Corrigendum: Molecular Characterization of Carbapenem and Ceftazidime-Avibactam Resistant Enterobacterales and Horizontal Spread of blaNDM-5 Gene at a Lebanese Medical Center
- 1Department of Pathology and Laboratory Medicine, American University of Beirut Medical Center, Beirut, Lebanon
- 2Department of Microbiology, Faculty of Medicine, University Hospital in Pilsen, Charles University, Pilsen, Czechia
- 3Department of Microbiology, University Hospital of Larissa, Larissa, Greece
- 4Faculty of Health Sciences, University of Balamand, Beirut, Lebanon
Introduction: In the battle against multidrug-resistant bacterial infections, ceftazidime- avibactam (CZA) stands as a pivotal defense, particularly against carbapenemresistant (CR) Gram-negative pathogens. However, the rise in resistance against this drug poses a significant threat to its effectiveness, highlighting the critical need for in-depth studies about its resistance mechanisms.
Methods: This research focuses on the genomic characterization of CR- and CZA-resistant Escherichia coli (n=26) and Klebsiella pneumoniae (n=34) strains, harboring the blaNDM and/or blaOXA-48-like genes, at a major Lebanese tertiary care medical center, using whole genome sequencing (WGS).
Results: Our findings revealed a notable prevalence of blaNDM in all K. pneumoniae strains isolates, with 27 of these also harboring blaOXA-48. On the other hand, E. coli strains predominantly carried the blaNDM-5 gene. Whole genome sequencing (WGS) identified a predominance of ST383 among K. pneumoniae strains, which possessed a multi-replicon IncFIB-IncHI1B plasmid harboring the blaNDM-5. Additionally, various Inc group plasmids in K. pneumoniae across multiple sequence types were found to carry the blaNDM. Similarly, diverse STs of E. coli were observed to carry blaNDM-5 on different plasmids.
Discussion: The study underscores NDM carbapenemases as a paramount resistance mechanism in Lebanon,jeopardizing critical last-resort treatments. It also illuminates the role of varied sequence types and mobile genetic elements in the spread of NDM resistance,stressing the urgent need for strategies to mitigate this threat, especially in nosocomial infections.
Introduction
Carbapenems are the drug of choice for the treatment of complicated multidrug-resistant (MDR) bacterial infections. However, their misuse has led to an increased emergence of resistant organisms (Gupta et al., 2011). The World Health Organization (WHO) has classified carbapenemase-producing Enterobacterales (CPE), especially Klebsiella pneumoniae and Escherichia coli, as a worldwide public health concern due to their proliferation in hospital settings (https://apps.who.int/iris/bitstream/handle/10665/312226/WHO-UHC-SDS-2019.6-eng.pdf?sequence=1&isAllowed=y). Resistance mechanisms to carbapenems include the increased activity of the efflux pumps, porin loss, and β-lactamases production, particularly carbapenemases (Ye et al., 2018). These carbapenemases are divided according to Ambler into four classes, with only class A [i.e., Klebsiella pneumoniae carbapenemase (KPC)], Class B [i.e. New-Delhi Metallo-β-lactamase (NDM)], and Class D [i.e., Oxacillinase (OXA)-48 and OXA-48-like] being associated with carbapenemase activity (Ambler, 1980; van Duin and Doi, 2017; Kopotsa et al., 2019).
NDM is among the most common and clinically significant carbapenemase in Enterobacterales capable of efficiently hydrolyzing a wide range of β-lactams, including penicillins, carbapenems, and cephalosporins (Nordmann et al., 2011; Nordmann and Poirel, 2014). It has rapidly spread across the Middle East, the Indian subcontinent, and the Balkan region (Dortet et al., 2014; Wu et al., 2019). In the United Kingdom, in 2011, NDM-5 was first detected, and two amino acid substitutions at positions 88 and 154 lead to increased NDM enzymatic activity (Hornsey et al., 2011).
Furthermore, there has been a notable spread of genes encoding OXA-48-like carbapenemases. In 2001, a K. pneumoniae strain resistant to all available β-lactams was reported in Turkey. A subsequent study by Poirel et al. in 2004 identified this isolate as carrying a novel bla variant, blaOXA-48 (Poirel et al., 2004). Isolates harboring blaOXA-48-like genes have garnered attention due to their rapid horizontal transmission and increasing detection across the Middle East and North Africa showing resistance to β-lactamase inhibitors (Poirel et al., 2004; Nordmann and Poirel, 2014; Al-Zahrani and Alsiri, 2018; Mairi et al., 2018). Co-production of NDM- and OXA-48-like enzymes has been increasingly reported, particularly in patients with travel histories from the Middle East, Southeast Asia, Europe, China, and Africa (Doi et al., 2014; Avolio et al., 2017; van Duin and Doi, 2017; Otlu et al., 2018; Solgi et al., 2020; Chudejova et al., 2021; Lorenzin et al., 2022).
In Lebanon, few studies highlighted the prevalence of carbapenem resistance (CR) and their mechanisms among clinical isolates in this country (Baroud et al., 2013; Araj et al., 2018). For example, a study conducted between 2008 and 2012 showed an increase in reduced susceptibility to ertapenem from 0.4% to 1.6% (Beyrouthy et al., 2014). By 2019, resistance levels in Enterobacterales reached 3.3%, with higher rates observed in Pseudomonas spp. (27.3%) and Acinetobacter spp. (53.7%) (Rima et al., 2022). In 2008, the first case of OXA-48 was detected in K. pneumoniae in Lebanon (Matar et al., 2008). Moreover, the first cases of NDM-1 and NDM-5, isolated in 2010 and in 2017, respectively, were identified in K. pneumoniae isolates (El-Herte et al., 2012; Nawfal Dagher et al., 2019). Between 2015 and 2019, carbapenemase-producing E. coli were found to be positive for blaOXA-48 (n=11), blaOXA-181 (n=12), and blaNDM-5 (n=4) and blaNDM-19 (n=9), while K. pneumoniae isolates harbored blaOXA-48 (n=6), blaOXA-181 (n=1), and blaNDM-5 (n=1) (Bitar et al., 2018; Rima et al., 2022). A recent study in Lebanon in 2019, following the introduction of ceftazidime-avibactam (CZA) as a treatment for extended spectrum β-lactamase (ESBL) and multi-drug resistant (MDR) infections, analyzed 150 isolates (E. coli and K. pneumoniae). These were classified as ESBL (n=20), MDR (n=20), or carbapenem resistant (CR) (n=110). All CR isolates exhibited a 100% resistance to ertapenem. Moreover, 60% (n=30) of CR E. coli and 65% (n=39) of CR K. pneumoniae showed resistance to CZA, with all resistant isolates being producers of NDM and/or OXA-48-like enzymes (Sobh et al., 2021).
This study, thus, was warranted at expanding our understanding of the resistance and dissemination by performing a whole genome characterization of CR- and CZA-resistant E. coli and K. pneumoniae strains producing NDM and/or OXA-48-like carbapenemases, at a major Lebanese tertiary care medical center.
Materials and methods
Bacterial isolates and determination of carbapenems minimal inhibitory concentrations
This study encompassed characterization a total of 60 Enterobacterales isolates (consisted of 34 isolates of K. pneumoniae and 26 isolates of E. coli) recovered at the Clinical Microbiology Laboratory of the Department of Pathology and Laboratory Medicine at the American University of Beirut Medical Center, between 2019 and 2021. These isolates as were identified by the matrix-assisted laser desorption ionization-time of flight mass spectrometry (MALDI-TOF MS) using the MALDI-biotyper software (Bruker Daltonics, Bremen, Germany). The MICs determination to three carbapenems and CZA was carried out as reported earlier (Sobh et al., 2021).
For further characterization, all isolates were sent to Charles University, Faculty of Medicine in Pilsen, Czech Republic. Carbapenemase production and activity were assessed using MALDI-TOF MS with the meropenem hydrolysis assay, as described by Rotova et al. (Rotova et al., 2017), and the double disk synergy test incorporating EDTA, phenylboronic acid, and temocillin, following previously described methodologies (Lee et al., 2003). In addition antimicrobial susceptibility testing was conducted using the broth microdilution method, adhering to the EUCAST guidelines, with results interpreted based on the EUCAST 2024 criteria (https://www.eucast.org/clinical_breakpoints) (Clinical breakpoints - breakpoints and guidance v13.0, 2023).
Gene content and multilocus sequence typing by PCR
The presence of blaIMP-like, blaKPC-like, blaNDM-like, blaVIM-like, and blaOXA-48-like genes was tested via PCR (Papagiannitsis et al., 2015). Additionally, multilocus sequence typing (MLST) was performed on the isolate collection as previously described (Diancourt et al., 2005; Wirth et al., 2006; Brisse et al., 2009).
Short-reads sequencing and analysis
Based on MLST and MIC results, 17 K. pneumoniae and 10 E. coli isolates were selected as representatives for whole genome sequencing. Genomic DNA was extracted using NucleoSpin Microbial DNA kit (Machery-Nagel, Germany). A multiplexed library was prepared using Nextera XT library preparation kit, and sequencing was performed on the Illumina MiSeq platform (Illumina Inc., San Diego, CA, United States) using the MiSeq v3 reagent kit using 600 cycle cartridges. Initial paired-end reads were quality assessed using fastqc, then trimmed and filtered to high quality using fastp (Andrews, 2010; Chen et al., 2018). Subsequent reads were assembled by a de Bruijn graph-based de novo assembler SPAdes (Prjibelski et al., 2020). The assembled contigs were analyzed to detect sequence types (STs) using MLST software, virulence genes using VFanalyzer, resistance genes using ResFinder, and plasmid incompatibility (Inc) types using PlasmidFinder (Carattoli et al., 2014; Liu et al., 2019; Florensa et al., 2022). Additionally, these contigs were also uploaded to PHASTER to detect phage genomes, CRISPRfinder to detect CRISPR-cas systems, and ISfinder to detect insertion sequences (Siguier et al., 2006; Arndt et al., 2016).
Long-read sequencing and analysis
Based on the previous short-read results, seven and four representative isolates corresponding to K. pneumoniae and E. coli isolates, respectively, were selected for long-read sequencing using PacBio Sequel I (Pacific Biosciences, CA, United States). Briefly, the extracted DNA was subjected to shearing using the Megaruptor 2 (Diagenode, Liege, Belgium); this will generate sheared fragments of ~15 kb each. Library preparation was performed using the microbial multiplexing protocol according to the manufacturer’s recommendation. During the library preparation, no size selection was performed. Using SMRT Link v10.1, the microbial assembly pipeline was used for the assembly and the circularizing of contigs using a minimum seed coverage of ×30. The assembled contigs were then analyzed using the same procedures as the short-read assembly. Finally, all genomes were annotated using the NCBI’s Prokaryotic Genome Annotation Pipeline (Tatusova et al., 2016; Haft et al., 2018; Li et al., 2021).
Phylogeny and single nucleotide polymorphism
To study the genetic diversity and phylogenetic relationship of the studied isolates and global isolates, genomes of K. pneumoniae, specifically of the ST383 type, were retrieved from the NCBI assembly database, encompassing both complete and draft genomes, totaling 105 genomes. Using parsnp v1.7.4 (available in the harvest suit), a core genome phylogeny of the model General Time Reversible (GTR) based on SNP and recombination, an SNP-based phylogeny was constructed between the sequenced genomes in this study and the 105 genomes downloaded from the NCBI database using KP1674 as a reference (complete circular genome) and K. quasipneumoniae as an outgroup (Edgar, 2004; Bruen et al., 2006; Price et al., 2010; Treangen et al., 2014). Briefly, SNPs identified in local collinear blocks were subsequently used for reconstructing an approximate maximum-likelihood tree using FastTree, while including the general time reversible (GTR) model of nucleotide substitution. The Shimodaira–Hasegawa test implemented in FastTree2 was used to assess the support for significant clustering in the observed phylogeny. The interactive tree of life or iTOL (https://itol.embl.de/) was used for the graphic illustration of the trees along with relative annotations.
Moreover, the SNPs between the ST383 genomes were detected to determine the extent of clonal dissemination in the hospital. SNPs were detected using snippy multicommand (snippy-base application v4.5.0) (Seeman, 2015), which generates a core genome multiple alignment against the common reference (KP1674). The pipeline detects the variants and generates a single file for each isolate listing the different variations.
Comparison of antimicrobial multidrug-resistant region
The MDR sequence from KP1633 isolate (complete circular genome) was used as a reference to compare with the rest of the isolates. Easyfig v2.2.0 was used for generating the figure (Sullivan et al., 2011). It uses blastn from the package blast + by NCBI to generate a similarity report between the fragments (Camacho et al., 2009). An alignment between the short-read sequences and the MDR sequence characterized with Pacbio was used to generate a consensus and then annotated using Prokka to include in the generation of the figure (Seemann, 2014).
Data availability
All genomes have been submitted to the NCBI database. Accession numbers are available under the BioProject PRJNA952851.
Results
Phenotypic antimicrobial resistance profile of the isolates
All 34 K. pneumoniae isolates showed resistance against CZA, carbapenems, tazobactam, ciprofloxacin, and tetracycline. Moreover, all isolates showed resistance against amikacin and gentamicin (except one isolate) (Table 1). Similarly, all E. coli isolates (n=26) were also resistant against CZA, carbapenem, and showed resistance to ciprofloxacin and tazobactam and tetracycline (except two isolates). However, all isolates were susceptible against amikacin apart from two resistant isolates (Table 2).
Molecular characteristics of the isolates
PCR screening showed that all the K. pneumoniae isolates were positive for blaNDM (n=34), while 27 of them were simultaneously positive for the presence of blaOXA-48. On the other hand, the E. coli isolates carried blaNDM-5 gene.
MLST was performed on all the collection. MLST of the K. pneumoniae isolates revealed the presence of a major ST: ST383 (n=26), while few other STs were present as well: ST101 (n=2), ST111 (n=1), ST147 (n=1), ST307 (n=1), ST147(n=1), ST39 (n=1), and ST15 (n=1) (Table 1). Conversely, based on Achtman Scheme, MLST of the E. coli isolates showed several STs circulating within the hospital: ST405 (n=9), ST167 (n=4), ST617 (n=3), ST361 (n=2), ST46 (n=2), ST648 (n=1), ST2450 (n=2), ST131 (n=1), ST998 (n=1), and ST1284 (n=1) (Table 2).
Whole genome sequencing
Based on the susceptibility profiles, antibiotic resistance genes (PCR results), and MLST results, representative isolates (17 K. pneumoniae and 10 E. coli) were selected for sequencing using short-read sequencing. WGS data showed that 13 of the 17 analyzed K. pneumoniae isolates carried blaNDM-5 while four isolates carried blaNDM-1. ST383 isolates co-harbored the blaNDM-5 and blaOXA-48 genes, while another ST147 isolate co-harbored blaNDM-1 and blaOXA-232. All the isolates harbored additional resistance genes against aminoglycoside [armA, and/or aph(3’)-VI, and/or aac(6′)-Ib], beta-lactams (blaSHV-145, blaOXA-48, blaNDM-5/1, and/or blaTEM-1B), quinolones (OqxB, OqxA, and/or qnrS1), streptogramin b [msr(E)], macrolides [mph(A), mph(E)], and tetracyclines [tet(A)] (Table 3). The genomic antibiotic resistance determinants were correlated with the resistant phenotype (Table 1). In the 10 analyzed isolates of E. coli, WGS data analysis revealed the presence of blaNDM-5 in all isolates. Moreover, additional resistance genes were detected conferring resistance to beta-lactams (blaNDM-5, and/or blaCTX-M-15, and/or blaCMY-145), macrolides (mph(A)), quinolones (the presence of efflux pump AcrAB), aminoglycoside in some isolates [aac(6′)-Ib-cr], and tetracyclines [tet(B)] (Table 4 and Supplementary Table S2). The genetic antibiotic resistance determinants were associated with the resistant phenotype (Table 2).
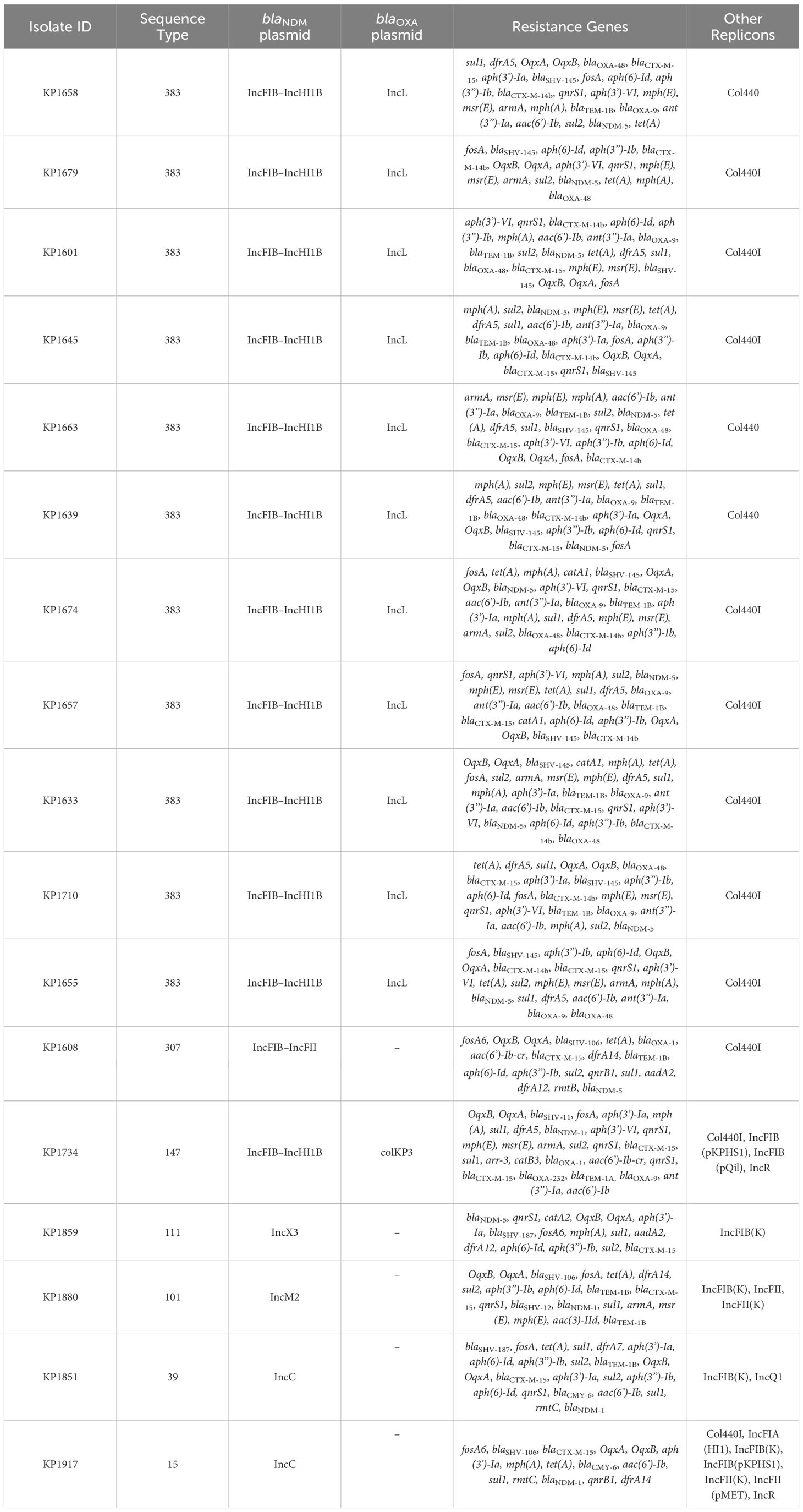
Table 3 WGS data representing resistance gene content and plasmid replicons of the K. pneumoniae isolates.
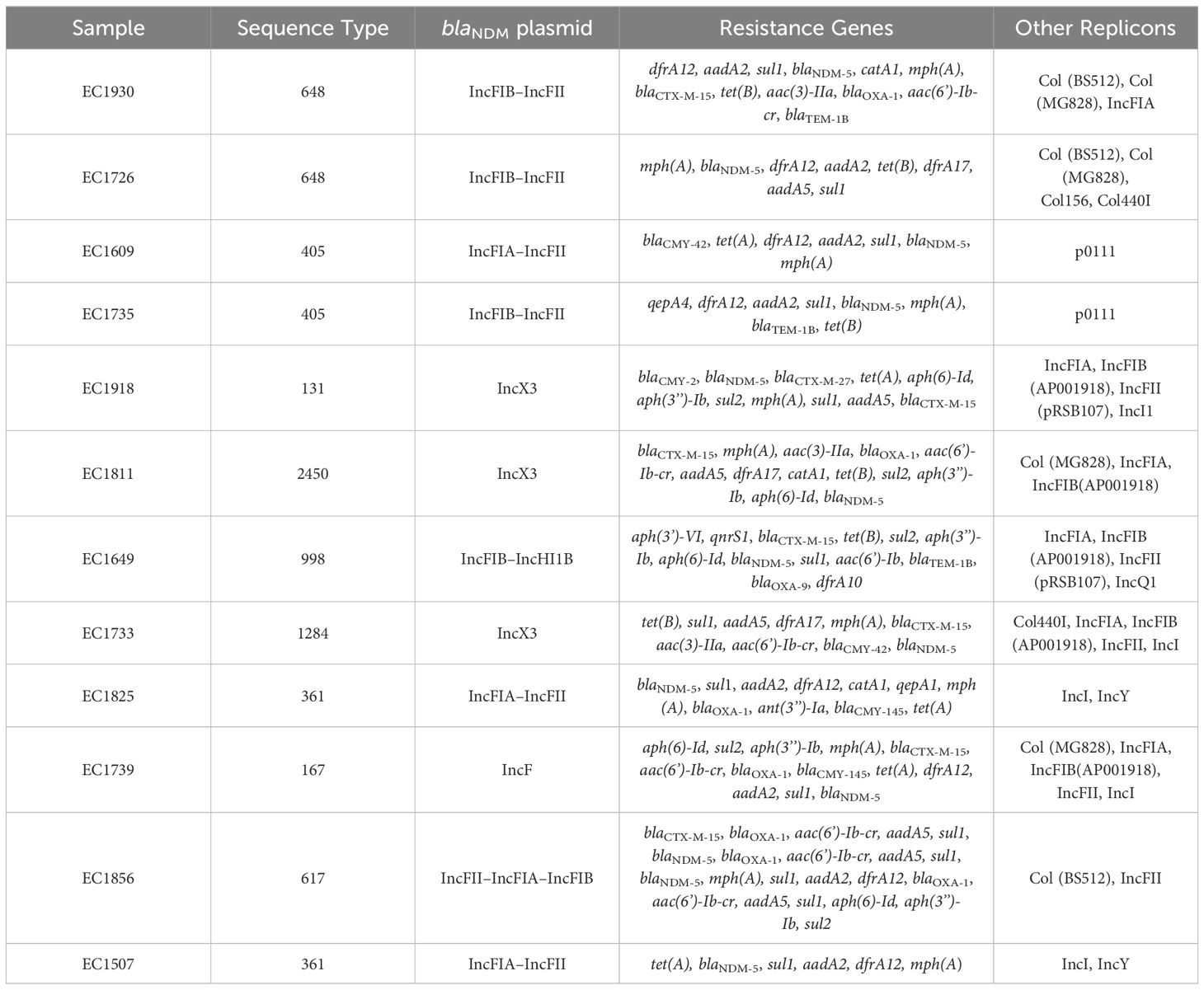
Table 4 WGS data representing resistance gene content and plasmid replicons of the E. coli isolates.
Based on the short-read sequencing results, seven K. pneumoniae isolates (one isolate each from ST307, ST15, ST147, ST39, and ST101 and two isolates from ST383) and four E. coli (one isolate each from ST405, ST131, ST2450, and ST617) were selected for long-read sequencing to have complete circular chromosomes and plasmids for downstream analysis.
In K. pneumoniae, the long-read sequencing of the two ST383, KP1633, and KP1674 showed that the blaNDM-5 was harbored on a fusion plasmid (IncFIB and IncHI1B) that was 372,845 bp (pKP1633LB_IncFIB_IncHI1B_NDM5) and 372,828 bp (pKP1674LB_IncFIB_IncHI1B_NDM5) in size, respectively. Upon blasting, the plasmid against the NCBI database showed 100% query coverage and 99.9% sequence identity with pFQ61_ST383_NDM_5 that was 376,754 bp in size (accession number CP091814), a plasmid isolated from a clinical K. pneumoniae strain in Qatar in 2016. In silico plasmid analysis of the rest of the ST383 K. pneumoniae isolates that were sequenced using short reads showed that all ST383 isolates carried the same plasmid type. Furthermore, pKP1633LB_IncFIB_IncHI1B_NDM5 carried a 35-kb MDR sequence (from 286,445 bp to 323,218 bp). The MDR region was composed of a transposase from IS1, followed by two additional transposases and ends with a transposase. Furthermore, the same MDR region was also detected in isolate KP1674 on the plasmid pKP1674LB_IncFIB_IncHI1B_NDM5 from position 52,157–85,730. In the case of short-read sequenced isolates, the MDR presence was confirmed using blast, and later, a consensus was made for subsequent analysis. Data revealed that this MDR sequence was present in most ST383 isolates, with the exception of isolate KP1679 lacking four resistance genes [blaTEM-1, blaOXA-9, ant(3′)-I, and aac(6′)-Ib]. Similarly, isolate KP1655 noted the absence of blaTEM-1. In addition to finding missing resistance genes across the isolates, some IS transposases were also found missing (IS1380-like only found in three isolates). Interestingly, the starting and ending IS transposases were found in all isolates (Figure 1). Furthermore, all ST383 K. pneumoniae strains also carried the IncL plasmid harboring blaOXA-48, which had the same plasmid size (68,942 bp). This plasmid has been reported extensively, and high similarity scores were noticed upon blasting against the NCBI database (Poirel et al., 2012a; Poirel et al., 2012b; Alousi et al., 2018).
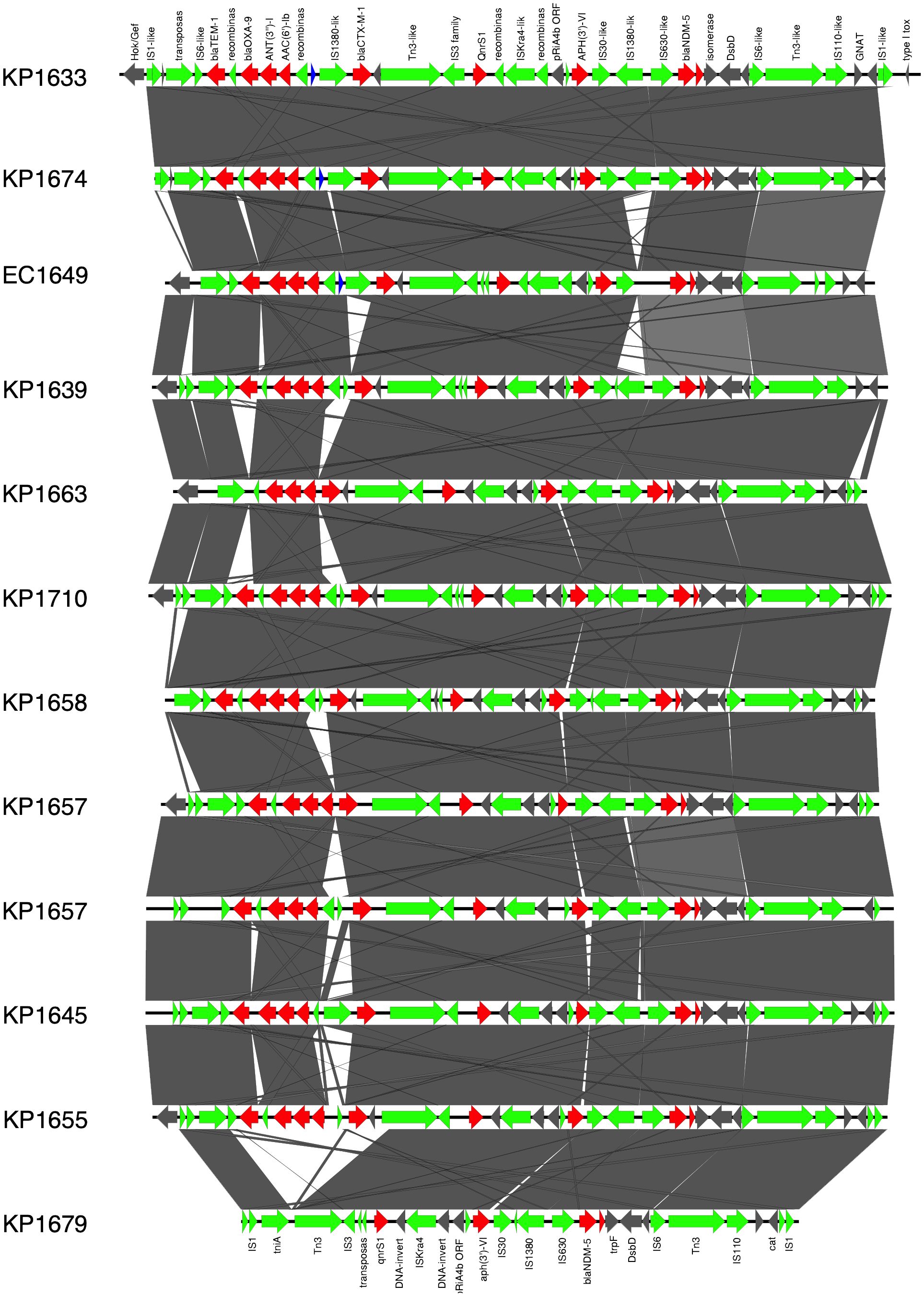
Figure 1 Prophage harboring blaNDM-5 region comparison, found in ST383 K. pneumonia plasmids and in E. coli EC1609, with the prophage sequence detected in the long-read sequenced KP1633 isolate. Red represents the resistance genes, green the insertion sequences, transposases in blue DUF domains; and in gray, other genes.
Other STs such as ST111 KP1859 carried the blaNDM-5 on an IncX3 plasmid, while ST307 KP1608 carried the blaNDM-5 on an IncFII plasmid. On the other hand, the blaNDM-1 was carried on different Inc plasmids; IncM2 plasmid (pKP1880LB_IncM2_NDM1 87,450 bp) was detected in KP1880 ST 101. This plasmid exhibited 100% query coverage and 100% sequence identity with AR_0127 plasmid (87,450 bp) identified in a Salmonella enterica clinical strain in USA in 2018. Moreover, ST39 KP1851 and ST15 KP1917 both carried the blaNDM-1 on the same IncC plasmid (pKP1851LB_IncC_NDM1 137,593 bp and pKP1917LB_IncC_NDM1 140,300 bp, respectively) (99% query coverage and 99.9% sequence identity). This plasmid exhibited 100% sequence identity and 98% query coverage with pNDM-US (accession number CP006661.1), which was isolated from a clinical strain in 2016 in the USA. Finally, ST147 KP1734 carried the blaNDM-1 on an IncFIB-IncHI1B plasmid (269,122 bp), while blaOXA-232 was carried on a ColKP3 plasmid (6,141 bp). The IncFIB–IncHI1B plasmid had similar sequence to those identified in ST383 isolates, KP1633 and KP1674, and the ColKP3 showed the same nucleotide sequence as that of pC06114_4 (accession number CP016038.1) (100% identity and 100% query coverage), a plasmid isolated in 2016 in Germany.
All the characterized E. coli isolates carried the blaNDM-5 gene. Long-read sequencing revealed that ST131 EC1918 and ST2450 EC1811 isolates carried the blaNDM-5 gene on the same IncX3 plasmid (79,672 bp). Moreover, short-read sequencing showed that ST1284 EC1733 carried an IncX3 plasmid, identical to pKP1859LB_IncX3_NDM5. Moreover, the blaNDM-5 gene was carried on IncFIA–IncFII (pEC1609LB_IncFIA_IncFII_NDM5, 118,953 bp) in ST405 EC1609 and on IncFII–IncFIA–IncFIB (pEC1856LB_IncFII_IncFIA_IncFIB_NDM5, 118 140,386 bp) in ST617 EC1856. Short-read sequences scaffolding showed that IncF plasmids (with different structures and sequences) carrying blaNDM-5 gene was found in the rest of E. coli despite STs.
ST383 K. pneumoniae virulence factor analysis revealed the presence of virulence genes responsible for acriflavine resistance, type VI secretion system, synthases, transporters, esterase, adherence, regulation, immune modulation, fimbriae, and delivery. Some genes were found to be missing, for example, the regulatory gene for the mucoid phenotype rmpA2 (KP1710, KP1679, KP1663, KP1657, KP1658, KP1655, KP1645, KP1639, and KP1601). In E. coli, the presence of the virulence genes was associated with acriflavine resistance, invasion, secretion, adherence, fimbriae, enterobactin, and yersiniabactin. Notably in ST648 EC1930 in comparison with ST648 EC1726, some virulence factors were found missing: fim genes for fimbriae, gsp genes for secretion, irp genes for capsule and invasion characteristics, and ybt genes for yersiniabactin. In addition, in comparison between the ST405 EC735 and ST405 EC1609, genes of esp variations responsible for secretion and two genes for adherence (papE and papF) were found to be missing (Supplementary Tables S1, S2).
Phylogeny and clonality
To evaluate the relatedness of the K. pneumoniae ST383 isolates in the study, global SNPs phylogeny was performed on all K. pneumoniae ST383 isolates in the NCBI database. The phylogenetic tree showed that our ST383 isolates clustered together along with previously detected NDM-5 producing ST383 isolates from Lebanon (Figure 2). Furthermore, SNP analysis was performed on all isolates belonging to this clade and showed high similarity with SNPs ranging from 11 to 73 (read, Table 5 and Supplementary Table S3). Plasmid analysis of the Lebanese clade showed that all the clustered isolates carried the blaNDM-5 gene on the same Inc plasmid (IncFIB–IncHI1B) previously detected. Remarkably, these clustered isolates were all NDM-5/OXA-48 co-producers, and their blaOXA-48 gene was similarly carried by an IncL plasmid (Figure 2).
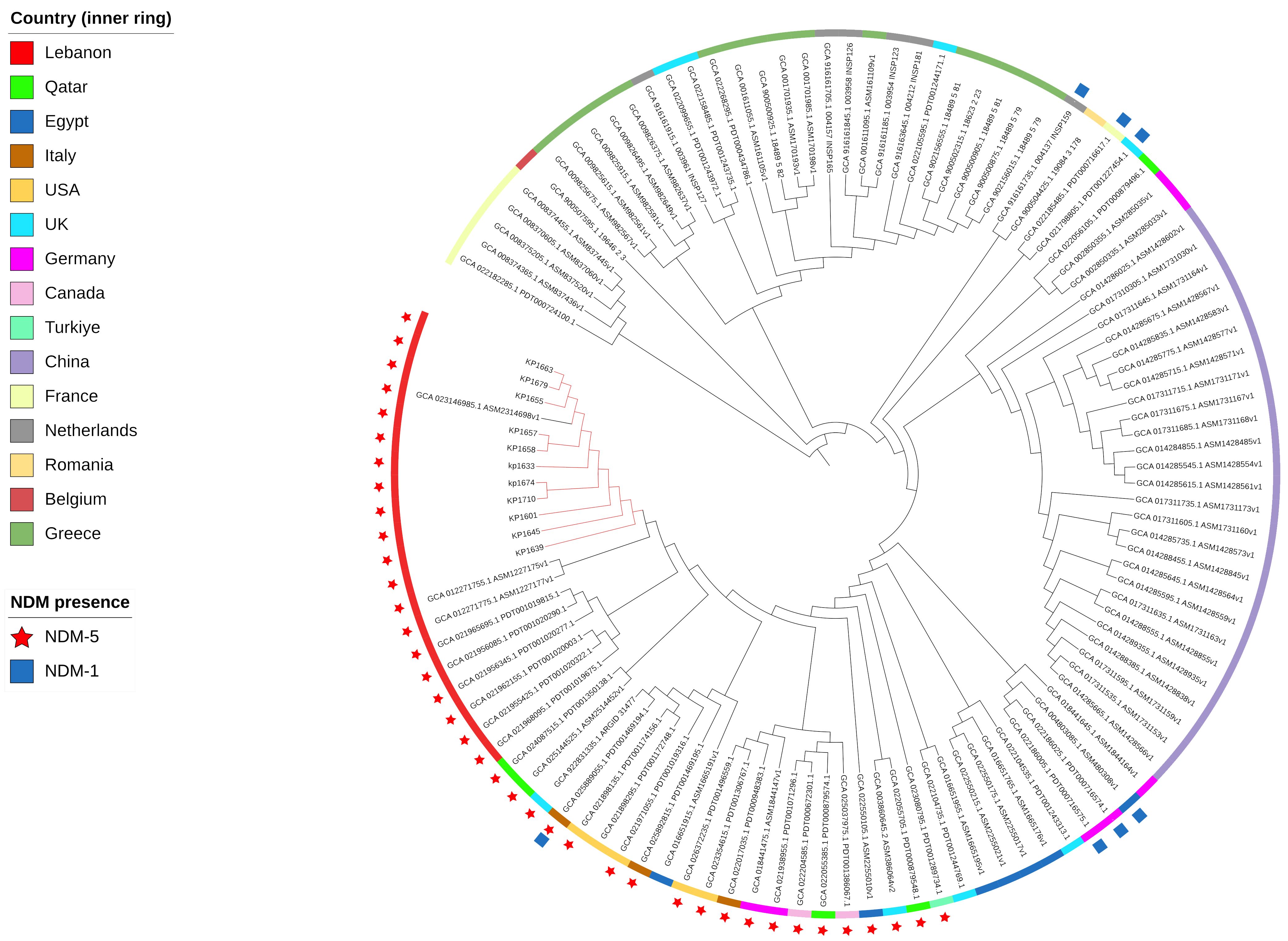
Figure 2 SNP-based phylogeny for global K. pneumoniae ST383 with 105 isolates downloaded from NCBI database. Red nodes highlight the isolates used in this study.
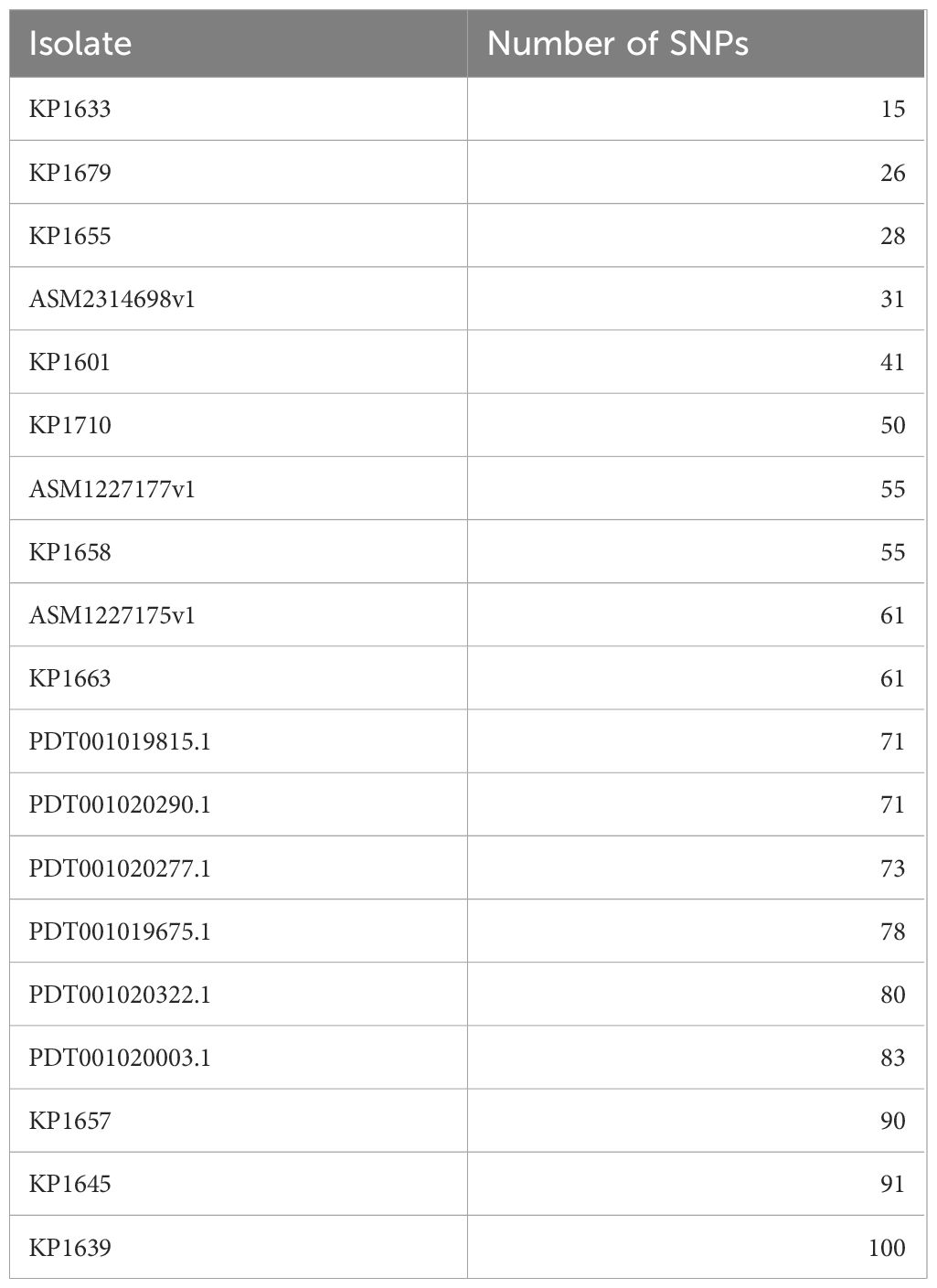
Table 5 Number of SNPs in the K. pneumoniae ST383 isolates in this study as compared to the reference KP1674.
Moreover, the clonality of the K. pneumoniae ST383 strains was assessed through analyzing the CRISPR array sequences. A population of any isolates that went through the same environmental conditions (subjected to phages or plasmids) will have a similar CRISPR array, since these sequences (correlated with foreign sequences) are added in chronological order (Gagaletsios et al., 2022). CRISPRCasFinder showed that all genomes have the CRISPR/Cas I-E type. The sequences were then aligned, and a SNPs-based phylogenetic tree was executed (Figure 3). The tree showed that the isolates were divided between two distinct clades (A and B) and one isolate (KP1655) that has two CRISPR arrays residing alone. The two clades were composed of both isolates from this study and previously identified in Lebanon. This result shows that the Lebanese isolates’ population is originating from at least three different ancestors, which underwent different environmental conditions.
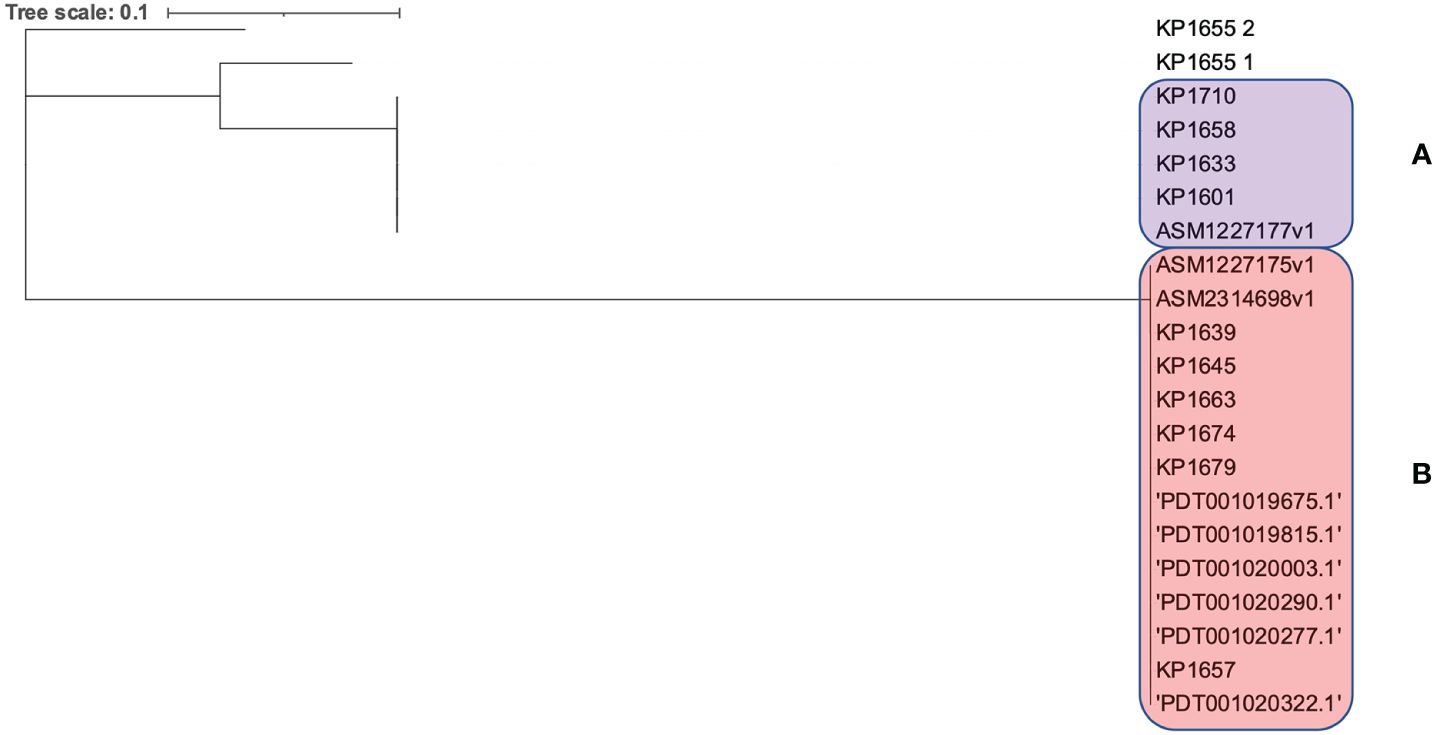
Figure 3 SNPs based phylogeny for the CRISPR/Cas arrays of the Lebanese ST383 K. pneumoniae isolates. (A) represents the clade in the pink while (B) represents the clade in purple.
Discussion
Lebanon confronts significant antibiotic resistance, notably with the recent emergence of resistance to ceftazidime-avibactam in CRE treatments (Araj et al., 2018; Iskandar et al., 2021; Rima et al., 2022; Zakhour et al., 2024),. This study investigated CR K. pneumoniae and E. coli strains exhibiting resistance to last line antibiotic choices, including carbapenems and CZA, as reported by (Sobh et al., 2021). It intended to explore mechanisms involved in their resistance and dissemination especially through characterizing the genetic features of blaOXA-48-like and/or blaNDM-positive K. pneumoniae and E. coli isolates recovered at a major Lebanese medical center. Sequence data showed the presence of blaNDM on plasmids of different Inc groups, in K. pneumoniae belonging to different STs (Table 3). Most of the K. pneumoniae isolates belonged to ST383 and carried a multireplicon plasmid (IncFIB–IncHI1B) with blaNDM-5. This sequence type has been reported in different parts of the world, including countries around the Mediterranean basin, where it contributes to the burden of CRE (Turton et al., 2018; Edward et al., 2022; Tsui et al., 2023). The detection of blaNDM-5 positive isolates raises significant concerns due to its potent ability to break down a broad spectrum of β-lactam antibiotics, including carbapenems. Recognized globally for its contribution to antibiotic resistance (Daaboul et al., 2023; Linkevicius et al., 2023; Simner et al., 2023; Tao et al., 2023), the prevalence of blaNDM-5 underscores the urgent challenge it poses to treating bacterial infections. The concurrent presence of blaNDM-5 and blaOXA-48 in our strains severely limits treatment options with last-resort antibiotics like ceftazidime-avibactam. This synergy of resistance genes, documented across the MENA region (Dandachi et al., 2016; Aqel et al., 2017; Rojas et al., 2017; Solgi et al., 2020; Ahmed El-Domany et al., 2021) and mirrored in findings from Italy (Lorenzin et al., 2022), emphasizes the global nature of this threat and underscores the need for international efforts in surveillance and containment. This finding highlights the important role of horizontal transfer in the worldwide spread of blaNDM resistance determinants (Paskova et al., 2018).
Additionally, the close clonal phylogenetic relationship of ST383 isolates was confirmed by SNPs phylogeny. ST383 is a high-risk clone associated with the spread of diverse resistance mechanisms (Papagiannitsis et al., 2010) like blaKPC and blaVIM. Nevertheless, CRISPR-Cas array analysis revealed genetic diversity within ST383 K. pneumoniae isolates, identifying distinct clades and indicating diverse ancestral origins, suggesting varied exposure to environmental pressures and selective forces.
On the other hand, blaNDM-5 was also found in E. coli isolates. E. coli isolates, which belonged to diverse STs, harbored a variety of blaNDM-5-carrying plasmids. The variation in plasmids involved in the spread of blaNDM-5 underlines the important role of mobile elements, like insertion sequences and transposons, in the horizontal spread of resistance mechanisms. However, short-read sequencing data suggested that, in most of the E. coli, which belonged to diverse STs, the blaNDM-5 gene was localized on IncF-type plasmids. Additionally, WGS data confirmed the presence of similar blaNDM-5-carrying plasmids, like pKP1674LB_IncFIB_IncHI1B_NDM5 (IncFIB and IncHI1B) and pEC1609LB_IncFIA_IncFII_NDM5 (IncF-type), into different STs or species. These findings highlight the important role of plasmids in the horizontal gene transfer (HGT) of resistance genes in the institution and community where such strains exist.
Conclusion
In conclusion, the current study confirmed the fact that production of NDM carbapenemases is one of the most clinically significant resistance mechanisms among CRE isolates in Lebanon, including ceftazidime-avibactam. This finding is in line with previous studies highlighting the importance of blaNDM spread, canceling last-line therapeutic options worldwide (Chudejova et al., 2021). Additionally, our study showed the contribution of divers STs and mobile genetic elements to the success of NDM resistance mechanism. Furthermore, the high-risk clone ST383 and the broad host range plasmids IncH and IncF has contributed to the successful prevalence and dissemination of NDM resistance. Thus, such findings emphasize the need of scrutinizing the implementation of infection control aspects to curb/control the spread of these “superbugs”.
Data availability statement
The datasets presented in this study can be found in online repositories. The names of the repository/repositories and accession number(s) can be found in the article/Supplementary Material.
Ethics statement
Ethical approval was not required for the study involving humans in accordance with the local legislation and institutional requirements. Written informed consent to participate in this study was not required from the participants or the participants’ legal guardians/next of kin in accordance with the national legislation and the institutional requirements.
Author contributions
GS: Methodology, Writing – review & editing. GA: Supervision, Writing – review & editing. MF: Data curation, Formal analysis, Software, Visualization, Writing – original draft. TS: Formal analysis, Investigation, Methodology, Writing – original draft. JH: Funding acquisition, Resources, Writing – review & editing. CP: Conceptualization, Data curation, Formal analysis, Investigation, Validation, Writing – original draft, Writing – review & editing. ME: Writing – review & editing. IB: Conceptualization, Formal analysis, Funding acquisition, Investigation, Project administration, Supervision, Visualization, Writing – original draft, Writing – review & editing.
Funding
The author(s) declare financial support was received for the research, authorship, and/or publication of this article. The study was supported by research project grants NU20J-05–00033 provided by the Czech Health Research Council and the project National Institute of Virology and Bacteriology (Program EXCELES, ID project no. LX22NPO5103) funded by the European Union–Next Generation EU.
Conflict of interest
The authors declare that the research was conducted in the absence of any commercial or financial relationships that could be construed as a potential conflict of interest.
The author(s) declared that they were an editorial board member of Frontiers, at the time of submission. This had no impact on the peer review process and the final decision.
Publisher’s note
All claims expressed in this article are solely those of the authors and do not necessarily represent those of their affiliated organizations, or those of the publisher, the editors and the reviewers. Any product that may be evaluated in this article, or claim that may be made by its manufacturer, is not guaranteed or endorsed by the publisher.
Supplementary material
The Supplementary Material for this article can be found online at: https://www.frontiersin.org/articles/10.3389/fcimb.2024.1407246/full#supplementary-material
References
Ahmed El-Domany, R., El-Banna, T., Sonbol, F., Abu-Sayedahmed, S. H. (2021). Co-existence of NDM-1 and OXA-48 genes in Carbapenem Resistant Klebsiella pneumoniae clinical isolates in Kafrelsheikh, Egypt. Afr Health Sci. 21, 489–496. doi: 10.4314/ahs.v21i2.2
Alousi, S., Salloum, T., Arabaghian, H., Matar, G. M., Araj, G. F., Tokajian, S. T. (2018). Genomic Characterization of MDR Escherichia coli Harboring bla(OXA-48) on the IncL/M-type Plasmid Isolated from Blood Stream Infection. BioMed. Res. Int. 2018, 3036143. doi: 10.1155/2018/3036143
Al-Zahrani, I. A., Alsiri, B. A. (2018). The emergence of carbapenem-resistant Klebsiella pneumoniae isolates producing OXA-48 and NDM in the Southern (Asir) province, Saudi Arabia. Saudi Med. J. 39, 23–30. doi: 10.15537/smj.2018.1.21094
Ambler, R. P. (1980). The structure of beta-lactamases. Philos. Trans. R Soc. Lond B Biol. Sci. 289, 321–331. doi: 10.1098/rstb.1980.0049
Andrews, S. (2010) FastQC: A Quality Control Tool for High Throughput Sequence Data. Available online at: http://www.bioinformatics.babraham.ac.uk/projects/fastqc/
Aqel, A. A., Giakkoupi, P., Alzoubi, H., Masalha, I., Ellington, M. J., Vatopoulos, A. (2017). Detection of OXA-48-like and NDM carbapenemases producing Klebsiella pneumoniae in Jordan: A pilot study. J. Infect. Public Health 10, 150–155. doi: 10.1016/j.jiph.2016.02.002
Araj, G. F., Avedissian, A. Z., Itani, L. Y., Obeid, J. A. (2018). Antimicrobial agents active against carbapenem-resistant Escherichia coli and Klebsiella pneumoniae isolates in Lebanon. J. Infect. Dev. Ctries 12, 164–170. doi: 10.3855/jidc.9729
Arndt, D., Grant, J. R., Marcu, A., Sajed, T., Pon, A., Liang, Y., et al. (2016). PHASTER: a better, faster version of the PHAST phage search tool. Nucleic Acids Res. 44, W16–W21. doi: 10.1093/nar/gkw387
(2023) Clinical breakpoints - breakpoints and guidance v13.0. Available online at: https://www.eucast.org/clinical_breakpoints
Avolio, M., Vignaroli, C., Crapis, M., Camporese, A. (2017). Co-production of NDM-1 and OXA-232 by ST16 klebsiella pneumoniae, Italy, 2016. Future Microbiol. 12, 1119–1122. doi: 10.2217/fmb-2017-0041
Baroud, M., Dandache, I., Araj, G. F., Wakim, R., Kanj, S., Kanafani, Z., et al. (2013). Underlying mechanisms of carbapenem resistance in extended-spectrum β-lactamase-producing Klebsiella pneumoniae and Escherichia coli isolates at a tertiary care centre in Lebanon: role of OXA-48 and NDM-1 carbapenemases. Int. J. Antimicrobial Agents 41, 75–79. doi: 10.1016/j.ijantimicag.2012.08.010
Beyrouthy, R., Robin, F., Dabboussi, F., Mallat, H., Hamze, M., Bonnet, R. (2014). Carbapenemase and virulence factors of Enterobacteriaceae in North Lebanon between 2008 and 2012: evolution via endemic spread of OXA-48. J. Antimicrob. Chemother. 69, 2699–2705. doi: 10.1093/jac/dku181
Bitar, I., Dagher, C., Araj, G., Tokajian, S. (2018). First report of an Escherichia coli from Lebanon carrying an OXA-181 carbapenemase resistance determinant. J. Global Antimicrobial Resistance 12, 113–114. doi: 10.1016/j.jgar.2018.01.002
Brisse, S., Fevre, C., Passet, V., Issenhuth-Jeanjean, S., Tournebize, R., Diancourt, L., et al. (2009). Virulent clones of Klebsiella pneumoniae: identification and evolutionary scenario based on genomic and phenotypic characterization. PLoS One 4, e4982. doi: 10.1371/journal.pone.0004982
Bruen, T. C., Philippe, H., Bryant, D. (2006). A simple and robust statistical test for detecting the presence of recombination. Genetics 172, 2665–2681. doi: 10.1534/genetics.105.048975
Camacho, C., Coulouris, G., Avagyan, V., Ma, N., Papadopoulos, J., Bealer, K., et al. (2009). BLAST+: architecture and applications. BMC Bioinf. 10, 421. doi: 10.1186/1471-2105-10-421
Carattoli, A., Zankari, E., Garcia-Fernandez, A., Voldby Larsen, M., Lund, O., Villa, L., et al. (2014). In silico detection and typing of plasmids using PlasmidFinder and plasmid multilocus sequence typing. Antimicrob. Agents Chemother. 58, 3895–3903. doi: 10.1128/AAC.02412-14
Chen, S., Zhou, Y., Chen, Y., Gu, J. (2018). fastp: an ultra-fast all-in-one FASTQ preprocessor. Bioinformatics 34, i884–i890. doi: 10.1093/bioinformatics/bty560
Chudejova, K., Kraftova, L., Mattioni Marchetti, V., Hrabak, J., Papagiannitsis, C. C., Bitar, I. (2021). Genetic plurality of OXA/NDM-encoding features characterized from enterobacterales recovered from Czech hospitals. Front. Microbiol. 12. doi: 10.3389/fmicb.2021.641415
Daaboul, D., Oueslati, S., Rima, M., Kassem, I. I., Mallat, H., Birer, A., et al. (2023). The emergence of carbapenemase-producing Enterobacterales in hospitals: a major challenge for a debilitated healthcare system in Lebanon. Front. Public Health 11. doi: 10.3389/fpubh.2023.1290912
Dandachi, I., Salem Sokhn, E., Najem, E., Azar, E., Daoud, Z. (2016). Carriage of beta-lactamase-producing Enterobacteriaceae among nursing home residents in north Lebanon. Int. J. Infect. Dis. 45, 24–31. doi: 10.1016/j.ijid.2016.02.007
Diancourt, L., Passet, V., Verhoef, J., Grimont, P. A., Brisse, S. (2005). Multilocus sequence typing of Klebsiella pneumoniae nosocomial isolates. J. Clin. Microbiol. 43, 4178–4182. doi: 10.1128/JCM.43.8.4178-4182.2005
Doi, Y., O'Hara, J. A., Lando, J. F., Querry, A. M., Townsend, B. M., Pasculle, A. W., et al. (2014). Co-production of NDM-1 and OXA-232 by klebsiella pneumoniae. Emerg. Infect. Dis. 20, 163–165. doi: 10.3201/eid2001.130904
Dortet, L., Poirel, L., Nordmann, P. (2014). Worldwide dissemination of the NDM-type carbapenemases in Gram-negative bacteria. Biomed Res Int. 2014 249856. doi: 10.1155/2014/249856
Edgar, R. C. (2004). MUSCLE: multiple sequence alignment with high accuracy and high throughput. Nucleic Acids Res. 32, 1792–1797. doi: 10.1093/nar/gkh340
Edward, E. A., Mohamed, N. M., Zakaria, A. S. (2022). Whole Genome Characterization of the High-Risk Clone ST383 Klebsiella pneumoniae with a Simultaneous Carriage of bla(CTX-M-14) on IncL/M Plasmid and bla(CTX-M-15) on Convergent IncHI1B/IncFIB Plasmid from Egypt. Microorganisms 10. doi: 10.3390/microorganisms10061097
El-Herte, R. I., Araj, G. F., Matar, G. M., Baroud, M., Kanafani, Z. A., Kanj, S. S. (2012). Detection of carbapenem-resistant Escherichia coli and Klebsiella pneumoniae producing NDM-1 in Lebanon. J. Infect. Dev. Ctries 6, 457–461. doi: 10.3855/jidc.2340
Florensa, A. F., Kaas, R. S., Clausen, P., Aytan-Aktug, D., Aarestrup, F. M. (2022). ResFinder - an open online resource for identification of antimicrobial resistance genes in next-generation sequencing data and prediction of phenotypes from genotypes. Microb. Genom 8. doi: 10.1099/mgen.0.000748
Gagaletsios, L. A., Papagiannitsis, C. C., Petinaki, E. (2022). Prevalence and analysis of CRISPR/Cas systems in Pseudomonas aeruginosa isolates from Greece. Mol. Genet. Genomics 297, 1767–1776. doi: 10.1007/s00438-022-01957-4
Gupta, N., Limbago, B. M., Patel, J. B., Kallen, A. J. (2011). Carbapenem-resistant Enterobacteriaceae: epidemiology and prevention. Clin. Infect. Dis. 53, 60–67. doi: 10.1093/cid/cir202
Haft, D. H., DiCuccio, M., Badretdin, A., Brover, V., Chetvernin, V., O'Neill, K., et al. (2018). RefSeq: an update on prokaryotic genome annotation and curation. Nucleic Acids Res. 46, D851–D860. doi: 10.1093/nar/gkx1068
Hornsey, M., Phee, L., Wareham, D. W. (2011). A novel variant, NDM-5, of the New Delhi metallo-beta-lactamase in a multidrug-resistant Escherichia coli ST648 isolate recovered from a patient in the United Kingdom. Antimicrob. Agents Chemother. 55, 5952–5954. doi: 10.1128/AAC.05108-11
Iskandar, K., Roques, C., Hallit, S., Husni-Samaha, R., Dirani, N., Rizk, R., et al. (2021). The healthcare costs of antimicrobial resistance in Lebanon: a multi-centre prospective cohort study from the payer perspective. BMC Infect. Dis. 21, 404. doi: 10.1186/s12879-021-06084-w
Kopotsa, K., Osei Sekyere, J., Mbelle, N. M. (2019). Plasmid evolution in carbapenemase-producing Enterobacteriaceae: a review. Ann. N Y Acad. Sci. 1457, 61–91. doi: 10.1111/nyas.14223
Lee, K., Lim, Y. S., Yong, D., Yum, J. H., Chong, Y. (2003). Evaluation of the Hodge test and the imipenem-EDTA double-disk synergy test for differentiating metallo-beta-lactamase-producing isolates of Pseudomonas spp. and Acinetobacter spp. J. Clin. Microbiol. 41, 4623–4629. doi: 10.1128/JCM.41.10.4623-4629.2003
Li, W., O'Neill, K. R., Haft, D. H., DiCuccio, M., Chetvernin, V., Badretdin, A., et al. (2021). RefSeq: expanding the Prokaryotic Genome Annotation Pipeline reach with protein family model curation. Nucleic Acids Res. 49, D1020–D1028. doi: 10.1093/nar/gkaa1105
Linkevicius, M., Bonnin, R. A., Alm, E., Svartstrom, O., Apfalter, P., Hartl, R., et al. (2023). Rapid cross-border emergence of NDM-5-producing Escherichia coli in the European Union/European Economic Area, 2012 to June 2022. Euro Surveill 28. doi: 10.2807/1560-7917.ES.2023.28.19.2300209
Liu, B., Zheng, D., Jin, Q., Chen, L., Yang, J. (2019). VFDB 2019: a comparative pathogenomic platform with an interactive web interface. Nucleic Acids Res. 47, D687–D692. doi: 10.1093/nar/gky1080
Lorenzin, G., Gona, F., Battaglia, S., Spitaleri, A., Saluzzo, F., Trovato, A., et al. (2022). Detection of NDM-1/5 and OXA-48 co-producing extensively drug-resistant hypervirulent Klebsiella pneumoniae in Northern Italy. J. Glob Antimicrob. Resist. 28, 146–150. doi: 10.1016/j.jgar.2022.01.001
Mairi, A., Pantel, A., Sotto, A., Lavigne, J. P., Touati, A. (2018). OXA-48-like carbapenemases producing Enterobacteriaceae in different niches. Eur. J. Clin. Microbiol. Infect. Dis. 37, 587–604. doi: 10.1007/s10096-017-3112-7
Matar, G. M., Cuzon, G., Araj, G. F., Naas, T., Corkill, J., Kattar, M. M., et al. (2008). Oxacillinase-mediated resistance to carbapenems in Klebsiella pneumoniae from Lebanon. Clin. Microbiol. Infection 14, 887–888. doi: 10.1111/j.1469-0691.2008.02059.x
Nawfal Dagher, T., Azar, E., Al-Bayssari, C., Chamieh, A. S., Rolain, J. M. (2019). First detection of colistin-resistant klebsiella pneumoniae in association with NDM-5 carbapenemase isolated from clinical lebanese patients. Microb. Drug Resist. 25, 925–930. doi: 10.1089/mdr.2018.0383
Nordmann, P., Poirel, L. (2014). The difficult-to-control spread of carbapenemase producers among Enterobacteriaceae worldwide. Clin. Microbiol. Infect. 20, 821–830. doi: 10.1111/1469-0691.12719
Nordmann, P., Poirel, L., Walsh, T. R., Livermore, D. M. (2011). The emerging NDM carbapenemases. Trends Microbiol. 19, 588–595. doi: 10.1016/j.tim.2011.09.005
Otlu, B., Yakupogullari, Y., Gursoy, N. C., Duman, Y., Bayindir, Y., Tekerekoglu, M. S., et al. (2018). [Co-production of OXA-48 and NDM-1 Carbapenemases in Providencia rettgeri: the first report]. Mikrobiyol Bul 52, 300–307. doi: 10.5578/mb.67153
Papagiannitsis, C. C., Giakkoupi, P., Vatopoulos, A. C., Tryfinopoulou, K., Miriagou, V., Tzouvelekis, L. S. (2010). Emergence of Klebsiella pneumoniae of a novel sequence type (ST383) producing VIM-4, KPC-2 and CMY-4 beta-lactamases. Int. J. Antimicrob. Agents 36, 573–574. doi: 10.1016/j.ijantimicag.2010.07.018
Papagiannitsis, C. C., Izdebski, R., Baraniak, A., Fiett, J., Herda, M., Hrabak, J., et al. (2015). Survey of metallo-beta-lactamase-producing Enterobacteriaceae colonizing patients in European ICUs and rehabilitation units, 2008–11. J. Antimicrob. Chemother. 70, 1981–1988. doi: 10.1093/jac/dkv055
Paskova, V., Medvecky, M., Skalova, A., Chudejova, K., Bitar, I., Jakubu, V., et al. (2018). Characterization of NDM-encoding plasmids from enterobacteriaceae recovered from czech hospitals. Front. Microbiol. 9. doi: 10.3389/fmicb.2018.01549
Poirel, L., Bonnin, R. A., Nordmann, P. (2012a). Genetic features of the widespread plasmid coding for the carbapenemase OXA-48. Antimicrob. Agents Chemother. 56, 559–562. doi: 10.1128/AAC.05289-11
Poirel, L., Heritier, C., Tolun, V., Nordmann, P. (2004). Emergence of oxacillinase-mediated resistance to imipenem in Klebsiella pneumoniae. Antimicrob. Agents Chemother. 48, 15–22. doi: 10.1128/AAC.48.1.15-22.2004
Poirel, L., Potron, A., Nordmann, P. (2012b). OXA-48-like carbapenemases: the phantom menace. J. Antimicrob. Chemother. 67, 1597–1606. doi: 10.1093/jac/dks121
Price, M. N., Dehal, P. S., Arkin, A. P. (2010). FastTree 2–approximately maximum-likelihood trees for large alignments. PLoS One 5, e9490. doi: 10.1371/journal.pone.0009490
Prjibelski, A., Antipov, D., Meleshko, D., Lapidus, A., Korobeynikov, A. (2020). Using SPAdes de novo assembler. Curr. Protoc. Bioinf. 70, e102. doi: 10.1002/cpbi.102
Rima, M., Oueslati, S., Dabos, L., Daaboul, D., Mallat, H., Bou Raad, E., et al. (2022). Prevalence and molecular mechanisms of carbapenem resistance among gram-negative bacilli in three hospitals of Northern Lebanon. Antibiotics (Basel) 11. doi: 10.3390/antibiotics11101295
Rojas, L. J., Hujer, A. M., Rudin, S. D., Wright, M. S., Domitrovic, T. N., Marshall, S. H., et al. (2017). NDM-5 and OXA-181 beta-lactamases, a significant threat continues to spread in the americas. Antimicrob. Agents Chemother. 61. doi: 10.1128/AAC.00454-17
Rotova, V., Papagiannitsis, C. C., Skalova, A., Chudejova, K., Hrabak, J. (2017). Comparison of imipenem and meropenem antibiotics for the MALDI-TOF MS detection of carbapenemase activity. J Microbiol Methods. 137, 30–33. doi: 10.1016/j.mimet.2017.04.003
Seeman, T. (2015) snippy: fast bacterial variant calling from NGS reads. Available online at: https://github.com/tseemann/snippy.
Seemann, T. (2014). Prokka: rapid prokaryotic genome annotation. Bioinformatics 30, 2068–2069. doi: 10.1093/bioinformatics/btu153
Siguier, P., Perochon, J., Lestrade, L., Mahillon, J., Chandler, M. (2006). ISfinder: the reference centre for bacterial insertion sequences. Nucleic Acids Res. 34, D32–D36. doi: 10.1093/nar/gkj014
Simner, P. J., Bergman, Y., Conzemius, R., Jacobs, E., Tekle, T., Beisken, S., et al. (2023). An NDM-producing escherichia coli clinical isolate exhibiting resistance to cefiderocol and the combination of ceftazidime-avibactam and aztreonam: another step toward pan-beta-lactam resistance. Open Forum Infect. Dis. 10, ofad276. doi: 10.1093/ofid/ofad276
Sobh, G. M., El Karaaoui, A. K., El Chaar, M., Araj, G. F. (2021). Emerging ceftazidime-avibactam resistance against carbapenem resistant Escherichia coli and Klebsiella pneumoniae in Lebanon. Int. Arabic J. Antimicrobial Agents. 10, 2–3. doi: 10.3823/858
Solgi, H., Nematzadeh, S., Giske, C. G., Badmasti, F., Westerlund, F., Lin, Y. L., et al. (2020). Molecular epidemiology of OXA-48 and NDM-1 producing enterobacterales species at a university hospital in tehran, Iran, between 2015 and 2016. Front. Microbiol. 11. doi: 10.3389/fmicb.2020.00936
Sullivan, M. J., Petty, N. K., Beatson, S. A. (2011). Easyfig: a genome comparison visualizer. Bioinformatics 27, 1009–1010. doi: 10.1093/bioinformatics/btr039
Tao, G., Tan, H., Chen, Q. (2023). The First Report of Escherichia coli and Klebsiella pneumoniae Strains That Produce Both NDM-5 and OXA-181 in Jiangsu Province, China. Infect. Drug Resist. 16, 3245–3255. doi: 10.2147/IDR.S412678
Tatusova, T., DiCuccio, M., Badretdin, A., Chetvernin, V., Nawrocki, E. P., Zaslavsky, L., et al. (2016). NCBI prokaryotic genome annotation pipeline. Nucleic Acids Res. 44, 6614–6624. doi: 10.1093/nar/gkw569
Treangen, T. J., Ondov, B. D., Koren, S., Phillippy, A. M. (2014). The Harvest suite for rapid core-genome alignment and visualization of thousands of intraspecific microbial genomes. Genome Biol. 15, 524. doi: 10.1186/s13059-014-0524-x
Tsui, C. K., Ben Abid, F., Al Ismail, K., McElheny, C. L., Al Maslamani, M., Omrani, A. S., et al. (2023). Genomic epidemiology of carbapenem-resistant klebsiella in Qatar: emergence and dissemination of hypervirulent klebsiella pneumoniae sequence type 383 strains. Antimicrob. Agents Chemother. 67, e0003023. doi: 10.1128/aac.00030-23
Turton, J. F., Payne, Z., Coward, A., Hopkins, K. L., Turton, J. A., Doumith, M., et al. (2018). Virulence genes in isolates of Klebsiella pneumoniae from the UK during 2016, including among carbapenemase gene-positive hypervirulent K1-ST23 and 'non-hypervirulent' types ST147, ST15 and ST383. J. Med. Microbiol. 67, 118–128. doi: 10.1099/jmm.0.000653
van Duin, D., Doi, Y. (2017). The global epidemiology of carbapenemase-producing Enterobacteriaceae. Virulence 8, 460–469. doi: 10.1080/21505594.2016.1222343
Wirth, T., Falush, D., Lan, R., Colles, F., Mensa, P., Wieler, L. H., et al. (2006). Sex and virulence in Escherichia coli: an evolutionary perspective. Mol. Microbiol. 60, 1136–1151. doi: 10.1111/j.1365-2958.2006.05172.x
Wu, W., Feng, Y., Tang, G., Qiao, F., McNally, A., Zong, Z. (2019). NDM metallo-beta-lactamases and their bacterial producers in health care settings. Clin. Microbiol. Rev. 32. doi: 10.1128/CMR.00115–18
Ye, Y., Xu, L., Han, Y., Chen, Z., Liu, C., Ming, L. (2018). Mechanism for carbapenem resistance of clinical Enterobacteriaceae isolates. Exp. Ther. Med. 15, 1143–1149. doi: 10.3892/etm.2017.5485
Keywords: Escherichia coli, Klebsiella pneumoniae, ST383, blaNDM-5, carbapenem resistance
Citation: Sobh G, Araj GF, Finianos M, Sourenian T, Hrabak J, Papagiannitsis CC, Chaar ME and Bitar I (2024) Molecular characterization of carbapenem and ceftazidime-avibactam-resistant Enterobacterales and horizontal spread of blaNDM-5 gene at a Lebanese medical center. Front. Cell. Infect. Microbiol. 14:1407246. doi: 10.3389/fcimb.2024.1407246
Received: 26 March 2024; Accepted: 28 May 2024;
Published: 19 June 2024.
Edited by:
Laura Fernandez-Garcia, Institute of Biomedical Research of A Coruña (INIBIC), SpainReviewed by:
Alberto Antonelli, University of Florence, ItalyCemal Sandalli, Recep Tayyip Erdoğan University, Türkiye
Copyright © 2024 Sobh, Araj, Finianos, Sourenian, Hrabak, Papagiannitsis, Chaar and Bitar. This is an open-access article distributed under the terms of the Creative Commons Attribution License (CC BY). The use, distribution or reproduction in other forums is permitted, provided the original author(s) and the copyright owner(s) are credited and that the original publication in this journal is cited, in accordance with accepted academic practice. No use, distribution or reproduction is permitted which does not comply with these terms.
*Correspondence: Marc Finianos, bWFyYy5maW5pYW5vc0BsaXZlLmNvbQ==; George F. Araj, Z2FyYWpAYXViLmVkdS5sYg==