- 1OASIS Research Unit, Faculty of Information Technology and Electronic Engineering, University of Oulu, Oulu, Finland
- 2Navico Oy, Oulu, Finland
Owing to increasing cases of sedentary lifestyles and their negative impact on health, practical solutions are needed to address the physical and mental wellbeing of citizens and to enhance their standard of living. Among the problems are premature mortality rates caused by physical inactivity, which leads to chronic diseases. Innovative solutions are needed to address many of the problems that we face as a society. Location-based games have been identified as effective solutions for increasing physical activity, enhancing social interaction, and exploration in urban environments. In this pilot study, we explore how to encourage active mobility (walking and cycling) through urban play by integrating technology into the built environment. We examined the usability of a web-based augmented reality application in providing interactive experience to users as they explored the urban environment looking for tasks. Overall, participants’ perceptions of the usability of the application were positive; they enjoyed how the application revealed the tasks at each location and all the checkpoints at the different locations had at least a couple of visitors. We present limitations and future research directions.
Introduction
Most of the world population living in urban areas continues to grow (United Nations, 2018). As the global population shifts to urban areas, there is an increased need for practical solutions to address the physical and mental wellbeing of citizens and to enhance the standard of living in urban environments. One of the major global challenges is premature mortality rates caused by physical inactivity and sedentary lifestyles, which lead to chronic diseases (Heath et al., 2006). According to World Health Organization (WHO), (n.d.), physical inactivity and sedentary behavior could partly be attributed to the effects of urbanization, which has led to detrimental factors such as increase in traffic, low air quality, and lack of recreational facilities. Reversing mortality rates resulting from physical inactivity and other lifestyle-related behaviors could enhance the overall health and mental wellbeing of the population and save healthcare costs (Heath et al., 2006; Lee and Maheswaran, 2011).
We need innovative solutions to address many of the problems that we face as a society (Morford et al., 2014). There has been a steady growth in systems aimed at motivating people to attain their goals by guiding them toward healthier behaviors (Chatterjee and Price, 2009). Digital interventions have transformed the sustainability, healthcare, and wellness sectors by enhancing users’ independence in improving their own wellbeing and overall health. Using technology in these different domains applying game mechanics to start and/or maintain self-beneficial behavior is nowadays commonplace (Morford et al., 2014; Hamari and Koivisto, 2015). Game mechanics are interactions through which players interact with a game or gamified system (Haahr, 2017). For example, Arjoranta, Kari, and Salo (2020) investigate how game features, such as catching new Pokémon and exploring Pokéstops, in a location-based game can be linked to specific behavior types by investigating users’ actual experiences while using the game.
This pilot study is part of an ongoing project on urban design and wellbeing. A major aim of the project is to investigate how to integrate technology into existing urban environments to encourage physical activity, city exploration, community awareness and to improve access to and the delivery of services. In this study, we examine the role of playful games in encouraging active mobility (walking and cycling) by using a web-based augmented reality (webAR) application to provide an enjoyable and interactive experience while exploring an urban environment. The study was set up as part of a family sports day where we analyzed the use of the application to engage users at the point of interaction (checkpoints) by revealing different tasks. We placed AR tags in 30 different locations across Oulu, a Finnish city in northern Finland, and scanning the tags revealed the tasks that participants were to do at each location. The specific focus was on 1) encouraging active mobility and exploration through interactive play, 2) exploiting characteristics of the urban environment, and 3) investigating users’ perception of the usability of the webAR application.
Research shows how the characteristics of urban environments can contribute positively to health by influencing people’s physical activity levels (Lopez 2012; Coombes, Sluijs, and Jones 2013; Sallis et al., 2016; Kärmeniemi et al., 2018). Environmental approaches promoting wellbeing may complement frequently used individual and lifestyle modification strategies because they can benefit all people exposed to the environment rather than focusing on influencing one person at a time (Heath et al., 2006). Such community-wide interventions are effective in increasing levels of mental wellbeing among the physically inactive and in promoting increased levels of physical activity (Coombes and Jones, 2016; Harris, 2018a, 2018b). Using locative media to move through familiar surroundings can also increase the quality of fun, social interaction, and exploration (Haahr, 2017; Korhonen et al., 2017; Papangelis et al., 2020; Tzima et al., 2021). However, the impact of locative media on physical activity tends to reduce over time (Broom and Flint, 2018) and research suggests that there are limited options or increased barriers for minority populations to play (Hjorth and Richardson, 2017).
This article reports on a study testing the usability of a webAR and how to enhance urban exploration and encourage active mobility thus augmenting other research on locative media (see, e.g., Kaczmarek et al., 2017; Broom and Flint 2018; Pang et al., 2019; Fonseca et al., 2021). The study also presents an example of how to integrate AR applications into existing urban environments to encourage play and physical activity at the point of interaction using the features present at a particular location.
Related Work
According to Chien et al. (2021), the most common research topics in location-based game research are the Urban Space (herein referred to as the built environment), Physical Activity, and Pokémon GO (AR). These are discussed in the following sections and are some of the key topics in the project and are important in this pilot study, which focuses on using fun and games to encourage active mobility in urban spaces.
Exploiting Features of the Built Environment to Promote Physical Activity
The topic Urban Space in relation to location-based game research focuses on where these games are played, and it is often (although not always) in the built environment (Chien et al., 2021). The built environment refers to the man-made elements that are part of everyday lives and Lopez (2012), for example, addresses the relationship between the built environment and health. The health effects of the built environment occur on multiple areas, such as houses, streets, neighborhoods, parks, and green spaces (Lopez, 2012). Growing evidence shows that some environments afford healthier living practices than others, and there are programs, policies, and projects that can change or be integrated into the environment to promote health (Lopez, 2012; Coombes et al., 2013; Sallis et al., 2016; Kärmeniemi et al., 2018). For example, objective Geographic Information System (GIS)-based measures of the built environment such as new infrastructure for walking, cycling or public transport, accessibility to sporting facilities, or in terms of distance to parks, recreational park improvements, and street network connectivity (Lee and Maheswaran, 2011; Kärmeniemi et al., 2018). Objective measures refer to improvements in neighborhood or town-level infrastructure (Kärmeniemi et al., 2018).
Urban areas can be designed to support various activities in outdoor and indoor environments including people’s mobility behaviors (Stibe and Larson, 2016). Examples of applying technology to outdoor environments include installations that transform public spaces into playgrounds that encourage social interactions. In Montreal, swings set up between two streets enable people to participate in a collective musical production in which a single moving swing sets off a note, and several create a melody (Figure 1A).1 To encourage cycling, street signs show an approximate number of calories burned between two points or the number of people who have passed through a specific location at a certain point in time (Figure 1B).2 In indoor environments, situated displays integrated with sensors could encourage people to use the stairs instead of the elevator (Stibe and Larson, 2016).
Below are a few examples of research that link characteristics of the environment with experimental studies that take advantage of objective built environment features to promote physical activity. Helbich et al. (2016) examined how the natural and built environments influence transport behavior by tracking children’s trips with a global positioning system. The results showed that the distance between locations was insignificant in children’s active travel to school if positive urban design features such as well-connected streets and cycling lanes were considered in the analyses. Coombes, Sluijs, and Jones (2013) showed that an activity’s intensity—whether light, moderate, or vigorous—and length depend on the characteristics of the built environment. In their study, they used accelerometers and global positioning systems in a sample of school children to investigate whether different intensities of activities occur in different environments (Coombes et al., 2013). The findings showed different environments—buildings, pavements, and green spaces (gardens, parks)—support varying intensities and lengths of activity (Coombes et al., 2013). In a later study, Coombes and Jones (2016) investigated how to get children more active by encouraging long-term sustainable mobility in their neighborhood and while traveling to schools. Using tracking technology and a reward scheme, they recruited the participating school children via an intervention and control school. They quantitatively tested the impact of the study that turns a city into a game where players register their walking or cycling journeys, on levels of active travel using objective measures of change in physical activity recorded by the tracking device. There was no large effect, but they observed that self-reported active travel increased at the intervention school compared to the control. Overall engagement with the system was low, but there was evidence that children who engaged more increased their physical activity times when commuting to school (Coombes and Jones, 2016). Investigating the same system and focusing on how physical activity is linked to mental wellbeing, Harris (2018a, 2018b) found comparable results to Coombes and Jones, (2016) on a more diverse sample—from school children under ten to adults over 70. The findings provided preliminary evidence that the system may be a promising approach to raising levels of mental wellbeing at a community-wide level for those who are physically inactive (Harris, 2018a; 2018b). However, future studies should measure a wider set of outcomes and use objective measures of physical activity (e.g., log data from activity trackers), in addition to exploring the mechanisms that underpin the relationship between physical activity and mental wellbeing (Harris, 2018a; 2018b).
Location-Based Games
Digital technologies’ importance in society is undeniable and technology increasingly “co-constitutes” our experience of the urban environment (Papangelis et al., 2020). As we increasingly interweave our lives with playful virtual environments, “mobile urban games can be seen as illustrative of the playful way in which we collectively and creatively perform place and our social selves” (Hjorth and Richardson, 2017).
Location-based games incorporate physical locations and resource gathering (involving physical movement) into the gaming experience (Haahr, 2017; Chien et al., 2021). In these games, location is tied to and accessed via specific geographic information. They encourage playful behavior by motivating people to discover places in their surroundings that they have not visited (Pang et al., 2019; Fonseca et al., 2021). Location-based games take place in the environment, promote citizen engagement, require exploration, and encourage physical activity (Kaczmarek et al., 2017; Broom and Flint, 2018; Pang et al., 2019; Fonseca et al., 2021; Tzima et al., 2021). However, in the same way that location-based games such as Pokémon GO lower social barriers and can be deployed to facilitate social interaction, they can also be used as a shield to avoid engagement with others in public spaces (Hjorth and Richardson, 2017).
Pang et al. (2019) investigate how location-based games can increase community awareness during transit. In the article, they investigate how users capture, share, and view location-based community information. Findings indicate that use of City Explorer, the application investigated in the study, did not adversely change users’ transit behaviors and most relied on their old ways during transit. In some cases, the recommendations from the application helped users explore nearby places, but most users did not feel the need to socially connect with other commuters during transit as it was considered their personal time. What they valued most from using City Explorer was the increased awareness of their travel routines (Pang et al., 2019).
Fonseca et al. (2021) propose design recommendations for serious games to promote social interaction and citizen engagement. According to Fonseca et al. (2021), even though it is known why digital serious games to increase social interaction are successful, there is hardly any information on how and why design choices are made and how they affect players’ behavior. Consequently, there are no concrete design guidelines for meaningful social interactions. The study focuses on teenagers’ meaningful interaction with the environment and strengthening the engagement with their own neighborhood. The teenagers take part in the design, development, and evaluation of a location-based game framework, which results in design guidelines for games for social interaction (Fonseca et al., 2021).
While location-based AR games have existed for more than a decade, it is only with the launch of Ingress and Pokémon GO that the genre entered the public mainstream (Haahr, 2017). No location-based mobile game has attracted as much attention as Pokémon GO, and it has been prominent in academic research from the time of its release in 2016 (Chien et al., 2021). Pokémon GO has primarily been investigated in the context of encouraging physical activity, social interaction, and exploration (Chien et al., 2021).
Broom and Flint (2018) investigated differences in physical activity, sitting time, and perceptions of physical activity and health between users and nonusers of Pokémon GO in a pre-and-post-test study—launch of the application in the United Kingdom and 3-month follow-up. Using Pokémon GO increased and maintained the frequency of physically active days at both time points but increased their sitting time on weekdays. This highlighted the need for an intervention to prevent prolonged sitting (Broom and Flint, 2018). Kaczmarek et al. (2017) examined whether specific gaming motives, for example, potential health benefits, influenced Pokémon GO gaming time and gaming health outcomes. Findings show that those who spend more time playing were more physically active, suggesting that health motivation is aligned with the previously identified reasons for gaming (Kaczmarek et al., 2017).
Location-based games are not beyond critique, however, as Lindqvist et al. (2018) and Hjorth and Richardson (2017) have outlined. Lindqvist et al. (2018) qualitatively studied the effects of Pokémon GO on families by recording their experiences. While generally positive, like the above studies, that indicated a positive correlation of increased physical activity with game play, Lindqvist et al. (2018) outlined potential safety concerns and dishonesty in game play by some players. In the study, participants reported accidents that occurred while playing the game (e.g., falling into a ditch). Parents also expressed concern about the lack of awareness of the surroundings when playing. Hjorth and Richardson (2017) outlined the possibility of location-based games accentuating feelings of loneliness for those less physically mobile and increasing dangers for those who are stigmatized or marginalized.
The above studies highlight the importance of social and health motivations in increasing gaming time. The studies also show that game mechanics and incentives can enhance people’s participation and experience with their community (Pang et al., 2019). However, in the case of Pokémon GO, the potential to be an effective intervention to increase physical activity in the long term is short-lived (Broom and Flint, 2018). When considering long-term engagement of locative media and full compatibility with the outdoor environment, there should be a balance between the physical world and the virtual world—offering a sense of immersion into the gameplay without losing the sense of presence in the physical space. Many location-based augmented reality games excel at the former but fail at the latter (Haahr, 2017). An example study that uses AR to retain the sense of presence in the real environment is by Tzima et al. (2021). In the study, players detected hidden physical objects and digital information using an AR application to solve riddles about the local cultural heritage. The game was designed to be implemented at the physical environment and could be played only in the selected place (Tzima et al., 2021). This creates a mediated experience that is close to the natural way of perceiving the environment (Haahr, 2017).
Materials and Methods
We set up the study as a test case to examine the usability of a webAR when integrated into the built environment to provide an interactive experience to participants as they actively explored the urban environment. The study was in partnership with an urban planning and design agency. We conducted the study during a family sports day held on September 12, 2020. It was organized as part of an initiative called Lähirähinä, which loosely translates to Good for Hood in English. This is a voluntary initiative started 5 years ago in Oulu to encourage people to be physically active within their own neighborhoods by using the available sporting facilities or playgrounds to organize different sporting events. The sports day was organized as a day for fun and to bring together residents from the different neighborhoods. This was the second time the sports event was organized.
Participant Recruitment
We asked participants to download and print instructions for completing the tasks from the events website3 if they wanted to participate. The event was publicized through social media and word of mouth. This included short video previews of some checkpoints’ location. Participation in the event and its activities was at participants’ own risk. They were also informed that they were responsible for their own medical cover in case of any accidents during the event. As the event was during the Covid-19 pandemic, we followed the health regulations and recommendations from the authorities. During the event, participants were requested to share photos and videos of themselves doing the tasks with the event’s hashtag and their location on social media. The event was open to all and no participant information was collected prior to the event. After the event, we sent a follow-up survey to those who participated in the raffle (see Launching the web augmented reality application at the checkpoints and Measures). Tables 1 shows the descriptive statistics of the participants who responded to the follow-up survey. The survey was anonymous and participants’ information could not be linked to their responses or the checkpoints they visited.
Implementation
The AR application was developed using Zappar’s platform to ensure there is webAR compatibility across a wide range of mobile devices. The application runs on the cloud on Zappar’s servers and a mobile device/client pulls data from there. This makes it easier compared to developing native AR applications. With Zappar’s platform one can create an AR experience using Zappar’s ZapWorks studio. For the application tested in this study, neither a database nor a backend system was used. Rather, just ZapWorks studio4 and C# on top of it were used.5
System log data whenever the application was accessed were also collected and reviewed after the event. The data recorded the location and the number of times the application was activated. As it was web-based, the application could run on any platform and all that was needed was a mobile device and internet connection.
Launching the Web-Augmented Reality Application at the Checkpoints
After downloading the task sheet, participants were free to start exploration from a checkpoint of their choice. We encouraged participants to either walk, cycle, or use public transportation to get to any of the 30 different checkpoints around the city of Oulu and its surroundings. Participants navigated across the city using an analog map, which guided them to the checkpoints (Figure 2).
At each of the checkpoints, they had to find where the poster with the AR tag was placed (Figure 3). The map hinted at the approximate location and clues where to find the AR tags (Figure 3A), instead of revealing the exact location. When they found the poster, they were to open a url6 on their mobile phone, press the “LAUNCH” button, and allow access to the devices’ camera. Then, align the camera with the AR tag and a video with the task was displayed (Figure 3B). Each location had a unique letter code displayed after the video played and participants were to enter this code in the task sheet (Figure 3C). The tasks required the use of facilities or the features available at each location, for example, using outdoor stairs for light workout, walking/jogging to the top of a hill and taking a photo of the view, exercising with outdoor gym equipment, long jumps, playing a short game of beach volleyball or basketball, and so on.
Completing a minimum of six tasks entitled participants free access to two football matches—starting at 2 and 5 pm—on the same afternoon of the sports day. Participants were free to complete as many tasks as they wanted and there were special prizes for the best performers. To enter a draw for the chance to win different prizes, participants needed to complete six or more tasks. The task sheet with their contact details was to be returned by 5 pm at an information point at the stadium where the matches were played. A raffle was held at the halftime break of the match starting at 5 pm and the winners were announced.
Measures
After the event, we posted a follow-up questionnaire asking the participants to record their sociodemographic information, how the webAR compared to AR applications they have used earlier, and the duration of weekly exercise. The questionnaire also included the system usability scale (SUS) (Brooke, 1996, 2013) added with an adjective rating scale (Bangor et al., 2009) to assess usability features of the application. The questionnaire was posted on the event’s pages on social media and also sent to the participants who participated in the raffle during the sports day.
SUS provides a measure of people’s subjective perceptions of the usability of a system and stands out with its simplicity and ease of use (Bangor et al., 2009; Brooke, 1996, 2013). SUS is composed of ten statements, each having a five-point scale that ranges from Strongly Disagree to Strongly Agree. There are five positive statements and five negative statements, which alternate to avoid response bias (Bangor et al., 2009; Brooke, 1996, 2013). The items were selected so that the common response to five of them was strong agreement and to the other five, strong disagreement (Brooke, 2013). As suggested in Bangor, Kortum, and Miller (2009), we added a short set of instructions to remind respondents to mark a response to every statement and not to dwell too long on any one statement. The 10 items in SUS and their Finnish translations are presented in Table 2. For the translation, the first author first translated the items, then the second author, a native speaker, reviewed the translated text. This was to ascertain the quality of the translation and its semantic equivalence. We slightly adjusted the wording of some items to fit the webAR context by replacing the word system in the SUS items with AR application.
To give an absolute judgement of the usability of a product, a 7-point adjective-anchored Likert scale is added as the 11th question. The question is: “Overall, I would rate the user-friendliness of this product as:” and the answer to this question ranges from “1: Worst imaginable” to “7: Best imaginable.” The aim was to explain what a single SUS score means on its own (for example, is a 68.5 good or bad?) (Bangor et al., 2009).
The authors considered SUS a viable instrument for this study because, in addition to encouraging active mobility and urban exploration through interactive play, we also wanted to investigate users’ perceptions of the application.
Data Analysis
The survey responses were downloaded in a structured format and analyzed in Python using the Jupyter Notebook.7 The analysis for the survey data is available online in a github repository8.
According to Tullis and Stetson (2004), sample sizes of at least 12–14 participants are enough to get reasonably reliable results from SUS analysis. In their study, Tullis and Stetson (2004) compared five questionnaires for assessing the usability of a website and analyzed the data to determine the changes in results at different subsample sizes from 6 to 14. Out of the 5, SUS and the computer usability questionnaire (CSUQ) converged most quickly to the correct conclusion regarding the differences in the website’s usability. They yielded the most reliable results across sample sizes. SUS was approximately 75% accurate at a sample size of 8 and this improved to between 90 and 100 in the 12–14 range (Tullis and Stetson, 2004). To calculate the SUS score, first sum the score contributions from each item. Each item's score contribution will range from 0 to 4. For the odd-numbered items, the score contribution is the scale position minus 1. For the even-numbered items, the contribution is five minus the scale position. After determining the sum of the scores, they are multiplied by 2.5 to get the overall value of SUS, usually in the 0 to 100 range (Brooke, 1996).
Results
Respondent Characteristics
Initially, we posted a public link of the survey to the social media pages of the event asking all those who took part to respond. This led to three responses, so we contacted those who had submitted their contact details on the day of the event. 65 participated in the raffle and 10 of them responded to the survey. We summarize their details in Table 1. Follow-up statistics from Webropol, the survey tool used, shows that 110 respondents opened the survey.
System Usability Scale
Analysis of SUS includes aggregating the overall usability scores. SUS yields a single number representing a composite measure of the overall usability of the system being studied. The adjective rating scale was calculated as outlined in Bangor, Kortum, and Miller (2009). This involves doing a correlational analysis to reveal how well the SUS scores match with the adjective rating scale (Figure 4). The results are in line with earlier findings showing a positive correlation between the SUS scores and the scale (r = 0.75). Previous studies have found correlations of 0.822 Bangor, Kortum, and Miller (2009), 0.94 Ghosh et al. (2018), and 0.848 Grier et al. (2013). However, in these earlier studies they were comparing the usability of 2 or more systems.
The average perceived usability of the AR application was 69.04, as it is close to the average score of 68.00; this indicates an acceptable system. This means that it is close to the 50th percentile (Bangor et al., 2009). We present the mean and standard deviations of the SUS scores and adjective rating scale of the AR application in Table 3. Overall, participants’ perceptions of the usability of the application were mostly positive. From the open text responses, one respondent stated that compared to other AR applications, this was easier to use. For some participants scanning the codes during the event took longer than expected or the application did not work at all. For example, three participants left a note on their task sheets stating that the application did not work in one of the six checkpoints they visited, so they could not get the code. The video was also interrupted whenever the phone camera shook or was moved.
Participants’ Online Posts and Completed Tasks
We scanned social media for posts using the event’s hashtags and other identifiable location hashtags related to the event. There were fewer than one hundred posts related to the event on Instagram and some of these were for a different event that used a similar hashtag. From the posts, participants enjoyed the overall experience of the event and appreciated how the application was used to reveal the challenges. An example comment translated from Finnish, “It is great that such events are organized. Technology was used with the help of AR codes, where a video was found that told the task and the identification code.” Many other posts just stated the locations participants visited.
Majority of the participants did six tasks. The highest number of completed tasks was 15 (Figure 5), meaning that there were participants who did the challenges in 15 out of the 30 different locations. Five participants completed 15 tasks, from the provided contact details in the returned tasks sheets, four were members of the same family.
Checkpoint Visits and Number of Application Downloads
Each time the application was opened at a checkpoint, it was counted as a download. System log data showed that three checkpoints had more than 100 unique downloads, 132 being the most at one location. The least visited location had only three downloads (Figure 6). Although it is uncertain whether all those who visited a checkpoint completed the tasks or were just curious to find out how the application worked.
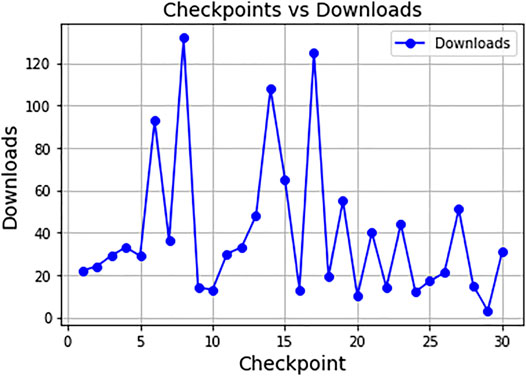
FIGURE 6. Participants filling in the task sheet (left) and returned task sheet (right). Checkpoint visits and number of application downloads.
Further analysis of the task sheets returned by the 65 participants revealed that one of the three checkpoints with the most downloads was among the most visited locations. This checkpoint was outside a movie theater and the task was to do a drop jump. The checkpoint had a total of 108 downloads and was visited by 53 of the 65 participants who returned the task sheets. The other two most visited checkpoints, with 46 and 33 downloads respectively, were both at the beach. The second most visited location was a few meters away from the most visited one. The task at this location was to test the temperature of the water by hand and/or to swim. At two checkpoints, the total number of downloads (33 and 14) matched the number of completed tasks in the returned task sheets. The tasks were to visit an e-sports center located in a gym, to count the number of steps leading up to the top of a castle by the beach, check how many strides were needed to get to the top, and once at the top, take a photo of the view. One location had 21 downloads, but none of these downloads were by the 65 participants who returned the task sheets. Figure 7A presents a summary of the numbers of tasks completed by each of the 65 participants and Figure 7B compares these to the total number of downloads at each checkpoint.
Discussion
In the present study, we examined how to encourage active mobility and exploration through interactive play by exploiting the characteristics of the urban environment. We also examined the usability of a webAR application in creating an interactive experience in the built environment by revealing tasks at different checkpoints. Of those who took part in the event, 13 responded to a questionnaire about the usability of the AR application. The low response rate could be because families took part in the event and only one person from a family with children, for example, responded. Although a small sample, it met the minimum requirement to test the usability of the application (Tullis and Stetson, 2004), but it was not enough for further analyses such as comparing differences between groups and/or learnable and usable scales as presented in Lewis and Sauro (2009) to extract additional information from SUS data. The advantage of SUS is that it is technology-agnostic and is suitable for usability testing as technology evolves (Brooke, 2013). SUS just gives an overview of the usability of the system but does not identify specific usability concerns. We received some of these concerns from the open text responses and in three task sheets where participants mentioned the problems they had launching the application at one of the checkpoints.
Despite the few respondents to the follow-up survey, from the system logs, returned task sheets and some of the posts in social media, we could see that many participants interacted with the application and visited the checkpoints. Based on the system logs, there were hundreds of interactions with the application during the event. As doing the tasks required some form of interaction and collaboration, the results show that the study created opportunities to socially interact and explore different outdoor environments. Although it was not digital, participants could navigate with the map we provided and find the location of the AR tags. Some checkpoints were easy to find, and others were in close proximity to each other. Scanning the tags revealed the tasks required of participants. Because the event was for a single day, these tasks were relatively simple and required minimal effort. Some tasks required participants to only visit a specific location, take a photo, and share it on social media. There were fewer than 100 posts related to the event and some of these were by the same participants. This study contributes to the research on play in urban spaces by examining how games contribute to social interaction, urban exploration, and community awareness (Lindqvist et al., 2018; Pang et al., 2019; Fonseca et al., 2021; Tzima et al., 2021). The online posts and number of tasks completed by the 65 participants who participated in the prize draw demonstrate that people value fun, competitive games, and rewards afforded through locative play in public spaces (Papangelis et al., 2020).
The research focused on active mobility and urban exploration because prior research has shown the health benefits of being physically active, the impact of the built environment on physical activity and the role of locative media in engaging users and promoting health (Heath et al., 2006; Lee and Maheswaran, 2011; Coombes et al., 2013; Helbich et al., 2016; Kaczmarek et al., 2017; Broom and Flint, 2018). Integrating interactive technology into the environment may also be an effective way to analyze tendencies of human behavior over time, compare, for example, how participants from different locations or age groups respond to certain design features, and the effect of changes in the built environment on exercise behaviors and overall wellbeing (Coombes and Jones, 2016; Harris, 2018a; Kärmeniemi et al., 2018). Although it is possible to analyze data from activity-tracking applications in given locations, augmenting technology into the built environment offers the possibility of gathering more precise information regarding people’s interactions with the features in the built environment (Kärmeniemi et al., 2018).
We did not have any data on the home location of the participants, so the study could not track how far participants ventured outside their own neighborhoods. However, analysis of the participants who visited the most locations shows they covered a distance of approximately 15 km (measured from the first location they visited to the last one—according to the numbered locations in the task sheet) (see Figure 5). The possibility of tracking their location as in Pang et al. (2019) would have provided information about their means of travel and how much time they spent at each checkpoint. This study was only for a day and the application was only used at the checkpoints, to have a better idea of how such an app can enhance interactivity and encourage active mobility; a longer duration and an application with more game elements are needed. Related studies are Pang et al. (2019) month-long field study on increasing community awareness during transit and Fonseca et al.’s (2021) study encouraging school-aged children to explore the environment searching for solutions to challenges and engage with other players and/or even strangers. The advantage of our study setup, although not conducive for evaluating the long-term impact, was that anyone could participate in playful exploration without feeling pressured or obligated to reveal any information. A future consideration for locative media is when considering residents with mobility challenges and those marginalized or stigmatized (Hjorth and Richardson, 2017; Lindqvist et al., 2018).
It was a rainy day when we conducted the study, which could have affected the level of participation. Maybe, such mobile urban games are more effective during dry weather as they rely on participants to travel actively outdoors (Coombes and Jones, 2016). When interpreting the results, it is also important to remember the limitations of the mostly educated survey demographic, a majority of whom were 41–50 years. The lack of prior recruitment, which would have given the researchers more control over participants’ actions, also limited the study. A mitigating factor in future studies would be to have a pre-and-post-test experimental design on a larger sample, where we collect participants’ physical activity measures at baseline and at the end of the study. With multimethod approaches combining both subjective (surveys and interviews) and behavioral data (system logs), it is possible to gain a more accurate understanding of the impact of locative media.
There were hundreds of interactions with the application, but we do not know whether all these were by participants or curious passers-by. Comparing the number of downloaded task sheets with application downloads would have revealed the actual number of participants. Another limitation was the activation of the AR tags. In some locations, the tags failed to display the video, and in others, the video was interrupted with any slight movement. This hindered the overall experience for some participants and future iterations should make this process more streamlined. For example, by displaying the videos once it scans the AR tag without needing to align the phone’s camera throughout the duration of the video, or receiving an alert when near a tag and the media displaying automatically or after being activated when within a specified distance. WebAR is perhaps more readily accessible, but it offers a limited selection of features compared to using a mobile application. In a mobile application, there are options for more interactivity.
Future improvements include adding gamified elements such as points, challenges, leaderboard, and virtual rewards (Pang et al., 2019; Fonseca et al., 2021) and considering player’s preferences as there are contexts where users prefer certain features over others, as in Lindqvist et al. (2018) where cooperation, especially among families, was valued over competition. Future work should also focus on addressing the balance between the virtual and the physical world (Haahr, 2017; Korhonen et al., 2017) and linking in-game virtual rewards to actual activity in the physical environment as highlighted in Korhonen, Oduor and Isomursu’s (2017) study about Zombies Run!
This pilot study outlined how to encourage urban exploration and active mobility through interactive games and is an initial step for a more comprehensive evaluation of community-wide location-based games. The study suggests that this may be effective if the challenge of getting participants to engage can be overcome (Coombes and Jones, 2016; Harris, 2018a). As with earlier research, the key challenge is always developing solutions with long-term impact on lifestyle behavior change. Designing locative games for wellbeing requires considerate design choices of how to augment game mechanics into the built environment and of players’ preferences and needs to take advantage of the strong connection between space and play (Dourish, 2006) to create playful designs. This would enable conducting research regarding the generality and maintenance of behavior change produced by these games (Morford et al., 2014). And how to integrate technology into the built environment to provide opportunities to influence behaviours at scale (Stibe and Larson, 2016).
Conclusion
The current study focused on encouraging active mobility and urban exploration through play. In setting up the study, we were interested in evaluating how the webAR application enhanced interactivity by having a decisive role in the activities but not dominating the user experience and interfering with the natural environment. We analyzed the usability of the application and users’ perception was mostly positive. Results show that there were hundreds of interactions with the application and all 30 checkpoints had at least one visitor. Those who participated in the draw, visited 29 of these checkpoints. Among the limitations include the short duration of the study without active recruitment of participants, the sample size, limited features, and web-based nature of the application which limited the user experience for some participants. Overall, the study offered a test case for how to combine the built environment with interactive technology to encourage urban exploration, social interaction, exploration, and active mobility.
Data Availability Statement
The dataset presented in this study can be found in online repository. The names of the repository can be found below: https://github.com/Modago/sus
Ethics Statement
Ethical review and approval was not required for the study on human participants in accordance with the local legislation and institutional requirements. Written informed consent from the participants’ legal guardian/next of kin was not required to participate in this study in accordance with the national legislation and the institutional requirements.
Author Contributions
MO was responsible for the literature review, analysis and documentation of the research. TP was responsible for the implementation of the study.
Conflict of Interest
The authors declare that the research was conducted in the absence of any commercial or financial relationships that could be construed as a potential conflict of interest.
Publisher’s Note
All claims expressed in this article are solely those of the authors and do not necessarily represent those of their affiliated organizations, or those of the publisher, the editors and the reviewers. Any product that may be evaluated in this article, or claim that may be made by its manufacturer, is not guaranteed or endorsed by the publisher.
Acknowledgments
We acknowledge grant support from the Finnish Cultural Foundation. The funder played no role in designing, conducting, interpreting or publishing the study.
Footnotes
1https://awards.ixda.org/entry/2013/21-balancoires/
2https://yle.fi/uutiset/3-11584433
3https://lahirahina.fi/lrp2020/
5This information was provided by a representative of the organization who developed the application we tested.
8https://github.com/Modago/sus
References
Arjoranta, J., Kari, T., and Salo, M. (2020). Exploring Features of the Pervasive Game Pokémon GO that Enable Behavior Change: Qualitative Study. JMIR Serious Games 8 (2), e15967. doi:10.2196/15967
Bangor, A., Kortum, P., and Miller, J. (2009). Determining what Individual SUS Scores Mean: Adding an Adjective Rating Scale. J. Usability Stud. 4, 114–123.
Brooke, J. (1996). “SUS: a `quick and dirty´ usability scale,” in Usability Evaluation in Industry. Editors P. W. Jordan, B. Thomas, B. A. Weerdmeester, and I. L. McClelland, 189–194.
Broom, D. R., and Flint, S. W. (2018). Gotta Catch 'Em All: Impact of Pokémon Go on Physical Activity, Sitting Time, and Perceptions of Physical Activity and Health at Baseline and Three-Month Follow-Up. Games Health J. 7 (6), 401–408. doi:10.1089/g4h.2018.0002
Chatterjee, S., and Price, A. (2009). Healthy Living with Persuasive Technologies: Framework, Issues, and Challenges. J. Am. Med. Inform. Assoc. 16 (2), 171–178. doi:10.1197/jamia.M2859
Chien, L., Koskinen, Elina., Leorke, Dale., Nummenmaa, Timo., and Peltonen, J. (2021). The World Is Your Playground: A Bibliometric and Text Mining Analysis of Location-Based Game Research. in Interactivity and Game Creation: 9th EAI International Conference, ArtsIT 2020, Aalborg, Denmark, December 10–11, 2020 (Springer Nature), 160.
Coombes, E., and Jones, A. (2016). Gamification of active travel to school: A pilot evaluation of the Beat the Street physical activity intervention. Health & Place 39, 62–69. doi:10.1016/j.healthplace.2016.03.001
Coombes, E., van Sluijs, E., and Jones, A. (2013). Is environmental setting associated with the intensity and duration of children's physical activity? Findings from the SPEEDY GPS study. Health & Place 20, 62–65. Health & Place. doi:10.1016/j.healthplace.2012.11.008
Dourish, P. (2006).Re-space-ing place. Proceedings of the 2006 20th Anniversary Conference on Computer Supported Cooperative Work. New York, United States: ACM Digital Library, 299–308. doi:10.1145/1180875.1180921
Fonseca, X., Slingerland, G., Lukosch, S., and Brazier, F. (2021). Designing for meaningful social interaction in digital serious games. Entertainment Comput. 36, 100385. doi:10.1016/j.entcom.2020.100385
Ghosh, D., Foong, P. S., Zhang, S., and Zhao, S. (2018). Assessing the Utility of the System Usability Scale for Evaluating Voice-based User Interfaces. Proc. Sixth Int. Symp. Chin. CHI - ChineseCHI ’18, 11–15. doi:10.1145/3202667.3204844
Grier, R. A., Bangor, A., Kortum, P., and Peres, S. C. (2013). The System Usability Scale. Proc. Hum. Factors Ergon. Soc. Annu. Meet. 57 (1), 187–191. doi:10.1177/1541931213571042
Haahr, M. (2017), Creating Location-Based Augmented-Reality Games for Cultural Heritage. Serious Games. JCSG 2017. Lecture Notes in Computer Science. Editors M. Alcañiz, S. Göbel, M. Maet al. 10622 (p. 313, 318). doi:10.1007/978-3-319-70111-0_29
Hamari, J., and Koivisto, J. (2015). Why do people use gamification services? Int. J. Inf. Management 35 (4), 419–431. doi:10.1016/j.ijinfomgt.2015.04.006
Harris, M. A. (2018b). Beat the Street: A Pilot Evaluation of a Community-wide Gamification-Based Physical Activity Intervention. Games Health J. 7 (3), 208–212. doi:10.1089/g4h.2017.0179
Harris, M. A. (2018a). The relationship between physical inactivity and mental wellbeing: Findings from a gamification-based community-wide physical activity intervention. Health Psychol. Open 5 (1), 205510291775385. doi:10.1177/2055102917753853
Heath, G. W., Brownson, R. C., Kruger, J., Miles, R., Powell, K. E., Ramsey, L. T., et al. (2006). The Effectiveness of Urban Design and Land Use and Transport Policies and Practices to Increase Physical Activity: A Systematic Review. J. Phys. Activity Health 3, S55–S76. doi:10.1123/jpah.3.s1.s55
Helbich, M., Emmichoven, M. J. Z. v., Dijst, M. J., Kwan, M.-P., Pierik, F. H., and Vries, S. I. d. (2016). Natural and built environmental exposures on children's active school travel: A Dutch global positioning system-based cross-sectional study. Health & Place 39, 101–109. doi:10.1016/j.healthplace.2016.03.003
Hjorth, L., and Richardson, I. (2017). Pokémon GO: Mobile media play, place-making, and the digital wayfarer. Mobile Media Commun. 5 (1), 3–14. Mobile Media & Communication. doi:10.1177/2050157916680015
Kaczmarek, L. D., Misiak, M., Behnke, M., Dziekan, M., and Guzik, P. (2017). The Pikachu effect: Social and health gaming motivations lead to greater benefits of Pokémon GO use. Comput. Hum. Behav. 75, 356–363. doi:10.1016/j.chb.2017.05.031
Kärmeniemi, M., Lankila, T., Ikäheimo, T., Koivumaa-Honkanen, H., and Korpelainen, R. (2018). The Built Environment as a Determinant of Physical Activity: A Systematic Review of Longitudinal Studies and Natural Experiments. Ann. Behav. Med. 52, 239–251. doi:10.1093/abm/kax043
Korhonen, O., Oduor, M., and Isomursu, M. (2017). Personalizing Narratives to Support Motivation for Physical Activity. Proceedings of the 30th Bled EConference: Digital Transformation: From Connecting Things to Transforming Our Lives. doi:10.18690/978-961-286-043-1.24
Lee, A. C. K., and Maheswaran, R. (2011). The health benefits of urban green spaces: A review of the evidence. J. Public Health 33 (2), 212–222. doi:10.1093/pubmed/fdq068
Lewis, J. R., and Sauro, J. (2009). “The Factor Structure of the System Usability Scale,” in Human Centered Design. Editor M. Kurosu (Germany: Springer Berlin Heidelberg), Vol. 5619, 94–103. doi:10.1007/978-3-642-02806-9_12
Lindqvist, A.-K., Castelli, D., Hallberg, J., and Rutberg, S. (2018). The Praise and Price of Pokémon GO: A Qualitative Study of Children's and Parents' Experiences. JMIR Serious Games 6 (1), e1. doi:10.2196/games.8979
Lopez, R. P. (2012). The Built environment and public health, Vol. 16. New Jersey, United States: John Wiley & Sons.
Morford, Z. H., Witts, B. N., Killingsworth, K. J., and Alavosius, M. P. (2014). Gamification: The Intersection between Behavior Analysis and Game Design Technologies. Behav. Analyst 37 (1), 25–40. doi:10.1007/s40614-014-0006-1
Pang, C., Pan, R., Neustaedter, C., and Hennessy, K. (2019). City Explorer. Proc. 2019 CHI Conf. Hum. Factors Comput. Syst., 1–15. doi:10.1145/3290605.3300571
Papangelis, K., Saker, M., and Jones, C. (2020). Smart Cities at Play: Technology and Emerging forms of playfulness. Behav. Inf. Technology 39 (6), 607–609. doi:10.1080/0144929X.2020.1749338
Sallis, J. F., Cerin, E., Conway, T. L., Adams, M. A., Frank, L. D., Pratt, M., et al. (2016). Physical activity in relation to urban environments in 14 cities worldwide: A cross-sectional study. The Lancet 387 (10034), 2207–2217. doi:10.1016/S0140-6736(15)01284-2
Stibe, A., and Larson, K. (2016). Persuasive cities for sustainable wellbeing: Quantified communities. Int. Conf. Mobile Web Inf. Syst., 271–282. doi:10.1007/978-3-319-44215-0_22
Tullis, T., and Stetson, J. (2004). A Comparison of Questionnaires for Assessing Website Usability, in Usability Professional Association Conference. (Minneapolis, MN, United States) 1, 1–12.
Tzima, S., Styliaras, G., and Bassounas, A. (2021). Revealing Hidden Local Cultural Heritage through a Serious Escape Game in Outdoor Settings. Information 12 (1), 10. doi:10.3390/info12010010
United Nations (2018). 68% of the world population projected to live in urban areas by 2050. United Nations - Department of Economic and Social Affairs. https://www.un.org/development/desa/en/news/population/2018-revision-of-world-urbanization-prospects.html.
World Health Organization (WHO) (n.d.). Physical Inactivity: A Global Public Health Problem. Retrieved from: https://www.who.int/teams/health-promotion/physical-activity. (November 20, 2021).
Keywords: urban play, physical activity, location-based games, built environment, usability
Citation: Oduor M and Perälä T (2021) Interactive Urban Play to Encourage Active Mobility: Usability Study of a Web-Based Augmented Reality Application. Front. Comput. Sci. 3:706162. doi: 10.3389/fcomp.2021.706162
Received: 06 May 2021; Accepted: 30 June 2021;
Published: 11 August 2021.
Edited by:
Mattia Thibault, Tampere University, FinlandReviewed by:
Joseph Macey, University of Turku, FinlandFrances Brazier, Delft University of Technology, Netherlands
Geertje Slingerland, Delft University of Technology, Netherlands, in collaboration with reviewer FB
Copyright © 2021 Oduor and Perälä. This is an open-access article distributed under the terms of the Creative Commons Attribution License (CC BY). The use, distribution or reproduction in other forums is permitted, provided the original author(s) and the copyright owner(s) are credited and that the original publication in this journal is cited, in accordance with accepted academic practice. No use, distribution or reproduction is permitted which does not comply with these terms.
*Correspondence: Michael Oduor, bWljaGFlbC5vZHVvckBvdWx1LmZp