- 1Key Laboratory of Respiratory Disease, People’s Hospital of Yangjiang, Yangjiang, China
- 2Guangzhou Institute of Respiratory Health, First Affiliated Hospital of Guangzhou Medical University, Guangzhou, China
Carbapenem-resistant Acinetobacter baumannii (CRAB) is resistant to almost all antibiotics. Eravacycline, a newer treatment option, has the potential to treat CRAB infections, however, the mechanism by which CRAB isolates develop resistance to eravacycline has yet to be clarified. This study sought to investigate the features and mechanisms of eravacycline heteroresistance among CRAB clinical isolates. A total of 287 isolates were collected in China from 2020 to 2022. The minimum inhibitory concentration (MIC) of eravacycline and other clinically available agents against A. baumannii were determined using broth microdilution. The frequency of eravacycline heteroresistance was determined by population analysis profiling (PAP). Mutations and expression levels of resistance genes in heteroresistant isolates were determined by polymerase chain reaction (PCR) and quantitative real-time PCR (qRT-PCR), respectively. Antisense RNA silencing was used to validate the function of eravacycline heteroresistant candidate genes. Twenty-five eravacycline heteroresistant isolates (17.36%) were detected among 144 CRAB isolates with eravacycline MIC values ≤4 mg/L while no eravacycline heteroresistant strains were detected in carbapenem-susceptible A. baumannii (CSAB) isolates. All eravacycline heteroresistant strains contained OXA-23 carbapenemase and the predominant multilocus sequence typing (MLST) was ST208 (72%). Cross-resistance was observed between eravacycline, tigecycline, and levofloxacin in the resistant subpopulations. The addition of efflux pump inhibitors significantly reduced the eravacycline MIC in resistant subpopulations and weakened the formation of eravacycline heteroresistance in CRAB isolates. The expression levels of adeABC and adeRS were significantly higher in resistant subpopulations than in eravacycline heteroresistant parental strains (P < 0.05). An ISAba1 insertion in the adeS gene was identified in 40% (10/25) of the resistant subpopulations. Decreasing the expression of adeABC or adeRS by antisense RNA silencing significantly inhibited eravacycline heteroresistance. In conclusion, this study identified the emergence of eravacycline heteroresistance in CRAB isolates in China, which is associated with high expression of AdeABC and AdeRS.
1 Introduction
Acinetobacter baumannii is a major pathogen responsible for nosocomial infections, primarily causing ventilator-associated pneumonia, bloodstream infections, and skin and soft tissue infections (Durante-Mangoni and Zarrilli, 2011). In recent years, due to the widespread use of carbapenem antibiotics, an increasing number of carbapenem-resistant Acinetobacter baumannii (CRAB) strains have been reported. CRAB strains typically exhibit multidrug resistance, leaving a few options, including ampicillin-sulbactam, polymyxins B, and tigecycline, to treat CRAB infections (Pogue et al., 2013). In recent years, polymyxins B and tigecycline-resistant isolates have been increasingly reported in CRAB isolates. Thus, there is an urgent need for novel antibiotics to treat CRAB infections in clinical practice.
Eravacycline is a novel synthetic fluorocycline antimicrobial agent that was modified from tigecycline. It is active against antibiotic-resistant pathogens, such as multidrug-resistant A. baumannii strains, methicillin-resistant Staphylococcus aureus, vancomycin-resistant Enterococci, and carbapenem-resistant Enterobacteriaceae (Monogue et al., 2016; Li et al., 2022). Notably, eravacycline is four times more effective than tigecycline against multidrug-resistant A. baumannii (Zhanel et al., 2016). Eravacycline was approved for the treatment of complicated intra-abdominal infections in adults based on the success of two phase III clinical trials, IGNITE 1 and IGNITE 4, by the U.S. Food and Drug Administration (FDA) in 2018 (Wang et al., 2021a). Eravacycline is an intravenous and oral medication with the potential to treat CRAB infections. In an analysis of phase III clinical trials, favorable clinical and microbiological responses were observed for eravacycline against CRAB. In patients with complicated intra-abdominal infections caused by multidrug-resistant A. baumannii, of which main strains were CRAB, eravacycline-treated patients achieved a 100% clinical and microbiologic cure (Poulakou et al., 2018). The common adverse events of eravacycline reported in phase I-III studies included infusion-site reactions and gastrointestinal effects such as nausea, vomiting and diarrhea, and it may cause the same adverse events as other tetracyclines (Yusuf et al., 2021).
Heteroresistance is a form of antibiotic resistance in which a bacterial isolate harbors a minority-resistant subpopulation that coexists with a majority-susceptible population. It is difficult to detect using standard antimicrobial susceptibility testing and often results in antibiotic treatment failure (Choby et al., 2021). While the clinical relevance of antibiotic heteroresistance is unclear, there is increasing evidence that heteroresistance causes treatment failure due to the selection of resistant subpopulations after antibiotic treatment (Wen et al., 2020). Thus, the detection and characterization of heteroresistance can provide appropriate therapeutic guidance for antibiotic treatment. Eravacycline heteroresistance is found in only a few bacteria, including Staphylococcus aureus, Enterococcus faecalis, and Klebsiella pneumoniae (Zheng et al., 2018b; Wang et al., 2020; Wen et al., 2020), but has not yet been investigated in CRAB.
This study sought to investigate the phenomenon and mechanism of eravacycline heteroresistance in CRAB clinical isolates from China. The frequency and characteristics of eravacycline heteroresistant strains were analyzed. Molecular sequencing, quantitative real-time PCR (qRT-PCR), and in vitro functional tests were performed to explore the incidence and underlying mechanism of eravacycline heteroresistance in CRAB isolates. These findings will inform the current understanding of eravacycline resistance mechanism.
2 Materials and methods
2.1 Bacterial strains, growth conditions, and antibiotics
From January 2020 to December 2022, 287 non-duplicate A. baumannii isolates, including 147 CRAB and 140 carbapenem-susceptible A. baumannii (CSAB) isolates, were collected from various clinically sampled infections of inpatients at Yangjiang People’s Hospital, a tertiary hospital with 2000 beds in Guangdong Province of China. Bacterial species were identified using a VITEK 2 compact system (BioMérieux, Marcy l’Etoile, France). All strains in this study were cultured on Mueller-Hinton (MH) broth (Oxoid, Basingstoke, UK), or in MH agar plates and Luria-Bertani (LB) broth (Oxoid, Basingstoke, UK) at 37°C. All procedures performed were approved by the Ethical Committee of Yangjiang People’s Hospital and were in accordance with the 1964 Helsinki Declaration and its later amendments. The novel fluorocycline antibiotic, eravacycline (catalog no. HY-16980A), was purchased from MedChem Express (MCE, Shanghai, China). The other antimicrobials were purchased from Meilunbio (Dalian, China). Escherichia coli ATCC 25922 was used for quality control.
2.2 Antimicrobial susceptibility testing
The minimum inhibitory concentrations (MICs) of eravacycline and several common antibiotics were determined using the broth microdilution method recommended by Clinical and Laboratory Standards Institute (CLSI) guidelines (CLSI-M100-S30). As A. baumannii MIC breakpoints for eravacycline and tigecycline have not yet been established by CLSI and FDA, this study categorized the MIC values into three levels described by prior studies (Marchaim et al., 2014; Abdallah et al., 2015): ≤2 mg/L (susceptible), 4 mg/L (intermediate), and ≥8 mg/L (resistant). The susceptibility results for other antibiotics, including meropenem, levofloxacin, gentamicin, cefepime, polymyxin B, tetracycline, doxycycline, and minocycline, were determined based on CLSI breakpoints for each antibiotic. In this study, CRAB strains were defined as those with an imipenem and/or meropenem MIC ≥16 mg/L, while CSAB strains with imipenem and meropenem MICs were both ≤4 mg/L (Fu et al., 2010).
2.3 Population analysis profiles
Population analysis profiling (PAP) was performed as described previously (Abe et al., 2022). The method was used as a reference to investigate eravacycline heteroresistance among 283 clinical A. baumannii isolates with eravacycline MIC values ≤4 mg/L. In brief, 50 μL aliquots of cell suspension (corresponding to a 0.5 McFarland standard for A. baumannii cultures grown on blood agar plates for 24 h at 37°C, 1.0–1.5 × 108 CFU/mL) were spread onto MH agar plates with or without eravacycline (1, 2, 4, 8, 16, and 32 mg/L). The plates were incubated for 24 h at 37°C and the number of colonies was counted. Based on the breakpoint of eravacycline MIC values for A. baumannii, eravacycline heteroresistance was defined as an eravacycline-susceptible isolate (MIC ≤ 4 mg/L) with subpopulations growing in the presence of ≥8 mg/L eravacycline at a detection threshold of 20 CFU/mL. Three colonies were selected from the 8 mg/L eravacycline concentration of the PAP test and categorized as the resistant subpopulations of each eravacycline-heteroresistant isolate (name as: strain-RS). The eravacycline-heteroresistant parental strain was considered as the susceptible subpopulation (name as: strain-HP) given that the majority subpopulations are susceptible to eravacycline (Band and Weiss, 2019). The resistant subpopulations of eravacycline MICs were reassessed after serial passaging on antibiotic-free medium to evaluate the stability of the heteroresistant phenotype.
2.4 Polymerase chain reaction for multilocus sequence typing, carbapenemase, and genetic mutations in the resistance genes
Total DNA from eravacycline heteroresistant parental strains and their resistant subpopulations were extracted using lysis buffer for microorganisms. Polymerase chain reaction (PCR) was performed using PCR Mastermix (Thermo Fisher Scientific, Waltham, MA) according to the manufacturer’s instructions. Multilocus sequence typing (MLST) of the strains was determined by PCR and sequence alignment, as described previously (Huang et al., 2016). The carbapenemase resistance genes, blaOXA, blaKPC, blaIMP, blaVIM, blaSIM, and blaNDM-1 were detected by PCR (Hassan et al., 2021). Genetic mutation of the RND efflux pump regulators, adeR, adeS, adeL, and adeN, and the ribosomal protein S10 encoding gene, rpsJ, were detected by PCR amplification and sequence alignment. The primers used for PCR are listed in Supplementary Tables 1–3.
2.5 Efflux pump inhibitors assays
The role of the efflux pump in the eravacycline heteroresistance of CRAB isolates was detected using the efflux pump inhibitors (EPIs), Phe-Arg-β-naphthylamide (PaβN; MCE, Shanghai, China), and carbonyl cyanide m-chlorophenylhydrazone (CCCP; MCE, Shanghai, China). Eravacycline MIC was determined using the agar dilution method in the presence and absence of PAβN (50 mg/L) or CCCP (16 mg/L) in resistant subpopulations of the heteroresistant isolates, as previously described (Jo and Ko, 2021). Efflux inhibition was defined as a ≥4-fold decrease in the MICs from their original values in the presence of EPI (Tambat et al., 2022). PAP assay with PAβN (50 mg/L) or CCCP (16 mg/L) added to the MH agar plates was used to validate the potential function of the efflux pump in eravacycline heteroresistance.
2.6 Quantitative real-time PCR analysis
The transcriptional levels of the RND-type efflux pump genes (adeB, adeG, and adeJ) and their regulators (adeS, adeL, and adeN) were quantified in eravacycline heteroresistant parental strains and resistant subpopulations using qRT-PCR as described previously (Yoon et al., 2013), with the primers listed in Supplementary Table 4. Since the three genes, adeA, adeB, and adeC, of the efflux pump, adeABC, are located within the same operon, adeB was considered representative of adeABC efflux pump expression in this study (Ju et al., 2021). The other genes shared the same principle. In brief, overnight cultures of the bacterial strains were diluted 1:100 in 10 mL of LB broth and incubated at 37°C with 220 rpm shaking until the growth reached a logarithmic phase. Total RNA was extracted using a Bacteria RNA Extraction Kit (Vazyme, Nanjing, China), and cDNA was synthesized with a HiScriptIII-RT SuperMix for qRT-PCR kit (Vazyme, Nanjing, China) according to the manufacturer’s instructions. The qRT-PCR was performed using a ChamQ Universal SYBR qRT-PCR Master Mix (Vazyme, Nanjing, China) in a LightCycler480II system (Roche, Basel, Switzerland), with the following parameters: 1 cycle at 95°C for 30 s, followed by 45 cycles of 95°C for 10 s and 60°C for 30 s. The internal control gene, rpoB, was used to normalize the expression of each candidate gene. Each sample was run in triplicate. Threshold cycle (Ct) numbers were determined using the qRT-PCR system software, and the relative transcription levels of all strains were calculated using the 2−ΔΔCt method. CRAB-9-HP was used as the reference strain (expression = 1.0). The expression levels of the resistant subpopulation target genes were compared with their parental strains.
2.7 AdeABC and AdeRS associated with heteroresistance were verified by antisense RNA silencing
To confirm the role of AdeABC and AdeRS in the eravacycline heteroresistance of CRAB isolates, the efflux pump genes were silenced by antisense RNA (asRNA) in strains with a high expression of adeABC or adeRS in resistant subpopulations and their parental strains. For silencing adeABC, an adeABC asRNA expression plasmid was constructed by first amplifying the Shine–Dalgarno sequence plus ∼148 nt downstream of the start codon of adeABC and then inserting the segment between the HindIII and BamHI sites downstream of the isopropyl β-D-thiogalactoside (IPTG; MCE, Shanghai, China)-inducible promoter in pHN679 (Zheng et al., 2018a). For generating expression plasmids of adeRS asRNA, the Shine–Dalgarno sequence plus ∼146 nt downstream of the start codon of adeRS was amplified. The asRNA expression plasmids, pASadeABC and pASadeRS, were verified by PCR and DNA sequencing, separately transformed into resistant subpopulations and their parental strains by electroporation, and then verified again using PCR. The pHN679 was also introduced into the isolates as a vector control. Strains used for asRNA silencing are listed in Supplementary Table 5 and primers used for the construction and validation of transformed strains are listed in Supplementary Table 6. The silencing efficacy of the target genes was measured using qRT-PCR as described above. For RNA isolation of the adeABC- and adeRS-silenced strains, the bacteria were pre-treated with 1 mM IPTG to induce expression of the asRNA silencing plasmids. The eravacycline MIC for the adeABC- and adeRS-silenced resistance subpopulations was performed using the broth microdilution method. The frequency of eravacycline heteroresistance in the adeABC- and adeRS-silenced parental strains was determined using the PAP test.
2.8 Statistical analysis
We used Student’s t-test for continuous variables, chi-square analysis for categorical variables. All tests were performed in IBM SPSS Statistics (version 22.0; IBM, Chicago, USA). P-values <0.05 are regarded as statistically significant.
3 Results
3.1 Frequency of eravacycline heteroresistance in clinical CRAB and CSAB isolates
The susceptibilities of 147 CRAB and 140 CSAB strains to eravacycline and other antibiotics are summarized in Supplementary Table 7. Eravacycline, tigecycline and polymyxins B exhibited excellent antimicrobial activity against CRAB. The MIC90 for eravacycline (1 mg/L) was lower than tigecycline (2 mg/L) and polymyxins B (2 mg/L) against these strains. Eravacycline heteroresistance was determined using the PAP test in 144 CRAB and 139 CSAB strains with an eravacycline MIC ≤4 mg/L. While 25 CRAB strains (17.36%) exhibited heteroresistance to eravacycline, no heteroresistant strains were detected in CSAB isolates. Furthermore, the frequency of eravacycline heteroresistance in CRAB isolates was increased with the patent of MIC value (Table 1).
Colonies selected from the 8 mg/L eravacycline PAP test concentration were categorized as the resistant subpopulations of each eravacycline-heteroresistant isolate. The parental strains of eravacycline-heteroresistant isolates were considered susceptible subpopulations in this study since the majority subpopulations were susceptible to eravacycline. The resistant subpopulations had 8- to 128-fold higher eravacycline MICs than the parental strains (Table 2). The eravacycline MICs of resistant subpopulations were 4- to 8-fold lower after 15 passages on antibiotic-free medium and were reversed to susceptible level after 30 passages (Supplementary Table 8). These findings suggest that these subpopulations lacked stable mutations conferring eravacycline resistance and could be reversed to the susceptible phenotype after removal of the antibiotic pressure.
3.2 Characteristics of eravacycline heteroresistance in CRAB isolates
The epidemiological and molecular characteristics of eravacycline heteroresistant strains are listed in Table 2. These strains were primarily shown to infect individuals >60 years of age (n = 22, 88%). The heteroresistant strains came from various specimens, including sputum (n = 13, 52%), wound secretions (n = 9, 36%), urine (n = 2, 8%), and blood (n = 1, 4%) that were primarily obtained from the intensive care unit (ICU) (n = 11, 44%), department of neurosurgery (n = 6, 24%) and department of burn plastic surgery (n = 4, 16%). Four patients infected with the heteroresistant strains had a fatal clinical outcome (n = 4, 16%). MLST revealed six sequence types (ST) in 25 eravacycline heteroresistant strains, with ST208 (n = 18, 72%) being the most prevalent. The predominant carbapenemase genes among these strains were blaOXA-23 (n = 25, 100%) and blaOXA-24 (n = 13, 52%) (Table 2).
3.3 Change in the antimicrobial activity to common antibiotics against resistant subpopulations
The in vitro antimicrobial activity of common antibiotics against resistant subpopulations and parental strains were compared to measure cross-resistance. The tigecycline MICs of all resistant subpopulations and the levofloxacin MICs of 21 resistant subpopulations were each more than 4-fold higher than those of parental strains (Table 3). These findings indicated that resistant subpopulations had developed cross-resistance toward eravacycline, tigecycline, and levofloxacin.
3.4 Effects of the efflux pump inhibitor on eravacycline heteroresistance
To investigate the mechanisms of eravacycline heteroresistance, the effect of the EPIs on the eravacycline MIC of resistant subpopulations was determined first. The eravacycline MICs were significantly decreased by ≥4 fold with the addition of the PaβN or CCCP in 88% (22/25) or 64% (16/25) of the resistant subpopulations, respectively (Table 4). These findings indicated that EPIs could potentiate eravacycline activity in heteroresistant CRAB isolates. We further examined the incidence of eravacycline heteroresistance in CRAB isolates by performing a PAP test with the EPIs. Only four eravacycline heteroresistant strains were detected from 144 CRAB isolates after the addition of PaβN, and only nine heteroresistant strains were identified with the addition of CCCP, significantly fewer than those detected in the absence of EPIs (P < 0.05) (Table 5). These results suggested that EPIs can inhibit the formation of eravacycline heteroresistance in CRAB isolates.
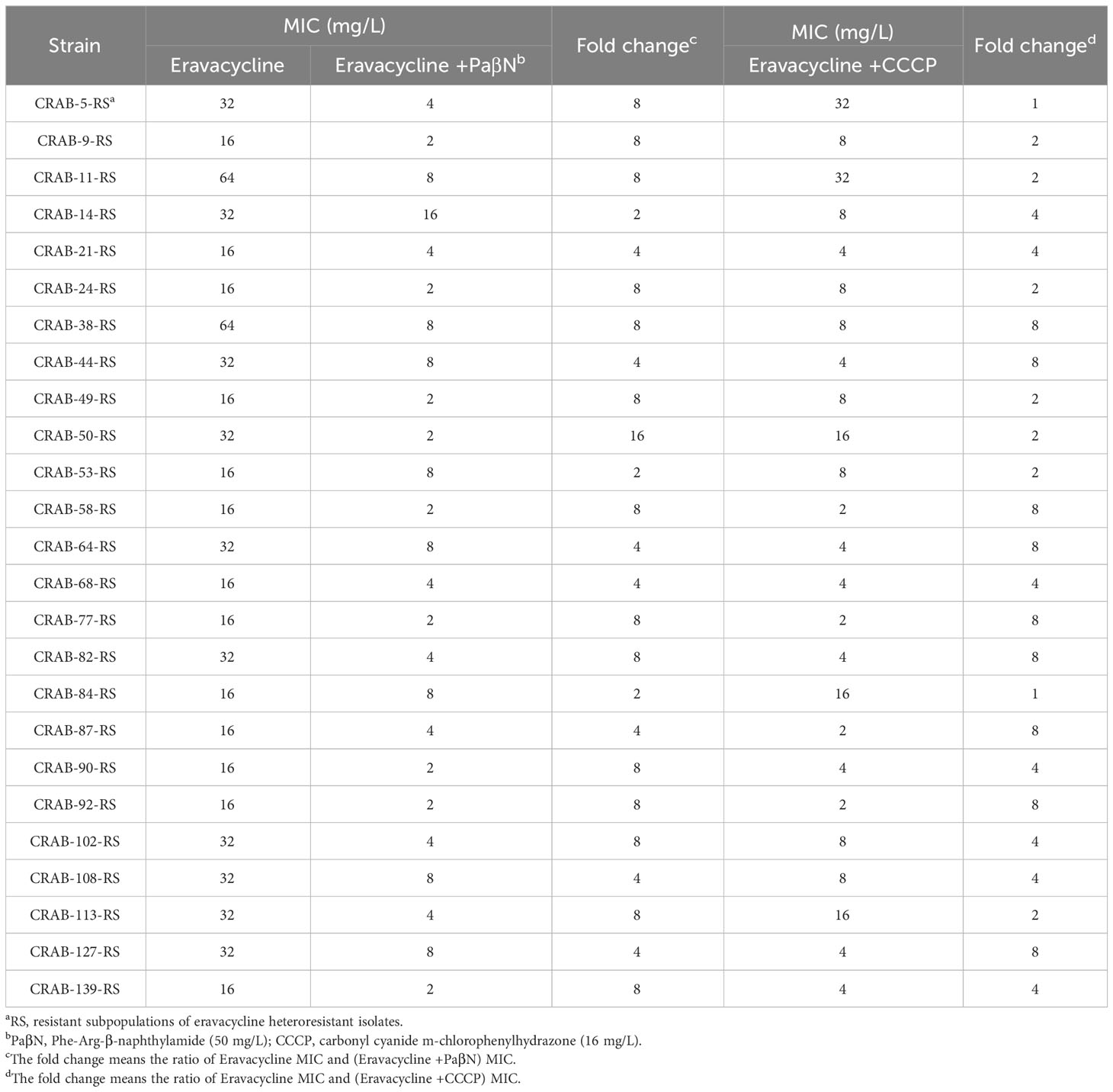
Table 4 Eravacycline MICs against the resistant subpopulations in the absence or presence of efflux pump inhibitors.
3.5 Eravacycline heteroresistance mechanism in CRAB isolates
The above results indicated that efflux pumps were involved in eravacycline heteroresistance. Thus, the relative expression of efflux pumps associated with eravacycline resistance was compared between eravacycline heteroresistant parental strains and their resistant subpopulations. The expression of adeB was significantly higher in 64% (16/25) of resistant subpopulations compared with the parental strains, and adeS expression was significantly increased in 56% (14/25) of resistant subpopulations (Figure 1). No significant differences were found in the expression of other efflux pump genes. These findings suggested that high expression of the efflux pump, AdeABC, and its regulator, AdeRS, may contribute to eravacycline heteroresistance. Genetic alterations of efflux pumps and ribosomes were then investigated in resistant subpopulations. An ISAba1 insertion was detected in adeS in 40% (10/25) of resistant subpopulations (Table 6), indicating that this insertion may be associated with eravacycline heteroresistance.
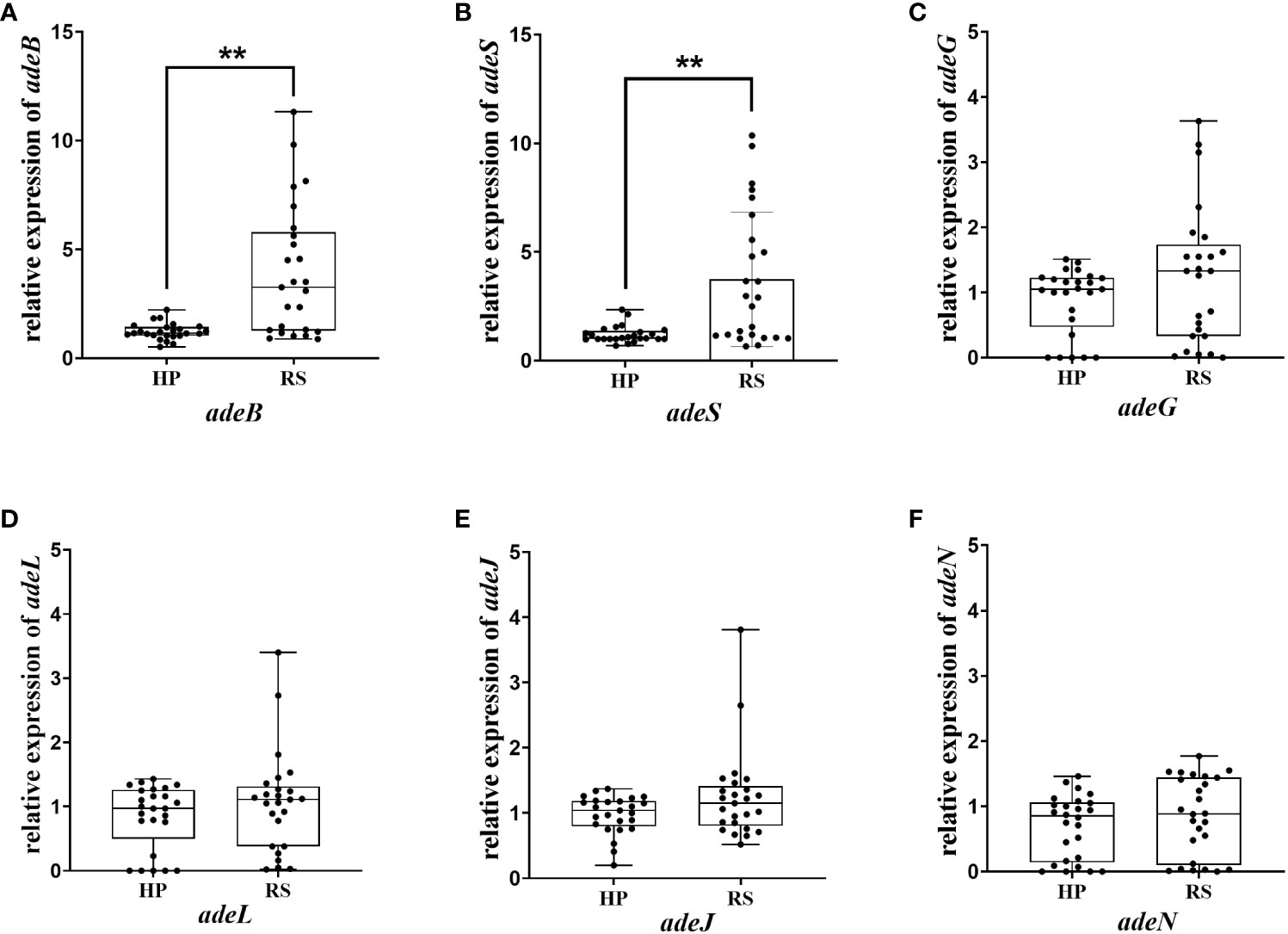
Figure 1 Relative transcription of the efflux pump gene in the heteroresistant parental strain and resistant subpopulations. Relative expression of adeB (A), adeS (B), adeG (C), adeL (D), adeJ (E) and adeN (F) were assessed by qRT-PCR analysis. The housekeeping gene, rpoB, was used as the endogenous reference gene. The heteroresistant parental strain CRAB-9-HP was used as the reference strain (expression = 1.0). All qRT-PCR experiments were carried out in triplicate. ∗∗ P <0.05. HP, eravacycline heteroresistant parental strain; RS, resistant subpopulations of eravacycline heteroresistant strains.
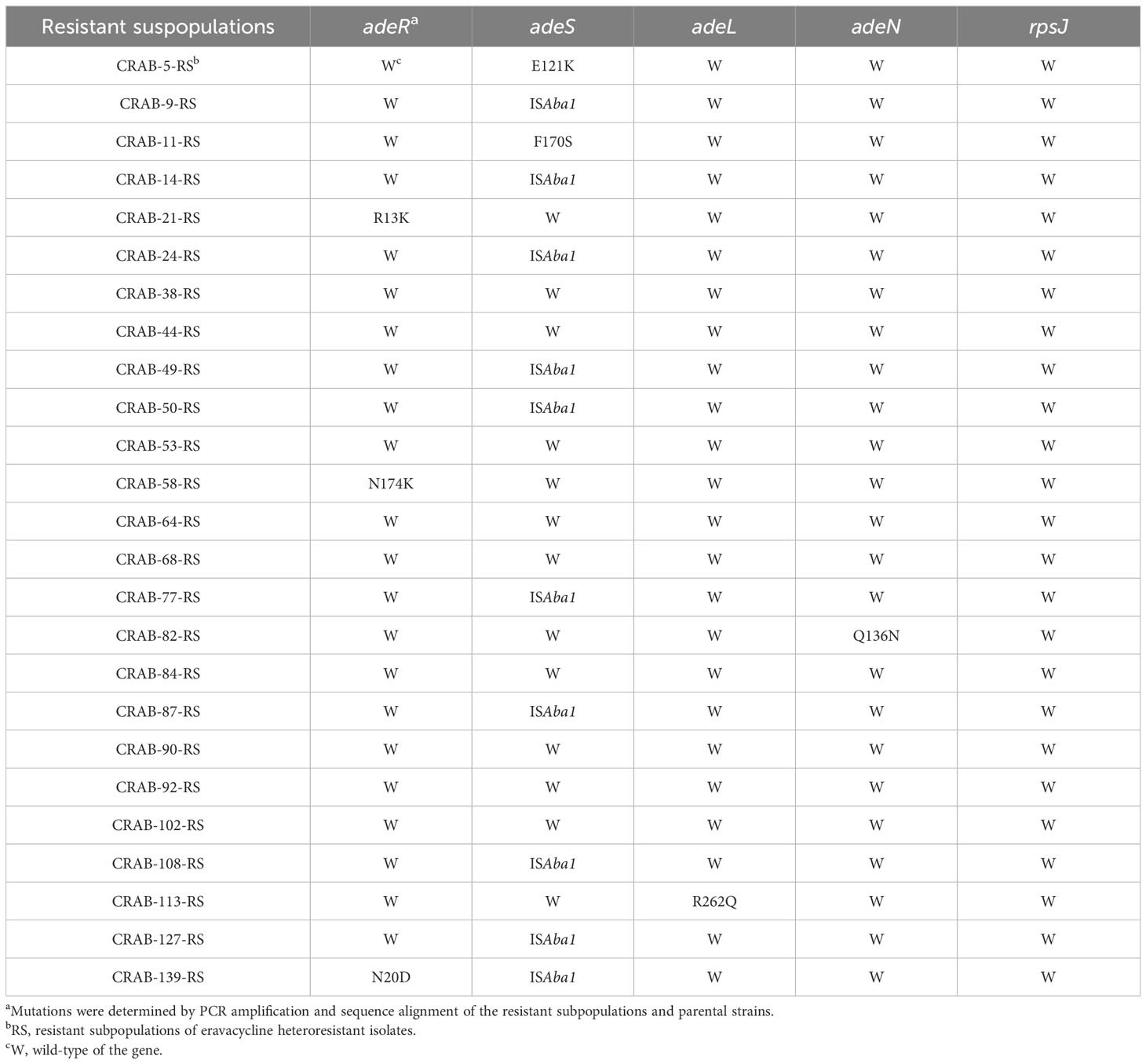
Table 6 Mutations of the efflux pump and ribosome genes in resistant subpopulations of eravacycline heteroresistant strains.
3.6 Validation of the roles of AdeABC and AdeRS in eravacycline heteroresistance
To further confirm the role of the efflux pump, AdeABC, and its regulator, AdeRS, in the eravacycline heteroresistance of CRAB isolates, asRNA was used to individually silence the expression of adeABC and adeRS in eravacycline heteroresistant parental strains and their resistant subpopulations. The asRNA plasmids, pASadeABC and pASadeRS, were constructed and transformed into resistant subpopulations or parental strains (five strains per gene) that showed high expression of the target gene, respectively (Supplementary Table 5). The silencing efficacy of the candidate genes in the transformed strains was confirmed by qRT-PCR. After induction with 1.0 mM IPTG, the expression of adeABC and adeRS was downregulated by >80% more in the constructed strains than in the wildtype strains (Figure 2). The eravacycline MICs of resistant subpopulations decreased by 4- to 8-fold after silencing adeABC or adeRS (Table 7). Furthermore, PAP revealed that silencing adeABC or adeRS led to a loss of the eravacycline heteroresistant phenotype in 80% (4/5) or 60% (3/5) of parental strains, respectively (Supplementary Table 9). These results confirmed that high expression of AdeABC or AdeRS contributed to the formation of eravacycline heteroresistance in CRAB isolates.
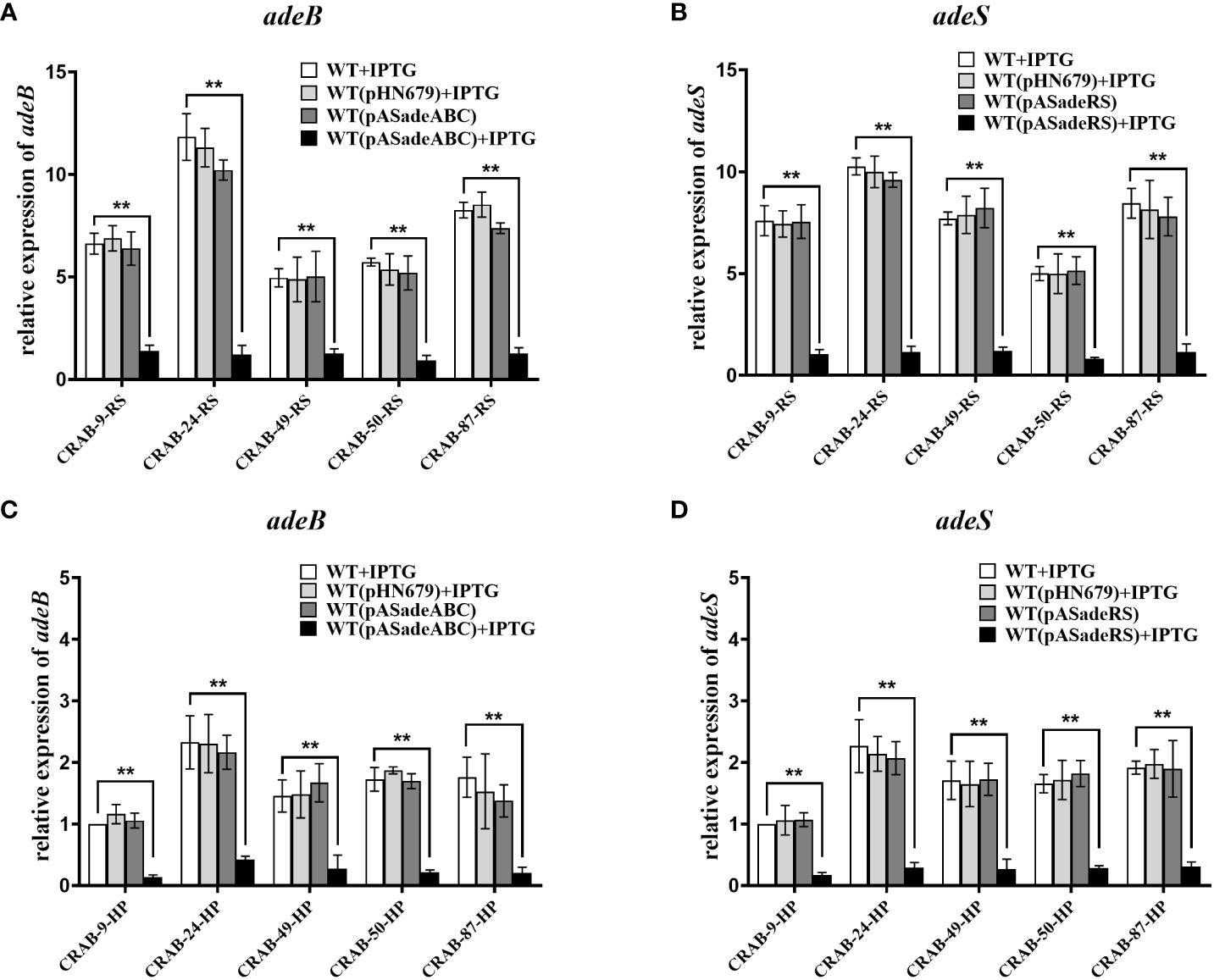
Figure 2 Transcriptional level of adeB/adeS in adeABC- and adeRS-silenced strains. Resistant subpopulations and parental strains transformed with pASadeABC or pASadeRS were grown in LB broth for 5 hours induced with or without 1 mM IPTG. Total RNA was extracted and the expressions of adeB (A, C) or adeS (B, D) were assessed by qRT-PCR. The housekeeping gene, rpoB, was used as an endogenous reference gene. CRAB-9-HP was used as the reference strain (expression = 1.0). All qRT-PCR experiments were carried out in triplicate. ** P <0.05. HP, eravacycline heteroresistant parental strain, RS, resistant subpopulations of eravacycline heteroresistant strains. WT, wild type of the strains. IPTG, isopropyl β-D-thiogalactoside.
4 Discussion
CRAB exhibits resistance to almost all commonly used antibiotics, which poses a significant threat to hospitalized patients. The available treatment options are extremely limited, with no option found to reduce mortality (which exceeds 40% in most studies) or substantially improve clinical outcomes (O'Donnell et al., 2021). Eravacycline confers excellent in vitro antimicrobial activity against CRAB, indicating its potential for use in the treatment of CRAB infections. While eravacycline heteroresistance in CRAB isolates is a serious issue in China, the mechanism of resistance has been poorly understood. This study investigated the characteristics and mechanisms of eravacycline heteroresistance in CRAB isolates to inform appropriate treatment options for CRAB infections.
In this study, eravacycline (MIC90 of 1 mg/L) showed better in vitro antimicrobial activity than tigecycline and polymyxins B, which is consistent with previous reports (Liu et al., 2022). However, 17.36% of the CRAB strains exhibited heteroresistance to eravacycline. Prior research has shown that more than half of the clinical A. baumannii isolates were heteroresistant to tigecycline (Jo and Ko, 2021). This suggests that heteroresistance may be prevalent in A. baumannii isolates treated with tetracycline antibiotics. However, the frequency of eravacycline heteroresistance in this study was significantly lower than tigecycline heteroresistance in Jo’s study, which indicates that tigecycline is more likely to develop heteroresistance in A. baumannii isolates than eravacycline. Risk of eravacycline heteroresistance has been shown in Enterococcus faecalis, Staphylococcus aureus, Streptococcus agalactiae, and Klebsiella pneumoniae (Zhang et al., 2018; Zheng et al., 2018b; Li et al., 2020; Wen et al., 2020). However, the current study is the first to report eravacycline heteroresistance in A. baumannii, with CRAB isolates exhibiting this trait while CSAB isolates did not. These results indicated that CRAB isolates are more likely to develop eravacycline heteroresistance. As the resistant subpopulations of heteroresistance occurs at a low frequency, they are often undetected during routine diagnostic processes and usually misclassified as susceptible strains (Stojowska-Swędrzyńska et al., 2021). Therefore, heteroresistance may lead to antibiotic treatment failure, persistent infection and even lethal outcomes (Juhász et al., 2017). Monitoring the frequency of heteroresistance can provide information about the potential risk of eravacycline resistance among CRAB isolates. In addition, the incidence of eravacycline heteroresistance in CRAB isolates increased with the patent of MIC value, which indicated that the frequency of eravacycline heteroresistance in A. baumannii may be MIC dependent (Kim et al., 2020; Jo and Ko, 2021). Thus, it is important to monitor strains that are close to the eravacycline resistance breakpoint (MIC of 4 mg/L) and make appropriate treatment decisions to avoid the emergence of heteroresistance in clinical practice. The eravacycline MICs of resistant subpopulations were restored to the susceptible level after serial passaging on antibiotic-free medium. This finding indicated that resistant subpopulations are reversible rather than a result of stable mutations, which is consistent with previous reports (Band et al., 2016).
Eravacycline heteroresistant strains mainly originate from the ICU and the burn and neurosurgery departments, where the patients are often immunocompromised. The emergence of heteroresistance and potential antibiotic treatment failure can put these patient populations at a serious health risk. Thus, preventive action is needed to prevent eravacycline heteroresistance in departments with a high incidence of infection. All eravacycline heteroresistant strains contain OXA-23, the most common carbapenemase in A. baumannii (Han et al., 2017), which is disseminated through transposons or plasmids (Zong et al., 2020). In current study, 72% of the eravacycline heteroresistant strains were ST208, one of the predominant clones in China (Jiang et al., 2021). Seruga et al. found that ST195 was the prevalent MLST of A. baumannii heteroresistant to colistin (Seruga Music et al., 2017). It indicates that the prevalent MLST of heteroresistant A. baumannii shows differences to various antibiotics. Previous reports have demonstrated that ST208 A. baumannii strains, which produce the OXA-23 enzyme, can spread rapidly and cause outbreaks in ICUs (Wang et al., 2021b). Our findings illustrated that A. baumannii ST208 producing OXA-23 carbapenemase exhibited heteroresistance to eravacycline, highlighting the need for strict ICU infection control interventions.
EPIs bind to efflux proteins, reducing the ability of the pumps to interact with their substrates and inhibiting multi-drug efflux (Jamshidi et al., 2017). Our data show that the eravacycline MICs were significantly decreased with the addition of the PaβN or CCCP in 88% or 64% of the resistant subpopulations, respectively. It indicates that the effect of PaβN to decrease eravacycline MIC in resistant subpopulations is better than CCCP, which may associate with the differences of their functional mechanism (Li et al., 2015). The current study showed that EPI addition could lower eravacycline MICs in resistant subpopulations and reduce eravacycline heteroresistance in parental strains. These findings indicate that efflux pumps may be involved in inducing eravacycline heteroresistance under antibiotic pressure. The resistance of A. baumannii to eravacycline and tigecycline is mainly associated with the overexpression of the RND-type efflux pumps, AdeABC, AdeFGH, and AdeIJK (Yang et al., 2019; Shi et al., 2020; Jo and Ko, 2021), which are controlled by the transcriptional regulators, AdeRS, AdeL, and AdeN (Gerson et al., 2018; Hua et al., 2021). Thus, qRT-PCR was used to assess the expression of these efflux pumps and their regulatory genes in the eravacycline heteroresistant parental strain and the resistant subpopulations. The expression of adeABC and adeRS were significantly upregulated in the resistant subpopulations, suggesting that eravacycline heteroresistance may be related to the high expression of AdeABC and AdeRS. These findings are similar to those reported in eravacycline resistance studies. Shi et al. found that a deletion mutation in the adeS gene could regulate high expression of the efflux pump, AdeABC, leading to eravacycline resistance in A. baumannii isolates (Shi et al., 2020).
Mutations in upstream regulatory genes of efflux pumps, including adeRS, adeL, and adeN and the ribosomal S10 protein encoding gene rpsJ are thought to contribute to tigecycline and eravacycline resistance in A. baumannii (Shi et al., 2020). To assess this, the current study performed genetic mutation analysis on these genes. The ISAba1 insertion was detected in adeS in 40% (10/25) of resistant subpopulations. This insertion sequence is a common genetic element present in the chromosomes and plasmids of A. baumannii that has been associated with resistance to various antibiotics (Sun et al., 2012). AdeS is a membrane-integrated sensor protein with histidine kinase domains that can transmit environmental stimuli to the response regulator, AdeR, to regulate the expression of the adeABC genes (Coyne et al., 2011). Sun et al. found that the adeS gene with an ISAba1 insertion can enhance adeR expression and upregulate adeABC expression in A. baumannii isolates (Sun et al., 2012). Thus, the current study assessed whether the ISAba1 insertion in adeS impacted the transcription of adeABC. The expression of adeB was significantly higher in all resistant subpopulations with the ISAba1 insertion in adeS than in the parental strains (Supplementary Figure 1), which are consistent with the findings of Sun et al (Sun et al., 2012). Besides, Jo et al. found that the upregulation of adeABC due to ISAba1 insertion in adeS might be the main heteroresistance mechanism of tigecycline in A. baumannii isolates (Jo and Ko, 2021). Our data and Jo’s finding suggest that the high expression of AdeABC efflux pumps may contribute to both eravacycline and tigecycline heteroresistance in CRAB isolates. Appropriate therapeutic options for CRAB infections should be choose as the two drugs share similar heteroresistant mechanism.
To further confirm the roles of the efflux pump, AdeABC, and its regulator, AdeRS, in eravacycline heteroresistant strains, asRNA was used to silence these genes in resistant subpopulations and their parental strains, respectively. Silencing adeABC or adeRS was able to restore sensitivity to eravacycline in resistant subpopulations and reduce eravacycline heteroresistance in parental strains. These findings indicated that high expression of AdeABC and AdeRS may contribute to eravacycline heteroresistance in CRAB isolates. The MIC values of eravacycline, tigecycline, and levofloxacin all increased in the resistant subpopulations, indicating potential cross-resistance between eravacycline and these two drugs. Prior reports have shown that high expression of AdeABC leads to tigecycline resistance (Ruzin et al., 2007), explaining the cross-resistance between eravacycline and tigecycline observed in this study. Mehdi et al. showed that the efflux pumps, EfrAB, EfmA, and EmeA, could induce fluoroquinolone resistance in E. faecium clinical isolates (Mirzaii et al., 2023) and our data suggest that high expression of AdeABC may affect fluoroquinolone resistance. Additional study is required to assess this further.
In conclusion, this study identified the emergence of eravacycline heteroresistance in CRAB isolates in China, with ST208 being the predominant clone. Eravacycline was shown to have cross-resistance with tigecycline and levofloxacin in resistance subpopulations. In addition, the high expression of AdeABC and AdeRS was found to contribute to eravacycline heteroresistance in CRAB isolates.
Data availability statement
The original contributions presented in the study are included in the article/Supplementary Material. Further inquiries can be directed to the corresponding authors.
Ethics statement
All procedures performed were approved by the Ethical Committee of Yangjiang People's Hospital and were in accordance with the 1964 Helsinki Declaration and its later amendments.
Author contributions
Y-TL: Investigation, Methodology, Writing – original draft, Writing – review & editing. X-DC: Methodology, Validation, Writing – review & editing. Y-YG: Writing – review & editing, Methodology, Formal analysis. S-WL: Methodology, Writing – review & editing. M-ZW: Investigation, Writing – review & editing. J-BX: Investigation, Writing – review & editing. X-HW: Methodology, Writing – review & editing. G-HH: Resources, Writing – review & editing. X-XT: Software, Writing – review & editing. CZ: Supervision, Writing – review & editing. Z-WL: Supervision, Writing – review & editing, Conceptualization, Funding acquisition.
Funding
The author(s) declare financial support was received for the research, authorship, and/or publication of this article. This work was supported by the Guangdong Basic and Applied Basic Research Foundation [grant number 2021A1515220090 and 2022A1515010643], Yangjiang Health Technology Project [grant number SF2023021], the Scientific Research Fund of People’s Hospital of Yangjiang [grant number G2020005, G2021003 and 2021001].
Conflict of interest
The authors declare that the research was conducted in the absence of any commercial or financial relationships that could be construed as a potential conflict of interest.
Publisher’s note
All claims expressed in this article are solely those of the authors and do not necessarily represent those of their affiliated organizations, or those of the publisher, the editors and the reviewers. Any product that may be evaluated in this article, or claim that may be made by its manufacturer, is not guaranteed or endorsed by the publisher.
Supplementary material
The Supplementary Material for this article can be found online at: https://www.frontiersin.org/articles/10.3389/fcimb.2024.1356353/full#supplementary-material
References
Abdallah, M., Olafisoye, O., Cortes, C., Urban, C., Landman, D., Quale, J. (2015). Activity of eravacycline against Enterobacteriaceae and Acinetobacter baumannii, including multidrug-resistant isolates, from New York City. Antimicrob. Agents Chemother. 59, 1802–1805. doi: 10.1128/AAC.04809-14
Abe, R., Akeda, Y., Iida, T., Hamada, S. (2022). Population analysis profiling: is it still the gold standard for the determination of heteroresistance in carbapenemase-producing Enterobacteriaceae? Int. J. Antimicrob. Agents 60, 106644. doi: 10.1016/j.ijantimicag.2022.106644
Band, V. I., Crispell, E. K., Napier, B. A., Herrera, C. M., Tharp, G. K., Vavikolanu, K., et al. (2016). Antibiotic failure mediated by a resistant subpopulation in Enterobacter cloacae. Nat. Microbiol. 1, 16053. doi: 10.1038/nmicrobiol.2016.53
Band, V. I., Weiss, D. S. (2019). Heteroresistance: A cause of unexplained antibiotic treatment failure? PloS Pathog. 15, e1007726. doi: 10.1371/journal.ppat.1007726
Choby, J. E., Ozturk, T., Satola, S. W., Jacob, J. T., Weiss, D. S. (2021). Widespread cefiderocol heteroresistance in carbapenem-resistant Gram-negative pathogens. Lancet Infect. Dis. 21, 597–598. doi: 10.1016/S1473-3099(21)00194-8
Coyne, S., Courvalin, P., Périchon, B. (2011). Efflux-mediated antibiotic resistance in Acinetobacter spp. Antimicrob. Agents Chemother. 55, 947–953. doi: 10.1128/AAC.01388-10
Durante-Mangoni, E., Zarrilli, R. (2011). Global spread of drug-resistant Acinetobacter baumannii: molecular epidemiology and management of antimicrobial resistance. Future Microbiol. 6, 407–422. doi: 10.2217/fmb.11.23
Fu, Y., Zhou, J., Zhou, H., Yang, Q., Wei, Z., Yu, Y., et al. (2010). Wide dissemination of OXA-23-producing carbapenem-resistant Acinetobacter baumannii clonal complex 22 in multiple cities of China. J. Antimicrobial Chemotherapy 65, 644–650. doi: 10.1093/jac/dkq027
Gerson, S., Nowak, J., Zander, E., Ertel, J., Wen, Y., Krut, O., et al. (2018). Diversity of mutations in regulatory genes of resistance-nodulation-cell division efflux pumps in association with tigecycline resistance in Acinetobacter baumannii. J. Antimicrob. Chemother. 73, 1501–1508. doi: 10.1093/jac/dky083
Han, L., Lei, J., Xu, J., Han, S. (2017). blaOXA-23-like and blaTEM rather than blaOXA-51-like contributed to a high level of carbapenem resistance in Acinetobacter baumannii strains from a teaching hospital in Xi'an, China. Med. (Baltimore) 96, e8965. doi: 10.1097/MD.0000000000008965
Hassan, R. M., Salem, S. T., Hassan, S. I. M., Hegab, A. S., Elkholy, Y. S. (2021). Molecular characterization of carbapenem-resistant Acinetobacter baumannii clinical isolates from Egyptian patients. PloS One 16, e0251508. doi: 10.1371/journal.pone.0251508
Hua, X., He, J., Wang, J., Zhang, L., Zhang, L., Xu, Q., et al. (2021). Novel tigecycline resistance mechanisms in Acinetobacter baumannii mediated by mutations in adeS, rpoB and rrf. Emerg. Microbes Infect. 10, 1404–1417. doi: 10.1080/22221751.2021.1948804
Huang, G., Yin, S., Gong, Y., Zhao, X., Zou, L., Jiang, B., et al. (2016). Multilocus sequence typing analysis of carbapenem-resistant acinetobacter baumannii in a chinese burns institute. Front. Microbiol. 7. doi: 10.3389/fmicb.2016.01717
Jamshidi, S., Sutton, J. M., Rahman, K. M. (2017). Computational study reveals the molecular mechanism of the interaction between the efflux inhibitor PAβN and the adeB transporter from acinetobacter baumannii. ACS Omega 2, 3002–3016. doi: 10.1021/acsomega.7b00131
Jiang, M., Chen, X., Liu, S., Zhang, Z., Li, N., Dong, C., et al. (2021). Epidemiological analysis of multidrug-resistant acinetobacter baumannii isolates in a tertiary hospital over a 12-year period in China. Front. Public Health 9. doi: 10.3389/fpubh.2021.707435
Jo, J., Ko, K. S. (2021). Tigecycline heteroresistance and resistance mechanism in clinical isolates of acinetobacter baumannii. Microbiol. Spectr. 9, e0101021. doi: 10.1128/Spectrum.01010-21
Ju, Y., Kim, Y.-J., Chang, C. L., Choi, G.-E., Hyun, K.-Y. (2021). Relationship between AdeABC Efflux pump genes and carbapenem in multidrug-resistant Acinetobacter baumannii. Biomed. Sci. Lett. 27, 59–68. doi: 10.15616/BSL.2021.27.2.59
Juhász, E., Iván, M., Pintér, E., Pongrácz, J., Kristóf, K. (2017). Colistin resistance among Blood culture isolates at tertiary Care centre Hungary. J. Glob. Antimicrob. Resist. 11, 167–170. doi: 10.1016/j.jgar.2017.08.002
Kim, H., Kim, E. S., Lee, S. C., Yang, E., Kim, H. S., Sung, H., et al. (2020). Decreased incidence of methicillin-resistant staphylococcus aureus bacteremia in intensive care units: a 10-year clinical, microbiological, and genotypic analysis in a tertiary hospital. Antimicrob. Agents Chemother. 64 (10), 10–1128. doi: 10.1128/AAC.01082-20
Li, Y., Cui, L., Xue, F., Wang, Q., Zheng, B. (2022). Synergism of eravacycline combined with other antimicrobial agents against carbapenem-resistant Enterobacteriaceae and Acinetobacter baumannii. J. Glob. Antimicrob. Resist. 30, 56–59. doi: 10.1016/j.jgar.2022.05.020
Li, X. Z., Plésiat, P., Nikaido, H. (2015). The challenge of efflux-mediated antibiotic resistance in Gram-negative bacteria. Clin. Microbiol. Rev. 28, 337–418. doi: 10.1128/CMR.00117-14
Li, P., Wei, Y., Li, G., Cheng, H., Xu, Z., Yu, Z., et al. (2020). Comparison of antimicrobial efficacy of eravacycline and tigecycline against clinical isolates of Streptococcus agalactiae in China: In vitro activity, heteroresistance, and cross-resistance. Microb. Pathog. 149, 104502. doi: 10.1016/j.micpath.2020.104502
Liu, P. Y., Ko, W. C., Lee, W. S., Lu, P. L., Chen, Y. H., Cheng, S. H., et al. (2022). In vitro activity of cefiderocol, cefepime/enmetazobactam, cefepime/zidebactam, eravacycline, omadacycline, and other comparative agents against carbapenem-non-susceptible Pseudomonas aeruginosa and Acinetobacter baumannii isolates associated from bloodstream infection in Taiwan between 2018-2020. J. Microbiol. Immunol. Infect. 55, 888–895. doi: 10.1016/j.jmii.2021.08.012
Marchaim, D., Pogue, J. M., Tzuman, O., Hayakawa, K., Lephart, P. R., Salimnia, H., et al. (2014). Major variation in MICs of tigecycline in Gram-negative bacilli as a function of testing method. J. Clin. Microbiol. 52, 1617–1621. doi: 10.1128/JCM.00001-14
Mirzaii, M., Alebouyeh, M., Sohrabi, M. B., Eslami, P., Fazli, M., Ebrahimi, M., et al. (2023). Antibiotic resistance assessment and multi-drug efflux pumps of Enterococcus faecium isolated from clinical specimens. J. Infect. Dev. Ctries 17, 649–655. doi: 10.3855/jidc.17304
Monogue, M. L., Thabit, A. K., Hamada, Y., Nicolau, D. P. (2016). Antibacterial efficacy of eravacycline in vivo against gram-positive and gram-negative organisms. Antimicrob. Agents Chemother. 60, 5001–5005. doi: 10.1128/AAC.00366-16
O'Donnell, J. N., Putra, V., Lodise, T. P. (2021). Treatment of patients with serious infections due to carbapenem-resistant Acinetobacter baumannii: How viable are the current options? Pharmacotherapy 41, 762–780. doi: 10.1002/phar.2607
Pogue, J. M., Mann, T., Barber, K. E., Kaye, K. S. (2013). Carbapenem-resistant Acinetobacter baumannii: epidemiology, surveillance and management. Expert Rev. Anti Infect. Ther. 11, 383–393. doi: 10.1586/eri.13.14
Poulakou, G., Lagou, S., Karageorgopoulos, D. E., Dimopoulos, G. (2018). New treatments of multidrug-resistant Gram-negative ventilator-associated pneumonia. Ann. Transl. Med. 6, 423. doi: 10.21037/atm.2018.10.29
Ruzin, A., Keeney, D., Bradford, P. A. (2007). AdeABC multidrug efflux pump is associated with decreased susceptibility to tigecycline in Acinetobacter calcoaceticus-Acinetobacter baumannii complex. J. Antimicrob. Chemother. 59, 1001–1004. doi: 10.1093/jac/dkm058
Seruga Music, M., Hrenovic, J., Goic-Barisic, I., Hunjak, B., Skoric, D., Ivankovic, T. (2017). Emission of extensively-drug-resistant Acinetobacter baumannii from hospital settings to the natural environment. J. Hosp Infect. 96, 323–327. doi: 10.1016/j.jhin.2017.04.005
Shi, Y., Hua, X., Xu, Q., Yang, Y., Zhang, L., He, J., et al. (2020). Mechanism of eravacycline resistance in Acinetobacter baumannii mediated by a deletion mutation in the sensor kinase adeS, leading to elevated expression of the efflux pump AdeABC. Infect. Genet. Evol. 80, 104185. doi: 10.1016/j.meegid.2020.104185
Stojowska-Swędrzyńska, K., Łupkowska, A., Kuczyńska-Wiśnik, D., Laskowska, E. (2021). Antibiotic heteroresistance in klebsiella pneumoniae. Int. J. Mol. Sci. 23 (1), 449. doi: 10.3390/ijms23010449
Sun, J. R., Perng, C. L., Chan, M. C., Morita, Y., Lin, J. C., Su, C. M., et al. (2012). A truncated AdeS kinase protein generated by ISAba1 insertion correlates with tigecycline resistance in Acinetobacter baumannii. PloS One 7, e49534. doi: 10.1371/journal.pone.0049534
Tambat, R., Mahey, N., Chandal, N., Verma, D. K., Jangra, M., Thakur, K. G., et al. (2022). A microbe-derived efflux pump inhibitor of the resistance-nodulation-cell division protein restores antibiotic susceptibility in Escherichia coli and Pseudomonas aeruginosa. ACS Infect. Dis. 8, 255–270. doi: 10.1021/acsinfecdis.1c00281
Wang, X., Du, Z., Huang, W., Zhang, X., Zhou, Y. (2021b). Outbreak of multidrug-resistant acinetobacter baumannii ST208 producing OXA-23-like carbapenemase in a children's hospital in shanghai, China. Microb. Drug Resist. 27, 816–822. doi: 10.1089/mdr.2019.0232
Wang, Z., Lin, Z., Bai, B., Xu, G., Li, P., Yu, Z., et al. (2020). Eravacycline susceptibility was impacted by genetic mutation of 30S ribosome subunits, and branched-chain amino acid transport system II carrier protein, Na/Pi cotransporter family protein in Staphylococcus aureus. BMC Microbiol. 20, 189. doi: 10.1186/s12866-020-01869-6
Wang, H., Nguyen, N., Cruz, C. (2021a). Eravacycline for the treatment of complicated intra-abdominal infections. Adv. Digestive Med. 8, 203–210. doi: 10.1002/aid2.13216
Wen, Z., Shang, Y., Xu, G., Pu, Z., Lin, Z., Bai, B., et al. (2020). Mechanism of eravacycline resistance in clinical enterococcus faecalis isolates from China. Front. Microbiol. 11. doi: 10.3389/fmicb.2020.00916
Yang, Y. S., Chen, H. Y., Hsu, W. J., Chou, Y. C., Perng, C. L., Shang, H. S., et al. (2019). Overexpression of AdeABC efflux pump associated with tigecycline resistance in clinical Acinetobacter nosocomialis isolates. Clin. Microbiol. Infect. 25, 512.e511–512.e516. doi: 10.1016/j.cmi.2018.06.012
Yoon, E.-J., Courvalin, P., Grillot-Courvalin, C. (2013). RND-type efflux pumps in multidrug-resistant clinical isolates of acinetobacter baumannii: major role for adeABC overexpression and adeRS mutations. Antimicrobial Agents Chemotherapy 57, 2989–2995. doi: 10.1128/AAC.02556-12
Yusuf, E., Bax, H. I., Verkaik, N. J., van Westreenen, M. (2021). An update on eight "New" Antibiotics against multidrug-resistant gram-negative bacteria. J. Clin. Med. 10 (5), 1068. doi: 10.3390/jcm10051068
Zhanel, G. G., Cheung, D., Adam, H., Zelenitsky, S., Golden, A., Schweizer, F., et al. (2016). Review of eravacycline, a novel fluorocycline antibacterial agent. Drugs 76, 567–588. doi: 10.1007/s40265-016-0545-8
Zhang, F., Bai, B., Xu, G. J., Lin, Z. W., Li, G. Q., Chen, Z., et al. (2018). Eravacycline activity against clinical S. aureus isolates from China: in vitro activity, MLST profiles and heteroresistance. BMC Microbiol. 18, 211. doi: 10.1186/s12866-018-1349-7
Zheng, J. X., Lin, Z. W., Chen, C., Chen, Z., Lin, F. J., Wu, Y., et al. (2018a). Biofilm Formation in Klebsiella pneumoniae Bacteremia Strains Was Found to be Associated with CC23 and the Presence of wcaG. Front. Cell Infect. Microbiol. 8. doi: 10.3389/fcimb.2018.00021
Zheng, J. X., Lin, Z. W., Sun, X., Lin, W. H., Chen, Z., Wu, Y., et al. (2018b). Overexpression of OqxAB and MacAB efflux pumps contributes to eravacycline resistance and heteroresistance in clinical isolates of Klebsiella pneumoniae. Emerg. Microbes Infect. 7, 139. doi: 10.1038/s41426-018-0141-y
Zong, G., Zhong, C., Fu, J., Zhang, Y., Zhang, P., Zhang, W., et al. (2020). The carbapenem resistance gene blaOXA-23 is disseminated by a conjugative plasmid containing the novel transposon Tn6681 in Acinetobacter johnsonii M19. Antimicrobial Resistance Infection Control 9, 182. doi: 10.1186/s13756-020-00832-4
Keywords: eravacycline, carbapenem-resistant Acinetobacter baumannii, heteroresistance, AdeABC efflux pump, ISAba1
Citation: Li Y-t, Chen X-d, Guo Y-y, Lin S-w, Wang M-z, Xu J-b, Wang X-h, He G-h, Tan X-x, Zhuo C and Lin Z-w (2024) Emergence of eravacycline heteroresistance in carbapenem-resistant Acinetobacter baumannii isolates in China. Front. Cell. Infect. Microbiol. 14:1356353. doi: 10.3389/fcimb.2024.1356353
Received: 15 December 2023; Accepted: 06 March 2024;
Published: 27 March 2024.
Edited by:
Marion Skalweit, Case Western Reserve University, United StatesReviewed by:
Mehrdad Gholami, Mazandaran University of Medical Sciences, IranMohammad Sholeh, Pasteur Institute of Iran (PII), Iran
Copyright © 2024 Li, Chen, Guo, Lin, Wang, Xu, Wang, He, Tan, Zhuo and Lin. This is an open-access article distributed under the terms of the Creative Commons Attribution License (CC BY). The use, distribution or reproduction in other forums is permitted, provided the original author(s) and the copyright owner(s) are credited and that the original publication in this journal is cited, in accordance with accepted academic practice. No use, distribution or reproduction is permitted which does not comply with these terms.
*Correspondence: Zhi-wei Lin, 422156321@qq.com; Chao Zhuo, chao_sheep@263.net
†These authors have contributed equally to this work