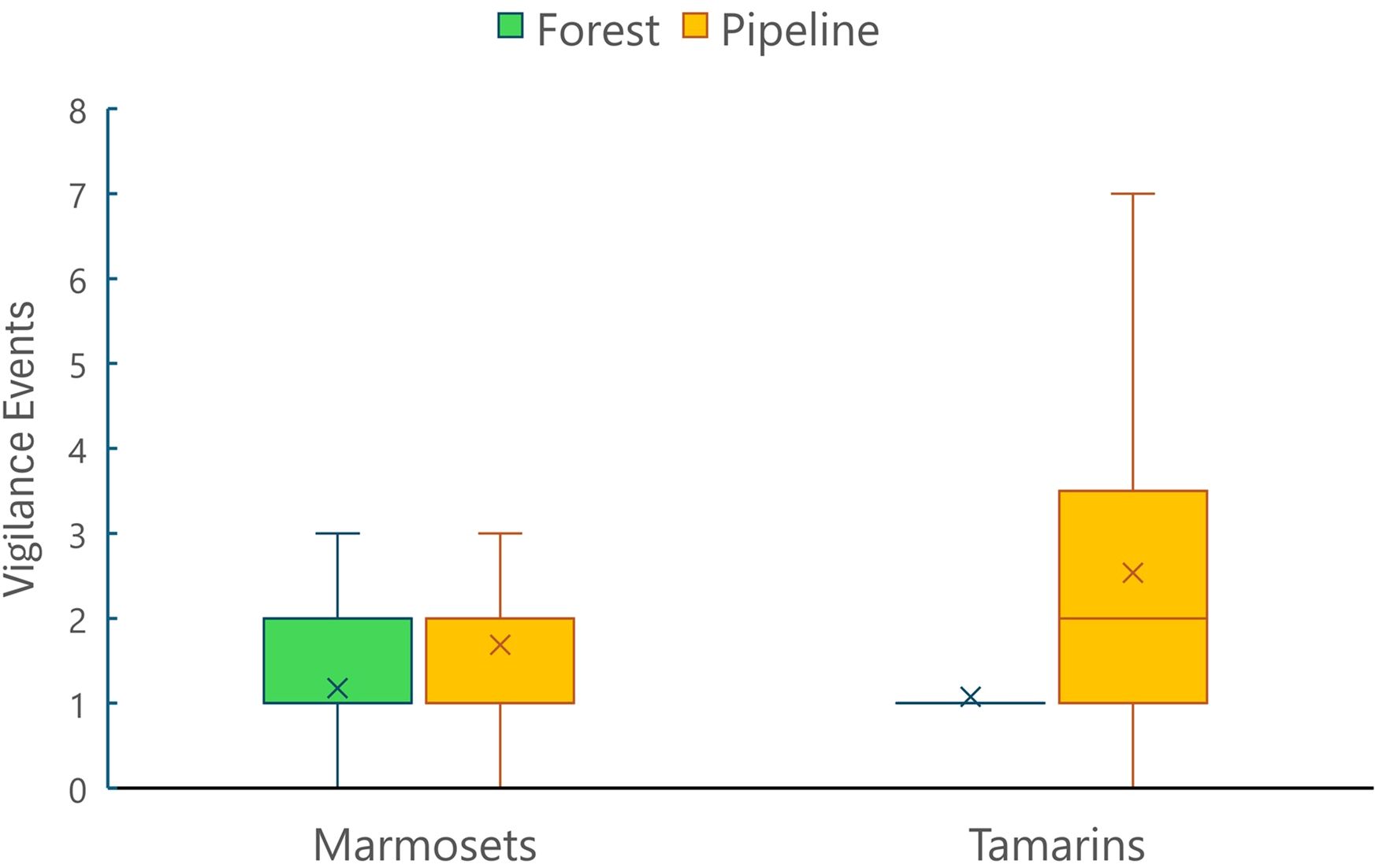
Figure 7. Mean (+) (and standard deviation) number of vigilance events per crossing event for marmosets (Callithrix spp.) and tamarins (Leontopithecus rosalia) over pipeline and forest bridges.
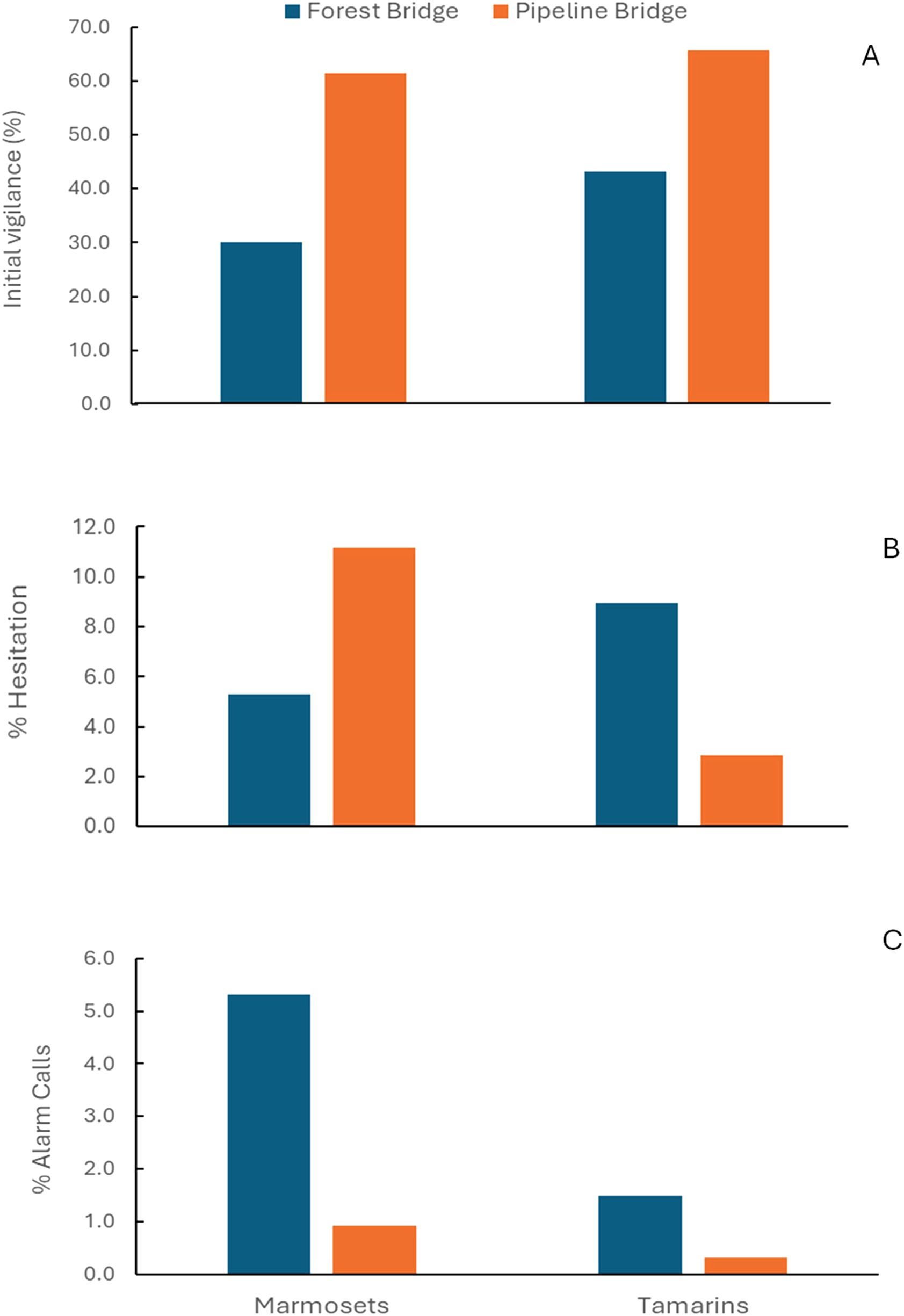
Figure 8. Percentage crossing events of bridges over pipeline strips and within the forest by species in which (A) there was initial vigilance behavior; (B) which individuals showed hesitation to cross; and (C) alarm vocalizations were emitted on bridges.
Bridge location did not have a significant effect on hesitation scores and alarm calls (Table 3). The score for hesitation showed opposite effects of bridge location for tamarins and marmosets resulting in a non-significant overall GLMM model (Figure 8B; Table 3). Marmosets showed hesitance to cross in a higher percentage of crossings over the pipeline bridges relative to the forest bridges, whereas tamarins showed a higher hesitance score in the forest bridges. Alarm calls occurred in less than 6% of the crossing events (Figure 8C) with no significant bridge location effect (Table 3).
A total of 173 group crossing events and 183 individual crossing events were compiled from the videos (Table 2). Tamarins crossed more often in groups (59.6%) than marmosets (38.9%; χ2 = 15.2, p< 0.0001). There was a significant effect of group size for both species on the proportion of events with fast speed (marmoset Group= 61.4%; Individual = 66.6%; Tamarins Group=33.6%, Individual= 40.3%) and hesitation (Marmosets Group= 9.4%; Individual = 8.6%; Tamarins Group=2.2%, Individual= 11.9%). Group size did not have a significant effect on vigilance events, initial vigilance, and emission of alarm calls. In group crossings, the crossing order (first and subsequent animals to cross) had not significant effect in vigilance (Table 3).
Behaviors that would reflect a low level of apprehension were exhibited in a few events, especially by tamarins: grooming (tamarins 4.7%, marmosets 1.2%), and both feeding (0.56%) and scent marking (3.9%) only tamarins. For marmosets, there were no differences in grooming occurrence between pipeline and forest bridges (χ2 = 3.8, p=0.7). For tamarins scent marking occurred significantly more in forest (17.9%) than pipeline bridges (5.1%; χ2 = 13.4, p<0.001). Feeding (Forest= 3%, Pipeline 0.6%, χ2 = 2.9, p=0.09) and grooming (forest=7.5%, pipeline= 4.1%, χ2 = 1.4, p=0.2) also occurred more often in forest and pipeline bridges but the differences were not significant. If these three behaviors are pooled, then the proportion of events in which behaviors reflecting low level of apprehension were exhibited was higher in forest (26.8%) than in pipeline bridges (9.2%; χ2 = 15.9, p<0.0001).
Discussion
Both species crossed the pipeline bridges with high frequencies throughout the study, even though their behavior reflects that they perceive the pipeline bridges as a place of heightened risk of predation. The perception of predation risk was sustained throughout the study, with only a slight waning of vigilance. Behavioral differences between the species during crossings support the hypothesis of different perceptions of risk between tamarins and marmosets. The similar frequency of use of the bridges by both species is not unexpected because these species associate frequently, and the marmosets often follow the tamarins to food sources (Ruiz-Miranda et al., 2006; Lucas et al., 2024).
The differences in behavior in the two types of bridges indicate that both species perceive crossing the open strip over the pipeline a task with risk of predation. As predicted by the hypothesis of increased long-distance visibility (Boinski et al., 2000), all measures of vigilance and crossing speed were significantly higher in the pipeline bridges. For the pipeline bridges, the first (or only) individual showed vigilance at the beginning and during the crossing and movement at fast speed. Within the forest, the middle stratum is the one with the least perceived predation risk (Campos and Fedigan, 2014; de Oliveira et al., 2003). This is where the forest bridges were located. The pipeline bridges were at the level of the middle strata but with open canopies, exposing the monkeys to aerial predators. These species are vulnerable to attack by birds of prey (Lledo-Ferrer et al., 2009). There was no record of predators attacking the primates during the crossings, but an attempt at predation was observed on the Iguape farm when an unidentified hawk attacked a squirrel (Guerlinguetus_ingrami) crossing the bridge over the pipeline. In addition, the raptor (Buteogallus urubitinga) was recorded preying on a coral snake (Micrurus corallinus) in the pipeline strip near the study area (Lucas et al., 2020). Later in the study these bridges were substituted by more elaborate ones, and birds of prey were recorded perching on them. No such observations were made at or near the bamboo bridges within the forest (Ruiz-Miranda et al., 2023). For these primate species, pre-emptive vigilance (done prior to the detection of the predator; Beauchamp, 2017) is the first line of protection against predators (Blanchard et al., 2018; Ferrari, 2009). Capuchins and tamarins increase vigilance in locations within their habitat with increased risk of predation (Campos and Fedigan, 2014; de Oliveira et al., 2003), and tamarins show combination of vigilance and alertness vocalizations (whines) when approaching risky locations such as capture platforms (Lucas et al., 2024). The lack of waning of behavior indicating risk perception over time (initial versus final phase of the study) may just reflect that the level of vigilance seen is the baseline level of predation risk assessment related to moving over open-canopy areas.
There were behavioral differences between lion tamarins and marmosets during bridge crossing events which may reflect how this risk perception is exhibited by species with different vulnerabilities and strategies to avoid predation (Ferrari, 2009). Tamarins tended to cross the bridges as groups and crossed more often using slowe speed behavior. Marmosets tended to cross individually, with fewer vigilance events, more hesitation and using faster locomotion. These differences may be a consequence of differences in risk perception and predator strategies in species with different body size and coat color. Marmosets are smaller and more cryptic in color than the reddish and conspicuous lion tamarins; the former emphasizing camouflage and inconspicuousness, the latter surveillance, detection and alarm (Ferrari, 2009; Stevenson and Rylands, 1988).
Pipeline bridges being a predation-risk site, we expected to observe more group crossings, as the risk of individual predation would be diluted in proportion to group size (Bernstein, 1987; Isbell, 1994; Boinski et al., 2000). Instead, it seems that both tamarins and marmosets use an individual strategy in which an attempt is made to scan the area, then cross silently without stopping, but with other members of the group watching from the edges of the forest, and in this way avoid attacks by an aerial predator that is flying or landing near the bridges. In the case of bridges made of bamboo, the best option could be to pass quickly, as there are not many opportunities for evasive behavior, and heading to the ground offers no protection. Yet, crossing in groups appeared to diminish the perception of risk: it slightly reduced the number of crossings using fast speed for both species and hesitation for tamarins.
Two of the behaviors showed occurrence below what could be expected for these situations: alarm calls and olfactory marking. Marmosets and golden lion tamarins in the act of crossing the bridge were rarely observed emitting alarm vocalizations. Observation of the videos indicate that most of the vocalizations came from other individuals who were in the forest near the bridge. There are three possible explanations for the low detection rate of alarm vocalizations. (1) Bridge crossing is not the kind of situation that elicits alarm vocalizations. Callitrichids emit specific alarm vocalizations to aerial predators and terrestrial predators, but only at the time of sighting of the predator or a cue of the predator (Caesar and Zuberbuehler, 2012; Ruiz-Miranda and Kleiman, 2002). The response to the aerial predator signal is to seek cover, including dropping from the branches toward the ground. For terrestrial predators, the answer is to recruit conspecifics that will mob. These contexts of presence of specific predators rarely occurred in bridges. On one occasion, a group of marmosets were observed reacting agitatedly, emitting multiple vocalizations, some individuals falling to the ground, after an alarm vocalization was emitted by a conspecific in the forest, but the camera did not detect the predator. Leontopithecus emitted mostly the “whine” vocalization, which has a generalized warning function (Ruiz-Miranda and Kleiman, 2002). (2) One hypothesis for the low emission rate is that these vocalizations (of the whine type) would make an animal crossing an open field stand out and easier to detect by a nearby aerial predator. (3) Finally, the low rate of alarm vocalization records may be a consequence of the limitation of this data collection method (Meek et al., 2016). The distance between the camera and the primates may be too long to pick up some sounds, as the primates advance to the opposite side of the pipeline it becomes difficult to recognize whether the alarms are from the individuals themselves crossing or from other individuals within the forest.
We expected to see olfactory marking in the bridges. Olfactory marking is part of primate social behavior and is used for different purposes, such as marking the location of food resources or to defend their territories (Lazaro-Perea et al., 1999; Miller et al., 2003). The marmosets did not show marking in the crossings analyzed. Marmosets mark food sources, such as tree scarifications for exudate extraction, with secretions from their circumgenital scent glands (Epple et al., 1993; Heymann, 2006). The lack of marking on the bridges may just reflect an inappropriate context for displaying this behavior. The golden lion tamarins scent marked with both the sternal and circumgenital glands on both the pipeline and forest bridges. Golden lion tamarins tend to mark substrates within the territory rather than at the edges or in areas of intergroup encounters, which has raised the hypothesis that the function of scent markings is to leave cues for the location of food resources and routine pathways to resources (Miller et al., 2003). In this sense, it is possible that the lion tamarins mark the bridges during the crossing to indicate the path to food resources for the members of the group, being the only appropriate substrate to cross the pipeline strip due to the absence of lianas or trees. The low incidence of marking may be a consequence of the bridges being located within the home range of a single group, thus there is no motivation for territorial marking (Miller et al., 2003). Another hypothesis could be because once the bridge is considered as a site of predation risk, the olfactory marking could lead other members of the group to inspect the smell and consequently putting themselves at risk, or it could be a sign or clue of the presence of prey left for predators. This hypothesis has been postulated to explain why golden lion tamarins avoid marking in places close to sleeping hollows (Franklin et al., 2007a, 2007b).
Finally, another set of results supporting the hypothesis that these species perceive these bridges as a predation-risk area is the absence of behaviors during crossing that would suggest low fear or acclimation such as play, feeding and social behaviors. Neither species fed or engaged in social behaviors during crossings or attempts to cross, even when young animals were present in the groups (often motivated to play). Tamarins avoid playing in forest strata or locations that could be considered risky and adults increase their vigilance, even posting sentinels, when young are at play in any substrate (de Oliveira et al., 2003). Olfactory marking could also be considered as a behavior suggesting low fear for the reasons outlined above.
The results of this study have relevance for the conservation of the golden lion tamarin and similar species in landscapes fragmented by linear structures. Primates can move over the ground, but clearings affect their movements, probably more so for smaller species (Estrada et al., 2017). Previous studies with golden lion tamarins have shown that fragmentation is one driver of genetic population structure and that wildlife bridges over a highway are key to population dynamics and viability (Ascensão et al., 2019; Moraes et al., 2018; Ruiz-Miranda et al., 2019; Dietz et al., 2024). Simple bridges spanning short distances can be used often by callitrichids. Similar results of using bridges over small spans (<25 meters) have been reported for other primate species and small arboreal mammals, albeit with species differences (Chan et al., 2020; Gregory et al., 2013; Teixeira et al., 2013). Low-cost canopy bridges seem to be a viable strategy for crossing open and exposed areas such as those arising from right-of-way strips, small roads or railways, and even aqueducts or canals (usually < 30 meters wide). They allow both special dispersal movements and routine habitat use movements, and consequently result in expansion of the home range or territory of social groups. However, these canopy bridges were perceived by both species as a place of risk. he decision to cross a bridge in open canopy is probably influenced by factors that change the cost-benefit ratio of traversing a high-risk area (e.g. presence of vulnerable young; body condition; resource availability; predation pressure) and these may ultimately affect the frequency of use of the bridge. A resulting hypothesis is that as the distance to be crossed increases, the perception of predation risk increases, which would decrease their use, or at least exacerbate species differences in use, ultimately affecting the achievement of the desired conservation outcomes.
A second point relevant to conservation is that bridges allowed movements of both a native species threatened with extinction and an invasive population of hybrids of two common species. The invasive species is considered a threat to the conservation of golden lion tamarins (de Morais et al., 2008; Ruiz-Miranda et al., 2000, 2019). A long-standing hypothesis is that invasive species are more aggressive and bolder (risk-prone) than related native species (Chapple et al., 2012; Carere and Gherardi, 2013; Damas-Moreira et al., 2019; Putman et al., 2020), and these differences would be more apparent in novel or human-altered environments (Chapple et al., 2012; Hufbauer et al., 2012). Marmosets are considered a highly adaptable invasive species that has successfully occupied both urban and new natural habitats all over Brazil (Malukiewicz et al., 2020). Yet, similar to invasive squirrels and anole lizards (Putman et al., 2020; Twining et al., 2020), they did not behave like risk-takers or any bolder than lion tamarins. Perhaps the invasive success of marmosets relies on the plasticity of their risk-assessment behavior phenotype (see Griffin et al., 2016; Damas-Moreira et al., 2019). It should also be considered that lion tamarins also possess several of these behavioral traits that facilitate dealing with new and anthropogenic environments as evidenced by the success of the reintroduction of captive-born individuals and the urban population of introduced golden headed lion tamarins near the city of Rio de Janeiro (Stoinski and Beck, 2004; Kierulff et al., 2012)
There was no evidence of aggressiveness or direct interference of the invasive species in the use of bridges toward the endangered native species. These two species associate frequently, and agonistic interactions are rare, increasing only during the winter when fruits are scarcer (Ruiz-Miranda et al., 2006). Golden lion tamarins are larger and successfully displace the marmosets from food sources (Ruiz-Miranda et al., 2006; Lucas et al., 2024). The dilemma for conservation is that the bridges facilitate dispersal and population dynamics of the invasive species, thereby mitigating the current negative effects of habitat fragmentation in this region (de Morais et al., 2008; de Morais, 2010; Malukiewicz et al., 2015). More data are needed on the ability of the invasive marmoset species, compared to the endangered golden lion tamarins, to transpose linear structures in order to assess the benefit that bridges represent, and thus evaluate the cost-benefit of this strategy to mitigate the barrier effect of linear structures in golden lion tamarins.
Data availability statement
The raw data supporting the conclusions of this article will be made available by the authors, without undue reservation.
Ethics statement
The animal study was approved by Comite de Ética no Uso de Animais para Pesquisa e ensino (CEUA #420), Universidade Estadual do Norte Fluminense, and permit SISBIO 64635. The study was conducted in accordance with the local legislation and institutional requirements.
Author contributions
LL-R: Conceptualization, Investigation, Methodology, Writing – original draft. PL: Conceptualization, Formal analysis, Writing – original draft, Writing – review & editing. TP: Conceptualization, Funding acquisition, Writing – review & editing, Project administration. CR-M: Conceptualization, Data curation, Formal analysis, Funding acquisition, Methodology, Supervision, Writing – original draft, Writing – review & editing.
Funding
The author(s) declare financial support was received for the research, authorship, and/or publication of this article. Funding came from PETROBRAS/CENPES R, D&I project Landscape Connectivity –Assessment of the effect of pipeline strips on landscape connectivity for mammals and analysis of the effectiveness of fauna crossing structures, coordinated by Dr Talita Aguiaro, through resources from RD&I investments clauses of Brazilian National Agency of Petroleum, Natural Gas and Biofuels (ANP). LL-R received a fellowship from Coordenação de Aperfeiçoamento de Pessoal de Nível Superior (CAPES)-Finance code 001 and CR-M was partially funded by a research productivity fellowship from the Brazilian National Research council (CNPq).
Acknowledgments
The authors thank Bianca Rodrigues for her assistance in sorting and cataloging the videos, and the Associação Mico Leão Dourado for logistic support, especially Andreia Martins, Renato, Josiel Quintanilha and Suelen S Ferreira for help selecting areas and installing the cameras.
Conflict of interest
The authors declare that the research was conducted in the absence of any commercial or financial relationships that could be construed as a potential conflict of interest.
The author(s) declared that they were an editorial board member of Frontiers, at the time of submission. This had no impact on the peer review process and the final decision.
Publisher’s note
All claims expressed in this article are solely those of the authors and do not necessarily represent those of their affiliated organizations, or those of the publisher, the editors and the reviewers. Any product that may be evaluated in this article, or claim that may be made by its manufacturer, is not guaranteed or endorsed by the publisher.
References
Arias J. D. R., Gonçalves P. R., de Oliveira L., Drummond T. A., Rahman F. A. K. A., Ruiz C. R., et al. (2023). Effect of oil pipelines on landscape connectivity for long-furred woolly mouse opossum (Marmosa Paraguayana) in the Brazilian Atlantic Forest. Hystrix Ital. J. Mammalogy. 34, 105–111. doi: doi 10.4404/hystrix-00595-2022
Ascensão F., Lucas P. S., Costa A., Bager A. (2017). The effect of road on edge permeability and movement patterns for small mammals: A case study with Montane Akodont. Landsc. Ecol. 32, 781–790. doi: 10.1007/s10980-017-0485-z
Ascensão F., Niebuhr B. B., Moraes A. M., Alexandre B. R., Assis J. C., Alves‐Eigenheer M. A., et al. (2019). End of the line for the golden lion tamarin? A single road threatens 30 years of conservation efforts. Conservation Science and Practice. doi: 10.1111/csp2.89
Barrientos R., Ascensão F., Beja P., Pereira H. M., Borda-de-Água L. (2019). Railway ecology vs road ecology: Similarities and differences. Eur. J. Wildlife Res. 65, 12. doi: 10.1007/s10344-018-1248-0
Beauchamp G. (2017). What can vigilance tell us about fear? Anim. Sentience 2 (15), 1. doi: 10.51291/2377-7478.1203
Bernstein I. S. (1987). The evolution of nonhuman primate social behavior. Genetics 73, 99–116. doi: 10.1007/BF00057442
Blanchard P., Lauzeral C., Chamaille-Jammes S., Brunet C., Lec’hvien A., Peron G., et al. (2018). Coping with change in predation risk across space and time through complementary behavioral responses. BMC Ecol. 18, 60. doi: 10.1186/s12898-018-0215-7
Boinski S., Treves A., Chapman C. A. (2000). A critical evaluation of the influence of predators on primates: effects on group travel. On the move: How and why animals travel in groups. Eds. Boinski S., Garber P. A. (Chicago: University of Chicago Press), 43–72.
Brooks M. E., Kristensen K., van Benthem K. J., Magnusson A., Berg C. W., Nielsen A., et al. (2017). glmmTMB balances speed and flexibility among packages for zero-inflated generalized linear mixed modeling. R J. 9, 378–400. Available at: https://journal.r-project.org/archive/2017/RJ-2017-066/index.html.
Buss G., Ludwig G., Beltrão-Mendes R., Valença-Montenegro, Mônica M., Martins A. B., et al. (2022). Installation and monitoring of a canopy bridge connecting Atlantic Forest fragments containing endangered species in Northeastern Brazil. Folia Primatologica 93, 493–505. doi: 10.1163/14219980-20211207
Caesar C., Zuberbuehler K. (2012). Referential alarm calling behaviour in New World primates. Curr. Zoology 58, 680–697. doi: 10.1093/czoolo/58.5.680
Campos F. A., Fedigan L. M. (2014). Spatial ecology of perceived predation risk and vigilance behavior in white-faced capuchins. Behav. Ecol. 25, 477–486. doi: 10.1093/beheco/aru005
Carere C., Gherardi F. (2013). Animal personalities matter for biological invasions. Trends Ecol. Evol. 28, 4–5. doi: 10.1016/j.tree.2012.08.019
Chan B. P. L., Lo Y. F. P., Hong X. J., Mak C. F., Ma Z. (2020). First use of artificial canopy bridge by the world’s most critically endangered primate the Hainan gibbon Nomascus hainanus. Sci. Rep. 10, 15176. doi: 10.1038/s41598-020-72641-z
Chapple D. G., Simmonds S. M., Wong B. B. (2012). Can behavioral and personality traits influence the success of unintentional species introductions? Trends Ecol. Evol. 27, 57–64. doi: 10.1016/j.tree.2011.09.010
Crane A. L., Feyten L. E. A., Preagola A. A., Ferrari M. C. O., Brown G. E. (2024). Uncertainty about predation risk: a conceptual review. Biol. Rev. Camb Philos. Soc. 99, 238–252. doi: 10.1111/brv.13019
Damas-Moreira I., Riley J. L., Harris D. J., Whiting M. J. (2019). Can behaviour explain invasion success? A comparison between sympatric invasive and native lizards. Anim. Behav. 151, 195–202. doi: 10.1016/j.anbehav.2019.03.008
Dammhahn M., Almeling L. (2012). Is risk taking during foraging a personality trait? A field test for cross-context consistency in boldness. Anim. Behav. 84, 1131–1139. doi: 10.1016/j.anbehav.2012.08.014
Darimont C. T., Cooke R., Bourbonnais M. L., Bryan H. M., Carlson S. M., Estes J. A., et al. (2023). Humanity’s diverse predatory niche and its ecological consequences. Commun. Biol. 6, 609. doi: 10.1038/s42003-023-04940-w
de Morais M. M. Jr (2010). The invasive marmosets (Callithrix spp., ERXLEBEN 1777) in the São João River basin, Rio de Janeiro: population biology and distribution pattern in a fragmented landscape (Campos dos Goytacazes: State University of Norte Fluminense), 78.
de Morais M. M. Jr., Ruiz Miranda C. R., Grativol A. D., de Andrade C. C., Lima C. S., Martins A., et al. (2008). “The marmosets, Callithirx jacchus and penicillata, as invasive species in the region of occurrence of the golden lion tamarin,” in Golden Lion Tamarin Conservation: Facing the Challenges of a Fragmented Landscape, 3rd ed. Eds. de Oliveira P. P., Grativol A. D., Ruiz Miranda C. R. (RJ: Universidade Estadual do Norte Fluminense, Campos dos Goytacazes), 86–117.
de Oliveira C. R., Ruiz-Miranda C. R., Kleiman D. G., Beck B. B. (2003). Play behavior in juvenile lion tamarins (Callitrichidae: Primates): Organization in relation to costs. Ethology 109, 593–612. doi: 10.1046/j.1439-0310.2003.00901.x
Dietz J. M., Mickelberg J., Traylor-Holzer K., Martins A. F., Souza M. N., Hankerson S. J. (2024). Golden lion tamarin metapopulation dynamics five years after heavy losses to yellow fever. Am. J. Primatol 86, e23635. doi: 10.1002/ajp.23635
Dorsey B., Olsson M., Rew L. J. (2015). “Ecological effects of railways on wildlife,” in Handbook of Road Ecology. Eds. van der Ree R. D., Smith J., Grilo C. (John Wiley & Sons, Hoboken), 219–227.
Efron B. (1978). Regression and ANOVA with zero-one data: Measures of residual variation. J. Am. Stat. Assoc. 73, 113–121. doi: 10.1080/01621459.1978.10480013
Epple G., Belcher A. M., Küderling I., Zeller U., Scolnick L., Greenfield K. L., et al. (1993). “Making sense out of scents: species differences in scent glands, scent-marking behavior, and scent-mark composition in the Callitrichidae,” in Marmosets and Tamarins: Systematics, Behaviour, and Ecology. Ed. Rylands A. B. (Oxford University Press, Oxford), 123–151.
Estrada A., Garber P. A., Rylands A. B., Roos C., Fernandez-Duque E., Di Fiore A., et al. (2017). Impeding extinction crisis of the world's primates: Why primates matter. Sci. Adv. 3, 1–16. doi: 10.1126/sciadv.1600946
Fahrig L. (2007). Non-optimal animal movement in human-altered landscapes. Functional Ecology 21, 6, 1003–1015. doi: 10.1111/j.1365-2435.2007.01326.x
Fahrig L., Rytwinski T. (2009). Effects of roads on animal abundance: An empirical review and synthesis. Ecl. Soc. 14, 21. Available at: http://www.ecologyandsociety.org/vol14/iss1/art21/.
Ferrari S. F. (2009). “Predation risk and antipredator strategies,” in South American Primates: comparative perspectives in the study of behavior, ecology and conservation. Eds. Garber P., Estrada A., Bicca-Marques J. C., Heymann E. W., Strier B. K. (New York: Springer), 251–278.
Figueiras T. S., Viana P. L. (2017). “Brazilian bamboo: morphology, taxonomy, distribution and conservation,” in Bamboos in Brazil: from biology to technology/organization Patricia Maria Drumond, Guilherme Wiedman, vol. 1 . Ed. Hoje C. (Rio de Janeiro: ICH), 28–41.
Flatt E., Basto A., Pinto C., Ortiz J., Navarro K., Reed N., et al. (2022). Arboreal wildlife bridges in the tropical rainforest of Costa Rica’s Osa Peninsula. Folia Primatologica 93, 419–435. doi: 10.1163/14219980-20211109
Ford A. T., Clevenger A. P. (2010). Validity of the prey-trap hypothesis for carnivore-ungulate interactions at wildlife-crossing structures. Conserv. Biol. 24, 1679–1685. doi: 10.1111/j.1523-1739.2010.01564.x
Franklin S. P., Hankerson S. J., Baker A. J. (2007b). Golden lion tamarin sleeping-site use and pre-retirement behavior during intense predation. Am. J. Primatology 69, 325–335. doi: 10.1002/ajp.20340
Franklin S. P., Miller K E., Baker A. J., Dietz J. M. (2007a). Do cavity-nesting primates reduce scent marking before retirement to avoid predators to sleeping sites? Am. J. Primatol 69, 255–266. doi: 10.1002/ajp.20339
Gavin S. D., Komers P. E. (2006). Do pronghorn (Antilocapra americana) perceive roads as a predation risk? Can. J. Zoology 84, 1775–1780. doi: 10.1139/z06-175
Gaynor K. M., Brown J. S., Middleton A. D., Power M. E., Brashares J. S. (2019). Landscapes of fear: spatial patterns of risk perception and response. Trends Ecol. Evol. 34, 355–368. doi: 10.1016/j.tree.2019.01.004
Geneletti D. (2006). Some common shortcomings in the treatment of impacts of linear infrastructures on natural habitat. Environ. Impact. Asses 26, 257–267. doi: 10.1016/j.eiar.2005.10.003
Goosem M., Harding E. K., Chester G., Tucker N., Harriss C., Oakley K. (2010). Roads in rainforest: Best practice guidelines for planning, design and management. Available online at: https://researchonline.jcu.edu.au/12113/1/goosem_guidelines.pdf (Accessed 13 July 2020).
Greggor A. L., Clayton N. S., Phalan B., Thornton A. (2014). Comparative cognition for conservationists. Trends Ecol. Evol. 29, 489–495. doi: 10.1016/j.tree.2014.06.004
Gregory T., Abra F., Linden B., Nekaris K. A. I., Soanes K., Teixeira F. Z. (2022a). A new window into canopy bridges as a mitigation strategy for arboreal mammals. Folia Primatologica 93, 197–203. doi: 10.1163/14219980-930306in
Gregory T., Carrasco-Rueda F., Alonso A., Kolowski J., Deichmann J. L. (2017). Natural canopy bridges effectively mitigate tropical forest fragmentation for arboreal mammals. Sci. Rep. 7, 3892. doi: 10.1038/s41598-017-04112-x
Gregory T., Carrasco-Rueda F., Balbuena D., Kolowski J. (2022b). Rush hour: arboreal mammal activity patterns in natural canopy bridges in the Peruvian Amazon. Folia Primatologica 93, 465–477. doi: 10.1163/14219980-20211209
Gregory T., Carrasco Rueda F., Deichmann J., Kolowski J., Costa Faura M., Dallmeier F., et al. (2013). Methods to establish canopy bridges to increase natural connectivity in linear infrastructure development. Soc. Petroleum Engineers. doi: 10.2118/165598-MS
Griffin A. S., Guez D., Federspiel I., Diquelou M., Lermite F. (2016). Invading new environments: a mechanistic framework linking motor diversity and cognition to establishment success. Biological invasions and animal behaviour. Eds Weis J. S., Sol D. (Cambridge: Cambridge University Press), 26–46.
Hankerson S. J., Dietz J. M. (2014). Predation rate and future reproductive potential explain home range size in golden lion tamarins. Anim. Behav. 96, 87–95. doi: 10.1016/j.anbehav.2014.07.026
Heymann E. W. (2006). Scent marking strategies of New World primates. Am. J. Primatol 68, 650–661. doi: 10.1002/ajp.20258
Hufbauer R. A., Facon B., Ravigne V., Turgeon J., Foucaud J., Lee C. E., et al. (2012). Anthropogenically induced adaptation to invade (AIAI): contemporary adaptation to human-altered habitats within the native range can promote invasions. Evol. Appl. 5, 89–101. doi: 10.1111/j.1752-4571.2011.00211.x
IBGE (2012). Technical Manual of Brazilian Vegetation: Phytogeographic System, Inventory of Forest and Grassland Formations, Techniques and Management of Botanical Collections, Procedures for Mapping, 2nd ed.; Coordination of Natural Resources and Environmental Studies (Rio de Janeiro, Brazil: IBGE), 276.
ICMBio (2008). Management Plan for the Environmental Protection Area of the São João River Basin/Golden Lion Tamarin (Brasilia, Brazil: Chico Mendes Institute for Biodiversity Conservation).
Isbell L. A. (1994). Predation on primates: ecological patterns and evolutionary consequences. Evolutionary Anthropology: Issues News Rev. 3, 61–71. doi: 10.1002/evan.1360030207
Jaeger J. A., Bowman J., Brennan J., Fahrig L., Bert D., Bouchard J., et al. (2005). Predicting when animal populations are at risk from roads: An interactive model of road avoidance behavior. Ecol. Modell 185, 329–348. doi: 10.1016/j.ecolmodel.2004.12.015
Kierulff M. C. M., Ruiz-Miranda C. R., Oliveira P. P., Beck B. B., Martins A., Dietz J. M., et al. (2012). The Golden lion tamarin Leontopithecus rosalia: a conservation success story. Int. Zoo Yearbook 46, 36–45. doi: 10.1111/j.1748-1090.2012.00170.x
Kleiman D. G., Hoage R. J., Green K. M. (1988). “The lion tamarins, Genus Leontopithecus,” in Ecology and behavior of neotropical primates, vol. 2 . Ed. d. Fonseca G. A. B. (New York: World Wildlife Fund), 299–347.
Köppen W. (1948). Climatología: Con un Estudio de los Climas de la Tierra (Pánuco, Mexico: Fondo de Cultura Económica), 478.
Laurence W. F., Cochrane M. A., Bergen S., Fearnside P. M., Delamônica P., Barber C., et al. (2001). The future of the Brazilian amazon. Science 291, 438–439. doi: 10.1126/science.291.5503.438
Lazaro-Perea C., Snowdon C. T., Arruda M. F. (1999). Scent-marking behavior in wild groups of common marmosets (Callithrix jacchus). Behav. Ecol. Sociobiology 46, 313–324. doi: 10.1007/s002650050625
Little S. J., Harcourt R. G., Clevenger A. P. (2002). Do wildlife passages act as prey-traps? Biol. Conserv. 107, 135–145. doi: 10.1016/s0006-3207(02)00059-9
Lledo-Ferrer Y., Hidalgo A., Heymann E. W., Peláez F. (2009). Field Observation of Predation of a Slate-Colored Hawk,Leucopternis schistacea, On a Juvenile Saddle-Back Tamarin,Saguinus fuscicollis. Neotropical Primates 16, 82–84. doi: 10.1896/044.016.0212
Loggins A. A., Shrader A. M., Monadjem A., McCleery R. A. (2019). Shrub cover homogenizes small mammals’ activity and perceived predation risk. Sci. Rep. 9, 16857. doi: 10.1038/s41598-019-53071-y
Lucas P. S., Alves-Eigenheer M., Francisco T. M., Dietz J. M., Ruiz-Miranda C. R. (2019). Spatial response to linear infrastructures by the endangered golden lion tamarin. Diversity 11, 100. doi: 10.3390/d11070100
Lucas P. S., Ruiz Miranda C. R., Alves-Eigenheer M., Francisco T. M., Martins A. F., Soares C. (2024). Assessment of the risk posed by interspecific encounters on baited capture platforms for monitoring an endangered platyrrhine. Int. J. Primatology. 45, 887–912. doi: 10.1007/s10764-024-00424-8
Lüdecke D., Ben-Shachar M. S., Patil I., Waggoner P., Makowski D. (2021). performance: An R package for assessment, comparison and testing of statistical models. J. Open Source Software 6, 3139. doi: 10.21105/joss.03139
Lumivero. (2024). XLSTAT statistical and data analysis solution. Available online at: https://www.xlstat.com/en (Accessed February 02, 2024).
Malo J. E., Suárez F., Díez A. (2004). Can we mitigate animal-vehicle accidents using predictive models? J. Appl. Ecol. 41, 701–710. doi: 10.1111/j.0021-8901.2004.00929.x
Malukiewicz J., Boere V., de Oliveira M. A. B., D’Arc M., Ferreira J. V. A., French J., et al. (2020). An introduction to the callithrix genus and overview of recent advances in marmoset research. ILAR J. 61, 110–138. doi: 10.1093/ilar/ilab027
Malukiewicz J., Boere V., Fuzessy L. F., Grativol A. D. (2015). Natural and Anthropogenic Hybridization in Two Species of Eastern Brazilian Marmosets (Callithrix jacchus and C. penicillata). PloS One. 10, e0127268. doi: 10.1371/journal.pone.0127268
Martinig A. R., Riaz M., St Clair C. C. (2020). Temporal clustering of prey in wildlife passages provides no evidence of a prey-trap. Sci. Rep. 10, 11489. doi: 10.1038/s41598-020-67340-8
Mata C., Herranz J., Malo J. E. (2020). Attraction and avoidance between predators and prey at wildlife crossings on roads. Diversity 12, 166. doi: 10.3390/d12040166
McKinney T. (2009). Anthropogenic change and primate predation risk: crested caracaras (Caracara plancus) attempt predation on mantled howler monkeys (Alouatta palliata). Neotropical Primates 16, 24–27. doi: 10.1896/044.016.0105
Meek P., Ballard G., Fleming P., Falzon G. (2016). Are we getting the full picture? Animal responses to camera traps and implications for predator studies. Ecol. Evol. 6, 3216–3225. doi: 10.1002/ece3.2016.6.issue-10
Miller K. E., Laszlo K., Dietz J. M. (2003). The role of scent marking in the social communication of wild golden lion tamarins, Leontopithecus rosalia. Anim. Behav. 65, 795–803. doi: 10.1006/anbe.2003.2105
Mitchell B., Harrison L., Ainley J., van der Ree R., Soanes K. (2023). Mitigating the effect of linear infrastructure on arboreal mammals in dense forest: A canopy bridge trial. Ecol. Manage. Restor. 23, 228–236. doi: 10.1111/emr.12568
Moraes A. M., Ruiz-Miranda C. R., Galetti P. M. Jr., Niebuhr B. B., Alexandre B. R., Muylaert R. L., et al. (2018). Landscape resistance influences effective dispersal of endangered golden lion tamarins within the Atlantic Forest. Biol. Conserv. 224, 178–187. doi: 10.1016/j.biocon.2018.05.023
Niebuhr B. B. S., Wosniack M. E., Santos M. C., Raposo E. P., Viswanathan G. M., da Luz M. G. E., et al. (2015). Survival in patchy landscapes: the interplay between dispersal, habitat loss and fragmentation. Sci. Rep. 5. doi: 10.1038/srep11898
Oliveira C., Ruiz-Miranda C., Kleiman D., Beck B. (2003). Play behavior in juvenile golden lion tamarins (Callitrichidae: Primates): Organization in relation to costs. Ethology 109, 593–612. doi: 10.1046/j.1439-0310.2003.00901.x
Lucas P.S., Pouble A.G., Ruiz-Miranda C. R. (2020). Micrurus corallinus (Painted Coral Snake) predation. Herpetol. Rev. 51, 148–149.
Putman B. J., Pauly G. B., Blumstein D. T. (2020). Urban invaders are not bold risk-takers: a study of 3 invasive lizards in Southern California. Curr. Zool 66, 657–665. doi: 10.1093/cz/zoaa015
R Core Team. (2020). A: A Language and Environment for Statistical computing (Vienna, Austria: R Foundation for Statistical Computing). Available at: https://www.R-project.org/.
Ruiz-Miranda C., Affonso A., de Morais M., Verona C., Martins A., Beck B. (2006). Behavioral and ecological interactions between reintroduced golden lion tamarins (Leontopithecus rosalia Linnaeus 1766) and introduced marmosets (Callithrix spp., Linnaeus 1758) in Brazil’s Atlantic coast forest fragments. Braz. Arch. Biol. Technol. 49, 99–109. doi: 10.1590/S1516-89132006000100012
Ruiz-Miranda C. R., Affonso A. G., Martins A., Beck B. B. (2000). Distribution of marmoset (Callithrix jacchus) in areas of occurrence of the golden lion tamarin in the State of Rio de Janeiro. Neotropical Primates 8, 98–101. doi: 10.62015/np.2000.v8.455
Ruiz-Miranda C. R., Alves-Eigenheer M., Braga C. A. C., Drummond L. O., Ferreira S. F., Francisco T. M., et al. (2023). Monitoramento de uso e travessia das faixas de dutos, antes e depois da instalação das estruturas de travessia, e do uso e travessia nestas estruturas. Technical report project: Avaliação do efeito de faixas de dutos na conectividade da paisagem para a mastofauna e análise da eficácia de estruturas de travessias de fauna. (Unpublished Report Rio de Janeiro: Petrobras: Petroleo Brasileiro S.A).
Ruiz-Miranda C. R., de Morais M. M. Jr., Dietz L. A., Rocha Alexandre B., Martins A. F., Ferraz L. P., et al. (2019). Estimating population sizes to evaluate progress in conservation of endangered golden lion tamarins (Leontopithecus rosalia). PloS One 14, e0216664. doi: 10.1371/journal.pone.0216664
Ruiz-Miranda C. R., Kleiman D. G. (2002). “Conspicuousness and complexity: themes in lion tamarin communication,” in The Lion Tamarins of Brazil: Twenty-Five Years of Research and Conservation. Ed. Rylands A. (Smithsonian institution Press, Washington, D.C), 256–275.
Russell R. E., Swihart R. K., Feng Z. (2003). Population consequences of movement decisions in a patchy landscape. Oikos 103, 142–152. doi: 10.1034/j.1600-0706.2003.12418.x
Santos E., Cordova M., Rosa C., Rodrigues D. (2022). Hotspots and season related to wildlife roadkill in the amazonia–cerrado transition. Diversity 14 (8), 657. doi: 10.3390/d14080657
Schiel N., Souto A., Bezerra B. M., Huber L. (2008). A stress-free method of identifying common marmosets (Callithrix jacchus) in the wild. A Primatologia no Brasil 9, 147–153.
Smith A. C., Kelez S., Buchanan-Smith H. M. (2004). Factors affecting vigilance within wild mixed-species troops of saddleback (Saguinus fuscicollis) and mustached tamarins (S. mystax). Behav. Ecol. Sociobiology 56, 18–25. doi: 10.1007/s00265-003-0753-6
Soanes K., Rytwinski T., Fahrig L., Huijser M. P., Jaeger J. A. G., Teixeira F. Z., et al. (2024). Do wildlife crossing structures mitigate the barrier effect of roads on animal movement? A global assessment. J. Appl. Ecol. 61, 417–430. doi: 10.1111/1365-2664.14582
Soanes K., Vesk P. A., van der Ree R. (2015). Monitoring the use of road-crossing structures by arboreal marsupials: insights gained from motion-triggered cameras and passive integrated transponder (PIT) tags. Wildlife Res. 42, 241–256. doi: 10.1071/wr14067
Stafford B. J., Ferreira F. M. (1995). Predation attempts on callitrichines in the Atlantic coastal rain forest of Brazil. Folia Primatologica 65, 229–233. doi: 10.1159/000156894
Stevenson M. F., Rylands A. B. (1988). “The marmosets, genus Callithrix,” in Ecology and Behavior of Neotropical Primates. Eds. Mittermeier R. A., Rylands A. B., Coimbra-Filho A. F., Fonseca G. A. B.. (Washington, D. C.: World Wildlife Fund) 2, 131–211.
Stoinski T. S., Beck B. B. (2004). Changes in Locomotor and Foraging Skills in Captive- Born, Reintroduced Golden Lion Tamarins (Leontopithecus rosalia rosalia). Am. J. Of Primatology 62, 1–13. doi: 10.1111/ele.13344
Suraci J. P., Clinchy M., Zanette L. Y., Wilmers C. C. (2019). Fear of humans as apex predators has landscape-scale impacts from mountain lions to mice. Ecol. Lett. 22, 1578–1586. doi: 10.1111/ele.13344
Taylor B. D., Goldingay R. L. (2010). Roads and wildlife: impacts, mitigation and implications for wildlife management in Australia. Wildlife Res. 37, 220-231. doi: 10.1071/wr09171
Taylor B. D., Goldingay R. L. (2012). Facilitated movement over major roads is required to minimise extinction risk in an urban metapopulation of a gliding mammal. Wildlife Res. 39, 685-695. doi: 10.1071/wr12142
Teixeira F. Z., Printes R. C., Fagundes J. C. G., Alonso A. C., Kindel A. (2013). Canopy bridges as road overpasses for wildlife in urban fragmented landscapes. Biota Neotropica 13, 117–123. doi: 10.1590/S1676-06032013000100013
Twining J. P., Montgomery W. I., Tosh D. G. (2020). The dynamics of pine marten predation on red and grey squirrels. Mamm. Biol. 100, 285–293. doi: 10.1007/s42991-020-00031-z
Willems E. P., Hill R. A. (2009). Predator-specific landscapes of fear and resource distribution: effects on spatial range use. Ecology 90, 546–555. doi: 10.1890/08-0765.1
Yokochi K., Bencini R. (2015). A remarkably quick habituation and high use of a rope bridge by an endangered marsupial, the western ringtail possum. Nat. Conserv. 11, 79–94. doi: 10.3897/natureconservation.11.4385
Keywords: predation, alarm calls, linear structures, conservation, anthropic effects, primates, fragmentation, pipelines
Citation: López-Ramirez L, Lucas P, Aguiaro Pereira TdA and Ruiz-Miranda CR (2024) Perception of predation risk by tamarins and marmosets crossing bridges over a pipeline right-of-way strip in the Atlantic forest of Brazil. Front. Conserv. Sci. 5:1473312. doi: 10.3389/fcosc.2024.1473312
Received: 30 July 2024; Accepted: 17 October 2024;
Published: 14 November 2024.
Edited by:
David R. Breininger, University of Central Florida, United StatesReviewed by:
Timothy C. Haas, University of Wisconsin–Milwaukee, United StatesBill Bateman, Curtin University, Australia
Melissa Seaboch, Salt Lake Community College, United States
Copyright © 2024 López-Ramirez, Lucas, Aguiaro Pereira and Ruiz-Miranda. This is an open-access article distributed under the terms of the Creative Commons Attribution License (CC BY). The use, distribution or reproduction in other forums is permitted, provided the original author(s) and the copyright owner(s) are credited and that the original publication in this journal is cited, in accordance with accepted academic practice. No use, distribution or reproduction is permitted which does not comply with these terms.
*Correspondence: Carlos R. Ruiz-Miranda, Y3J1aXpAdWVuZi5icg==