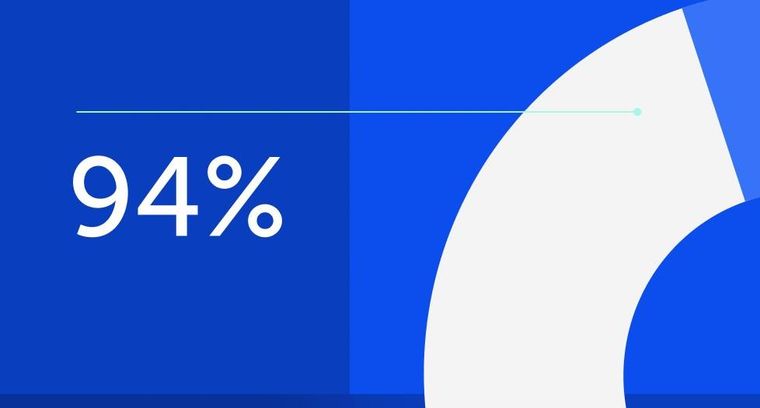
94% of researchers rate our articles as excellent or good
Learn more about the work of our research integrity team to safeguard the quality of each article we publish.
Find out more
ORIGINAL RESEARCH article
Front. Comput. Sci., 20 October 2023
Sec. Networks and Communications
Volume 5 - 2023 | https://doi.org/10.3389/fcomp.2023.1191853
This article is part of the Research TopicInfrastructure Sharing in Broadband Networks: Impact on Telecommunications Operators and ConsumersView all 5 articles
How cost-efficient are potential infrastructure sharing business models for the 5G era (and beyond)? This significant question needs to be addressed if we are to deliver universal affordable broadband in line with Target 9.1 of the UN Sustainable Development Goals. Although almost two-thirds of the global population is now connected, many users still lack access to high-speed and reliable broadband connectivity. Indeed, some of the largest connectivity issues are associated with those living in areas of low economic viability. Consequently, this assessment evaluates the cost implications of different infrastructure sharing business models using a techno-economic assessment framework. The results indicate that a rural 5G neutral host network (NHN) strategy helps to reduce total cost between 10 and 50% compared with other sharing strategies. We also find that, compared to a baseline strategy with No Sharing, the net present value of rural 5G sharing strategies can earn between 30 and 90% more profit. The network upgrades to 5G using various sharing strategies are most sensitive to changes in the average revenue per user, the adoption rate, and the amount of existing site infrastructure. For example, the results from this study show that a 20% variation in demand revenue is estimated to increase the net present value of the sharing strategies by 2–5 times compared to the No Sharing strategy. Similarly, a 10% increase in existing infrastructure lowers the net present value by 8–30%. The infrastructure sharing strategies outlined in this study have the potential to enhance network viability while bridging the digital divide in remote and rural locations.
Recent advancements in wireless broadband connectivity have greatly benefited societies and the wider global economy. Several facets of human life, particularly during the COVID pandemic, have benefited from broadband connectivity (Grijpink et al., 2020; Holmes and Burgess, 2020). Indeed, despite almost two-thirds of the world's population now being connected to the Internet, many users are still under-served and experience poor broadband connectivity (ITU, 2021, 2022). More often, it is the rural and remote areas that experience poor broadband services, if coverage is even offered at all. Rural Internet connectivity remains limited for various reasons including monetary, policy, regulatory, and technological constraints (Frias et al., 2020; Shruthi et al., 2021). Thus, building wireless broadband infrastructure is a pressing economic development issue (Freeman et al., 2016; Oughton et al., 2021; Chen et al., 2023). Importantly, wireless broadband can have a relatively low investment cost compared to other broadband communications technologies (e.g., fixed broadband networks) (Samdanis et al., 2016; Yaacoub and Alouini, 2020). However, this needs to be supported by evidence exploring cost-efficient ways to invest in the limited financial capital available, ensuring that the right technologies and business models are selected to maximize societal benefits (Luong et al., 2019; Banda et al., 2022).
The fifth generation (5G) broadband cellular network is now being widely deployed around the world, predominantly in urban and sub-urban areas where the population density is very high (Blackman and Forge, 2019). The key use cases of 5G include enhanced mobile broadband (eMBB), ultra-reliable and low latency communications (uRLLC), and massive machine type communications (mMTC) (Al-Dulaimi et al., 2018; Saarnisaari et al., 2020). However, in low-demand locations, it can be difficult for 5G to be economically viable using traditional deployment strategies due to the cost involved in meeting the demands such as high capacity and low latency performance requirements (Chiaraviglio et al., 2017; Jiang et al., 2021). One emerging technology enabled by 5G is “network slicing,” which supports network virtualization and consists of independent logical networks, called slices (Ghosh et al., 2019). Slicing technology can support the deployment of shared neutral host networks (NHN) where multiple tenants/operators can co-exist on the same physical network but different virtual networks (Gomes et al., 2021). The survey on the need for sharing the telecommunication infrastructure, especially at the edge is explored in Lehr and Stocker (2023). This research brings out the need for supportive policies for end-user infrastructure sharing, especially to meet 5G performance requirements.
Currently, 5G network sharing strategies can be classified into 4 broad types: No Sharing, Passive Sharing, Active Sharing, and NHN (GSMA, 2019a). In No Sharing, each operator deploys their own independent network, whereas in Passive Sharing, multiple operators share non-electronic components, such as towers and site compounds. Alternatively, in Active Sharing, the operators share all passive and electronic telecommunication components, except for different spectrum bands and the network core. Finally, in a NHN the operators share all passive and active components between themselves and other potential slice tenants.
A recent techno-economic assessment has indicated that a 5G business case that involves infrastructure sharing can lead to an increase in operator revenue, resulting from more efficient usage of infrastructure (Schneir et al., 2019; Walia et al., 2019; Allawi et al., 2022), motivating the study of this topic. Advances in 5G techno-economic approaches have been attempting to better integrate more realistic aspects of the underlying infrastructure in engineering-economic evaluation (Smail and Weijia, 2017). Indeed, techno-economic studies often focus entirely on greenfield deployments, excluding the fact that there might already be existing infrastructure in rural locations providing basic connectivity. For example, many rural areas may have a 2G cellular infrastructure deployed, with those assets still repaying the debt used to finance the existing construction (Smail and Weijia, 2017; Kusuma and Suryanegara, 2019). In such a circumstance, where the rural community has an existing basic telecommunications network, the key questions are:
1. How should the network be upgraded to a future cellular generation (such as 5G or beyond)?
2. What level of sharing might deliver the best outcomes for the operator, users, and wider society?
Consequently, the research in this paper explores future infrastructure sharing strategies for rural areas, predicated on the notion that most locations already have at least some existing infrastructure assets providing basic connectivity (for example, 2G, 3G, or 4G). The key contribution is the estimation of quantitative viability metrics and sensitivity analysis for four different infrastructure sharing strategies to address the digital divide, especially in rural and remote areas. Given each rural area faces a unique set of challenges due to its geographic location, there is a need to investigate solutions for generic rural areas while taking into account as many variables as practical.
The key aim of this study is to analyze the cost of minimizing the digital divide over the next decade, for users within the reach of existing infrastructure. Thus, providing the higher quality of service (QoS) offered by 5G compared to legacy technologies. Indeed, the initial goal of SDG 9.1 is to build sustainable and inclusive infrastructure, especially as availability is essential to increase community adoption and digital literacy. Over the long term, as demand for wireless broadband grows in rural areas, operators may need to later pivot to other infrastructure strategies, for example, by densifying the network with small cells to serve higher traffic quantities.
This paper is organized as follows. Firstly, Section II provides an overview of the literature on different 5G network sharing strategies. The method is then presented in Section III, while Section IV presents the results. Finally, Section V discusses the advantages along with any challenges of the business models appraised, while Section VI provides conclusions.
Generally, there are two main types of possible wireless broadband infrastructure approaches in rural areas:
• Greenfield deployment refers to a scenario where there is no form of existing broadband infrastructure in place, therefore requiring an operator to build network assets from scratch. While capital intensive to build greenfield assets, the network operator does have flexibility in what to deploy as no legacy systems are present. However, decisions need to select the most suitable and cost-efficient technology to deploy to support their requirements (ITU, 2022; Simon, 2022).
• Brownfield deployment refers to a scenario where some form of broadband technology is deployed. Hence, the network operator needs to upgrade the existing radio equipment and any other supporting infrastructure. While recent ITU data might demonstrate that ≥70% of the global population is now online, many of these users may lack decent high-speed Internet (ITU, 2022)
Assessments indicate that the majority of global people already live within reach of existing mobile infrastructure (Shruthi et al., 2021; Oughton, 2023). However, huge coverage and capacity problems exist due to users being under-served. Therefore, upgrading existing infrastructure is becoming a major focal point for overcoming the digital divide over the next decade (Commission, 2022). This will largely involve upgrading legacy 2G/3G systems to support newer cellular generations, such as enhanced 4G/5G mobile broadband. It is possible for a single 4G site to serve up to 20,000 subscribers, with up to 2,000 active devices (Parkvall, 2023). In theory, a 5G site is expected to support up to a million devices (Hossain and Hasan, 2015).
Many studies have investigated the costs of deploying and operating a nationwide 5G network and concluded that changes to rural telecommunication business models will drive enhanced connectivity (Firli et al., 2015; Jha and Saha, 2019; Kusuma and Suryanegara, 2019). Furthermore, a key observation is that MNOs take a long time to deploy near-ubiquitous coverage because the provisioning of telecommunication services is a costly procedure with a low or negative return on investment (ROI) in rural or remote rural areas (Yaghoubi et al., 2018; Cano et al., 2019). Hence, there is a need to explore different network-sharing strategies to minimize the digital divide, with the ambition of bringing the next generation of cellular technology to rural areas (e.g., 5G). Infrastructure sharing at any level eases the process of network deployment and opens up newer revenue streams (Meddour et al., 2011). The key advantage of shared infrastructure is the increased resource utilization and network capacity as a result of infrastructure along with spectrum sharing among the tenants on the network (Kliks et al., 2018; Schneir et al., 2019).
In network slicing, each slice can be tailored to support the use case to be served with distinctive 5G key performance indicators (KPIs), such as for latency, data rates, error rates, minimum resource allocation, etc., (Series, 2017; Zhang, 2019). The key technology enablers for network slicing are software-defined networking (SDN), and network function virtualization (NFV), which provide the lifecycle management of network slices by dynamically instantiating, modifying, and terminating the slices as per the end-user requirements (Afolabi et al., 2018). Network slicing is integrated with multi-access edge computing (MEC) to combine the benefits offered by SDN, NFV, and service function chaining (SFC) (Mach and Becvar, 2017). This integration helps to overcome the static resource allocation issue and convert it to dynamic resource allocation while still satisfying the network performance requirements for the slice users. The key benefits offered by this integration are dynamicity and efficient use of resources (Filali et al., 2020). This technique of capacity partitioning helps reduce the overall capital expenditure (CAPEX) and operational expenditure (OPEX) while leveraging the benefits of dynamic resource sharing and allocation (Foukas et al., 2017; Shen et al., 2020).
The working definition of an NHN is “a self-contained cellular network deployed by a service provider that builds and operates an integrated technology platform that is solely for sharing purposes” (Badmus et al., 2019). This helps to reduce the duplication of resources while providing services in the same area (Matinmikko-Blue and Latva-aho, 2017). A NHN approach allows a single physical infrastructure to be built for multiple operators acting as tenants and could use shared spectrum bands for its operation. A survey of ongoing research on neutral hosts, especially using 5G suggests that an NHN approach can enhance capacity and coverage, especially in dense small cell deployments, with the right policies in place that encourage incumbent operators to participate (Walia et al., 2017; Maeng et al., 2020; Lähteenmäki, 2021). The potential tenants could be mobile network operators (MNOs) (Matinmikko et al., 2017; Oladejo and Falowo, 2017; Colman-Meixner et al., 2019), Internet service providers (ISPs) (Pries et al., 2016; Frank et al., 2022), communication providers (CP) (Cavalcante et al., 2021), hospitals, and other private networks (Giambene et al., 2019; Zhang et al., 2019). In a NHN model, each slice tenant has an end-to-end 5G virtual network with all components of a typical wireless network (Zhang et al., 2017; Kaloxylos, 2018). Indeed, many researchers have examined the challenges associated with 5G network slicing. For example, this includes evaluating different business models, deployment options, and techno-economic feasibility levels, with an approach based on an NHN using shared spectrum, being the most cost-efficient option (Ramasetty and Masilamani, 2019; Quadri et al., 2020; Bajracharya et al., 2022). As network slicing and a NHN approach encourage an open network with dynamic resource allocation, this idea is being expanded to 6G where network slicing is one of its key enablers (Cao et al., 2022).
Infrastructure sharing may also include spectrum sharing among operators, hence necessitating the need to share both underlying infrastructure costs and spectrum licensing costs for a site across the potential tenants (Meddour et al., 2011). As national operators upgrade the network, the infrastructure will use their nationally licensed or locally shared spectrum bands for operations (Matinmikko-Blue et al., 2018, 2019). The ongoing research on NHNs helps to understand the various aspects of 5G network sharing strategies, especially in terms of technology, spectrum, security, policies, regulations, and techno-economic feasibility (Khodashenas et al., 2016; Tseliou et al., 2019; Wang et al., 2019).
Rural 5G networks can be used for a variety of purposes. For example, eMBB applications that require high bandwidth and data rates (video/voice calling, remote video monitoring, remote health care, wide-area industrial automation, video streaming, e-governance, e-commerce, and online learning). Moreover, uRLLC applications that require very low latency and low data volume (disaster management and response, control of critical services, and machine-to-machine communication). Additionally, mMTC applications that require low power and data volume (sensors and IoT devices with limitless data transmission). These applications enable the deployment of 5G in rural areas, potentially generating multiple revenue streams for infrastructure providers (InP) and ensuring network viability.
To viably deliver affordable rural connectivity there are several challenges that need to be overcome. Many issues are technological, but there are also challenges pertaining to adoption, revenue generation, and business models (Noll et al., 2018; Yusuf et al., 2021). For example, to address the digital divide, many technologies have been trialed and tested to assess their suitability, including long range Wi-Fi, 4G, unmanned aerial vehicles (UAVs), satellite systems, balloons, and TV whitespace (TVWS) (Osoro et al., 2023). However, the common shortcomings of these technologies include network performance during changing operating conditions, scalability, user roaming, high-speed data rates, reliability, data security, latency, and other performance criteria for different telecommunication operators (Fourati et al., 2022; Kumar et al., 2022; Randell-Moon and Hynes, 2022).
Moreover, to address the issue of rural connectivity, researchers have begun testing the suitability of 5G network designs to address the needs of rural use cases. Indeed, a rural 5G pilot trial that uses IEEE P2061 5G standards develops an architecture designed to support low-cost rural broadband communication using a 5G NHN without network slicing and Wi-Fi (Khaturia et al., 2020). The proposed solution utilized macro-cells to support 5G access technologies, with backhaul connectivity relying on a TVWS link and the last-mile using wireless local area networks (WLAN) (Khaturia et al., 2020). In another report by GSMA, the authors encourage sharing to reduce the digital divide using a local service provider in rural areas (Handforth, 2019). The authors find the key to minimizing the coverage gap and connecting these locations at a reasonable cost is to lower network deployment and operational costs and find newer business models. It is also important to account for risks associated with the adoption of 5G, as this has a large impact on commercial viability. The use of spectrum sharing in local 5G networks deployed by the InP is explored in Perez Guirao et al. (2017), Anderson et al. (2020). These studies highlight the benefits and ease of spectrum sharing in 5G bands, especially at higher frequencies. In another pilot trial, the 3.5 GHz band using the GSM and TV broadcast tower sites in rural areas was used for long-range coverage to provide broadband services (Lun et al., 2019). The network was able to achieve good downlink data rates even near the cell edge, however, the uplink performance was inadequate.
Many studies were carried out to investigate the costs of deploying and operating a nationwide 5G network with a duration of 10 years in different countries (Oughton and Frias, 2018; Oughton et al., 2019a,b). The conclusion was that encouraging the correct policies, technology choices, and innovations in rural telecommunication business models will drive infrastructure deployment. Ultimately, MNOs require a long time to install near-universal coverage using conventional deployment strategies since, in remote or rural locations, providing telecommunications services is a costly option with a low or negative return on investment. As a result, the research in this paper examines 5G infrastructure sharing strategies as an alternative solution for providing broadband connectivity in rural areas.
Figure 1 shows the possible upgrade sharing strategies for existing cellular sites to 5G involving many options, ranging from No Sharing to either Passive Sharing, Active Sharing, or a NHN.
Figure 1. Network architecture of various sharing strategies of 5G upgrade: (A) no Sharing; (B) passive sharing; (C) active Sharing; (D) 5G NHN.
Figure 1A shows the network architecture for a No Sharing strategy. In this approach, the incumbent MNO has full control over the network and its equipment from end-to-end. There is no competition over the QoS provided, as typically, this type of strategy has only one operator in a rural area. It will be expensive for another operator to deploy their infrastructure, especially in places with negative or poor ROI (Jeanjean, 2022).
Figure 1B shows the network architecture for a Passive Sharing strategy which involves sharing of backhaul, telecommunication sites, ducts, masts, towers, equipment rooms, and related power supplies, air conditioning, and security systems. The operators using this strategy would have to work toward the goal of reducing the overall cost and agree upon a common cell plan management and upgrade. The key challenge with this strategy is finding operators with similar goals in terms of deployment locations, desired site construction materials, tower heights, network protections, and backhaul capacity requirements (Oughton and Frias, 2018; Jeanjean, 2022).
Figure 1C shows the network architecture for an Active Sharing strategy. This business model involves sharing radios, base stations, backhaul, telecommunication sites, ducts, masts, towers, equipment rooms and related power supplies, air conditioning, and security systems. The spectrum bands are not shared, therefore, each operator uses their licensed bands. This method is preferred by operators who have long-term contractual agreements with each other, along with clearly defined agreements regarding operational conditions. The crucial factors affecting deployment include trust among competitors and the policies laid out by the national telecommunication regulator. The challenges with this strategy include making this a long-term commitment, network complexity, and the fact that each individual operator must relinquish their own independent decision-making e.g., for network upgrades. Similar pricing plans could act as a threat to disrupt operator cooperation (Frisanco et al., 2008).
Figure 1D shows the network architecture for a NHN strategy which involves the sharing of spectrum, core networks, radios, base stations, backhaul, telecommunication sites, ducts, masts, towers, equipment rooms, power supplies, air conditioning, and security systems. This method involves end-to-end network sharing (at all passive and active levels, including spectrum) among the slice tenants (Samdanis et al., 2016; Gomes et al., 2021; Jeanjean, 2022). Unlike the previously articulated sharing strategies, the potential operators would have a network agreement only with the 5G NHN infrastructure operator. This strategy also allows other potential slice tenants, and the operators, to co-exist on the network (Fernandez-Fernandez et al., 2021; Lappalainen and Rosenberg, 2022). In the final NHN strategy, all MNOs would lease slices from the incumbent and ideally provide services at all sites. The key challenges of this strategy are similar pricing plans and market strategies, a decline in infrastructure-based competition, management of dynamic resource allocation, and the security of data on each slice (Paglierani et al., 2020; Pápai et al., 2022). An important aspect of a successful upgrade to a 5G network using a NHN model is cooperation among the slice tenants and their corresponding resource allocation schemes (Sanguanpuak et al., 2019; Tran and Le, 2020). These barriers and obstacles to adopting the NHN strategy should be explored as future work to stimulate discussions among stakeholders. The widespread usage of this technology lies in the slicing capabilities offered by the network, and associated security aspects (Raza et al., 2019; Psyrris et al., 2021; Sciancalepore et al., 2022).
A preferable first option for operators may be No Sharing, as it would enable absolute control over network capacity resources (GSMA, 2019b; Jeanjean, 2022). However, this is not always economically viable because of the required investment, existing debt, and potential revenue. Indeed, operators in many markets worldwide have been experiencing static or declining profits while also being saddled with sizeable existing debt payments (van Kranenburg and Hagedoorn, 2008; Veligura et al., 2020; Sahoo and Sahoo, 2022). Thus, there has been a need for MNOs to seek newer 5G revenue streams, as explored in many research papers (Oughton and Russell, 2020; Bajracharya et al., 2022). Therefore, operators may choose to explore other sharing strategies (Frisanco et al., 2008; Oughton et al., 2022b).
With a weak economic outlook for MNOs but also the need to invest in new infrastructure, the willingness for operators to share assets and spectrum bands is increasing (Matinmikko et al., 2017; Oproiu et al., 2018; Colman-Meixner et al., 2019). The MNO business models utilizing 5G network sharing strategies have been found to be cost-efficient in different deployment scenarios (Atherley, 2020; Psyrris et al., 2021; Kenechi and Stefano, 2022). In the NHN case, the approach supports MNOs, private networks, ISPs, and other potential tenants to co-exist without interfering with each other's operations. Studies have shown that horizontal slices support use cases while vertical slices support multi-tenancy (Kaloxylos, 2018; Lee et al., 2019; Shruthi et al., 2021).
In recent years, techno-economic assessment (TEA) frameworks have been trying to include additional simulation parameters which better match real-world deployment conditions (Bouras et al., 2020; Oughton and Russell, 2020; Frank et al., 2022; Schneir et al., 2023). Table 1 summarizes the different TEA models and their adopted methods, parameters, and the key findings of those studies. Consumer and government pressure to provide enhanced telecommunication infrastructure, with higher data throughput per user and better overall QoS, has been encouraging operators to upgrade their networks and expand coverage (Oughton et al., 2021; Pryce, 2022). Many of the studies presented in Table 1 focus on urban deployment scenarios or specific vertical use cases. Hence, there is a need to define a generic theoretical framework of assessment to enable the techno-economic feasibility evaluation of infrastructure sharing strategies and business model options.
Typically, each rural location has a unique set of network feasibility conditions. These depend upon a range of factors, including population density, per capita income, the adoption rate, local business composition, fiber backhaul availability, and existing competition among operators (Walia et al., 2017; Kumar et al., 2022; UN, 2022). As a result, in this study, we explore the suitability and the techno-economic viability of different rural network sharing strategies. We also examine how the input parameters of the developed model affect the feasibility of 5G infrastructure sharing.
This section will detail a method for answering the research question. We focus on solutions with sustainable data rates higher than 30 Mbps per user (Schneir and Xiong, 2016; Ioannou et al., 2020; ITU, 2021), for low-frequency bands (<1GHz) and mid-frequency bands (1–6 GHz). For this study, the existing backhaul could either be wireless or wired technology that may also require upgrading.
Techno-economic assessment can help determine the technical and economic requirements for the profitability of successful infrastructure deployment strategies (Oughton and Lehr, 2022). Thus, Figure 2 illustrates the techno-economic modeling framework used in this study for understanding the business case feasibility of 5G rural upgrades via different infrastructure sharing business models. The model takes inputs capturing future traffic demand, existing infrastructure assets, and network parameters and estimates the number of necessary upgrades (Jang, 2022). This model is derived from many studies across the literature (Yaghoubi et al., 2018; Schneir et al., 2019; Oughton and Lehr, 2022), and further extends the approach to include different infrastructure sharing strategies, in order to investigate their feasibility.
Figure 2. Techno-economic modeling for the assessment of 5G upgrade using different sharing strategies.
The incumbent operator is treated as having four key infrastructure sharing strategies to select from, depending upon the overall cost requirements in terms of CAPEX, OPEX, and existing debt. Rational network operators will aim to minimize the cost of potential infrastructure upgrades while attempting to maximize the revenue opportunity in any sharing strategy (Watson, 2002; Duan et al., 2013).
The capacity assessment helps to estimate the current level of available data traffic that existing assets are capable of transporting (Oughton et al., 2022b). Initially, incumbent operators need to assess the sites that require upgrading and their parameter requirements, such as spectrum, bandwidth, latency, 5G KPIs, network congestion during busy hours, and throughput (Ioannou et al., 2020; Oughton and Lehr, 2022). The number of site upgrades necessary can be estimated based on the number of potential future subscribers of the network, the number of concurrent users, and other possible slice tenants' applications (Duan et al., 2013; Oughton et al., 2022b). The number of sites that would require upgrading varies depending on the sharing strategies, demand assessment, and combined area of coverage.
In reality, the incumbent network operator would conduct a survey in the region of interest and list the location of each telecommunication site, its existing backhaul capacity, operating frequency bands (licensed and unlicensed bands), latency, bandwidth, throughput, data rates, busy hour traffic capacity, the population that it serves, the coverage area, the technologies supported, user plane and data plane management, servers, and other network performance indicators. With this information, it is possible the incumbent operator can analyze existing assets in detail such as cells that show high traffic congestion and hotspots where the demand is very high (Zulfadli, 2022). It is important to maximize the use of existing infrastructure during rural network upgrades to keep costs down (GSMA, 2019b; Frank et al., 2022; Kenechi and Stefano, 2022).
Let A km2 be the area of study region for the network upgrade. Assume, that there are N incumbent operators, each having xmc, i macro cells and xsc, i small cells, such that i ϵ N in the region of interest.
The overall site density in the region of interest, ρsite, is given as
The average coverage area per site, β km2 is estimated as follows:
The theoretical data throughput for a 5G site is calculated using the equation given below (Lim, 2020):
where, PRB is the physical resource blocks (PRBs), J is the sum of 5G carriers in carrier aggregation, ν(j) is the number of layers that a gNodeB transmitter streams to a piece of user equipment (UE), is the modulation order (shown in Table 2), fj is the scaling factor, and Rmax is a number equal to . Finally, is the resource block allocation that is determined by the sub-carriers depending upon μ numerology and BW in bandwidth, Ts is the symbol time, and Oh is overhead.
Next, to understand the practical throughput implications of multiple 5G sites in close proximity, we need to utilize a 5G new radio (NR) link budget. Via stochastic geometry, spectral efficiency values can be estimated producing a distribution of capacity among different UEs at varying distances from a gNodeB (Lim, 2020; Jang, 2022). The NR link budget estimation considers a standard deviation of 6 dB for a rural scenario and different propagation models for rural areas (Lim, 2020; Oughton and Jha, 2021), in line with the literature (Oughton, 2020a,b). The analysis also considers interference from nearby base stations. The NR link budget per UE (in dBm), shown in Equation (4), is described in the 3GPP 38.901 standard (Lim, 2020). The link budget depends on a range of factors including climatic conditions, foliage, building clutter, distance between the transmitter and the receiver, indoor or outdoor location of the receiver, atmospheric conditions, and frequency of operations.
The 5G pathloss equations (PLpropagationModel) for the rural macro cell scenario as defined in 3GPP 38.901 standards for a line of sight (LOS), PLRMALOS, is as given below (Ghosh et al., 2019; Jang, 2022; Lin, 2022):
where c is the speed of light, d2D is the ground distance between BS and UE, hBS and hUT are the height of the base station and UE, respectively, and fc is the center frequency in Hz. For PL1 has a shadow fading of, σSF = 4, hBS = 35m, hUT = 1.5m, while for PL1 has a shadow fading of, σSF = 6. These formulas are valid for 10m ≤ hBS ≤ 150m and 1m ≤ hUT ≤ 10m.
The signal-to-noise ratio (SINR), γ = , values are used in Equation (7) to calculate the capacity and spectral efficiency per user. The actual channel capacity per site, C bits/sec of the existing infrastructure, is estimated using bandwidth B, channel utilization χ, SINR γ, and spectral efficiency, μ (Capozzi et al., 2013). Generally, realistic channel capacity C, is lower than theoretical channel capacity C5G (Abozariba et al., 2019; Lin, 2022).
Network modeling focuses on capturing congested peak demand periods, with the network consequently able to handle traffic during less congested periods. Moreover, traffic estimation helps to understand network traffic demanded by 5G users and the ability to meet user requirements. Additionally, the network should be flexible enough to accommodate future growth in 5G demand. To understand the demand per site, 5G NR traffic modeling as well as scheduling is utilized (Benoist, 2018; Zainal, 2022).
As the number of active users on the network grows, there is a need to increase the number of gNodeB assets deployed to meet the minimum user data rate requirements (Comşa et al., 2018; Nor et al., 2022). The number of sites that are required to be upgraded is estimated using link budget analysis, traffic management, and user scheduling (Oughton et al., 2023). The incumbent operator would select the outcome which provides the maximum number of towers for the upgrade, to account for future demand from end-users and their applications (Lee et al., 2014; Amer and Puttaswamy, 2019).
Data traffic demand is estimated by determining market share, anticipated smartphone users or other business subscribers, population distribution, active users exchanging traffic at peak times, the amount of traffic per user, and then the amount of traffic handled per site (Sciancalepore et al., 2017). Here, we follow the demand method applied in the digital infrastructure costing estimator (DICE) (Oughton et al., 2023). This model was developed for the analysis of universal broadband studies with the aim of quantitatively modeling the various factors described above to estimate traffic demand, which is broadly commensurate with other modeling approaches commonly found in the literature (de la Torre et al., 2020; Oughton et al., 2022b; Oughton, 2023).
Rural areas tend to have a small number of settlements, although there are a few outliers (Yaacoub and Alouini, 2020). The demand estimation also includes business subscriber data and throughput requirements for potential end-user applications, including Internet of Things (IoT) devices or other technologies for health, energy, transportation, etc. (Musacchio et al., 2006). Another major unknown parameter that affects network feasibility is the expected average revenue per user (ARPU). In theory, if an operator expects the existing ARPU to increase following the deployment of new services, then there would be a higher appetite to invest, for example, in upgrading to 5G services (Kenechi and Stefano, 2022). This situation, however, has considerable uncertainty, which requires scenario analysis (Oughton et al., 2022b). Finally, compared to consumers, business subscribers are typically expected to pay higher subscription rates (which may translate into a more reliable service, and revenue stream) (Lappalainen and Rosenberg, 2022).
In this step, the incumbent operator would estimate the potential 5G subscribers and their use cases. There would be a survey/discussion with the potential slice tenants about their application requirements that the network would need to satisfy. The incumbent operator would tabulate the demand assessment model's outputs and estimate the ARPU that end-users would be willing to pay for their services. The end-users could be business-to-business (B2B) or business-to-consumer (B2C) (Psyrris et al., 2021; Schneir et al., 2022). The number of small and macro cells that require an upgrade is dependent on this analysis.
To estimate the traffic demand that should be supported by the network over a period of T years (say, T is the study period), there is a need to include the data obtained from the demand assessment model. Let the expected average user traffic be given as δt GB/user/month, such that, t ϵ T. Then, the data consumed per day per user, δt, day MB/day (Oughton and Frias, 2018; Oughton and Jha, 2021). The minimum data speed required per user ζ in Mbps, during the busiest hour of the day (BHF) using the conversion value of 1 Byte (B) with 8 bits (b), and 1 h with 3,600 s (Oughton et al., 2022b), is calculated as:
Then the population density, ρpop for the study area A with population P. Typically, x% of the population density, ρpop for the study area A of the P, would be the number of subscribers for a service.
Finally, the area traffic ιarea is estimated as (Oughton and Russell, 2020; Oughton, 2023),
This assessment includes the estimation of the cost incurred in deploying and operating the different business model options. The expenditure for a particular 5G upgrade is calculated first per site and then aggregated to a local statistical area level. A rational incumbent MNO designs and deploys a forward-looking network that accounts for future traffic demand over the next 10–20 years. The discounted total cost of ownership (TCO) ω is estimated as the sum of CAPEX ωc and OPEX ωo over this time horizon (Chiaraviglio et al., 2017; Ioannou et al., 2020; Oughton and Lehr, 2022). CAPEX includes the cost of the radio equipment upgrade ωRAN, the backhaul upgrade (wired as well as wireless) and any labor ωb, a small edge cloud site ωedge, spectrum ωs, and any core network upgrades necessary ωcore. OPEX includes the cost of power, administrative operations, core network maintenance, routine maintenance of radio equipment, operational spectrum, and edge cloud maintenance.
Generally, there is a need to upgrade the backhaul capacity to support 5G data rates. Additionally, unlike other studies which exclude current asset debts, ωd, this assessment also includes a nominal existing debt payment per site which is closer to what is experienced in reality (Cheng et al., 2003). The debt payment factor ωd, is not included in OPEX because it lacks the traits required for ongoing routine operations and maintenance. As a result, they are included in CAPEX, which is in accordance with the discussion with operators related to the inclusion of debt in their estimates. By adopting this parameter, analysts can more accurately reflect the level of debt owed to each operator and its impact on brownfield telecommunications deployment. Furthermore, as per standard industry practice, the backhaul cost is split between CAPEX and ongoing OPEX (Oughton et al., 2022a), while the existing debt payment is factored into CAPEX (Brach, 2016). Therefore, the modified TCO for this study is (Yaghoubi et al., 2018; Chiha et al., 2020; Oughton and Lehr, 2022).
The 5G network upgrade assessment estimates the infrastructure requirements for future assets. This model includes details about site locations, additional backhaul capacity, macro and small cell quantities, future spectrum bandwidth, expected spectral efficiency, usage of the network traffic, and slice requirements of various potential tenants. The tenants may find it desirable to obtain the resources they lease on a near-real-time basis (Sanguanpuak et al., 2019; Jeanjean, 2022). In addition, the incumbent may also need to account for upgrades to support potential future tenants.
Table 3 shows the number of physical components for upgrade per cellular site for each sharing strategy in a 4-operator scenario. The total number of sites required for the upgrade is subject to the existing coverage areas and network sharing strategy. From Table 3, it can be observed that in a No Sharing (baseline) deployment, most sites and base stations of the incumbent would need to be upgraded and no physical sites are shared. In a Passive Sharing deployment, the resources required for upgrading are reduced compared to the baseline scenario as the physical site locations and other passive components are shared among all operators, whereas the radios, spectrum, hardware, and core are not shared. Furthermore, in an Active Sharing approach, the operators deploy a lower number of radios and hardware components compared to Passive Sharing. Finally, in the NHN deployment, the total resources for a 5G network upgrade reduce further as end-to-end components are shared by all operators.
Let κ be the number of towers that require a network upgrade and ω be the TCO of the network upgrade. The key optimization equation to lower the TCO associated with 5G brownfield deployments in rural areas, while satisfying the aims to increase coverage (β) and data rates, (C) but minimizing the number of towers that require an upgrade, is stated as follows (Duan et al., 2013; Shruthi et al., 2021):
This framework performs a feasibility analysis of the possible infrastructure sharing strategies for each rural 5G business model. The results of the network upgrade requirements, specifically, the number of necessary upgrades needing to be made, are then fed forward to be combined with the potential costs of each component from the cost model, thus producing the key assessment result metrics.
The revenue per year Ri such that i ϵ T, and the total revenue, R, over the period T for ARPU Ψ5G for 5G services are calculated as (Ioannou et al., 2020; Oughton et al., 2021):
where Ψold shows the ARPU for existing infrastructure, ρnew5G are the additional new subscribers joining the network who require 5G KPIs for their applications, and ρup, sub is the existing subscribers who upgrade their services to 5G technology who can be charged more than existing technologies. The cash flow for year i, αi such that i ϵ T, is estimated as (Besanko and Braeutigam, 2020):
where ωi is the cost per year toward the upgrade. The incumbent operator would upgrade the network sequentially to match the network demand and earn higher revenues. For analyzing the profitability of the network upgrade to 5G using different sharing strategies, the net present value (NPV), Υ, method is used with a discount factor r and is calculated as (Ye and Tiong, 2000; Besanko and Braeutigam, 2020):
when Υ = 0, then it is a no profit-no loss scenario for the infrastructure provider. This helps in the estimation of the minimum ARPU, Ψmin, at which the infrastructure provider would consider deploying the 5G network. The minimum ARPU would in turn help in determining the pricing range which the operators should charge to the end-users (Shruthi et al., 2021).
As incumbent operators shift their 5G business models to rural areas, they are driven both by a desire to reduce overall TCO by maximizing current and future resources and to sell new vertical services to increase revenue (Partners, 2019; Gómez et al., 2022; Pryce, 2022). Hence, the appraisal outputs focus on population coverage in rural areas: the minimum provided speed per user in busy hours, the percentage of subscribers with 30+ Mbps peak speed, an NPV feasibility analysis, and a sensitivity analysis for uncertainties. The various costs for network upgrades for an area with an existing 2G/3G network tend to be higher than upgrades from existing 4G networks. For example, existing 4G hardware can support future infrastructure upgrades with relatively minimal software updates, lowering the upgrade cost significantly (Oughton et al., 2021; Kenechi and Stefano, 2022; Lappalainen and Rosenberg, 2022).
In this section, the results are reported using the methodology illustrated in Figure 2. The network planning simulation was modeled using the Python model, which is available on the GitHub repository (Shruthi, 2023). The overall TCO is calculated for a 5G network upgrade over a time horizon of 10 years for a generic rural context.
Consider a generic rural study area (A) of 500 km2 with interspersed low population density villages (<300 people per km2) for the time period of 2023–2032. In this study, the population density for the base scenario is 36 people per km2. Assume there are four national MNOs, and each wants to be omnipresent within their operating country (Saha, 2020). Table 4 provides a summary of the simulation parameters and the inputs for different models. The data for the study are obtained from various sources from the literature (Oughton and Frias, 2018; Grijpink et al., 2020; Ofcom, 2020; Oughton and Jha, 2021; 5G-New-Thinking, 2022; ITU, 2022; Lappalainen and Rosenberg, 2022). Typically for rural areas, the operating frequencies are in the range of 700 MHz and 3,800 MHz (Partners, 2019). Note that a higher coverage is offered by 700 MHz (at lower throughput rates), while higher throughput rates are offered at 3,800 MHz band (but with lower coverage) (Wahyudin et al., 2021; GSA, 2022). For example, during the network upgrade the 3,800 MHz band could be used to serve a small rural hamlet with enhanced mobile broadband services, while the 700 MHz band could be used for greater wide-area coverage across the cell (e.g., for highly mobile users in vehicles). The network could use either time or frequency division duplex (TDD/FDD) as a modulation scheme.
In this evaluation, for the existing technology with a take-up rate (x), 50% of P, consider that 30% of those users would upgrade to 5G services, and an additional 20% of P would join the network for 5G services. Therefore, the rural region of interest in this study, especially sensitivity analysis, is treated as having between 1,000 to 25,000 mobile subscribers along with thousands of devices for private networks and IoT applications (Oughton and Mathur, 2021). These subscribers could belong to local businesses or corporate companies. Today, the ARPU from wireless broadband around the world ranges from $2 to $45 (ITU, 2021). Therefore, the incumbent MNO would expect a higher ARPU from the 5G services and higher data rates along with exponentially improved performance, say +20% compared to the ARPU of the 4G services (Suryanegara, 2018; Yang, 2022). But over time, the price per GB would drop, which would encourage operators to share the network, which would further encourage infrastructure sharing among the operators (Hunukumbure et al., 2022). Next, the annual increase in the subscriber base is considered to be around 4% although the rural population growth is below zero (Bank, 2021), this is because improved wireless broadband will increase the demand for connectivity for use cases such as remote job opportunities, personal communication, e-governance, farming, and healthcare facilities.
In this paper, we follow a long run incremental cost (LRIC) approach where a “hypothetical MNO” is modeled representing an entity with an average market share, average spectrum portfolio, average set of existing sites, etc. In a perfectly competitive market, each of the four MNOs would acquire roughly 25% of the entire 5G services market share, adding up to 100% of the market share. For this study, we consider the busy hour factor (BHF) to be 0.15, and maintain low latency (<50 ms). An aspirational target for governments globally is to provide a minimum 30 Mbps average data speed per user and to increase the data usage to a minimum of 30 GB/Month per user (UN, 2022). Therefore, in this study, we consider a minimum average data speed of 30 Mbps per user and data consumption of 50 GB/month per user. Table 4 shows the modeling conditions for the study location and its simulation parameters, along with the cost for various components required for the 5G upgrade.
The average cost per site for the macro-cell and small-cell varies considerably. In this study, we estimate that a brownfield macro-cell upgrade is approximately $45,000 including RAN upgrades, core, spectrum ($0.7 per MHz at 700 MHz, and $0.03 per MHz at 3,800 MHz), and other parameters, while the greenfield small-cell deployment is around $12,000 (Osio, 2021; Oughton and Jha, 2021). The variation in the costs depends on the selected strategy and the upgrade required for the existing hardware and software components such as the core, radios, site, gNodeB, and processing units. Next, the cost of an average backhaul upgrade would be approximately $10,000 for macro-cell and greenfield deployment would cost around $5,000 for small-cell. The OPEX for the small cell could be as low as $800 per year, while the same for the macro cell would be around $2,500. The brownfield deployment already has an existing debt payment to be cleared, which is about 5% of the TCO of each site (Bhatia, 2022). Note that the debt payment is for the existing infrastructure (2G, 3G, and 4G), not the new 5G network. The study considers that the CAPEX and backhaul depreciate at a 3% rate, while the OPEX and debt payment appreciate each year at 5 and 2%, respectively (Schneir et al., 2019).
Generally, there would be around 60 active users at any instance in time per site, except during congestion periods when the capacity can be expanded using virtual and cloud resources via network slicing (Chiha et al., 2020). As the number of active users grows, a corresponding rise in the number of demand requests for scheduling users takes place. Furthermore, as demand rises, SINR values decrease because resources are shared among many users (Benoist, 2018; Zulfadli, 2022).
Figure 3 reports the stochastic geometry analysis of the SINR, spectral efficiency, and channel capacity estimate of the realistic channel throughput at the cell edge for a 95% confidence interval, for UEs operating on a 5G cell site. Indeed, there is a clear relationship between increasing UE distance from the cell site, and a decreasing SINR leading to lower spectral efficiency and thus poorer channel capacity. For the 5G network upgrade, both small- and macro-cell strategies are considered. We treat:
• macro cells as providing coverage up to 7 km,
• small cells at a mid-band frequency covering up to 3.5 km, and
• small cells with high millimeter-wave frequencies covering up to 100 m.
Figure 3. Stochastic modeling results per user in 5G cells at different frequencies: (A) SINR, (B) spectral efficiency, and (C) capacity.
The minimum theoretical peak cell throughput is around 177 Mbps and 1,700 Mbps at 700 MHz and 3,800 MHz frequency bands respectively, with power levels below 4 W (Biradar and Hallur, 2022; Vinogradov, 2022; Shruthi, 2023). Small cells offer higher peak data rates than macro cells but require higher quantities to provide services in the same geographic area (Wang et al., 2014). Moreover, the densification of the network by the deployment of small cells at millimeter-wave frequencies depends on the number of subscribers and the potential ROI (Wahyudin et al., 2021).
The stochastic and traffic modeling results for the base scenario of 36 people per km2 suggest that each MNO would aim to upgrade an average of 4 macro-cells, and additionally deploy 8 small-cells, to provide maximum coverage at 30 Mbps in the brownfield rural area 5G deployment. Furthermore, the overall increase in data rates is evident only when the backhaul capacity is above 5 Gbps, specifically to support users and applications that demand more resources.
In this section, we explore the different infrastructure sharing strategies and their techno-economic implications. The TCO is estimated for all the 5G network upgrade sharing strategies and shows that OPEX becomes higher over time due to increased network complexity, breakdowns, repairs, and inflation. MNOs are likely to prefer different sharing strategies depending on existing demand and resource utilization. In order to meet 5G security and dynamic slicing requirements, an NHN has greater equipment requirements that must be met because multiple network operators are using the network infrastructure simultaneously (Raza et al., 2019; Sciancalepore et al., 2022). Hence, the single site cost for upgrading to the NHN strategy is the most expensive. In reality, an upgraded 5G network in rural areas helps to provide eMBB-related applications while supporting other 5G rural applications relating to vertical sectors, such as health and transportation.
Figure 4 shows the overall upgrading cost for all the sites for each year in the period of 2023-2032. The estimated costs shows that the TCO for No Sharing (baseline) is approximately $1,996,791, for Passive Sharing $1,459,224, for Active Sharing $994,446 and for NHN $659,864. Figure 4 and Table 3 show that for an incumbent MNO, the cost of upgrading to a rural NHN per site is higher compared to the incumbent MNO's upgrade to 5G per site, by 6–20% against other 5G network sharing strategies.
Figure 4. CAPEX-OPEX estimation per year for various strategies in the region of interest: (A) no sharing, (B) passive sharing, (C) active sharing, and (D) 5G NHN.
Moreover, Figure 4 presents the financial cost savings possible from 5G infrastructure sharing strategies. Passive Sharing strategies exhibit substantial savings between 10–20% for 50 GB/Month against the baseline. Meanwhile, the Active Sharing strategy results in savings between 20–35% for 50 GB/Month against the baseline. Lastly, a rural NHN provides impressive cost savings of around 35–50% against the baseline scenario.
Additionally, Figure 4 shows that for each network sharing strategy, the cost per year increases due to various factors, including inflation, the loan interest rate, and operating costs. Indeed, the cost increases by 7.6, 6, 5.6, and 5.5% for No Sharing, Passive Sharing, Active Sharing, and NHN, respectively. Also, Figure 4 shows that in the four sharing strategies, the CAPEX to OPEX ratio is around 1.9 in the first year and falls to almost 0.95 in the final year of assessment.
Figure 5 shows the minimum investment required per user toward the 5G network for a sustainable business case. It can be observed that as the number of users increases, the investment required per user decreases. For subscribers below 500, only Active Sharing and NHN strategies are profitable for the operators with per user investment less than $60, which is very high for a monthly ARPU (Taylor, 2023). As the number of subscribers increases, the minimum investment required per user falls below $10, increasing viability. When the number of subscribers is above 10,000 in the region of interest, then the minimum investment required per user is below $1.5 per subscriber. In the present scenario, the deployment is self-sustaining since the network is profitable for the operator while being affordable for end users.
Figure 5. Investment per user analysis at a subscriber growth rate of 4% per year: (A) no sharing; (B) passive sharing, (C) active sharing, and (D) 5G NHN.
Figure 6 shows the sensitivity of the NPV by varying the revenue from −93% to +100% of the baseline value at a population density of 36 people per km2 (=18,000 people in the study area), with a subscriber growth rate of 4% per year. The results show that the increase in subscription demand leads to a commensurate rise in revenue, which overall provides an improvement in the viability of the 5G deployment across the sharing strategies. Also, the estimates in Figure 6 illustrate that the NHN business case is superior by at least 15% compared to other sharing strategies under the same revenue and demand conditions. For a network to be profitable at a low ARPU, say $10 per month, the subscriber base needs to be very high per cellular study area, e.g., above 20,000 subscribers per area. As the ARPU increases, say at $60, the required number of subscribers could reduce to as low as 3,400 subscribers in the NHN strategy for rural areas. The base scenario is calculated with a monthly APRU of $30 per subscriber. Figure 6 shows that all sharing strategies are feasible when the ARPU is higher than $40, that is, viable business models.
Figure 6. 5G SC + MC upgrade using various strategies in the region of interest with varying revenue sensitivities compared to a base scenario ARPU $30 and population density of 36 people per km2: (A) no sharing, (B) passive sharing, (C) active sharing, and (D) 5G NHN.
These results demonstrate that the techno-economic feasibility in rural areas is extremely sensitive to the number of subscribers and ARPU for the network, along with the number of towers requiring upgrading. The estimates also demonstrate the difference in the ROI for each 5G sharing strategy. Figure 6 shows that at $30, the ROI is negative for No Sharing and Passive Sharing, whereas the ROI is positive for Active Sharing and NHN.
Figure 7 shows the sensitivity analysis for the different 5G network sharing strategies. It can be observed that for a 20% increase in the ARPU, the NPV increases twice in Passive Sharing, 4 times in Active Sharing, and 5 times in NHN compared to the NPV of the baseline scenario (No Sharing). Similarly, when the existing infrastructure increases by 10%, the NPV doubles in Passive Sharing, trebles in Active Sharing and trebles in NHN approach compared to the NPV of the baseline scenario. The network is least sensitive to the debt repayment amount. The NPV hardly changes from the base NPV even when the debt payment parameter changes by 60% for all sharing strategies.
Figure 7. Sensitivity analysis of sharing strategies: (A) no sharing, (B) passive sharing, (C) active sharing, and (D) 5G NHN.
The results obtained in this study are compared against the existing rural connectivity studies in Oughton and Jha (2021), Laitsou et al. (2022), Oughton (2023). According to a recent study, Active Sharing and Passive Sharing strategies for 10–30 GB/month in an African scenario would reduce the cost by 48–78% and 10–44% respectively, while the same strategies in this study for 50 GB/month would reduce the cost by 20–35% and 10–20% for Active Sharing and Passive Sharing, respectively (Oughton, 2023). Meanwhile, when considering developing countries with a case study of the Indian context, the cost per user of 4 × 4 active sharing with 5–50 Mbps QoS is 70% lower compared to that of traditional LTE deployments (Oughton and Jha, 2021). These figures are slightly higher than the reduction in the investment per user of 65% estimated here. Similarly, according to a recent study for European countries, it was shown that greenfield 5G standalone fixed wireless access without infrastructure sharing is expensive in rural areas having a cost per user over $100 per month, whereas wireless broadband using infrastructure sharing could potentially be feasible for less than $60 per month (Laitsou et al., 2022).
Studies in the literature on the successful NHN trials and initiatives have shown that a 5G NHN approach is beneficial to MNOs in places where the population density is very high or low, especially if there are multiple use cases targeted (Lähteenmäki, 2021). In this study, the cost savings offered by using a 5G NHN are approximately 35–50% compared to the baseline No Sharing approach. In a city-wide NHN deployment for an ultra-dense urban area, such as in London, the cost-savings are expected to be around 40% (GSMA, 2018; Schneir et al., 2019). Similarly, cost savings for different deployment scenarios, such as a 100-story commercial building in urban indoor and outdoor settings, were estimated to be 70 and 80%, respectively (Allawi et al., 2022). Whereas, for a university campus a NHN approach is estimated to result in a 20% saving (Walia et al., 2017). Finally, for an industry vertical, such as a seaport at Hamburg, a NHN strategy could result in up to a 50% cost saving (Schneir et al., 2022).
The evaluation carried out in this paper explores four major infrastructure sharing strategies for a brownfield deployment capable of supporting future technologies such as 5G (or beyond), including: No Sharing, Passive Sharing, Active Sharing, and 5G NHN. For the two main research questions identified, we find that the investment cost of a 5G network upgrade is significant for all network-sharing strategies tested in this analysis. However, the findings show promising business model options for different deployment strategies, which are common to all operators. An MNO could incorporate them to better reflect their goals, i.e., different levels of sharing might deliver the best outcomes for the operator, users, and broader society. For instance, they may operate their own network in some areas while sharing in others to better reflect their strategic priorities and the economics of network provision. Each incumbent MNO will appraise its asset position, possible future revenues, and the NPV for all sharing strategies to make informed strategic decisions on the most appropriate 5G deployment options. Given there will be different deployment strategies based on the demand conditions in each context, with rural and remote areas being the most challenging locations, the following discussion summarizes each one:
• No Sharing is suitable when the incumbent MNO has a lucrative business model. The MNO would want to retain the monopoly of being an exclusive service provider for all applications and use cases in the region of interest. In the case of a high traffic load that requires each MNO to build a network to meet these demands, maintaining this monopoly position may not have a negative impact on society as a whole.
• Passive Sharing is preferred when the sunk costs are high and potential ROI is lower than the No Sharing scenario. The operators distinguish themselves using metrics, such as coverage and QoS. The operators can still maintain control of the type of active components of the network deployment while sharing specific passive assets (e.g., the site and backhaul) to reduce costs while hopefully improving business case feasibility.
• Active Sharing is an appropriate option when there are fewer sites to compete or fewer available licensed spectrum bands, and operators would like to complement each other's services. One example may include the provision of national user roaming, with operators collaborating to provide reciprocal coverage in each other's service regions. Alternatively, some hard-to-serve low-demand areas may not feasibly support multiple infrastructure networks, making active sharing an attractive option.
• A 5G NHN is the most advanced network sharing configuration and is suitable if multiple operators have a degree of trust in each other. Although it is the most cost-effective strategy, the operators leasing resources from the incumbent MNO need to be able to obtain these resources at a fair price, along with confidence in their longer-term price expectations. This option is the most viable for areas with low subscriber counts and can cater to the full range of 5G applications. This may also be a viable option in very high-traffic areas where a single neutrally hosted network would provide a more optimal engineering design owing to reduced interference and improved cell coordination (Schneir et al., 2019, 2022; Allawi et al., 2022).
The proposed business model strategies for rural areas could prove to be attractive options (Allawi et al., 2022; Oughton, 2023). Whereas this paper focused on the cost-efficiency and viability of the proposed upgrade strategies, the one noteworthy subject not touched on which deserves attention is governance. MNOs generally have substantial experience in negotiating contractual terms and conditions between each other, with some operators having already entered into passive and/or active sharing agreements for infrastructure assets. However, future research needs to explore the pragmatic approaches for MNOs to undertake network sharing in practice, such as toward a company joint venture between MNOs to deploy shared infrastructure or automated policy enforcement by either regulatory bodies or other operators. These policy agreement models may differ considerably by context, in extremely high-density places (such as stadia, campuses, seaports, etc.) or rural areas with very low viability. Similar to this, the TEA model's revenue projections are likely to vary depending on the geographic context.
As a conclusion to this discussion, three key areas of research need to be examined in future studies to provide new insights into infrastructure sharing. Firstly, from an engineering perspective, a comprehensive analysis is required to define the impact of different resource allocation processes, including the control for end-users of each slice, interference management, and spectrum management. Secondly, from a microeconomic perspective, it is not yet clear what the optimal pricing plans should be and how changes in pricing affect the incumbent, tenants, end-users, and wider society. Finally, a new analysis needs to be undertaken from an industrial organization perspective to provide insight into the competitive impacts of infrastructure sharing, especially as operators move toward implementing neutrally hosted networks. Indeed, the dominant theme over the past three decades has been that more infrastructure competition is fundamentally a good thing. However, the mobile industry is now moving toward greater consolidation as a consequence of (i) changing economic circumstances and (ii) the ability to enter into the types of business models appraised in this paper.
This article presented a techno-economic assessment of 5G infrastructure sharing business models in rural areas. This began with the presentation of a theoretical model capable of assessing various infrastructure sharing strategies, and then the application of this model to a case study example. The key contribution is the provision of comparative quantitative information on the cost efficiency of the four different business model options, and their sensitivities (including No Sharing, Passive Sharing, Active Sharing, and NHN). The evaluation considered the total cost of ownership over ten years for a generic rural area.
The results indicate that the NHN strategy reduces the overall cost by 10–50% compared to other 5G sharing strategies. It is evident from the estimated NPV that infrastructure sharing business models can increase viability by 30–90%. However, these sharing approaches can be highly sensitive to changes in demand, as well as the level of existing available infrastructure. Given the current challenges in achieving Target 9.1 of the UN Sustainable Development Goals, the cost-saving measures explored here provide a potential solution for lowering the overall costs of the deployment in more challenging rural and remote areas. However, the implementation of infrastructure sharing strategies cannot happen in isolation and needs to be balanced against prudent technology and policy choices (by operators and governments). Without a comprehensive strategic approach to deploying wireless broadband connectivity, the aim to deliver affordable universal mobile broadband to all by 2030 will be more challenging.
The datasets presented in this study can be found in online repositories. The names of the repository/repositories and accession number(s) can be found below: https://github.com/shruthiKa-kas/infrasharing5Gupgrade.
SK did modeling, analysis, data visualization, and writing. EO undertook data visualization and writing. All authors contributed to the article and approved the submitted version.
The authors would like to thank the reviewers for their valuable feedback which significantly improved the manuscript.
The authors declare that the research was conducted in the absence of any commercial or financial relationships that could be construed as a potential conflict of interest.
All claims expressed in this article are solely those of the authors and do not necessarily represent those of their affiliated organizations, or those of the publisher, the editors and the reviewers. Any product that may be evaluated in this article, or claim that may be made by its manufacturer, is not guaranteed or endorsed by the publisher.
3GPP (2020). TR 138 901-V16.1.0-5G. Available online at: https://www.etsi.org/deliver/etsi_tr/138900_138999/138901/16.01.00_60/tr_138901v160100p.pdf (accessed September 30, 2023).
5G-New-Thinking (2022). Our main aims and outputs. Available online at: https://www.5gnewthinking.co.uk/5g-project-outputs (accessed September 30, 2023).
Abozariba, R., Davies, E., Broadbent, M., and Race, N. (2019). “Evaluating the real-world performance of 5G fixed wireless broadband in rural areas,”? in 2nd 5G World Forum (5GWF) (IEEE), 465–470. doi: 10.1109/5GWF.2019.8911655
Afolabi, I., Taleb, T., Samdanis, K., Ksentini, A., and Flinck, H. (2018). Network slicing and softwarization: A survey on principles, enabling technologies, and solutions. IEEE Commun. Surv. Tutor. 20, 2429–2453. doi: 10.1109/COMST.2018.2815638
Al-Dulaimi, A., Wang, X., and Chih-Lin, I. (2018). 5G Networks: Fundamental Requirements, Enabling Technologies, and Operations Management. New York, NY: John Wiley &Sons.
Allawi, Y. M., Mohammed, A. F., Lee, J., and Choi, S. G. (2022). A sustainable business model for a neutral host supporting 5G and beyond (5GB) ultra-dense networks: Challenges, directions, and architecture. Sensors 22, 5215. doi: 10.3390/s22145215
Amer, M., and Puttaswamy, S. (2019). Traffic model for cellular network analysis. Master's thesis, Department of EIT, Faculty of Engg., LTH, LUND UNIVERSITY.
Anderson, D., Shruthi, K., David, C., and Robert, S. (2020). “Evolving spectrum sharing methods, standards and trials: tvws, cbrs, multefire and more,”? in Spectrum Sharing: The Next Frontier in Wireless Networks 59–74. doi: 10.1002/9781119551539.ch4
Atherley, R. (2020). GSMA Intelligence Shares “Global Mobile Trends 2021”. Available online at: https://www.gsma.com/newsroom/press-release/gsma-intelligence-shares-global-mobile-trends-2021/ (accessed September 30, 2023).
Badmus, I., Matinmikko-Blue, M., Walia, J. S., and Taleb, T. (2019). “Network slice instantiation for 5G micro-operator deployment scenarios,”? in 2019 European Conference on Networks and Communications (EuCNC) (IEEE), 133–138. doi: 10.1109/EuCNC.2019.8802013
Bajracharya, R., Shrestha, R., Jung, H., and Shin, H. (2022). Neutral host technology: The future of mobile network operators. IEEE Access 10, 99221–99234. doi: 10.1109/ACCESS.2022.3207823
Banda, L., Mzyece, M., and Mekuria, F. (2022). 5G business models for mobile network operators - a survey. IEEE Access, 10, 94851–94886. doi: 10.1109/ACCESS.2022.3205011
Bank, W. (2021). Rural population growth (annual %). Available online at: https://data.worldbank.org/indicator/SP.RUR.TOTL.ZG (accessed September 30, 2023).
Bank, W., and OECD (2021). GDP growth (annual %). Available online at: https://data.worldbank.org/indicator/NY.GDP.MKTP.KD.ZG (accessed September 30, 2023).
Benoist, S. (2018). NR; NR and NG-RAN Overall description; Stage-2 38.300. Available online at: https://portal.3gpp.org/desktopmodules/Specifications/SpecificationDetails.aspx?specificationId=3191 (accessed September 30, 2023).
Bhatia, J. (2022). The Indian road to financialisation: a case study of the Indian telecommunication sector. Cambridge J. Econ. 46, 1025–1044. doi: 10.1093/cje/beac039
Biradar, S. B., and Hallur, G. G. (2022). “Economic implication of spectrum bands used in 5G: A multicountry study of spectrum allocation,”? in International Conferences on Decision Aid Sciences and Applications (DASA) (IEEE), 584–590. doi: 10.1109/DASA54658.2022.9765228
Blackman, C., and Forge, S. (2019). “5G deployment: State of play in Europe, USA and Asia,”? in EPRS: European Parliamentary Research Service 13–22.
Bouras, C., Kokkalis, S., Kollia, A., and Papazois, A. (2020). Techno-economic comparison of MIMO and DAS cost models in 5G networks. Wirel. Netw. 26, 1–15. doi: 10.1007/s11276-018-1780-6
Brach, T. J. A. (2016). Managing CAPEX in the telecom industry. PhD thesis, Massachusetts Institute of Technology.
Cano, L., Carello, G., Cesana, M., Passacantando, M., and Sansó, B. (2019). Modeling the techno-economic interactions of infrastructure and service providers in 5G networks with a multi-leader-follower game. IEEE Access 7, 162913–162940. doi: 10.1109/ACCESS.2019.2951697
Cao, H., Du, J., Zhao, H., Luo, D. X., Kumar, N., Yang, L., et al. (2022). Toward tailored resource allocation of slices in 6G networks with softwarization and virtualization. IEEE Internet Things J. 9, 6623–6637. doi: 10.1109/JIOT.2021.3111644
Capozzi, F., Piro, G., Grieco, L. A., Boggia, G., and Camarda, P. (2013). Downlink packet scheduling in LTE cellular networks: Key design issues and a survey. IEEE Commun. Surv. Tutor. 15, 678–700. doi: 10.1109/SURV.2012.060912.00100
Cavalcante, A. M., Marquezini, M. V., Mendes, L., and Moreno, C. S. (2021). 5G for remote areas: Challenges, opportunities and business modeling for Brazil. IEEE Access 9, 10829–10843. doi: 10.1109/ACCESS.2021.3050742
Chen, Y. P., Oughton, E. J., Zagdanski, J., Jia, M. M., and Tyler, P. (2023). Crowdsourced data indicates broadband has a positive impact on local business creation. Telemat. Inform. 84, 102035. doi: 10.1016/j.tele.2023.102035
Cheng, J. Z., Tsyu, J. Z., and Hsiao-Cheng, D. Y. (2003). Boom and gloom in the global telecommunications industry. Technol. Soc. 25, 65–81. doi: 10.1016/S0160-791X(02)00060-X
Chiaraviglio, L., Blefari-Melazzi, N., Liu, W., Gutiérrez, J. A., Van De Beek, J., Birke, R., et al. (2017). Bringing 5G into rural and low-income areas: Is it feasible? IEEE Commun. Stand. Magaz. 1, 50–57. doi: 10.1109/MCOMSTD.2017.1700023
Chiha, A., Van der Wee, M., Colle, D., and Verbrugge, S. (2020). Network slicing cost allocation model. J. Netw. Syst. Manage. 28, 627–659. doi: 10.1007/s10922-020-09522-3
Colman-Meixner, C., Khalili, H., Antoniou, K., Siddiqui, M. S., Papageorgiou, A., Albanese, A., et al. (2019). Deploying a novel 5G-enabled architecture on city infrastructure for ultra-high definition and immersive media production and broadcasting. IEEE Trans. Broadc. 65, 392–403. doi: 10.1109/TBC.2019.2901387
Commission, B. (2022). The State of Broadband 2022: Accelerating broadband for new realities. Available online at: https://www.broadbandcommission.org/publication/state-of-broadband-2022/ (accessed September 30, 2023).
Comşa, I. S., Zhang, S., Aydin, M. E., Kuonen, P., Lu, Y., Trestian, R., et al. (2018). Towards 5G: A reinforcement learning-based scheduling solution for data traffic management. IEEE Trans. Netw. Serv. Manag. 15, 1661–1675. doi: 10.1109/TNSM.2018.2863563
de la Torre, D., Ortiz-Gomez, F. G., Salas-Natera, M., and Martnez, R. (2020). “Analysis of the traffic demand on very high throughput satellite for 5G,” in Conference: Simposio Nacional de la Union Cientifica Internacional de Radio, URSI 1–4.
Duan, L., Huang, J., and Walrand, J. (2013). “Economic analysis of 4G network upgrade,”? in 2013 Proceedings IEEE INFOCOM 1070–1078. doi: 10.1109/INFCOM.2013.6566897
Fernández-Fernández, A., Colman-Meixner, C., Ochoa-Aday, L., Betzler, A., Khalili, H., Siddiqui, M. S., et al. (2021). Validating a 5G-enabled neutral host framework in city-wide deployments. Sensors 21, 8103. doi: 10.3390/s21238103
Filali, A., Abouaomar, A., Cherkaoui, S., Kobbane, A., and Guizani, M. (2020). Multi-access edge computing: A survey. IEEE Access 8, 197017–197046. doi: 10.1109/ACCESS.2020.3034136
Firli, A., Primiana, I., and Kaltum, U. (2015). The impact of increasing CAPEX on customer number, profit, and ROI in Indonesia telecommunication industry. Am. J. Econ. 5, 135–138. doi: 10.5923/c.economics.201501.14
Foukas, X., Patounas, G., Elmokashfi, A., and Marina, M. K. (2017). Network slicing in 5G: Survey and challenges. IEEE Commun. Magaz. 55, 94–100. doi: 10.1109/MCOM.2017.1600951
Fourati, F., Alsamhi, S. H., and Alouini, M.-S. (2022). Bridging the urban-rural connectivity gap through intelligent space, air, and ground networks. arXiv preprint arXiv:2202.12683.
Frank, H., Colman-Meixner, C., Assis, K. D. R., Yan, S., and Simeonidou, D. (2022). Techno-economic analysis of 5G non-public network architectures. IEEE Access 10, 70204–70218. doi: 10.1109/ACCESS.2022.3187727
Freeman, J., Park, S., Middleton, C., and Allen, M. (2016). The importance of broadband for socio-economic development: A perspective from rural Australia. Austral. J. Inf. Syst. 20, 1192. doi: 10.3127/ajis.v20i0.1192
Frias, Z., Mendo, L., and Oughton, E. J. (2020). How does spectrum affect mobile network deployments? empirical analysis using crowdsourced big data. IEEE Access 8, 190812–190821. doi: 10.1109/ACCESS.2020.3031963
Frisanco, T., Tafertshofer, P., Lurin, P., and Ang, R. (2008). “Infrastructure sharing and shared operations for mobile network operators from a deployment and operations view,”? in NOMS IEEE Network Operations and Management Symposium 129–136. doi: 10.1109/ICC.2008.419
Ghosh, A., Maeder, A., Baker, M., and Chandramouli, D. (2019). 5G evolution: A view on 5G cellular technology beyond 3GPP release 15. IEEE Access 7, 127639–127651. doi: 10.1109/ACCESS.2019.2939938
Giambene, G., Addo, E. O., and Kota, S. (2019). 5G aerial component for IoT support in remote rural areas,”? in IEEE 2nd 5G World Forum (5GWF) 572–577. doi: 10.1109/5GWF.2019.8911667
Gomes, A., Kibiłda, J., Farhang, A., Farrell, R., and DaSilva, L. A. (2021). Multi-operator connectivity sharing for reliable networks: A data-driven risk analysis. IEEE Trans. Netw. Serv. Manag. 18, 2800–2811. doi: 10.1109/TNSM.2021.3073841
Gómez, J., Pérez-Aradros, B., and Salazar, I. (2022). How to beat early movers: The role of competitive strategy and industry dynamism on followers performance in the telecommunications industry. Long Range Plann. 55, 102244. doi: 10.1016/j.lrp.2022.102244
Grijpink, F., Kutcher, E., Menard, A., Ramaswamy, S., Schiavotto, D., and Manyika, J. (2020). Connected world: An evolution in connectivity beyond the 5G revolution. Available online at: https://www.mckinsey.com/industries/technology-media-and-telecommunications/our-insights/connected-world-an-evolution-in-connectivity-beyond-the-5g-revolution?cid=other-eml-alt-mip-mckandhdpid=8502adaa-835f-4a4e-b18c-26a33728ba02andhctky=9280917andhlkid=49c1016cdb1449a6906270a96e03c458 (accessed September 30, 2023).
GSA (2022). Prices paid for spectrum in C-band, millimetre-wave and 700 MHz during auctions. Available online at: https://www.telecomlead.com/5g/prices-paid-for-spectrum-in-c-band-millimetre-wave-and-700-mhz-during-auctions-106337 (accessed September 30, 2023).
GSMA (2018). Case Study: CCS helping Telefonica enable Neutral Host Networks in London. Available online at: https://www.gsma.com/futurenetworks/wiki/ccs-case-study/ (accessed September 30, 2023).
GSMA (2019a). GSMA infrastructure sharing: An overview of future networks. Available online at: https://www.gsma.com/futurenetworks/wiki/infrastructure-sharing-an-overview/ (accessed September 30, 2023).
GSMA (2019b). Infrastructure Sharing: An Overview. Available online at: https://www.gsma.com/futurenetworks/wiki/infrastructure-sharing-an-overview/ (accessed September 30, 2023).
Handforth, C. (2019). GSMA closing the coverage gap: How innovation can drive rural connectivity, mobile for development. Available online at: https://www.gsma.com/mobilefordevelopment/resources/closing-the-coverage-gap-how-innovation-can-drive-rural-connectivity/ (accessed September 30, 2023).
Holmes, H., and Burgess, G. (2020). Opinion: Coronavirus has intensified the UKs digital divide. Available online at: https://www.cam.ac.uk/stories/digitaldivide (accessed September 30, 2023).
Hossain, E., and Hasan, M. (2015). 5G cellular: Key enabling technologies and research challenges. IEEE Instrument. Measur. Magaz. 18, 11–21. doi: 10.1109/MIM.2015.7108393
Hunukumbure, M., Coon, J. P., Allen, B., and Vernon, T. (2022). The Business of a Mobile Operator. New York, NY: Wiley-IEEE Press.
Ioannou, N., Katsianis, D., and Varoutas, D. (2020). Comparative techno-economic evaluation of LTE fixed wireless access, FTTdp G. fast and FTTC VDSL network deployment for providing 30 Mbps broadband services in rural areas. Telecommun. Policy 44, 101875. doi: 10.1016/j.telpol.2019.101875
ITU (2021). ITU ICT price basket. Available online at: https://www.itu.int/en/ITU-D/Statistics/Dashboards/Pages/IPB.aspx (accessed September 30, 2023).
ITU (2022). ITU global connectivity report. Available online at: https://www.itu.int/itu-d/reports/statistics/global-connectivity-report-2022/ (accessed September 30, 2023).
Jang, Y. (2022). 5G; Study on channel model for frequencies from 0.5 to 100 GHz. Available online at: https://www.3gpp.org/DynaReport/38901.htm (accessed September 30, 2023).
Jeanjean, F. (2022). Co-investment in the sharing of telecommunications infrastructures. Theor. Econ. Lett. 12, 1297–1314. doi: 10.4236/tel.2022.125070
Jha, A., and Saha, D. (2019). “Coverage and capacity dynamics in 4G-LTE deployment in India,”? in ICEIC (IEEE), 1–8. doi: 10.23919/ELINFOCOM.2019.8706452
Jiang, W., Han, B., Habibi, M. A., and Schotten, H. D. (2021). The road towards 6G: A comprehensive survey. IEEE Open J. Commun. Soc. 2, 334–366. doi: 10.1109/OJCOMS.2021.3057679
Kaloxylos, A. (2018). A survey and an analysis of network slicing in 5G networks. IEEE Commun. Stand. Magaz. 2, 60–65. doi: 10.1109/MCOMSTD.2018.1700072
Kenechi, O., and Stefano, S. (2022). The Mobile Economy 2022. Available online at: https://data.gsmaintelligence.com/research/research/research-2022/the-mobile-economy-2022 (accessed September 30, 2023).
Khaturia, M., Jha, P., and Karandikar, A. (2020). Connecting the unconnected: Toward Frugal 5G network architecture and standardization. IEEE Commun. Stand. Magaz. 4, 64–71. doi: 10.1109/MCOMSTD.001.1900006
Khodashenas, P. S., Aznar, J., Legarrea, A., Ruiz, C., Siddiqui, M. S., Escalona, E., et al. (2016). “5G network challenges and realization insights,” in 2016 18th International Conference on Transparent Optical Networks 1–4. doi: 10.1109/ICTON.2016.7550539
Kliks, A., Musznicki, B., Kowalik, K., and Kryszkiewicz, P. (2018). Perspectives for resource sharing in 5G networks. Telecommun. Syst. 68, 605–619. doi: 10.1007/s11235-017-0411-3
Kumar, S. K. A., Ihita, G. V., Chaudhari, S., and Arumugam, P. (2022). “A survey on rural internet connectivity in India,”? in 2022 14th International Conference on COMmunication Systems and NETworkS (COMSNETS) (IEEE), 911–916. doi: 10.1109/COMSNETS53615.2022.9668358
Kusuma, A. A., and Suryanegara, M. (2019). “Upgrading mobile network to 5G: The technoeconomic analysis of main cities in Indonesia,”? in 16th International Conference on Quality in Research: International Symposium on Electrical and Computer Engineering 1–6. doi: 10.1109/QIR.2019.8898260
Lähteenmäki, J. (2021). The evolution paths of neutral host businesses: Antecedents, strategies, and business models. Telecommun. Policy 45, 102201. doi: 10.1016/j.telpol.2021.102201
Laitsou, E., Ioannou, N., and Katsianis, D. (2022). 5G fixed Wireless Access for Rural Broadband. Calgary: International Telecommunications Society (ITS).
Lappalainen, A., and Rosenberg, C. (2022). Can 5G fixed broadband bridge the rural digital divide? IEEE Commun. Stand. Magaz. 6, 79–84. doi: 10.1109/MCOMSTD.0001.2100092
Lee, D., Zhou, S., Zhong, X., Niu, Z., Zhou, X., and Zhang, H. (2014). Spatial modeling of the traffic density in cellular networks. IEEE Wirel. Commun. 21, 80–88. doi: 10.1109/MWC.2014.6757900
Lee, W., Na, T., and Kim, J. (2019). “How to create a network slice? A 5G core network perspective,”? in 2019 21st International Conference on Advanced Communication Technology (ICACT) 616–619. doi: 10.23919/ICACT.2019.8701936
Lehr, W., and Stocker, V. (2023). Next-generation networks: Necessity of edge sharing. Front. Comput. Sci. 5, 1099582. doi: 10.3389/fcomp.2023.1099582
Lim, S. S. (2020). NR; User Equipment (UE) radio access capabilities - Specification 38.306. Available online at: https://portal.3gpp.org/desktopmodules/Specifications/SpecificationDetails.aspx?specificationId=3193 (accessed September 30, 2023).
Lin, X. (2022). An overview of 5G advanced evolution in 3GPP release 18. IEEE Commun. Stand. Magaz. 6, 77–83. doi: 10.1109/MCOMSTD.0001.2200001
Lun, J., Frenger, P., Furuskar, A., and Trojer, E. (2019). “5G new radio for rural broadband: how to achieve long-range coverage on the 3.5 GHz band,”? in 2019 IEEE 90th Vehicular Technology Conference (VTC2019-Fall) (IEEE), 1–6. doi: 10.1109/VTCFall.2019.8891556
Luong, N. C., Wang, P., Niyato, D., Liang, Y. C., Han, Z., and Hou, F. (2019). Applications of economic and pricing models for resource management in 5G wireless networks: A survey. IEEE Commun. Surv. Tutor. 21, 3298–3339. doi: 10.1109/COMST.2018.2870996
Mach, P., and Becvar, Z. (2017). Mobile edge computing: A survey on architecture and computation offloading. IEEE Commun. Surv. Tutor. 19, 1628–1656. doi: 10.1109/COMST.2017.2682318
Maeng, K., Kim, J., and Shin, J. (2020). Demand forecasting for the 5G service market considering consumer preference and purchase delay behavior. Telem. Inform. 47, 101327. doi: 10.1016/j.tele.2019.101327
Mark, J. (2021). H2 2020 take-up of the UK superfast broadband rollout project. Available online at: https://www.ispreview.co.uk/index.php/2021/03/h2-2020-take-up-of-the-uk-superfast-broadband-rollout-project.html (accessed September 30, 2023).
Marketing Group, M. (2022). World internet users statistics and 2020 world population stats. Available online at: https://www.internetworldstats.com/stats.htm (accessed September 30, 2023).
Matinmikko, M., Latva-Aho, M., Ahokangas, P., Yrjölä, S., and Koivumäki, T. (2017). Micro operators to boost local service delivery in 5G. Wirel. Personal Commun. 95, 69–82. doi: 10.1007/s11277-017-4427-5
Matinmikko-Blue, M., and Latva-aho, M. (2017). “Micro operators accelerating 5G deployment,”? in ICIIS (IEEE), 1–5. doi: 10.1109/ICIINFS.2017.8300396
Matinmikko-Blue, M., Yrjölä, S., Ahokangas, P., Seppänen, V., Hämmäinen, H., and Jurva, R. (2019). Value of the Spectrum for Local Mobile Communication Networks: Insights Into Awarding and Pricing the 5G Spectrum Bands. Calgary: International Telecommunications Society (ITS).
Matinmikko-Blue, M., Yrjölä, S., Seppänen, V., Ahokangas, P., Hämmäinen, H., and Latva-aho, M. (2018). “Analysis of spectrum valuation approaches: The viewpoint of local 5G networks in shared spectrum bands,” in 2018 IEEE International Symposium on Dynamic Spectrum Access Networks (DySPAN) (IEEE), 1–9. doi: 10.1109/DySPAN.2018.8610409
Meddour, D.-E., Rasheed, T., and Gourhant, Y. (2011). On the role of infrastructure sharing for mobile network operators in emerging markets. Comput. Netw. 55, 1576–1591. doi: 10.1016/j.comnet.2011.01.023
Morris, L. (2022). How Europe's biggest telcos lost control of their towers. Available online at: https://www.lightreading.com/5g/how-europes-biggest-telcos-lost-control-of-their-towers/a/d-id/779072 (accessed September 30, 2023).
Musacchio, J., Walrand, J., and Wu, S. (2006). “A game theoretic model for network upgrade decisions,”? in Proceedings of the 44th Annual Allerton Conference on Communication, Control, and Computing, Monticello, IL 191–200.
Noll, J., Dixit, S., Radovanovic, D., Morshedi, M., Holst, C., and Winkler, A. S. (2018). “5G network slicing for digital inclusion,”? in 2018 10th International Conference on Communication Systems and Networks (COMSNETS) (IEEE), 191–197. doi: 10.1109/COMSNETS.2018.8328197
Nor, A. M., Fratu, O., and Halunga, S. (2022). Quality of service based radio resources scheduling for 5g embb use case. Symmetry 14, 2193. doi: 10.3390/sym14102193
OFCOM (2020). Connected nations 2020. Available online at: https://www.ofcom.org.uk/research-and-data/multi-sector-research/infrastructure-research/connected-nations-2020 (accessed September 30, 2023).
Oladejo, S. O., and Falowo, O. E. (2017). “5G network slicing: A multi-tenancy scenario,”? in GWS (IEEE), 88–92. doi: 10.1109/GWS.2017.8300476
Oproiu, E. M., Iordache, M., Costea, C., Brezeanu, C., and Patachia, C. (2018). “5G network architecture, functional model and business role for 5G smart city use case: Mobile operator perspective,”? in COMM 361–366. doi: 10.1109/ICComm.2018.8484747
Osio, J. (2021). US C-band auction becomes world's costliest mid-band 5G auction yet. Available online at: https://www.spglobal.com/marketintelligence/en/news-insights/research/us-c-band-auction-becomes-worlds-costliest-mid-band-5g-auction-yet (accessed September 30, 2023).
Osoro, O. B., Oughton, E. J., Wilson, A. R., and Rao, A. (2023). Sustainability assessment of low earth orbit (LEO) satellite broadband mega-constellations. https://arxiv.org/abs/2309.02338 (accessed September 30, 2023).
Oughton, E. (2020a). CDCAM: Cambridge digital communications assessment model. J. Open Source Softw. 5, 1911. doi: 10.21105/joss.01911
Oughton, E. (2020b). ITMLOGIC: The irregular terrain model by Longley and Rice. J. Open Source Softw. 5, 2266. doi: 10.21105/joss.02266
Oughton, E., Amaglobeli, D., and Moszoro, M. (2023). Estimating digital infrastructure investment needs to achieve universal broadband. IMF Working Paper. doi: 10.2139/ssrn.4472068
Oughton, E. J. (2023). Policy options for broadband infrastructure strategies: A simulation model for affordable universal broadband in Africa. Telem. Inform. 76, 101908. doi: 10.1016/j.tele.2022.101908
Oughton, E. J., Boch, E., and Kusuma, J. (2022a). Engineering-economic evaluation of diffractive NLOS backhaul (e3nb): A techno-economic model for 3D wireless backhaul assessment. IEEE Access 10, 3430–3446. doi: 10.1109/ACCESS.2022.3140421
Oughton, E. J., Comini, N., Foster, V., and Hall, J. W. (2022b). Policy choices can help keep 4G and 5G universal broadband affordable. Technol. Forecast. Soc. Change 176, 121409. doi: 10.1016/j.techfore.2021.121409
Oughton, E. J., and Frias, Z. (2018). The cost, coverage and rollout implications of 5G infrastructure in Britain. Telecommun. Policy 42, 636–652. doi: 10.1016/j.telpol.2017.07.009
Oughton, E. J., Frias, Z., van der Gaast, S., and van der Berg, R. (2019a). Assessing the capacity, coverage and cost of 5G infrastructure strategies: Analysis of the Netherlands. Telem. Inform. 37, 50–69. doi: 10.1016/j.tele.2019.01.003
Oughton, E. J., and Jha, A. (2021). Supportive 5G infrastructure policies are essential for universal 6G: Assessment using an open-source techno-economic simulation model utilizing remote sensing. IEEE Access 9, 101924–101945. doi: 10.1109/ACCESS.2021.3097627
Oughton, E. J., Katsaros, K., Entezami, F., Kaleshi, D., and Crowcroft, J. (2019b). An open-source techno-economic assessment framework for 5G deployment. IEEE Access 7, 155930–155940. doi: 10.1109/ACCESS.2019.2949460
Oughton, E. J., and Lehr, W. (2022). Surveying 5G techno-economic research to inform the evaluation of 6G wireless technologies. IEEE Access 10, 25237–25257. doi: 10.1109/ACCESS.2022.3153046
Oughton, E. J., Lehr, W., Katsaros, K., Selinis, I., Bubley, D., and Kusuma, J. (2021). Revisiting Wireless Internet Connectivity: 5G vs Wi-Fi 6. Telecommun. Policy 45, 102127. doi: 10.1016/j.telpol.2021.102127
Oughton, E. J., and Mathur, J. (2021). Predicting cell phone adoption metrics using machine learning and satellite imagery. Telem. Inform. 62, 101622. doi: 10.1016/j.tele.2021.101622
Oughton, E. J., and Russell, T. (2020). The importance of spatio-temporal infrastructure assessment: Evidence for 5G from the Oxford-Cambridge arc. Comput. Environ. Urban Syst. 83, 101515. doi: 10.1016/j.compenvurbsys.2020.101515
Paglierani, P., Neokosmidis, I., Rokkas, T., Meani, C., Nasr, K. M., Moessner, K., et al. (2020). Techno-economic analysis of 5G immersive media services in cloud-enabled small cell networks: The neutral host business model. Trans. Emer. Telecommun. Technol. 31, e3746. doi: 10.1002/ett.3746
Pápai, Z., McLean, A., Nagy, P., Szabó, G., and Csorba, G. (2022). The impact of network sharing on competition: the challenges posed by 5G. Dig. Policy Regul. Gover. 24, 274–291. doi: 10.1108/DPRG-02-2021-0040
Parkvall, S. (2023). Evolved Universal Terrestrial Radio Access (E-UTRA); Physical channels and modulation. Available online at: https://portal.3gpp.org/desktopmodules/Specifications/SpecificationDetails.aspx?specificationId=2425 (accessed September 30, 2023).
Partners, G. R. (2019). 5G RuralFirst: Project conclusion report. Available online at: https://www.5gruralfirst.org/project-conclusion-report/ (accessed September 30, 2023).
Perez Guirao, M. D., Wilzeck, A., Schmidt, A., Septinus, K., and Thein, C. (2017). Locally and temporary shared spectrum as opportunity for vertical sectors in 5G. IEEE Netw. 31, 24–31. doi: 10.1109/MNET.2017.1700079
Pries, R., Morper, H. J., Galambosi, N., and Jarschel, M. (2016). “Network as a service-a demo on 5G network slicing,”? in 2016 28th International Teletraffic Congress (ITC 28) (IEEE), 209–211. doi: 10.1109/ITC-28.2016.136
Pryce, J. (2022). Neutral host: Spectrum. Available online at: https://5gruraldorset.org/2021/07/19/2204/ (accessed September 30, 2023).
Psyrris, A., Kargas, A., and Varoutas, D. (2021). “MNOs business models and roles enabled by 5G technologies and use cases: Transformation, challenges and strategies,”? in 2021 14th CMI International Conference- Critical ICT Infrastructures and Platforms (CMI) 1–11. doi: 10.1109/CMI53512.2021.9663842
Quadri, C., Premoli, M., Ceselli, A., Gaito, S., and Rossi, G. P. (2020). Optimal assignment plan in sliced backhaul networks. IEEE Access 8, 68983–69002. doi: 10.1109/ACCESS.2020.2986535
Ramasetty, P., and Masilamani, S. (2019). “5G rural strategy in India,”? in Optical Fiber Communication Conference (OFC) 2019 (Optica Publishing Group), M1G.4. doi: 10.1364/OFC.2019.M1G.4
Randell-Moon, H. E. K., and Hynes, D. (2022). Too smart: Infrastructuring the Internet through regional and rural smart policy in Australia. Policy Internet 14, 151–169. doi: 10.1002/poi3.286
Raza, U., Usman, M., Asghar, M. R., Ansari, I. S., and Granelli, F. (2019). “Integrating public safety networks to 5G: Applications and standards,”? in Enabling 5G Communication Systems to Support Vertical Industries 233–251. doi: 10.1002/9781119515579.ch11
Saarnisaari, H., Dixit, S., Alouini, M. S., Chaoub, A., Giordani, M., Kliks, A., et al. (2020). A 6G white paper on connectivity for remote areas. arXiv preprint arXiv:2004.14699.
Saha, R. K. (2020). Licensed countrywide full-spectrum allocation: A new paradigm for millimeter-wave mobile systems in 5G/6G era. IEEE Access 8, 166612–166629. doi: 10.1109/ACCESS.2020.3023342
Sahoo, D. K., and Sahoo, P. K. (2022). Efficiency, productivity dynamics and determinants of productivity growth in Indian telecommunication industries: An empirical analysis. J. Public Affairs 22, e2353. doi: 10.1002/pa.2353
Samdanis, K., Costa-Perez, X., and Sciancalepore, V. (2016). From network sharing to multi-tenancy: The 5G network slice broker. IEEE Commun. Magaz. 54, 32–39. doi: 10.1109/MCOM.2016.7514161
Sanguanpuak, T., Niyato, D., Rajatheva, N., and Latva-Aho, M. (2019). Radio resource sharing and edge caching with latency constraint for local 5G operator: Geometric programming meets stackelberg game. IEEE Trans. Mobile Comput. 20, 707–721. doi: 10.1109/TMC.2019.2948630
Schneir, J. R., Ajibulu, A., Konstantinou, K., Bradford, J., Zimmermann, G., Droste, H., et al. (2019). A business case for 5G mobile broadband in a dense urban area. Telecommun. Policy 43, 101813. doi: 10.1016/j.telpol.2019.03.002
Schneir, J. R., Bradford, J., Ajibulu, A., Pearson, K., Konstantinou, K., Osman, H., et al. (2022). A business case for 5G services in an industrial sea port area. Telecommun. Policy 46, 102264. doi: 10.1016/j.telpol.2021.102264
Schneir, J. R., Mlleryd, B. G., Oughton, E., and Mas-Machuca, C. (2023). Guest editorial: Techno-economic analysis of telecommunications systems. IEEE Commun. Magaz. 61, 22–23. doi: 10.1109/MCOM.2023.10047852
Schneir, J. R., and Xiong, Y. (2016). A cost study of fixed broadband access networks for rural areas. Telecommun. Policy 40, 755–773. doi: 10.1016/j.telpol.2016.04.002
Sciancalepore, V., Samdanis, K., Costa-Perez, X., Bega, D., Gramaglia, M., and Banchs, A. (2017). “Mobile traffic forecasting for maximizing 5G network slicing resource utilization,”? in INFOCOM 1–9. IEEE. doi: 10.1109/INFOCOM.2017.8057230
Sciancalepore, V., Zanzi, L., Costa-Perez, X., and Capone, A. (2022). ONETS: online network slice broker from theory to practice. IEEE Trans. Wirel. Commun. 21, 121–134. doi: 10.1109/TWC.2021.3094116
Series, M. (2017). Minimum requirements related to technical performance for IMT-2020 radio interface(s). Report 2410–0.
Shen, X., Gao, J., Wu, W., Lyu, K., Li, M., Zhuang, W., et al. (2020). Ai-assisted network-slicing based next-generation wireless networks. IEEE Open J. Vehic. Technol. 1, 45–66. doi: 10.1109/OJVT.2020.2965100
Shruthi, K. A. (2023). Shruthika-kas / infrasharing5gupgrade. Available online at: https://github.com/shruthiKa-kas/infraSharing5Gupgrade (accessed September 30, 2023).
Shruthi, K. A., Sachin, C., Robert, S., and David, C. (2021). Techno-economic study of 5G network slicing to improve rural connectivity in India. IEEE Open J. Commun. Soc. 2, 2645–2659. doi: 10.1109/OJCOMS.2021.3131370
Simon, K. (2022). Digital 2022: Global overview report. Available online at: https://datareportal.com/reports/digital-2022-global-overview-report (accessed September 30, 2023).
Smail, G., and Weijia, J. (2017). “Techno-economic analysis and prediction for the deployment of 5G mobile network,”? in 20th Conference on ICIN 9–16. doi: 10.1109/ICIN.2017.7899243
Steve (2015). Telephony terms explained: Erlang &Erlang B. Available online at: https://www.unifiedcommunications.nl/2015/01/27/telephony-terms-explained-erlang-erlang-b/ (accessed September 30, 2023).
Suryanegara, M. (2018). “The Economics of 5G: Shifting from Revenue-per-User to Revenue-per-Machine,”? in International Symposium on Communications and Information Technologies (ISCIT) 191–194. doi: 10.1109/ISCIT.2018.8588006
Taylor, P. (2023). Mobile ARPU per SIM card by country from 2015 to 2020. Available online at: https://www.statista.com/statistics/668966/mobile-average-revenue-per-user-by-country/ (accessed September 30, 2023).
Tran, T. D., and Le, L. B. (2020). Resource allocation for multi-tenant network slicing: A multi-leader multi-follower Stackelberg game approach. IEEE Trans. Vehic. Technol. 69, 8886–8899. doi: 10.1109/TVT.2020.2996966
Tseliou, G., Adelantado, F., and Verikoukis, C. (2019). NetSliC: Base station agnostic framework for network slicing. IEEE Trans. Vehic. Technol. 68, 3820–3832. doi: 10.1109/TVT.2019.2902320
UN (2022). Take Action for the Sustainable Development Goals - United Nations Sustainable Development. Available online at: https://www.un.org/sustainabledevelopment/sustainable-development-goals/ (accessed September 30, 2023).
van Kranenburg, H. H., and Hagedoorn, J. (2008). Strategic focus of incumbents in the European telecommunications industry: The cases of BT, Deutsche Telekom and KPN. Telecommun. Policy 32, 116–130. doi: 10.1016/j.telpol.2007.08.005
Veligura, N. V., Chan, K. K. K., Van Ingen, F. M. P., and Cufre, G. (2020). COVID-19 impact on the global telecommunications industry. Policy Commons.
Vinogradov, O. (2022). 5G NR Throughput calculator bar 5G-Tools.com. Available online at: https://5g-tools.com/5g-nr-throughput-calculator/ (accessed September 30, 2023).
Wahyudin, A., Hikmaturokhman, A., and Harish, D. A. (2021). “Spectrum fee license analysis on 3.5, 26, and 28 GHz frequency for 5G implementation in Indonesia?” in International Conferences on Communication, Networks and Satellite (COMNETSAT) (IEEE), 274–279. doi: 10.1109/COMNETSAT53002.2021.9530804
Walia, J. S., Hämmäinen, H., Kilkki, K., and Yrjölä, S. (2019). 5G network slicing strategies for a smart factory. Comput. Ind. 111, 108–120. doi: 10.1016/j.compind.2019.07.006
Walia, J. S., Hämmäinen, H., and Matinmikko, M. (2017). “5G micro-operators for the future campus: A techno-economic study,”? in Internet of Things Business Models, Users, and Networks (IEEE), 1–8. doi: 10.1109/CTTE.2017.8260985
Wang, Y., Gu, Y., and Tao, X. (2019). Edge network slicing with statistical QoS provisioning. IEEE Wirel. Commun. Lett. 8, 1464–1467. doi: 10.1109/LWC.2019.2922605
Wang, Y., Li, J., Huang, L., Jing, Y., Georgakopoulos, A., and Demestichas, P. (2014). 5G mobile: Spectrum broadening to higher-frequency bands to support high data rates. IEEE Vehic. Technol. Magaz. 9, 39–46. doi: 10.1109/MVT.2014.2333694
Yaacoub, E., and Alouini, M.-S. (2020). A key 6G challenge and opportunity connecting the base of the pyramid: A survey on rural connectivity. Proc. IEEE 108, 533–582. doi: 10.1109/JPROC.2020.2976703
Yaghoubi, F., Mahloo, M., Wosinska, L., Monti, P., de Souza Farias, F., Costa, J. C. W. A., et al. (2018). A techno-economic framework for 5G transport networks. IEEE Wirel. Commun. 25, 56–63. doi: 10.1109/MWC.2018.1700233
Yang, S. (2022). Equity research-BT Group PLC. PhD thesis, Instituto Superior de Economia e Gest ao.
Ye, S., and Tiong, R. L. (2000). NPV-at-risk method in infrastructure project investment evaluation. J. Constr. Eng. Manag. 126, 227–233. doi: 10.1061/(ASCE)0733-9364(2000)126:3(227)
Yusuf, A. S., Muhammad, A. I., David, C., Robert, S., and Stephen, S. (2021). Can wireless technology alone blur the digital divide? Available online at: https://www.comsoc.org/publications/ctn/can-wireless-technology-alone-blur-digital-divide (accessed September 30, 2023).
Zainal, Z. (2022). Zulfadli zainal/5g-nr-planning-and-dimensioning. Available online at: https://github.com/zulfadlizainal/nr-dimensioning (accessed September 30, 2023).
Zhang, H., Liu, N., Chu, X., Long, K., Aghvami, A. H., and Leung, V. C. (2017). Network slicing based 5G and future mobile networks: mobility, resource management, and challenges. IEEE Commun. Magaz. 55, 138–145. doi: 10.1109/MCOM.2017.1600940
Zhang, L., Mei, C., Li, J., Liang, Y., and Song, J. (2019). “A survey on 5G network slicing enabling the smart grid,”? in 2019 IEEE 25th International Conference on Parallel and Distributed Systems (ICPADS) (IEEE), 911–916.
Zhang, S. (2019). An overview of network slicing for 5G. IEEE Wirel. Commun. 26, 111–117. doi: 10.1109/MWC.2019.1800234
Zulfadli, Z. (2022). 5G NR Planning and Dimensioning Simulations. Available online at: https://github.com/zulfadlizainal/nr-dimensioning (accessed September 30, 2023).
Keywords: 5G, network slicing, network upgrade, rural connectivity, techno-economic feasibility, wireless and mobile technology
Citation: Koratagere Anantha Kumar S and Oughton EJ (2023) Techno-economic assessment of 5G infrastructure sharing business models in rural areas. Front. Comput. Sci. 5:1191853. doi: 10.3389/fcomp.2023.1191853
Received: 22 March 2023; Accepted: 28 September 2023;
Published: 20 October 2023.
Edited by:
Dimitris Varoutas, National and Kapodistrian University of Athens, GreeceReviewed by:
Jason Whalley, Northumbria University, United KingdomCopyright © 2023 Koratagere Anantha Kumar and Oughton. This is an open-access article distributed under the terms of the Creative Commons Attribution License (CC BY). The use, distribution or reproduction in other forums is permitted, provided the original author(s) and the copyright owner(s) are credited and that the original publication in this journal is cited, in accordance with accepted academic practice. No use, distribution or reproduction is permitted which does not comply with these terms.
*Correspondence: Shruthi Koratagere Anantha Kumar, ay5hLnNocnV0aGlAc3RyYXRoLmFjLnVr
Disclaimer: All claims expressed in this article are solely those of the authors and do not necessarily represent those of their affiliated organizations, or those of the publisher, the editors and the reviewers. Any product that may be evaluated in this article or claim that may be made by its manufacturer is not guaranteed or endorsed by the publisher.
Research integrity at Frontiers
Learn more about the work of our research integrity team to safeguard the quality of each article we publish.