- 1Clinical Pharmacy, Xiangtan Center Hospital, Xiangtan, Hunan, China
- 2Pulmonary and Critial Care Medicine, Zhongshan Hospital of Traditional Chinese Medicine, Zhongshan, Guangdong, China
- 3Pulmonary and Critial Care Medicine, Xiangtan Center Hospital, Xiangtan, Hunan, China
Objective: Exploring the effect of SJQJD on the pulmonary microbiota of chronic obstructive pulmonary disease (COPD) rats through 16S ribosomal RNA (rRNA) sequencing.
Methods: A COPD rat model was constructed through smoking and lipopolysaccharide (LPS) stimulation, and the efficacy of SJQJD was evaluated by hematoxylin and eosin (H&E) staining and Enzyme-Linked Immunosorbnent Assay (ELISA). The alveolar lavage fluid of rats was subjected to 16S rRNA sequencing. The diversity of lung microbiota composition and community structure was analyzed and differential microbiota were screened. Additionally, machine learning algorithms were used for screening biomarkers of each group of the microbiota.
Results: SJQJD could improve lung structure and inflammatory response in COPD rats. 16s rRNA sequencing analysis showed that SJQJD could significantly improve the abundance and diversity of bacterial communities in COPD rats. Through differential analysis and machine learning methods, potential microbial biomarkers were identified as Mycoplasmataceae, Bacillaceae, and Lachnospiraceae.
Conclusion: SJQJD could improve tissue morphology and local inflammatory response in COPD rats, and its effect may be related to improve pulmonary microbiota.
1 Introduction
COPD is a common respiratory disease in clinical practice, and its risk factors include advancing age, long-term smoke irritation, high incidence, and mortality, with continuous airflow restriction being the main pathological characteristic. Relevant statistics (Fang et al., 2018) show that there are approximately 100 million patients with COPD and increasing incidences of COPD in China. According to the World Health Organization, COPD may become the third leading cause of mortality worldwide by 2030 (Kim et al., 2021). Acute exacerbation of COPD (AECOPD) refers to a clinical event characterized by worsening of respiratory symptoms in patients with COPD, leading to changes in symptoms beyond the daily variation range and drug treatment regimens, which is critical for treating COPD disease. This results in reduced quality of life of patients, accelerated decline of lung function, and increased mortality rate of hospitalized patients (Baqdunes et al., 2021; Celli et al., 2021). Presently, there is no effective treatment for AECOPD; therefore, exploring effective prevention and treatment of COPD is one of the most urgent demands of the medical field worldwide.
The respiratory tract constantly exchanges gases with the environment; hence, it is also a system with bacterial colonization. Studies on respiratory microbiota remain in the initial stages. Reportedly, the pulmonary microbiota is closely related to the host’s autoimmune function and participates in the regulation of the immune microenvironment (Cao et al., 2023; Wu et al., 2023). The lungs were presumed to be sterile in healthy individuals; however, owing to the continuous development of medical science and technology, 16S rRNA sequencing has revealed microbial communities detected in the lungs of healthy individuals (Ramsheh et al., 2021; Yagi et al., 2021). The human microbiome includes all forms of microorganisms and their genomes residing within the body of an individual at a specific time, such as in the gut and other mucosal surfaces including the skin, mouth, airways, and vagina (Anand and Mande, 2018, Shi et al., 2021). Ecological imbalance refers to any compositional changes in the microbiome compared with that of healthy individuals (Shi et al., 2021). The low diversity of microbial communities indicates ecological imbalance (Valdes et al., 2018), whereas high diversity is often associated with health and temporal stability (Leitao Filho et al., 2019; Vaughan et al., 2019; Shi et al., 2021).
SJQJD is a medicinal formulation composed of 30 g of mori cortex, 15 g of chrysanthemi indici flos, 40 g of semen benincasae, 20 g of trichosanthis pericarpium, 20 g of pheretima, 20 g of fritillariae cirrhosae bulbus, 50 g of phragmitis rhizoma, 150 g of plantaginis semen, 20 g of concretio silicea bambusae, and 10 g of glycyrrhizae radix et rhizoma. SJQJD exerts considerable clinical effects on patients with COPD presenting phlegm-heat obstructing lung (Yee et al., 2022); however, the specific mechanism underlying SJQJD-mediated treatment of COPD remains unclear. Herein, we constructed a COPD rat model and investigated the effects of SJQJD on the pulmonary microbiota of COPD rats through 16S rRNA sequencing. Modern pharmacology indicates that the extract of mori cortex has a regulatory effect on oxidative stress (Zhai et al., 2022), chrysanthemi indici flos, trichosanthis pericarpium, pheretima, fritillariae cirrhosae bulbus, phragmitis rhizoma all exhibit anti-inflammatory activity (Park et al., 2016; Liu et al., 2020; Tian et al., 2020; Li et al., 2022; Liu et al., 2024), plantaginis semen has the function of regulating lipid metabolism and immune response (Sun et al., 2019; Ren et al., 2021), glycyrrhizae radix et rhizoma has anti-inflammatory and detoxifying effects (Li et al., 2019; Jiang et al., 2022). These physiological processes are involved in various stages of physiological pathology. However, there is currently limited research by semen benincaae and concretio silicea bambusae.
2 Methods
2.1 SJQJD preparation
SJQJD is an internal preparation of Zhongshan Traditional Chinese Medicine Hospital (specific lot number: Guangdong Medicine Preparation Z20071015). All traditional Chinese medicine decoction pieces are provided by the Chinese Pharmacy of Zhongshan Traditional Chinese Medicine Hospital, and identified as qualified authentic products by Deputy Chief Pharmacist He Jianhong. The abovementioned 10 herbs were soaked in water for 30 minutes, and the decoction treatment was performed twice for 1.5 hours. Both decoctions were combined, filtered, concentrated, and added with 200 g of sugar, 3 g of sodium benzoate, and 0.5 g of hydroxyethyl ester. The mixture was boiled and brought to a constant volume of 1 L. Following this, it was allowed to stand for 1 day, and the supernatant was isolated and packaged to complete the preparation.
2.2 Animal experiments
Experimental grouping: Specific pathogen free grade 10 week old Wistar male rats (250 ± 20), purchased from Spelford Beijing Biotechnology Co., Ltd. In total, 30 rats were randomly divided into the following five groups (n = 6): control, model, model + SJQJD (high-dose [H]: 1.2 g/mL), model + SJQJD (medium-dose [M]: 0.8 g/mL), and model + SJQJD (low-dose [L]: 0.6 g/mL) groups.
Animal model construction: Both cigarette smoke exposure and lipopolysaccharide (LPS) intratracheal instillation were used to establish the COPD model, as follows: (1) LPS intratracheal instillation: 0.2 mL of LPS solution (1 mg/mL) was instilled into the airway on the 1st and 14th day of modeling; and (2) smoking: from day 2 to 28, rats were transferred to a dedicated disinfection box and exposed to smoke daily (except for day 14), 10 cigarettes per time for 30 minutes, twice a day in the morning and afternoon.
Medication intervention: The control group was not subjected to LPS intratracheal instillation and smoking procedures and was administered 2.5 mL of physiological saline by gavage every day; the model group was administered 2.5 mL of physiological saline by gavage every day; the administration groups were orally administered 2.5 mL of SJQJD (H, M, and L) every day. The alveolar lavage fluids and lung tissues of rats were retrieved after administration for subsequent experiments.
2.3 H&E staining experiment
The retrieved lung tissues were fixed with 10% formaldehyde solution. Following this, the tissues were cut into 2-mm thick tissue blocks, which were then dehydrated using gradient ethanol, made transparent using xylene, and embedded in paraffin. Next, the tissue blocks were cut into 5-μm thick slices, stained with H&E, and sealed with neutral gum. The morphology of the lung tissues was observed under a microscope and their photos were captured.
2.4 ELISA testing
The alveolar lavage fluids of rats were centrifuged at 4°C at 1800 r/min for 5 minutes, and the supernatants were collected for detection. Next, ELISA was performed to detect interleukin (IL)-6, IL-8, matrix metallopeptidase (MMP)-2, MMP-3, secretory immunoglobulin (sIg)A, and tumor necrosis factor (TNF)-α in the supernatant of the alveolar lavage fluid according to the instructions of the kit (JiangLai, China).
2.5 16S rRNA sequencing and bioinformatics analyses
The 16s rRNA sequencing was performed by Shenzhen Weikemeng Technology Group Co., Ltd. using the experimental alveolar lavage fluid, including DNA extraction, polymerase chain reaction-mediated amplification, and Illumina high-throughput sequencing. Bioinformatics analyses were performed using the Wekemo Bioincloud (https://www.bioincloud.tech). Operational taxonomic units (OTUs) were clustered with 97% consistency, and the sequences of OTUs were annotated with species to obtain the corresponding species information and species-based abundance distribution. Additionally, α-diversity analysis was performed utilizing the following evaluation indexes: Chao1 index for evaluating microbial abundance, and Shannon and Simpson indexes for evaluating microbial evenness and abundance. Furthermore, β-diversity was analyzed to compare the diversity among different ecosystems, and cluster analysis was performed on the sample distance matrix to construct a hierarchical visualization of differences among samples. Linear discriminant analysis effect size (LEfSe) analysis was performed to test the significance of differences in species composition and community structure of the grouped samples, further analyzing the microbiome composition of the two groups at the phylum and genus levels, and determining the species abundances with significant differences. Finally, the characteristic microbial communities of each group were screened using machine-learning methods.
2.6 Statistic analyses
The GraphPad Prism 9.0 software was used to process data and visualize the data. The comparison between two groups was conducted using t-test method, the comparison between three groups was conducted using one-way ANOVA test method, and the comparison between three groups that did not follow a normal distribution was conducted using Kruskal Wallis test. The P-value of < 0.05 was considered statistically significant.
3 Results
3.1 SJQJD improves the lung tissue morphology in COPD rats
The tissue morphology of the control group was intact with no notable inflammatory cell infiltration (Figure 1); however, that of the COPD model group was disordered, with considerable detectable inflammatory cell infiltration and epithelial goblet cell proliferation, indicating the successful establishment of the model. In the SJQJD-H group of rats, a few inflammatory cells were observed in the lung tissue, along with an enlargement of the alveolar spaces. The SJQJD-M group of rats showed reduced aggregation of inflammatory cells in the lung tissue, with some increase in the alveolar septa and inflammatory cell infiltration. The SJQJD-L group of rats demonstrated moderate interstitial inflammatory changes in the lung tissue, along with the widening of the alveolar septa and inflammatory cell infiltration.
3.2 SJQJD inhibited the release of inflammatory factors in COPD rats
ELISA was performed to detect the content of inflammatory factors in the alveolar lavage fluid. The results showed that compared with the control group, the levels of IL-6 (P < 0.0001), IL-8 (P < 0.0001), MMP-2 (P < 0.0001), MMP-3 (P < 0.0001), sIgA (P < 0.0001), and TNF-α (P < 0.0001) in the model group increased considerably, whereas SJQJD (H, M, and L) suppressed their increase in a concentration-dependent manner (Figure 2).
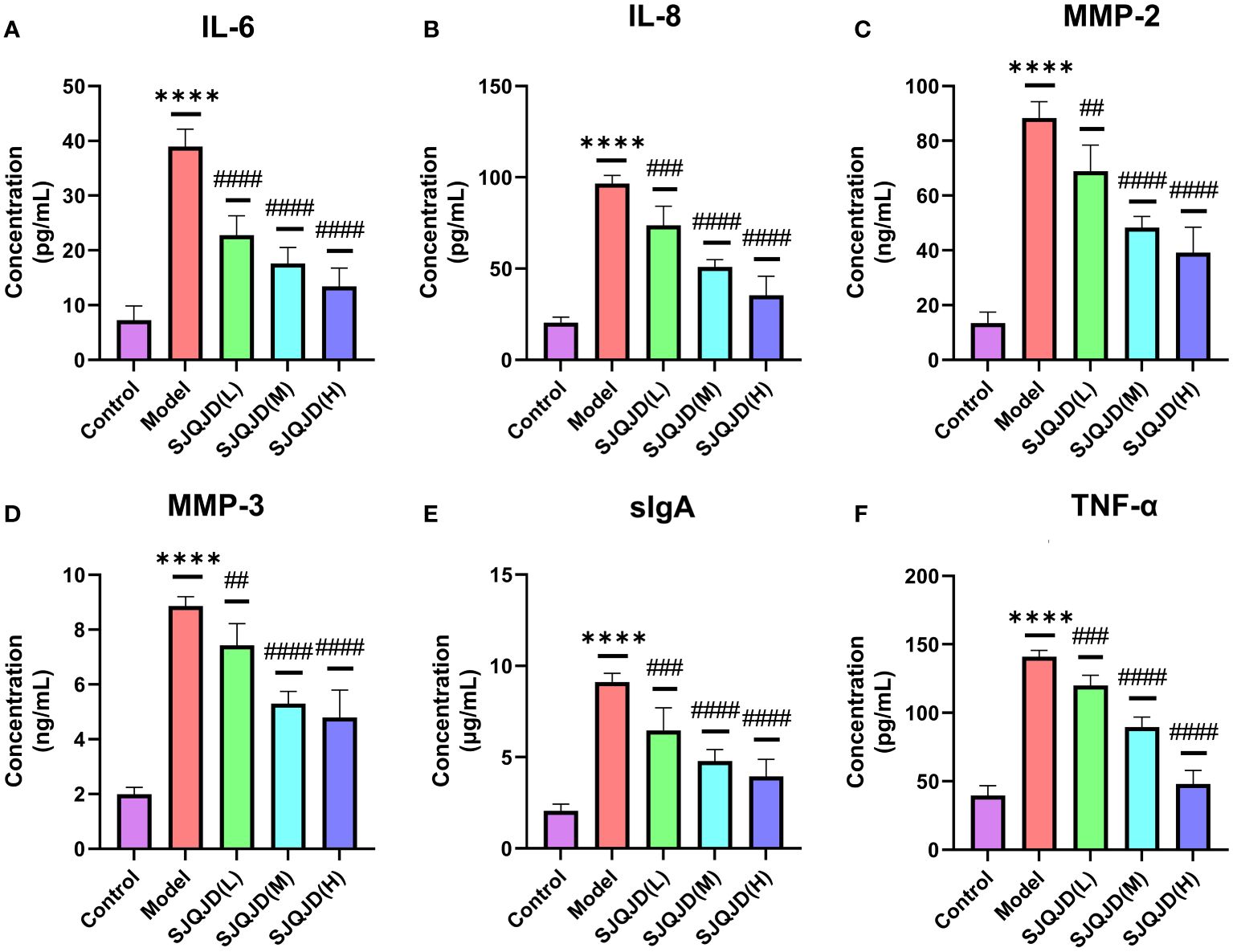
Figure 2 SJQJD improved pulmonary inflammatory response. The level of (A) IL-6, (B) IL-8, (C) MMP-2, (D) MMP-3, (E) sIgA, and (F) TNF- α in rat alveolar lavage fluid. The data was displayed as the mean ± SD (n=6). # P < 0.05, ## P < 0.01, ### P < 0.001, #### P < 0.0001, * P < 0.05, ** P < 0.01, *** P < 0.001, **** P < 0.0001.
3.3 Analysis of the effects of SJQJD on the pulmonary microbiota
The composition structures of the lung microbiota of each group of rats were analyzed at the phylum and genus levels through 16S RNA high-throughput sequencing to explore the effects of SJQJD. At the phylum level, the composition of Tenericutes notably increased in the model group and considerably decreased in the control and SJQJD groups. In contrast, the compositions of Proteobacteria, Actinobacteria, Unspecified_Bacteria, and Firmicutes considerably decreased in the model group and markedly increased in the control and SJQJD groups (Figures 3A, B). At the genus level, the composition of Mycoplasmataceae considerably increased in the model group and markedly decreased in the control and SJQJD groups. In contrast, the composition of Streptomycetaceae, Enterobacteriaceae, Microbacteriaceae, and Bacillaceae markedly decreased in the model group and notably increased in the control and SJQJD groups (Figures 3C, D).
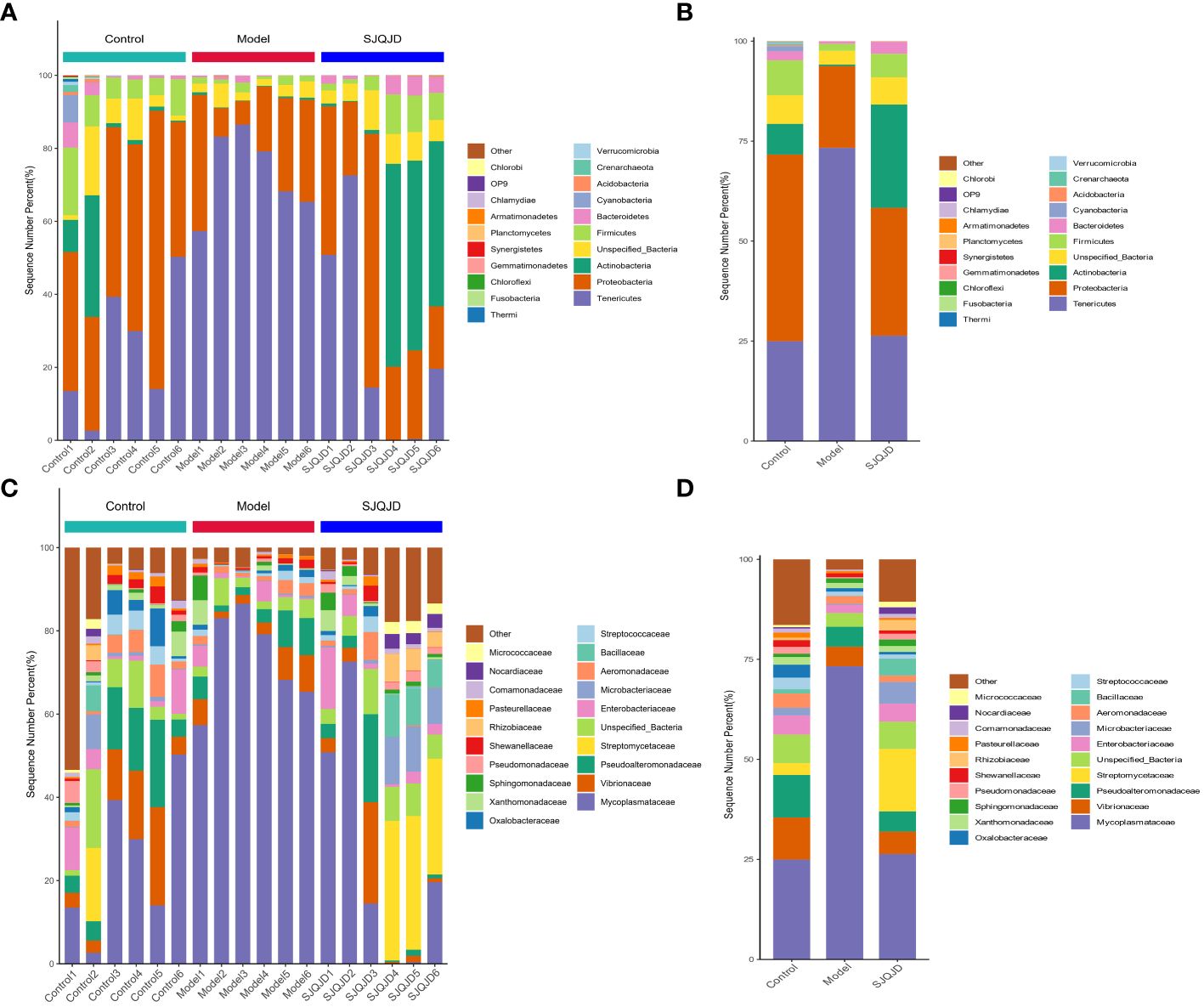
Figure 3 Stacking diagram of the relative abundance of microbial communities. (A) The relative abundance of microbial communities at the phylum level in each sample. (B) The relative abundance of microbial communities at the phylum level in each group. (C) The relative abundance of microbial communities at the genus level in each sample. (D) The relative abundance of microbial communities at the genus level in each group.
3.4 Analyses of α- and β-diversities
Compared with the control rats, the α-diversity indexes, namely Chao1 (P = 0.0397), Shannon (P = 0.0030), and Simpson (P = 0.0021) indexes, of COPD rats were markedly reduced (Figures 4A–C), suggesting a decrease in both microbial abundance and diversity under COPD conditions. SJQJD reversed the decrease in the aforementioned three indexes, demonstrating its therapeutic effects on COPD rats. β-diversity distance measurements, performed to study the structural changes of the pulmonary microbiota among samples, showed notable differences in the microbial communities of control and COPD rats (Figure 4D). However, the SJQJD administration reversed this phenomenon.
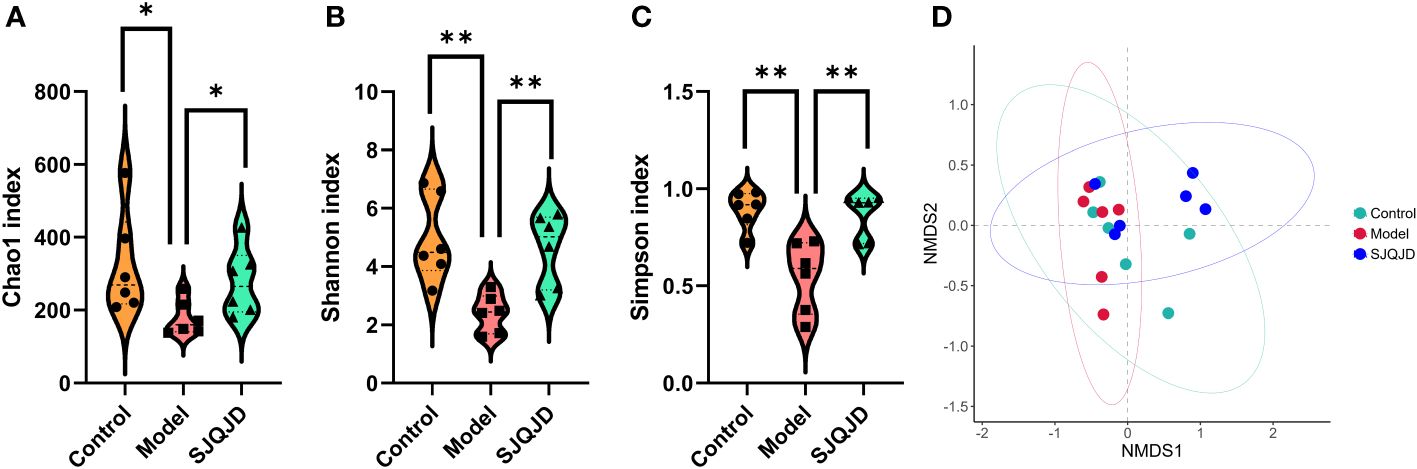
Figure 4 SJQJD improved the diversity of lung microbiota. (A) Chao1 index. (B) Shannon index. (C) Simpson index. (D) NMDS. * P < 0.05, ** P < 0.01.
3.5 Analysis of dominant microbial communities in each group
The bacterial taxa with statistically significant differences among the groups were identified based on the Linear Discriminant Analysis (LDA) value, and the results were visualized by creating a LefSe cladogram (Figure 5A) and a histogram of LDA values (Figure 5B). Actinobacteria, Phyllobacteriaceae, and Alphaproteobacteria were the dominant bacterial taxa in the control group, whereas Mycoplasmatales, Mycoplasmataceae, Tenericutes, and Mollicutes were the dominant taxa in the model group. Proteobacteria, Gammaproteobacteria, Weeksellaceae, and others were characteristic taxa for the SJQJD group.
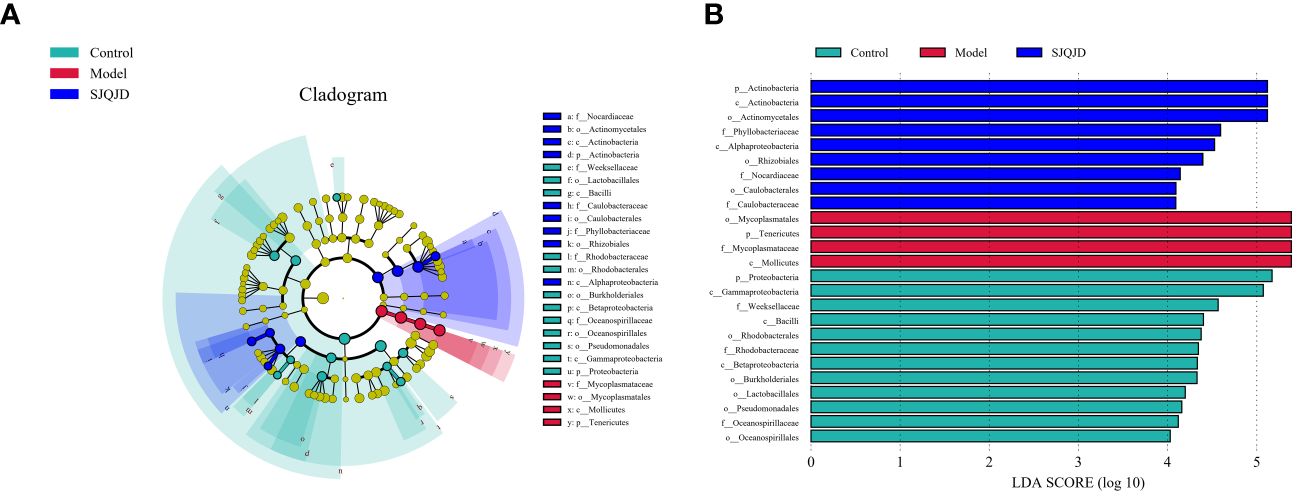
Figure 5 Analysis results of the dominant microbial communities in each group. (A) The branch diagram was obtained through LEfSe analysis. (B) LDA effect size analysis of major biomarker taxonomic groups.
3.6 Analysis of species differences in the pulmonary microbiota
The differences in bacterial communities among groups were analyzed at different levels, such as the family level. The results suggest that compared with the control group, the composition of families Peptostreptococcaceae, Mycoplasmataceae, Rikenellaceae, Listeriaceae, and Ruminococcaceae considerably increased in the model group, whereas that of Chthoniobacteraceae, Streptomycetaceae, and Enterococcaceae markedly reduced (Figures 6A, B). Compared with the model group, the composition of families such as Bacillaceae, Nocardiaceae, and Micrococcaceae notably increased in the SJQJD group, whereas that of Listeriaceae, Clostridiaceae, Campylobacteraceae, and Mycoplasmataceae considerably reduced (Figures 6A, C). Notably, the abundance of the family Mycoplasmataceae (P = 0.0021) was considerably increased in the model group, whereas it remained low in both the control and SJQJD groups, suggesting its potential as a therapeutic marker (Figure 6D). Although there were no statistically significant differences in Bacillaceae abundance among the groups, we observed that this family was almost absent in the model group but exhibited high abundances in both the control and SJQJD groups, suggesting its possible protective role in the disease (Figure 6E).
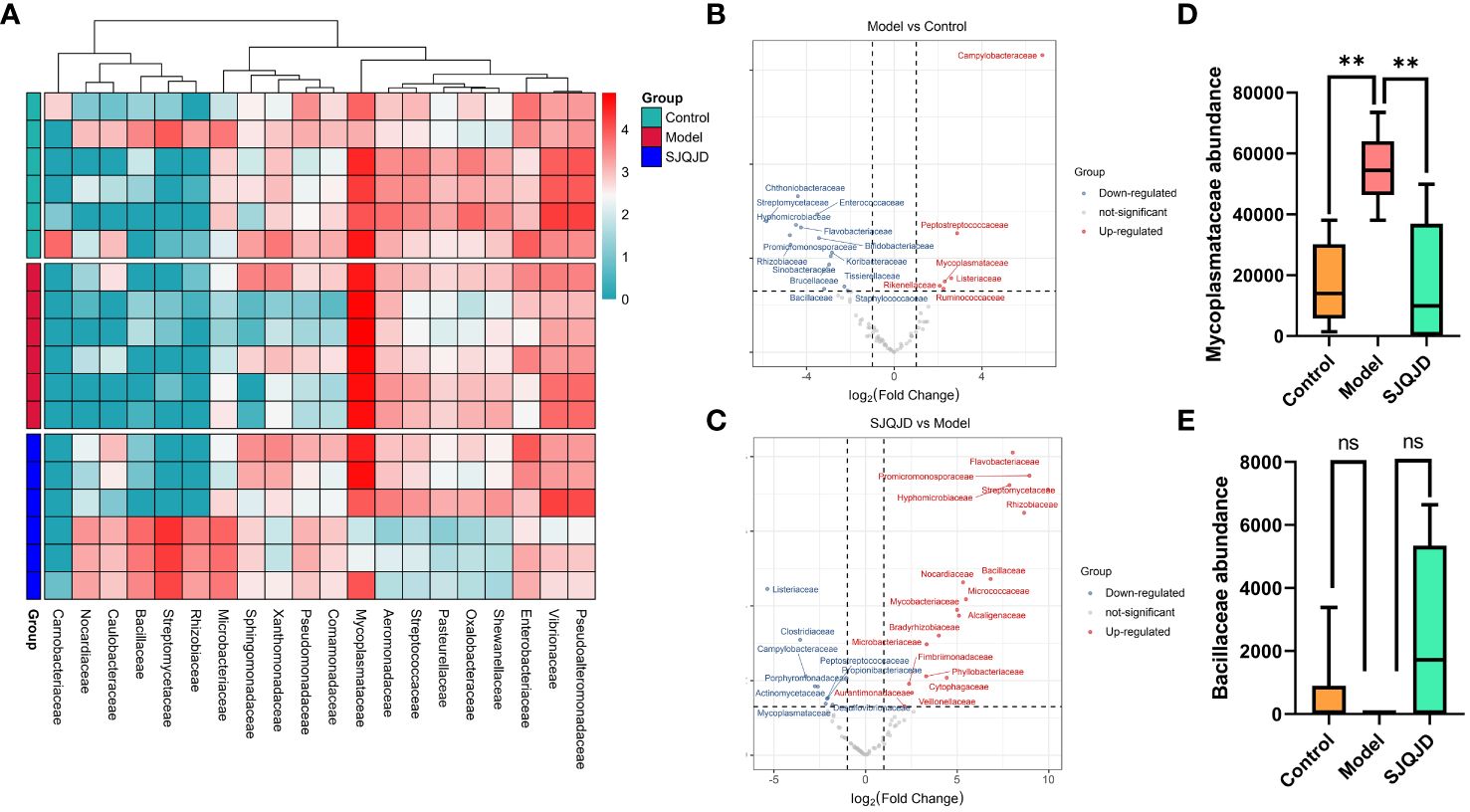
Figure 6 Analysis results of species differences in pulmonary microbiota. (A) Heatmap of differential microbial communities among groups. (B) Volcano diagram of Model vs. Control. (C) Volcano diagram of SJQJD vs. Model. (D) The abundance of Mycoplasmataceae in three groups. (E) The abundance of Bacillaceae in the three groups. ** P < 0.01, ns, no significant.
3.7 Machine-learning method-based screening of biomarkers
The markers in the microbiota of each group were further screened through machine learning. The results show that characteristic microbes of the control group obtained using the random forest algorithm included Lachnospiraceae, Enterococcaceae, and Staphylococcaceae, whereas the model group featured Mycoplasmataceae, Pasteurellaceae, and Aeromonadaceae. The characteristic microbes of the SJQJD group included Nocardiaceae, Lachnospiraceae, and Burkholderiaceae (Figures 7A, B; Table 1). Additionally, the characteristic microbes of the control group obtained using the support vector machine algorithm included Rhodobacteraceae, Lachnospiraceae, and Moraxellaceae; those of the model group included Campylobacteraceae and Moraxellaceae, and those of the SJQJD group included Lachnospiraceae, Burkholderiaceae, and Phyllobacteriaceae (Figures 7C, D; Table 1). By intersecting the results, we found the family Lachnospiraceae to be a common marker between the control and SJQJD groups (Figure 7E).
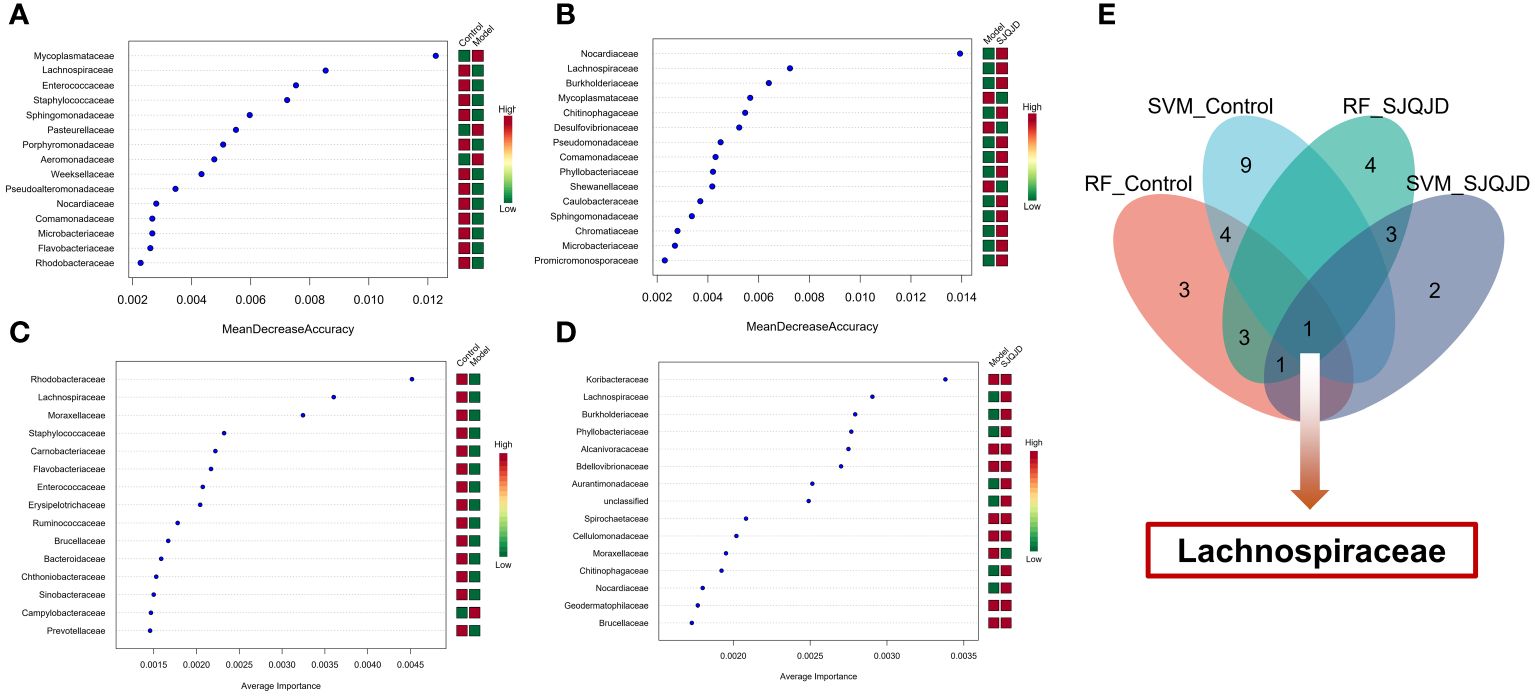
Figure 7 Machine learning screening of microbial biomarkers. (A, B) The results of RF method screening for characteristic microbial communities. (C, D) The results of SVM method screening for characteristic microbial communities. (E) Intersection results of feature microbial communities selected by machine learning.
4 Discussion
COPD is a chronic inflammatory disease characterized by persistent restriction of the small airways, and it often affects multiple systems. Studies predict that because of the increasing number of smokers and population aging, the annual COPD-associated mortality and number of patients may exceed 5.4 million (GBD 2017 Causes of Death Collaborators, 2018) by the 2060s. Acute exacerbation, leading to frequent medical visits, hospitalizations, and changes in medication regimens, is a major cause of mortality in patients with COPD (Wu et al., 2014; Vogelmeier et al., 2017; Hua et al., 2020; Li et al., 2021). Microbial cultures indicate that the lung microbiota is related to COPD pathogenesis (Shi et al., 2021), and the advances in metagenomic technologies have further validated this conclusion (Karakasidis et al., 2023).
SJQJD is a hospital-prepared medication formulation reviewed by the drug regulatory authority. Clinical studies have shown the good therapeutic effects of SJQJD when used in combination with Western medicine to treat phlegm-heat obstructed lung-type community-acquired pneumonia. Reportedly, the combined treatment of SJQJD with Western medicine for bronchiectasis can effectively improve the lung function, forced vital capacity (FVC), forced expiratory volume in the 1st second (FEV1), and FEV1/FVC levels of the patients, with a total effective rate of 97.50%, compared with 85.00% in the control group (P < 0.05) (Dong et al., 2018). Additionally, clinical research on patients with phlegm-heat congested lung-type AECOPD has shown (Huang et al., 2021) that after treatment with SJQJD in combination with Western medicine, the T lymphocyte subgroup cluster of differentiation (CD)4+ and the CD4+/CD8+ ratio increased, compared with those before the treatment, and CD8+ reduced, indicating the significantly better optimization in the combined treatment group than that in the Western medicine control group (P < 0.05). This suggested that SJQJD might improve the immune function of patients, thereby enhancing their resistance. These findings imply that SJQJD may exert its therapeutic effect on COPD by altering the lung microenvironment and consequently modulating the lung microbiota.
Herein, a COPD rat model was constructed through a combined approach of cigarette smoke exposure and intratracheal LPS instillation, which is a widely used model for COPD. The COPD model group showed structural disorder with considerable inflammatory cell infiltration and epithelial goblet cell proliferation. However, the SJQJD administration reversed these phenomena, indicating its interventional effects on COPD. Moreover, SJQJD improved pulmonary inflammation.
The lung microbiome plays an important role in maintaining stability within the lungs. The airways of patients with COPD often harbor Haemophilus influenzae, Streptococcus pneumoniae, and Moraxella catarrhalis, which in severe cases can be colonized by Klebsiella pneumoniae, Pseudomonas aeruginosa, and other Gram-negative bacteria. Various factors affect the composition of the respiratory microbiota, including the anatomy of the airways, gender, age, and the immune function of the host (Whiteside et al., 2021). In healthy individuals, the lung microbiota is transient and can be regulated by normal lung defense mechanisms, such as bronchial epithelial cilia movements, coughing, and the immune function of the host. Under healthy conditions, the regional growth conditions generally do not support the extensive proliferation of bacteria, resulting in relatively fewer bacteria. However, inflammatory responses increase the vascular permeability of the airways, providing abundant nutrients, such as amino acids, vitamins, carbon sources, and iron, for bacterial reproduction. Inflammation damages epithelial cells, exposing the basement membrane matrix and promoting bacterial adhesion. Similar to the gut microbiota, dysbiosis of the lung microbiota promotes the persistent progression of COPD (Bowerman et al., 2020). Reduced microbial diversity has been associated with COPD exacerbation events (Sze et al., 2012; Wang et al., 2019; Enaud et al., 2020; Su et al., 2022). Herein, the results showed that the microbial abundance and diversity in the COPD model group were significantly reduced, and SJQJD could considerably reverse this phenomenon, suggesting its role in improving the lung microbiota.
The family Mycoplasmataceae includes prokaryotic bacteria such as Mycoplasma and genital Ureaplasma that are pathogenic to humans (Wood et al., 2021). Reportedly, mycoplasmas are one of the common pathogens in patients with COPD (14%) (Lieberman et al., 2001, 2002). Mycoplasmas can evade the host immune system, induce apoptosis, generate free radicals, and cause oxidative-reductive imbalance in the cellular glutathione potential through pro-inflammatory cytokines, thus leading to AECOPD (Sessa et al., 2009; Papaetis et al., 2010). The family Bacillaceae includes rod-shaped, endospore-forming, Gram-positive bacteria (Liu et al., 2015). They are widely found in nature, including both pathogenic and beneficial strains (Hathout et al., 2000). However, their specific role in COPD remains unelucidated. Reportedly, the increase of Bacillus in the mouse lungs can aggravate local inflammatory response, resulting in more severe pulmonary emphysema (Richmond et al., 2018), which suggests that Bacillus may be a risk factor for COPD. Moreover, a considerably higher population of Bacillaceae has been reported in the rat lungs treated with particulate matter 2.5, a COPD-inducing factor, compared with that in the control (Laiman et al., 2023). Furthermore, lower levels of Bacillus have been detected in the sputum of patients with COPD (Simpson et al., 2016), indicating that Bacillus may play a protective role in COPD. The results of this study indicated a low abundance of Bacillaceae in the COPD model, whereas it was considerably higher in the control and SJQJD treatment groups, suggesting that Bacillaceae may have a role in combating COPD. Reportedly, most members of the Lachnospiraceae family in the gut are associated with decreased lung function; however, the abundances of some members are markedly reduced in COPD (Bowerman et al., 2020; Chiu et al., 2021). To date, only a few studies are on the distribution and role of Lachnospiraceae in the lungs. Herein, we discovered Lachnospiraceae to be a marker of the microbiota, with a high abundance in the control and SJQJD groups and a low abundance in the model group, suggesting the regulatory role of its members in COPD in the lungs. Lachnospiraceae members can metabolize dietary fiber into short-chain fatty acids (SCFAs), and the SCFA levels are positively correlated with the severity of COPD because of their participation in the maturation process of immune cells, which then exert local and systemic anti-inflammatory effects (Jang et al., 2020; Song et al., 2023). The above evidence suggests that Lachnospiraceae likely regulate the pulmonary microenvironment and local immune function through their metabolic products.
Furthermore, our study only presented the above results at the animal level. The identification of biomarkers still needs further validation in clinical human specimens. In addition, this article also has certain limitations, as it only observed changes in lung microbiota and did not further verify whether these changes will be involved in the occurrence and development of COPD, as well as the specific mechanisms involved in the process. These are the topics that we need to delve deeper into in the future.
5 Conclusion
The findings of this study show that SJQJD can improve COPD in rats. Pulmonary microbiome analysis combined with machine learning identified Mycoplasmataceae, Bacillaceae, and Lachnospiraceae as potential key biomarkers for SJQJD intervention in COPD; however, more in-depth studies are required to elucidate their specific mechanisms and clinical significance.
Data availability statement
The 16S sequencing data involved in this article has been uploaded to the figshare database, which can be obtained through the link: https://figshare.com/articles/dataset/16s_sequencing_data_of_Sangju_Qingjie_Detection_
SJQJD_on_pulmonary_microbiota_in_COPD_rats/25156670.
Ethics statement
The animal study was approved by Experimental Animal Ethics Committee of Nankai Hospital in Tianjin. The study was conducted in accordance with the local legislation and institutional requirements.
Author contributions
ZL: Conceptualization, Data curation, Visualization, Writing – original draft. YH: Conceptualization, Data curation, Project administration, Writing – review & editing. CH: Writing – original draft. XL: Writing – review & editing.
Funding
The author(s) declare financial support was received for the research, authorship, and/or publication of this article. This work was supported by projects SF-YB20231022 and SF-YB20211018 from the Science and Technology Bureau of Xiangtan City, Scientific Research Program of Health Commission of Hunan Province, (No. B2017166).
Conflict of interest
The authors declare that the research was conducted in the absence of any commercial or financial relationships that could be construed as a potential conflict of interest.
Publisher’s note
All claims expressed in this article are solely those of the authors and do not necessarily represent those of their affiliated organizations, or those of the publisher, the editors and the reviewers. Any product that may be evaluated in this article, or claim that may be made by its manufacturer, is not guaranteed or endorsed by the publisher.
Abbreviation
SJQJD, Sangju Qingjie Decoction; COPD, Chronic Obstructive Pulmonary Disease; AECOPD, Acute Exacerbation of Chronic Obstructive Pulmonary Diseases; LPS, Lipopolysaccharide; H&E, Hematoxylin and Eosin; IL-6, Interleukin 6; IL-8, Interleukin 8; MMP-2, Matrix Metallopeptidase 2; MMP-3, Matrix Metallopeptidase 3; sIgA, secretory Immunoglobulin A; TNF-α, Tumor Necrosis Factor α; OTUs, Operational Taxonomic Units; LEfSe, Linear discriminant analysis effect size; ELISA, Enzyme-Linked Immunosorbnent Assay; LDA, Linear Discriminant Analysis; FVC, Forced Vital Capacity; FEV1, Forced Expiratory Volume in the 1st second; SCFAs, Short-Chain Fatty Acids.
References
Anand, S., Mande, S. S. (2018). Diet, microbiota and gut-lung connection. Front. Microbiol. 9. doi: 10.3389/fmicb.2018.02147
Baqdunes, M. W., Leap, J., Young, M., Kaura, A., Cheema, T. (2021). Acute exacerbation of chronic obstructive pulmonary disease. Crit. Care Nurs. Q 44, 74–90. doi: 10.1097/CNQ.0000000000000341
Bowerman, K. L., Rehman, S. F., Vaughan, A., Lachner, N., Budden, K. F., Kim, R. Y., et al. (2020). Disease-associated gut microbiome and metabolome changes in patients with chronic obstructive pulmonary disease. Nat. Commun. 11, 5886. doi: 10.1038/s41467-020-19701-0
Cao, Q., Wu, X., Chen, Y., Wei, Q., You, Y., Qiang, Y., et al. (2023). The impact of concurrent bacterial lung infection on immunotherapy in patients with non-small cell lung cancer: a retrospective cohort study. Front. Cell Infect. Microbiol. 13. doi: 10.3389/fcimb.2023.1257638
Celli, B. R., Fabbri, L. M., Aaron, S. D., Agusti, A., Brook, R., Criner, G. J., et al. (2021). An updated definition and severity classification of chronic obstructive pulmonary disease exacerbations: the Rome proposal. Am. J. Respir. Crit. Care Med. 204, 1251–1258. doi: 10.1164/rccm.202108-1819PP
Chiu, Y. C., Lee, S. W., Liu, C. W., Lin, R. C., Huang, Y. C., Lan, T. Y., et al. (2021). Comprehensive profiling of the gut microbiota in patients with chronic obstructive pulmonary disease of varying severity. PloS One 16, e0249944. doi: 10.1371/journal.pone.0249944
Dong, Z. Q., Huang, Y., Lin, Y. Z. (2018). Clinical observation of Sangju Qingjie soup in treatment of bronchiectasis. China Med. Pharm. 8, 60–62. doi: 10.3969/j.issn.2095-0616.2018.07.018
Enaud, R., Prevel, R., Ciarlo, E., Beaufils, F., Wieërs, G., Guery, B., et al. (2020). The gut-lung axis in health and respiratory diseases: A place for inter-organ and inter-kingdom crosstalks. Front. Cell Infect. Microbiol. 10. doi: 10.3389/fcimb.2020.00009
Fang, L., Gao, P., Bao, H., Tang, X., Wang, B., Feng, Y., et al. (2018). Chronic obstructive pulmonary disease in China: a nationwide prevalence study. Lancet Respir. Med. 6, 421–430. doi: 10.1016/S2213-2600(18)30103-6
GBD 2017 Causes of Death Collaborators. (2018). Global, regional, and national age-sex-specific mortality for 282 causes of death in 195 countries and territories 1980-2017: a systematic analysis for the Global Burden of Disease Study 2017. Lancet 392, 1736–1788. doi: 10.1016/s0140-6736(18)32203-7
Hathout, Y., Ho, Y. P., Ryzhov, V., Demirev, P., Fenselau, C. (2000). Kurstakins: a new class of lipopeptides isolated from Bacillus thuringiensis. J. Nat. Prod 63, 1492–1496. doi: 10.1021/np000169q
Hua, J. L., Hu, W. P., Zuo, Y. H., Zhang, J. (2020). Prevention of acute exacerbation in subjects with moderate-to-very severe COPD by modulating lower respiratory microbiome: protocol of a prospective, multicenter, randomized controlled trial. Int. J. Chron Obstruct Pulmon Dis. 15, 2985–2990. doi: 10.2147/COPD.S274005
Huang, Y., Huang, J., Dong, Z. Q., Chen, S. F., Chen, J. F., Huang, Z. ,. Y. (2021). Effect of sangju qingjie decoction on chronic obstructive pulmonary disease with phlegm-heat accumulation and its influence on immune function. Chin. Arch. Tradit. Chin. Med. 39, 184–187. doi: 10.13193/j.issn.1673-7717.2021.07.046
Jang, Y. O., Lee, S. H., Choi, J. J., Kim, D. H., Choi, J. M., Kang, M. J., et al. (2020). Fecal microbial transplantation and a high fiber diet attenuates emphysema development by suppressing inflammation and apoptosis. Exp. Mol. Med. 52, 1128–1139. doi: 10.1038/s12276-020-0469-y
Jiang, X., Lin, Y., Wu, Y., Yuan, C., Lang, X., Chen, J., et al. (2022). Identification of potential anti-pneumonia pharmacological components of Glycyrrhizae Radix et Rhizoma after the treatment with Gan An He Ji oral liquid. J. Pharm. Anal. 12, 839–851. doi: 10.1016/j.jpha.2022.07.004
Karakasidis, E., Kotsiou, O. S., Gourgoulianis, K. I. (2023). Lung and gut microbiome in COPD. J. Pers. Med. 13, 1–16. doi: 10.3390/jpm13050804
Kim, J., Kim, B., Bak, S. H., Oh, Y. M., Kim, W. J. (2021). A comparative study of chest CT findings regarding the effects of regional dust exposure on patients with COPD living in urban areas and rural areas near cement plants. Respir. Res. 22, 43. doi: 10.1186/s12931-021-01649-4
Laiman, V., Lo, Y. C., Chen, H. C., Yuan, T. H., Hsiao, T. C., Chen, J. K., et al. (2023). Data on lung and intestinal microbiome after air pollution exposure in ageing rats. Data Brief 47, 109004. doi: 10.1016/j.dib.2023.109004
Leitao Filho, F. S., Alotaibi, N. M., Ngan, D., Tam, S., Yang, J., Hollander, Z., et al. (2019). Sputum microbiome is associated with 1-year mortality after chronic obstructive pulmonary disease hospitalizations. Am. J. Respir. Crit. Care Med. 199, 1205–1213. doi: 10.1164/rccm.201806-1135OC
Li, X., Li, Y., Mao, J., Bian, Q., Xuan, Y., Shen, T., et al. (2021). Combination of chinese and western medicine optimizes the intestinal microbiota of exacerbated chronic obstructive pulmonary disease in rats. Evid Based Complement Alternat Med. 2021, 9975407. doi: 10.1155/2021/9975407
Li, S., Yang, Q., Chen, F., Tian, L., Huo, J., Meng, Y., et al. (2022). The antifibrotic effect of pheretima protein is mediated by the TGF-β1/Smad2/3 pathway and attenuates inflammation in bleomycin-induced idiopathic pulmonary fibrosis. J. Ethnopharmacol 286, 114901. doi: 10.1016/j.jep.2021.114901
Li, N., Zhou, T., Wu, F., Wang, R., Zhao, Q., Zhang, J. Q., et al. (2019). Pharmacokinetic mechanisms underlying the detoxification effect of Glycyrrhizae Radix et Rhizoma (Gancao): drug metabolizing enzymes, transporters, and beyond. Expert Opin. Drug Metab. Toxicol. 15, 167–177. doi: 10.1080/17425255.2019.1563595
Lieberman, D., Lieberman, D., Ben-Yaakov, M., Lazarovich, Z., Hoffman, S., Ohana, B., et al. (2001). Infectious etiologies in acute exacerbation of COPD. Diagn. Microbiol. Infect. Dis. 40, 95–102. doi: 10.1016/S0732-8893(01)00255-3
Lieberman, D., Lieberman, D., Ben-Yaakov, M., Shmarkov, O., Gelfer, Y., Varshavsky, R., et al. (2002). Serological evidence of Mycoplasma pneumoniae infection in acute exacerbation of COPD. Diagn. Microbiol. Infect. Dis. 44, 1–6. doi: 10.1016/S0732-8893(02)00421-2
Liu, P., Tan, X. Y., Zhang, H. Q., Su, K. L., Shang, E. X., Xiao, Q. L., et al. (2024). Optimal compatibility proportional screening of Trichosanthis Pericarpium – Trichosanthis Radix and its anti – Inflammatory components effect on experimental zebrafish and coughing mice. J. Ethnopharmacol 319, 117096. doi: 10.1016/j.jep.2023.117096
Liu, J., Wang, X., Li, M., Du, Q., Li, Q., Ma, P. (2015). Jilinibacillus soli gen. nov., sp. nov., a novel member of the family Bacillaceae. Arch. Microbiol. 197, 11–16. doi: 10.1007/s00203-014-1032-9
Liu, S., Yang, T., Ming, T. W., Gaun, T. K. W., Zhou, T., Wang, S., et al. (2020). Isosteroid alkaloids with different chemical structures from Fritillariae cirrhosae bulbus alleviate LPS-induced inflammatory response in RAW 264.7 cells by MAPK signaling pathway. Int. Immunopharmacol 78, 106047. doi: 10.1016/j.intimp.2019.106047
Papaetis, G. S., Anastasakou, E., Tselou, T., Sotiriou, A., Rarra, V. C., Roussou, P., et al. (2010). Serological evidence of Mycoplasma pneumoniae infection in patients with acute exacerbation of COPD: analysis of 100 hospitalizations. Adv. Med. Sci. 55, 235–241. doi: 10.2478/v10039-010-0031-6
Park, S. J., Kim, Y. W., Park, M. K., Byun, S. H., Kim, S. C., Lee, J. R. (2016). Anti-inflammatory steroid from phragmitis rhizoma modulates LPS-mediated signaling through inhibition of NF-κB pathway. Inflammation 39, 727–734. doi: 10.1007/s10753-015-0299-6
Ramsheh, M. Y., Haldar, K., Esteve-Codina, A., Purser, L. F., Richardson, M., Müller-Quernheim, J., et al. (2021). Lung microbiome composition and bronchial epithelial gene expression in patients with COPD versus healthy individuals: a bacterial 16S rRNA gene sequencing and host transcriptomic analysis. Lancet Microbe 2, e300–e310. doi: 10.1016/S2666-5247(21)00035-5
Ren, Z., Yu, R., Meng, Z., Sun, M., Huang, Y., Xu, T., et al. (2021). Spiky titanium dioxide nanoparticles-loaded Plantaginis Semen polysaccharide as an adjuvant to enhance immune responses. Int. J. Biol. Macromol 191, 1096–1104. doi: 10.1016/j.ijbiomac.2021.09.184
Richmond, B. W., Du, R. H., Han, W., Benjamin, J. T., van der Meer, R., Gleaves, L., et al. (2018). Bacterial-derived neutrophilic inflammation drives lung remodeling in a mouse model of chronic obstructive pulmonary disease. Am. J. Respir. Cell Mol. Biol. 58, 736–744. doi: 10.1165/rcmb.2017-0329OC
Sessa, R., Di Pietro, M., Schiavoni, G., Macone, A., Maras, B., Fontana, M., et al. (2009). Chlamydia pneumoniae induces T cell apoptosis through glutathione redox imbalance and secretion of TNF-alpha. Int. J. Immunopathol. Pharmacol. 22, 659–668. doi: 10.1177/039463200902200311
Shi, C. Y., Yu, C. H., Yu, W. Y., Ying, H. Z. (2021). Gut-lung microbiota in chronic pulmonary diseases: evolution, pathogenesis, and therapeutics. Can. J. Infect. Dis. Med. Microbiol. 2021, 9278441. doi: 10.1155/2021/9278441
Simpson, J. L., Baines, K. J., Horvat, J. C., Essilfie, A. T., Brown, A. C., Tooze, M., et al. (2016). COPD is characterized by increased detection of Haemophilus influenzae, Streptococcus pneumoniae and a deficiency of Bacillus species. Respirology 21, 697–704. doi: 10.1111/resp.12734
Song, W., Yue, Y., Zhang, Q. (2023). Imbalance of gut microbiota is involved in the development of chronic obstructive pulmonary disease: A review. BioMed. Pharmacother. 165, 115150. doi: 10.1016/j.biopha.2023.115150
Su, L., Qiao, Y., Luo, J., Huang, R., Li, Z., Zhang, H., et al. (2022). Characteristics of the sputum microbiome in COPD exacerbations and correlations between clinical indices. J. Transl. Med. 20, 76. doi: 10.1186/s12967-022-03278-x
Sun, X., Lan, J., Tong, R., Zhang, H., Sun, S., Xiong, A., et al. (2019). An integrative investigation on the efficacy of Plantaginis semen based on UPLC-QTOF-MS metabolomics approach in hyperlipidemic mice. BioMed. Pharmacother. 115, 108907. doi: 10.1016/j.biopha.2019.108907
Sze, M. A., Dimitriu, P. A., Hayashi, S., Elliott, W. M., McDonough, J. E., Gosselink, J. V., et al. (2012). The lung tissue microbiome in chronic obstructive pulmonary disease. Am. J. Respir. Crit. Care Med. 185, 1073–1080. doi: 10.1164/rccm.201111-2075OC
Tian, D., Yang, Y., Yu, M., Han, Z. Z., Wei, M., Zhang, H. W., et al. (2020). Anti-inflammatory chemical constituents of Flos Chrysanthemi Indici determined by UPLC-MS/MS integrated with network pharmacology. Food Funct. 11, 6340–6351. doi: 10.1039/D0FO01000F
Valdes, A. M., Walter, J., Segal, E., Spector, T. D. (2018). Role of the gut microbiota in nutrition and health. Bmj 361, k2179. doi: 10.1136/bmj.k2179
Vaughan, A., Frazer, Z. A., Hansbro, P. M., Yang, I. A. (2019). COPD and the gut-lung axis: the therapeutic potential of fiber. J. Thorac. Dis. 11, S2173–s2180. doi: 10.21037/jtd.2019.10.40
Vogelmeier, C. F., Criner, G. J., Martinez, F. J., Anzueto, A., Barnes, P. J., Bourbeau, J., et al. (2017). Global strategy for the diagnosis, management, and prevention of chronic obstructive lung disease 2017 report. GOLD executive summary. Am. J. Respir. Crit. Care Med. 195, 557–582. doi: 10.1164/rccm.201701-0218PP
Wang, Z., Maschera, B., Lea, S., Kolsum, U., Michalovich, D., Van Horn, S., et al. (2019). Airway host-microbiome interactions in chronic obstructive pulmonary disease. Respir. Res. 20, 113. doi: 10.1186/s12931-019-1085-z
Whiteside, S. A., McGinniss, J. E., Collman, R. G. (2021). The lung microbiome: progress and promise. J. Clin. Invest. 131, 1–10. doi: 10.1172/JCI150473
Wood, A. M., Tang, M., Truong, T., Feldman, C., Pieper, C., Murtha, A. P. (2021). Vaginal Mycoplasmataceae colonization and association with immune mediators in pregnancy. J. Matern Fetal Neonatal Med. 34, 2295–2302. doi: 10.1080/14767058.2019.1663820
Wu, D., Hou, C., Li, Y., Zhao, Z., Liu, J., Lu, X., et al. (2014). Analysis of the bacterial community in chronic obstructive pulmonary disease sputum samples by denaturing gradient gel electrophoresis and real-time PCR. BMC Pulm Med. 14, 179. doi: 10.1186/1471-2466-14-179
Wu, X., Zhou, Z., Cao, Q., Chen, Y., Gong, J., Zhang, Q., et al. (2023). Reprogramming of Treg cells in the inflammatory microenvironment during immunotherapy: a literature review. Front. Immunol. 14. doi: 10.3389/fimmu.2023.1268188
Yagi, K., Huffnagle, G. B., Lukacs, N. W., Asai, N. (2021). The lung microbiome during health and disease. Int. J. Mol. Sci. 22, 1–13. doi: 10.3390/ijms221910872
Yee, N., Kim, H., Kim, E., Cha, Y. H., Ma, L., Cho, N. E., et al. (2022). Effects of sangju honey on oral squamous carcinoma cells. J. Cancer Prev. 27, 239–246. doi: 10.15430/JCP.2022.27.4.239
Zhai, Y., Li, D., Wang, Z., Shao, L., Yin, N., Li, W. (2022). Cortex mori radicis attenuates streptozotocin-induced diabetic renal injury in mice via regulation of transient receptor potential canonical channel 6. Endocr. Metab. Immune Disord. Drug Targets 22, 862–873. doi: 10.2174/1871530322666220110161458
Keywords: SJQJD, COPD, pulmonary microbiota, biomarker, machine learning
Citation: Liu Z, Huang Y, Hu C and Liu X (2024) The impact of Sangju Qingjie Decoction on the pulmonary microbiota in the prevention and treatment of chronic obstructive pulmonary disease. Front. Cell. Infect. Microbiol. 14:1379831. doi: 10.3389/fcimb.2024.1379831
Received: 31 January 2024; Accepted: 27 March 2024;
Published: 30 April 2024.
Edited by:
Gang Ye, Sichuan Agricultural University, ChinaReviewed by:
Dan Yang, Yibin Vocational and Technical College, ChinaJianan Zhao, Shanghai Guanghua Rheumatology Hospital, China
Copyright © 2024 Liu, Huang, Hu and Liu. This is an open-access article distributed under the terms of the Creative Commons Attribution License (CC BY). The use, distribution or reproduction in other forums is permitted, provided the original author(s) and the copyright owner(s) are credited and that the original publication in this journal is cited, in accordance with accepted academic practice. No use, distribution or reproduction is permitted which does not comply with these terms.
*Correspondence: Xiang Liu, lcyx58214813@163.com
†These authors have contributed equally to this work