- 1Department of Parasitology, School of Basic Medical Science, Central South University, Changsha, China
- 2Human Microbiome and Health Group, Department of Microbiology, School of Basic Medical Science, Central South University, Changsha, China
- 3Department of General Surgery, Xiangya Hospital, Central South University, Changsha, China
Schistosoma japonicum causes serious pathological organ damage and alteration of the intestinal microbiome in the mammalian host, threatening the health of millions of people in China. Bacillus amyloliquefaciens has been reported to be able to alleviate the damage to the gut and liver and maintain the homeostasis of the intestinal microenvironment. However, it was unclear whether B. amyloliquefaciens could alleviate the hepatic and intestinal symptoms caused by S. japonicum. In this study, the intragastric administration of B. amyloliquefaciens was performed to treat S. japonicum-infected mice during the acute phase. Histopathological analysis and 16S rRNA gene sequencing were used to evaluate the pathological damage and changes in the intestinal microbiome. The results of the study showed that B. amyloliquefaciens treatment significantly reduced the degree of granuloma and fibrosis in infected mice. Additionally, recovery of diversity in the intestinal microbiome, decrease in the relative abundance of potential pathogenic bacteria such as Escherichia–Shigella, and reshaping of the interactive network between genera in the intestine were also observed after treatment with B. amyloliquefaciens. Our findings indicated that treatment with B. amyloliquefaciens effectively alleviated the pathological injuries of the liver and intestine in mice infected with S. japonicum by modulating the intestinal microbiome, implying that this probiotic can function as an effective therapeutic agent against schistosomiasis. We hope our study will provide auxiliary strategies and methods for the early prevention of schistosomiasis japonica.
1 Introduction
Schistosomiasis is a zoonotic parasitic disease that seriously endangers human health. It has been an endemic in 78 countries and regions worldwide, threatening a population of 800 million (McManus et al., 2018). In China, schistosomiasis japonica was mainly prevalent in 12 provinces, municipalities, and autonomous regions in the middle and lower Yangtze River and its south regions (McManus et al., 2010). It has been reported in studies that Schistosoma japonicum matures into adults in the intrahepatic portal system and then migrates in pairs to mesenteric veins, where it copulates and lays eggs in the blood vessels of the intestinal wall. Therefore, diarrhea, fatigue, and anemia could be caused by schistosomiasis japonica in the early stage of infection and portal hypertension syndrome, ascites, and liver fibrosis in the late stage (Colley et al., 2014). It generally takes 23–35 days from the time of transcutaneous infection with cercariae to produce eggs by mating. Therefore, the exacerbation phase of schistosomiasis japonica would appear in 4–5 weeks, and this stage is called the acute phase of S. japonicum infection (Li et al., 2018). Studies revealed that mature eggs contain S. japonicum miracidium, which secretes cytolytic material that penetrates through the eggshell into the intestinal mucosa, damaging the vessel wall to form granulomas and breaking the surrounding intestinal mucosal tissue into necrosis (Barnett, 2018). It was found previously that due to intestinal peristalsis and an increase in intra-abdominal pressure with intravascular pressure, worm eggs with necrotic tissue fall into the intestinal lumen and get discharged with the feces. The symptoms of intestinal granuloma caused by S. japonicum infection are similar to those of inflammatory bowel disease (IBD), typically characterized by hematochezia and unformed stools (McManus et al., 2018).
It was observed that the bacterial community of the gut constitutes the largest microbiota in the human body, and its homeostasis and balance are essential for human health. Disturbance of the intestinal microbiota can easily cause diarrhea, IBD, and even increase the risk of colorectal cancer (CRC) (Choi et al., 2017). Previous studies have shown that alterations in the intestinal microenvironment caused by granulomas could disrupt the stability and composition of the bowel microbiome (Zhao Y. et al., 2019). Odoribacter and Lachnospiraceae UCG-001 have been observed to become more abundant after infection, and Alistipes might be potential biomarkers that could produce anti-inflammatory metabolites to promote the differentiation of anti-inflammatory Treg/Tr1 cells in the gut of mice (Dziarski et al., 2016; Li et al., 2016; Hu et al., 2020). Furthermore, studies reported that the composition of the intestinal microbiota was distinct during the acute and chronic phases of S. japonicum infection (Zhou et al., 2022). Previous researchers found that gut microbiota changes are capable of ameliorating the pathological conditions caused by S. japonicum. By depletion of the gut microbiome in infected mice by antibiotics and its cohousing with healthy mice, researchers discovered that microbiome changes in the gut of infected mice indeed attenuated intestinal granuloma formation as well as the fibrotic response and reduced inflammatory factor levels (Zhang et al., 2020). These findings illustrated that modification of the intestinal microbiome can effectively alleviate the symptoms of S. japonicum infection, indicating a close relationship between S. japonicum infection and the host intestinal microbiome.
It has been stated in previous studies that praziquantel has been used clinically as the first-choice drug for the prevention and treatment of schistosomiasis japonica (Yahya et al., 2023), but despite being a broad-spectrum anti-helminth drug, it faced several challenges, including high side effects, low solubility, low efficacy against S. japonicum juveniles, and resistance development in S. japonicum caused by long-term and massive use (Li et al., 2011). Therefore, the development of new anti-schistosomal drugs and the implementation of more effective means of prevention and treatment appear to be extremely important and urgent. Probiotics could maintain the balance of the intestinal microbiota and possess anti-inflammatory and anti-cancerous activity, defined by the World Health Organization as living microorganisms that benefit the health of the host when administered in adequate amounts (Guarino et al., 2015). At present, probiotics are employed clinically as a therapeutic approach to prevent intestinal diseases. Through early prevention and treatment with probiotics, the symptoms of intestinal diseases and the disorders of the microbiota can be alleviated, and the homeostasis of the gut microbiome can be inextricably improved and optimized to maintain a normal state of function (Sanders et al., 2011). Intervention with probiotics in the treatment of schistosomiasis japonica is a therapeutic advancement. It has been proven by earlier studies that gavage of probiotics effectively alleviates the symptoms of S. japonicum infection, as Bacillus subtilis reduces pathological damage and regulates gene expression in the host mice (Lin et al., 2021).
Bacillus amyloliquefaciens, a bacterium with probiotic properties, has been introduced to livestock feed on an industrial level. It was added to reduce poultry mortality by reducing the impact of pathogenic bacteria on their gut (Abriouel et al., 2011). Recently, it was found that the number of B. amyloliquefaciens in dog intestines was significantly increased, whereas Escherichia coli decreased significantly after gavage with B. amyloliquefaciens, indicating that B. amyloliquefaciens has intestinal adhesive and antimicrobial activity (Vazquez-Mendoza et al., 2018). Moreover, B. amyloliquefaciens was found to ameliorate pathological damage in mice fed a high fiber diet by reducing the degree of obesity and inflammation as well as alleviating liver injury (Wang et al., 2019). In addition, B. amyloliquefaciens treatment was reported to promote the clearance of E. coli in RAW 264.7 cells by activating autophagy and to produce anti-inflammatory effects by reducing the level of JNK phosphorylation caused by E. coli (Wu et al., 2017). In general, B. amyloliquefaciens can modulate the homeostasis of the intestinal microbiota, reduce the level of intestinal inflammation, and reduce the degree of liver injury. However, it was unclear whether B. amyloliquefaciens could alleviate the symptoms caused by S. japonicum infection.
In this study, we performed intragastric administration of B. amyloliquefaciens to treat mice acutely infected with S. japonicum and then evaluated its effects on hepatic and intestinal injuries. Additionally, 16S rRNA gene sequencing was conducted to analyze the changes in gut microbiota in infected mice post treatment with B. amyloliquefaciens. Our work demonstrated that treatment with B. amyloliquefaciens during the acute phase could alleviate the pathological injuries in mice infected with S. japonicum. We aimed to provide an effective agent for the early prevention and treatment of S. japonicum infections.
2 Methods
2.1 Preparation for suspensions of B. amyloliquefaciens
B. amyloliquefaciens was isolated from healthy human feces and identified by using full-length primers of 16S rRNA through NCBI-Blast. Approximately 0.5 g of human feces were diluted in a 40 ml PBS solution, followed by water bathing at 80°C for 20 min, and then left to cool down at room temperature. Next, 100 μl of the supernatant was aspirated into lysogeny broth (LB) plates and spread, followed by incubation at 37°C. Subsequently, single colonies were picked according to the bacterial morphology and streaked for passaging. Single colonies were isolated after three passages of culture. The extraction of single-colony DNA was performed as per the protocol of the universal genomic DNA kit (Cwbio, Jiangsu, China). Afterward, the full-length 16S rRNA gene was amplified by polymerase chain reaction (PCR) using the forward primers 27F (5’-AGAGTTTGATCMTGGCTCAG-3’) and reverse primers 1492R (5’-GGTTACCTTGTTACGACTT-3’) and sequenced by first-generation sequencing. Finally, B. amyloliquefaciens was identified by NCBI-Blast. Moreover, B. amyloliquefaciens was stored at −80 °C for further culturing.
One day before intragastric administration, B. amyloliquefaciens was activated and cultured in LB medium at 37 °C and 200 rpm in the constant-temperature shaker until its OD600 reached 0.8–1.0. Intragastric administration was performed on each mouse with a 0.3-ml suspension of B. amyloliquefaciens every 3 days at a fixed time. The bacterial suspension was refreshed every time before intragastric administration.
2.2 Mice infection and intragastric administration of B. amyloliquefaciens
A total of 28 female pathogen-free BALB/c mice (approximately 5 weeks old) were purchased from Hunan Sleek Jingda (SLAC), Changsha, China. All mice were settled in plastic cages with metal fence covers. The environmental conditions were sterile, with free access to standard rat food and drinking water under controlled temperature (25 ± 5°C), humidity (60%–70%), and light (12/12-hour light/dark cycle). After adaptive feeding, mice (approximately 7 weeks old, body weight: 20 ± 2 g) were separated into four groups randomly: PBS, healthy mice with intragastric administration of PBS (phosphate buffered saline); BA, healthy mice with intragastric administration of B. amyloliquefaciens; SJ, S. japonicum-infected mice with intragastric administration of PBS; and SJBA, S. japonicum infected mice with intragastric administration of B. amyloliquefaciens. Each group contained seven mice. Based on the design of the experiment (Figure 1A), mice in the SJ and SJBA groups were infected with 27 ± 3 S. japonicum cercariae via shaved abdominal skin. The PBS and BA groups served as controls without any treatment for infection. All the mice were returned to original environment to house after infection. Intragastric administration of PBS and B. amyloliquefaciens started one week before infection and was conducted according to the design of the experiment (Figure 1A) after infection.
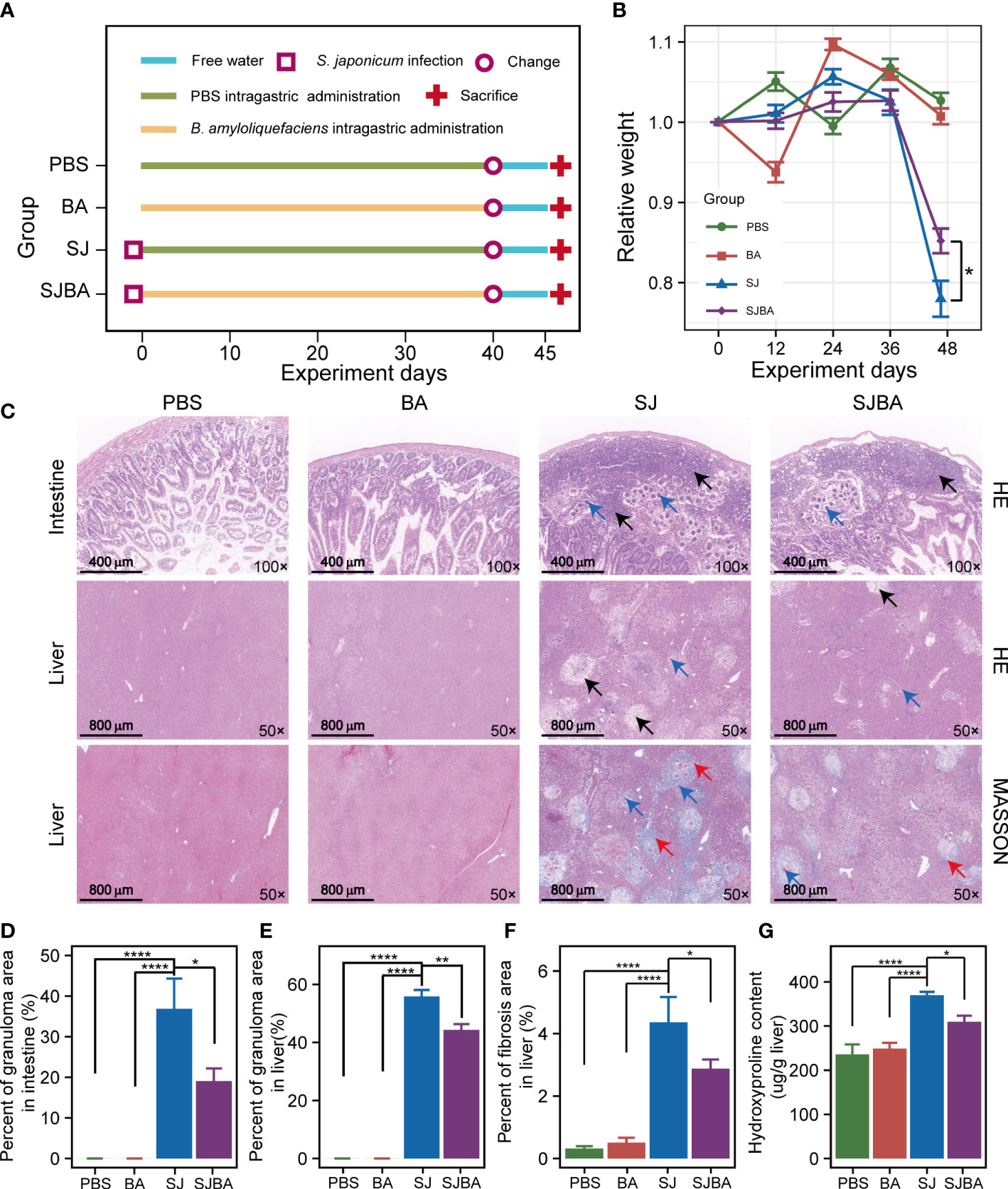
Figure 1 Treatment of B. amyloliquefaciens alleviated S. japonicu-induced hepatic and intestinal granulomas in mice. (A) Experimental design schedule for the study. (B) Changes in relative body weight among the four groups. Seven mice were included from each group for calculation on days 0, 12, 24, and 36. Meanwhile, seven mice in the PBS group, seven mice in the BA group, four mice in the SJ group, and five mice in the SJBA group were included in the calculation on the 45th day. Data were analyzed by one-way analysis of variance (ANOVA) and Fisher’s least significant difference (LSD) multiple comparison posttest. (C) H&E staining and Masson’s trichrome staining of the small intestine and liver. The numbers in the lower right represent the magnification of images. The black arrows indicated granulomas. The red arrows indicated fibrosis. Blue arrows indicated eggs of S. japonicum. (D) The percentage of granuloma area in the small intestine. (E) The percentage of granuloma area in the liver. The data were analyzed by the Kruskal–Wallis test. (F) The percentage of fibrosis area in the liver. The data were analyzed by one-way ANOVA and LSD’s multiple comparison posttest. (G) The hydroxyproline content in the liver. Data were analyzed by one-way ANOVA and LSD’s multiple comparison posttest. *P <0.05; **P <0.01; ****P <0.0001.
2.3 Sample collection
The body weight of all mice was measured once every 12 days. Fecal samples of all mice were collected once every 12 days and stored at −80 °C for further analysis. On day 45 after infection, all mice were sacrificed by chloral hydrate asphyxiation and cervical dislocation. The collection of fecal samples and the measurement of weight were conducted one day before sacrifice. Whole liver and spleen samples were collected to measure weight. The left liver lobes and small intestines were fixed in 4% paraformaldehyde for histopathological analysis. The remaining liver samples were assayed for hydroxyproline content by following the protocol of the hydroxyproline content assay kit (Solarbio, Beijing, China).
2.4 Histological staining
The samples of livers and intestines were used for histopathological analysis. Each group contained three livers and three small intestines for analysis. The slices of livers and intestines were subjected to H&E (hematoxylin–eosin) staining and Masson’s trichrome staining. Images of slices were captured and scanned by a Pannoramic panoramic slide scanner (3DHISTECH, Hungary). The whole tissue area and the blue-positive region were analyzed using Image-Pro Plus 6.0 software (Media Cybernetics, USA) and CaseViewer 2.4 software (3DHISTECH, Hungary). The percentage of the fibrotic area was calculated based on the area of the blue-labeled region/the total area of the whole liver tissue. Granulomatous responses in the intestine and liver were estimated by the ratio of granulomas to the area of the whole tissue.
2.5 DNA extraction and high-throughput sequencing of 16S rRNA
Three samples from each group at different sampling time points were selected for 16S rRNA sequencing. Total genomic DNA from fecal samples was extracted by following the protocol of the OMEGA Soil DNA Kit (M5636-02) (Omega Bio-Tek, Norcross, GA, USA) and stored at −20°C prior to further analysis. The quantity and quality of extracted DNA were measured using the NanoDrop NC2000 spectrophotometer (Thermo Fisher Scientfic, Waltham, MA, USA) and agarose gel electrophoresis, respectively. The V3–V4 region of the 16S rRNA gene was subsequently amplified by polymerase chain reaction (PCR) using the forward primers 338F (5’-ACTCCTACGGGAGGCAGCA-3’) and reverse primers 806R (5’-GGACTACHVGGGTWTCTAAT-3’). The qualified libraries were then sequenced on the Illumina NovaSeq platform with the NovaSeq-PE250 sequencing strategy at Shanghai Personal Biotechnology Co., Ltd. (Shanghai, China). After sequencing, the data was decomposed into appropriate samples based on barcodes, and the appropriate sequences were imported into downstream software. Quality control and denoising of raw reads were performed based on the standard amplicon pipeline as described previously (Liu et al., 2021). The feature table and taxonomy annotation table were used for further data analysis.
2.6 Data analysis
All statistical analysis was performed using R (version 4.2.1) (R Core Team, 2022). The statistical results were visualized by the “ggplot2” package unless specified otherwise (Wickham, 2016). Shannon index and beta diversity were calculated by the “vegan” package (Oksanen J et al., 2022). The survival curves of mice were performed by packages of ‘survival’ and ‘survminer’ (Therneau and Grambsch, 2000; Alboukadel Kassambara and Przemyslaw, 2021). The analysis of constrained principal coordinate analysis (CPCoA) based on Bray–Curtis distance was performed by the “amplicon” package (Liu Y and Chen, 2021). In addition, genera with significant changes in relative abundance were discovered by Linear Discriminant Analysis (LDA) Effect Size (LEfSe) (http://huttenhower.sph.harvard.edu/galaxy/) (Galaxy, 2022). The threshold of significance was set at 0.05, while the threshold of the LDA score was set at 3.5. The analysis of Spearman correlation for genera with significant changes in relative abundance was calculated by the “Hmisc” package (F, 2022) and visualized by the “pheatmap” package (Kolde, 2019). The network showed that interactions between genera were established based on Spearman correlation analysis by the “igraph” package (Gabor Csardi, 2016) and Gephi software (version 0.10.0) (Bastian M. and Jacomy, 2009). The threshold of the P-value corrected by the Benjamin and Hochberg false discovery rate (FDR) was 0.05.
3 Results
3.1 B. amyloliquefaciens treatment significantly alleviates the pathological injuries in mice infected with S. japonicum
To determine the effects of B. amyloliquefaciens on S. japonicum-infected mice, the experiment was designed on schedule for parasitic infection (Figure 1A). It was reported that loss of body weight in mice is a common feature of S. japonicum infection. Therefore, the changes in relative weight of the mice were recorded and analyzed during the experiment. The results demonstrated that there was no significant difference in the relative weight of mice among the four groups within 36 days post-infection, but it decreased dramatically after 36 days in the SJ and SJBA groups compared to the PBS and BA groups, indicating that infected mice began to show obvious pathological responses after 36 days in this experiment (Figure 1B). Although the weight loss of mice caused by S. japonicum infection is dramatic, even below 20%, we found that treatment with B. amyloliquefaciens significantly prevented weight reduction. At the same time, we found that the intervention of B. amyloliquefaciens also reduced the mortality of infected mice (Supplementary Figure S1). Visceral enlargement and fibrosis are typical pathological injuries in schistosomiasis japonica. Here, we found that the liver and spleen of mice in the PBS and BA groups appeared pink and soft in texture but became enlarged, black, and brittle at 45 days post-infection with S. japonicum (Supplementary Figure S2A). A sharp increase in the weight of the liver and spleen has been observed in the SJ group, which significantly decreased after B. amyloliquefaciens intervention (Supplementary Figures S2B, C).
In addition, histopathological analysis was performed to assess the degree of fibrosis and granulomas. According to the results of H&E staining, we found that eggs of S. japonicum and granulomas were found in the submucosa of the SJ and SJBA groups, affecting the integrity of the mucosa compared to the small intestine of the PBS and BA groups (Figure 1C). But the percentage of granulomatous area significantly declined both in the liver and small intestine in the SJBA group after treatment with B. amyloliquefaciens (Figures 1D, E). In the results of Masson’s trichrome staining, the percentage of fibrosis area in the liver decreased significantly in the SJBA group (Figure 1F). Subsequently, we found the content of hydroxyproline, which is an index of liver fibrosis, decreased significantly in S. japonicum-infected mice after treatment with B. amyloliquefaciens (Figure 1G). These results indicated that the use of B. amyloliquefaciens as a treatment can reduce S. japonicum-induced hepatic and intestinal granulomas and fibrosis, thereby protecting mice from infection.
3.2 B. amyloliquefaciens treatment modulates the dynamic changes of diversity and bacterial composition of gut microbiota
To evaluate the homeostasis and composition of the bacterial community, we analyzed the dynamic changes in diversity and bacterial composition in the gut of mice among four groups. Alpha diversity was presented by the Shannon index, and we found that the trend of the Shannon index was the same as the changes in relative body weight. There was no significant difference before 36 days post-infection within the four groups, but it declined dramatically on day 45 in the SJ group (Figure 2A). Furthermore, the results obtained from differential analysis by Shannon index on day 45 showed that infection with S. japonicum caused a sharp decline in Shannon index, which can be reversed by treatment with B. amyloliquefaciens (Supplementary Figure S3A). To clarify the variations among samples following the experimental time, we performed CPCoA analysis based on the Bray–Curtis distance. We found that the distance between each two samples at every time point became larger, and the distance between each time point was also significantly distinguished (Figure 2B). The results from CPCoA analysis on day 45 showed that PBS and BA groups were closer in distance, and S. japonicum infection led to a larger distance between PBS and BA groups, while administration of B. amyloliquefaciens resulted in a larger distance compared to the SJ group. (Supplementary Figure S3B). The Bray–Curtis distance between each time point and day 0 was calculated, and a linear fit was performed. Our study observed that the distance increased dramatically with the passage of time in the SJ group, while the SJBA group showed stable trends at the end of the experimental day (Supplementary Figure S3C). These results indicated that the intervention of B. amyloliquefaciens reshapes the composition of the bacterial community and helps reduce variations among samples after S. japonicum infection.
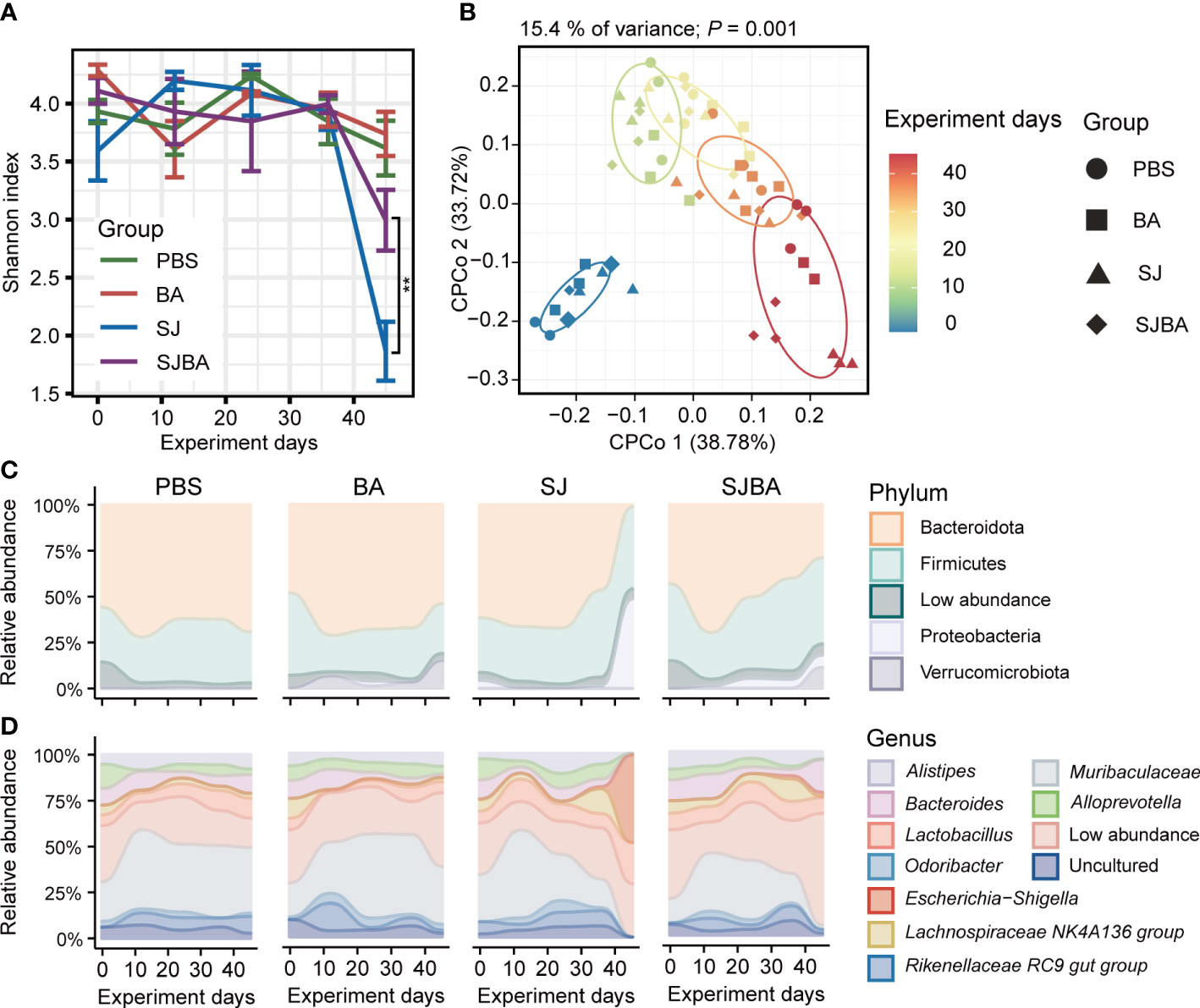
Figure 2 Treatment of B. amyloliquefaciens altered the diversity and composition of the gut microbiota of S. japonicum-infected mice. (A) The changes in the Shannon index with the progress of the experiment in four groups. (B) Analysis of CPCoA among the four groups. The color bar represented experimental days. Different shapes distinguished the four groups. (C) The changes in relative abundance of phyla in four groups during the experiment. (D) The changes in relative abundance of genera in four groups during the experiment. The X-axis represented the experimental days. The Y-axis represents the relative abundance of different phyla or genera. Modules in different colors separated different phyla or genus. “Low abundance” represents a genus in low relative abundance that cannot be clearly shown in the figure.
To elucidate how the treatment with B. amyloliquefaciens influenced the composition of the bacterial community in S. japonicum-infected mice, we analyzed the composition of the microbiome at the levels of phylum and genus. Significant changes were observed in bacterial composition after 36 days of infection but not before this period. Contrary to the PBS and BA groups, on day 45, the relative abundance of the phylum Bacteroidota dropped vividly, while the dominance of Proteobacteria displayed a significant rise in the SJ group, but this tends to return to normal levels in the SJBA group (Figure 2C). By compositional analysis at the level of genera, we observed an obvious increase of up to 50% in the relative abundance of Escherichia–Shigella in the SJ group and a clear decrease in Muribaculaceae to almost 0% on day 45 in the SJ group. Meanwhile, in the SJBA group, the relative abundance of Escherichia–Shigella dropped to the same level as the PBS and BA groups, but the abundance of Bacteroides increased (Figure 2D).
3.3 The relative abundance of Muribaculaceae and Escherichia–Shigella changed most significantly during exacerbations of acute phase
As indicated by the above results, day 36 proved to be a key time point for the progression of schistosomiasis japonica. Nearly all the pathological responses and changes in the homeostasis of the bacterial community worsened between days 36 and 45 post-infection. Thus, LEfSe analysis was performed to explain which bacteria had significant changes in their relative abundance from levels of phylum to genus between days 36 and 45. The threshold of adjusted P and LDA score was 0.05 and 3.5. The findings demonstrated that the phyla Bacteroidota and Proteobacteria were the most significantly altered ones. (Figure 3B). At the genus level, Escherichia–Shigella, Clostridium sensu stricto 1, Proteus, and Gemella had significant increases in their relative abundance in the SJ group. Bacteroides was significant in the SJBA group with increased relative abundance (Supplementary Figure S4). Meanwhile, Muribaculaceae, Marvinbryantia, and Rikenellaceae RC9 gut groups were significantly associated with the PBS group, and Akkermansia, Alloprevotella, Odoribacter, and Clostridia UCG 014 were significantly associated with the BA group (Figure 3B). The relative abundance of all the genera related to the PBS and BA groups dropped almost to 0% in the SJ group but slightly resurged in the SJBA group (Supplementary Figure S4). In terms of relative abundance, Muribaculaceae and Escherichia–Shigella were the most altered genera. The cladogram showed the most relevant clades among groups, which was in accordance with the above-mentioned results (Figure 3A).
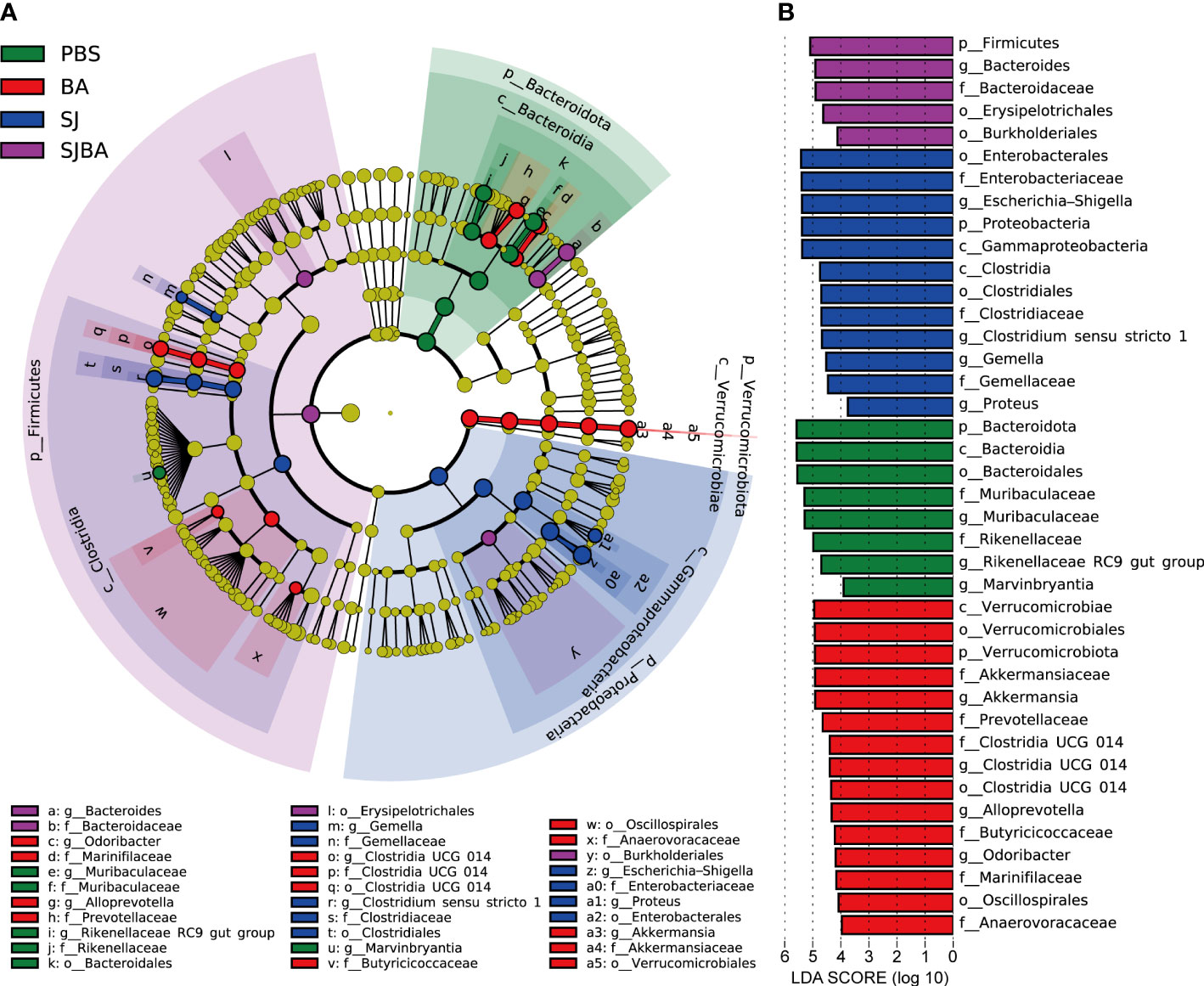
Figure 3 Genera with significant changes in the relative abundance in the gut of S. japonicum-infected mice after treatment with B. amyloliquefaciens. (A) A cladogram showing the discriminated taxa in different groups. Regions with different colors represented different groups. Nodes in different colors represented significant changes in relative abundance in different groups. The tellow nodes indicated no significant changes in the corresponding group. (B) A histogram with linear discriminant analysis (LDA) scores in four groups. Taxa highlighted in different colors indicate overrepresentation in the corresponding groups. The threshold of significance is set at 0.05. The threshold of the LDA score is set at 3.5.
3.4 Intervention of B. amyloliquefaciens reshaped the interaction network between genera with significant changes in relative abundance
To illustrate the relationship and interaction of genera in terms of changes in relative abundance among groups, we performed Spearman correlation and network analysis. Genera included in the correlation analysis were selected by the LEFSe analysis (Figure 3). All genera had significant differences in relative abundance under the screening based on the set threshold (refer to Methods). Spearman correlation was used to calculate the correlation between the picked genera. Then, genera with significant correlations were picked out to reconstruct the correlation heatmap figure for easy interpretation by readers. In the meantime, those selected genera were also used to perform an analysis of the interaction network. As suggested by the results of Spearman correlation, the significant correlation between genera in the SJ and SJBA groups was notably more complex than in the PBS and BA groups (Supplementary Figure S5). In the PBS and BA groups, genera with significant correlations were relatively few, and the correlation network was simple (Figures 4A, B). In the SJ group, there was a significant positive correlation between Proteus, Escherichia–Shigella, and Clostridium sensu stricto 1. The same correlation was also shown between Clostridia UCG 014, Odoribacter, Rikenellaceae RC9 gut group, Muribaculaceae, Marvinbryantia, and Alloprevotella in the SJ group. It was noted that Muribaculaceae, Marvinbryantia, and Alloprevotella had a highly significant negative correlation with Proteus, Escherichia–Shigella, and Clostridium sensu stricto 1. Especially, Escherichia–Shigella exhibited significant negative correlations with other genera that were significantly associated with the PBS and BA groups (Figure 4C). After treatment with B. amyloliquefaciens, the original correlation network was altered. Muribaculaceae, Marvinbryantia, and Clostridia UCG 014 established an extremely positive correlation with each other. Proteus showed a negative correlation with Bacteroides and Clostridium sensu stricto 1 while presenting a positive correlation with Alloprevotella, Muribaculaceae, Marvinbryantia, and Clostridia UCG 014 (Figure 4D).
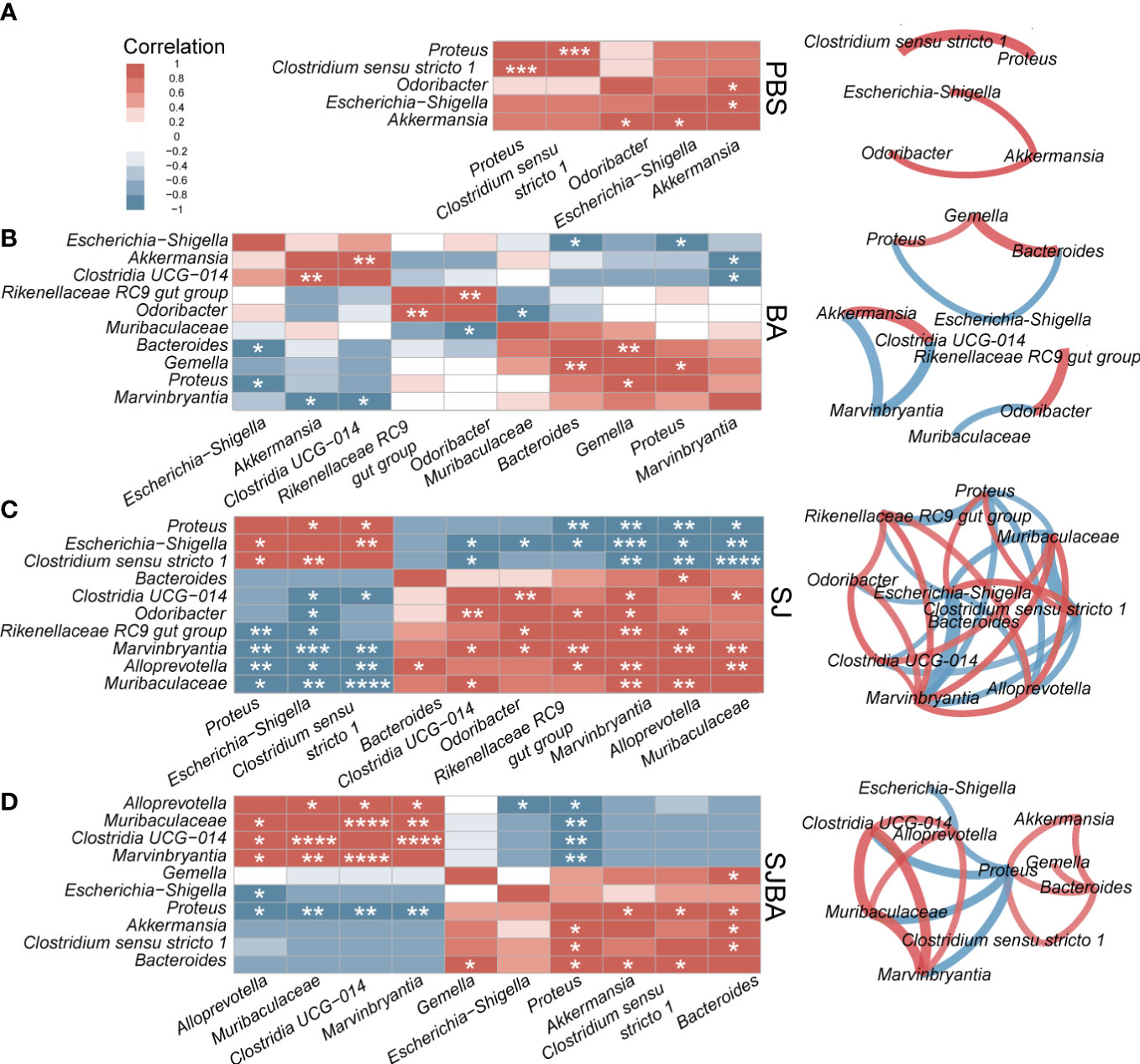
Figure 4 The analysis of correlation and network among the genera with significant differences in relative abundance. Correlation and network analysis among the genera with significant differences in relative abundance was performed among the groups of PBS (A), BA (B), SJ (C), and SJBA (D). Different colors represented positive or negative correlations. The intensity of the color represented the strength of the correlation. Edges in different colors between two genera represent the positive or negative correlation. The thickness of the edges represents the strength of the correlation. *P <0.05; **P <0.01; ***P <0.001; ****P <0.0001.
4 Discussion
S. japonicum, the only human blood fluke present in China, severely threatens the health of millions of people and affects socioeconomic development (Xue et al., 2022). It was stated earlier that organ edema, fibrosis of the liver, and granulomas in intestinal tissues could be caused by S. japonicum infection (Yang et al., 2021). Furthermore, the homeostasis and composition of the intestinal bacterial community were reported to be disrupted by alterations in the gut microenvironment caused by intestinal granulomas (Zhou et al., 2020). Studies suggested there was no target drug for treating schistosomiasis japonica except praziquantel, which is a broad-spectrum anti-helminthic drug (Wang et al., 2022). Previous studies suggested that changes in the gut microbiome reduce the symptoms caused by S. japonicum infection (Zhang et al., 2020). Therefore, we performed this study to evaluate whether intragastric administration of B. amyloliquefaciens could also alleviate the pathological injuries in mice infected with S. japonicum during the acute phase or not. Our results demonstrated that the employment of B. amyloliquefaciens was able to relieve S. japonicum-induced hepatic as well as intestinal granulomas and reshape the bacterial community in the intestine of S. japonicum-infected mice. Additionally, the diversity and composition of the intestinal microbiome were gradually returned to normal levels after treatment with B. amyloliquefaciens. Moreover, the relative abundance of Escherichia–Shigella was significantly reduced in the SJBA group, which was observed to be greatly elevated in the acute phase of S. japonicum infection. Furthermore, the interaction network among genera was also reshaped in the SJBA group, contrary to that in the SJ group.
Fibrosis of the liver and intestinal granulomas were the typical symptoms caused by the S. japonicum infection (Gunda et al., 2020). An earlier study stated that S. japonicum matures into an adult worm in the hepatic portal vein and subsequently migrates in pairs to the mesenteric veins to clasp and lay eggs. Eggs were regarded as one of the main pathogenic factors in schistosomiasis japonica (Burke et al., 2009). It was found that eggs of S. japonicum induce a granulomatous immune response in the host, which is largely characterized by lymphocytes, eosinophils, and alternatively activated macrophages (Pearce and MacDonald, 2002; Fairfax et al., 2012). By observing the pathological response in the acute phase of S. japonicum infection, weight loss can be significantly reversed after treatment with B. amyloliquefaciens. Furthermore, the degree of liver fibrosis as well as the granuloma area of the liver and small intestine were also observed to be significantly reduced after treatment with B. amyloliquefaciens. These results indicate that treatment with B. amyloliquefaciens can relieve the hepatic and intestinal fibrosis and granuloma in the acute phase of schistosomiasis japonica. B. amyloliquefaciens was widely used in food processing as a probiotic. Some of its primary and secondary metabolites, like lipopeptides, have anti-inflammatory and anticancerous effects, thus protecting the mucosal layer of the gut (WoldemariamYohannes et al., 2020). According to other studies, B. amyloliquefaciens SC06 could reduce the levels of JNK phosphorylation triggered by E. coil, thereby preventing acute inflammation (Wu et al., 2017; Wang et al., 2019). Therefore, there might be relationships between metabolites of B. amyloliquefaciens and host immune responses caused by granuloma, but further research is needed. Consequently, our results demonstrated that treatment with B. amyloliquefaciens can effectively attenuate the pathological damage caused by S. japonicum infection, indicating that it can act as a potential therapeutic agent for schistosomiasis, but further investigation is required.
The intestinal microbiome was found to have a close association with infection by S. japonicum. S. japonicum infection was found to cause dysbiosis of the gut microbiome, while modifications in the microbiota would alleviate the symptoms caused by S. japonicum infection (Zhang et al., 2020). Alpha diversity presented the number, category, and abundance of microbiomes within groups, and beta diversity such as Bray–Curtis distance refers to microbiota differences between samples or groups, which helped to understand whether differences in microbiota composition between two groups were significant (Qian et al., 2020). Our results showed that dysbiosis of gut microbiota caused by S. japonicum, manifested itself as rapid decline in alpha diversity and sharp increase in difference of bacterial composition in samples within groups, along with a steady rise and domination in the relative abundance of some phyla and genera, which were in accordance with the results of previous studies (Hu et al., 2020). By treating with B. amyloliquefaciens, the variation in alpha diversity, beta diversity, and bacterial composition were significantly improved. The results indicated that employment of B. amyloliquefaciens reshaped the intestinal ecology, improved the homeostasis of microbiome, and avoided the simplification of bacterial composition. Previous studies have shown that the intervention of B. amyloliquefaciens can reduce the levels of inflammatory factors and resume the dysbiosis of microbiota in the DSS-induced enteritis model (Cao et al., 2018). In addition, early colonization by B. amyloliquefaciens alleviated jejunal villus damage with reduced expression of proapoptotic genes in a study of enteritis caused by Clostridium perfringens (Jiang et al., 2021). Thus, combined with the results of histological staining, we found that there might be a potential correlation between amelioration of dysbiosis in microbiota and attenuation of pathological responses. The changes of intestinal microbiota due to treatment with B. amyloliquefaciens might be one of the reasons why schistosomiasis japonica was alleviated.
According to the results, the phenotype of mice from days 36 to 45 was completely different from that during the first 36 days, particularly manifested by a sudden drop in diversity of the microbiota and a trend toward simplification of bacterial composition. The analysis of LEfSe using samples between days 36 and 45 was performed to evaluate dysbiosis in the microbiota caused by S. japonicum infection. The characteristic of disturbance in the gut microbiota refers to changes in the composition of the microbiota associated with the host’s disease state (Ni et al., 2017). Our results showed that the infection of S. japonicum led to a significant increase in the relative abundance of Escherichia–Shigella, Clostridium sensu stricto 1, Proteus, and Gemella. Exclusively Escherichia–Shigella, rapidly increased to nearly 50% in relative abundance. Meanwhile, we observed a sharp decline in relative abundance of Muribaculaceae, Marvinbryantia, Rikenellaceae RC9 gut group, Akkermansia, Alloprevotella, Odoribacter, and Clostridia UCG 014, which fell to nearly 0% in the SJ group. Interestingly, the relative abundance of Escherichia–Shigella and Muribaculaceae was the most variable. Escherichia–Shigella has a strong association with IBD. As a potential biomarker of dysbiosis of the gut microbiome, it could stimulate the secretion of IL-6 and TNF-α, promoting inflammatory responses (Berry et al., 2015; Di Lorenzo et al., 2019). Therefore, a rise in the relative abundance of Escherichia–Shigella has a positive correlation with the disease severity in ulcerative colitis (UC) patients (Kojima et al., 2012). Muribaculaceae, a potentially beneficial bacterium, could modulate immune cells and decrease proinflammatory factor levels, and it has been proven to be a promising agent in alleviating and treating IBD (Qi et al., 2017; Mo et al., 2022; Niu et al., 2022). However, Clostridium sensu stricto 1, Proteus, and Gemella have been proven to have a role in accelerating the occurrence of IBD (Lopez-Dupla et al., 1996; FitzGerald et al., 2006; Garrett et al., 2010; Wen et al., 2021). Inclusively, Marvinbryantia, Rikenellaceae RC9 gut group, Akkermansia, Alloprevotella, Odoribacter, and Clostridia UCG 014 were all potential beneficial bacteria that could promote the synthesis of short-chain fatty acids (SCFAs) with the function of inhibiting the expression of inflammatory factors (Gao et al., 2022; Hu, 2022; Leibovitzh et al., 2022; Cai et al., 2023; Gao et al., 2023; Han et al., 2023). Therefore, the dramatic changes in Escherichia-Shigella and Muribaculaceae relative abundance might be one of the main reasons for deterioration and death from days 36 to 45. Moreover, a quantitative increase in Clostridium sensu stricto 1, Proteus, and Gemella and a decrease in potential beneficial bacteria boosted this trend. After treatment with B. amyloliquefaciens, the relative abundance of Escherichia–Shigella sharply dropped, accompanied by decrease in the relative abundance of other potentially harmful bacteria. Many studies have shown that B. amyloliquefaciens was able to produce bacteriocin-like inhibitory substances (BLIS), which had antimicrobial activity against pathogenic bacteria like Clostridium perfringens, E. coli and Yersinia (Abriouel et al., 2011). Therefore, BLIS might be an important factor that could cause a decrease in the relative abundance of pathogenic bacteria, thereby attenuating the pathological response in S. japonicum-infected mice. We found a notable increase in the relative abundance of Bacteroides in the SJBA group, which was known to significantly increase colonic inflammation in UC and modulate inflammatory responses by modulating Treg cell responses (Bloom et al., 2011; Zhao H. et al., 2019). Consequently, the elevated relative abundance of Bacteroides and the failure to reverse the relative abundance to a normal level in the case of beneficial bacteria might explain the reason for the death of infected mice even after treatment with B. amyloliquefaciens. The results from the analysis of LEfSe demonstrated that the relative abundance of genera significantly changed during the exacerbation phase. Meanwhile, the intervention of B. amyloliquefaciens reshapes the composition of the bacterial community and might reduce the relative abundance of potential pathogenic bacteria by secreting BLIS. These results indicated that B. amyloliquefaciens treatment could alleviate the pathological responses induced by S. japonicum infection by ameliorating the disturbance of the gut microbiota.
To elucidate the relationship among the genera with significant changes in relative abundance, we performed an analysis of correlation. In the PBS and BA groups, the interaction network among genera was relatively simple. Potential beneficial bacteria dominated, while potential pathogenic bacteria had a relatively low relative abundance, resulting in the stability of the intestinal microenvironment. In the SJ group, the interaction network became more complex. The relative abundance of Escherichia–Shigella, Clostridium sensu stricto 1, and Proteus increased with significant positive relationships among each other. Our results showed the sum of the relative abundances of the three potential harmful bacteria was close to 60%. Escherichia–Shigella had a significant negative correlation with almost all potential beneficial bacteria. Therefore, it was difficult to effectively inhibit pathogenic bacteria due to the low relative abundance of beneficial bacteria and the dominant position of Escherichia–Shigella. The intervention of B. amyloliquefaciens changed the interaction network relationships among the genera in the gut microbiota. Specifically, the positive correlation among potential pathogenic bacteria was destroyed with the decrease in the relative abundance of Escherichia–Shigella. Moreover, the relative abundance of all potential beneficial bacteria increased in positive correlation with each other. The results suggested that the symbiotic relationship among beneficial bacteria may be one of the key factors in alleviating the severity of disease in infected mice. It is worth noting that the relative abundance of Bacteroides also increased and maintained a significant positive correlation with other potential pathogenic bacteria except Escherichia–Shigella, which may be the reason of mice deaths even after receiving B. amyloliquefaciens as a treatment. Through our analysis, we found that the symbiotic and competitive relationship among bacteria with significant changes may affect the severity and outcome of symptoms in S. japonicum-infected mice. It has also been indicated that the intervention of B. amyloliquefaciens could destroy the mutually promoting relationship among potential pathogenic bacteria and promote the positive relationship among potential beneficial bacteria; eventually, it might improve the pathological reaction of S. japonicum-infected mice.
In general, treating mice with B. amyloliquefaciens could alleviate the pathological reaction in mice infected with S. japonicum. In addition, it also reshapes the intestinal microbiome to achieve a stable state by resuming the diversity of gut flora, reducing the relative abundance of Escherichia–Shigella, and intervening in the correlation between potential pathogenic genera and beneficial genera. But there were still some shortcomings in our study. Fibrosis and granulomas were the typical symptoms and lethal causes of schistosomiasis japonica. Our study explained the effectiveness of B. amyloliquefaciens intervention for alleviating schistosomiasis japonica based on the results of alterations in the gut microbiome, but mechanisms remained unexplained. In our future studies, we expect to explore the mechanisms of alleviating pathological responses. Multi-omics analysis techniques such as metagenomics and metabolomics will be used to analyze the effects of B. amyloliquefaciens intervention on the metabolic pathways and metabolites of the intestinal microbiome, observe the changes in expression of inflammatory factors through measurement, and elucidate how the intervention of B. amyloliquefaciens could alleviate pathological damage by modifying the intestinal microenvironment from the perspective of the gut–liver axis. The current treatment with probiotics has been widely used clinically to treat IBD, but it is rarely used in the treatment of schistosomiasis japonica. For regulating host immunity by means of killing pathogens and secreting metabolites, probiotics have great prospects for schistosomiasis control (Yadav et al., 2022).
In conclusion, our results indicated that treating and alleviating schistosomiasis japonica by using B. amyloliquefaciens may prove to be an effective method. The establishment of early colonization with probiotics can reduce the pathological symptoms in mice infected with S. japonicum. Meanwhile, it can reshape the intestinal microenvironment of infected mice by modulating the relative abundance of potentially beneficial and harmful bacteria as well as the network of interactive relationships of the intestinal microbiome. These results also indicated that there is a potential relationship between pathological damage and changes in the gut microenvironment. Our study provided potential auxiliary therapeutic strategies and means for the early prevention and treatment of schistosomiasis japonica.
Data availability statement
The data presented in the study are deposited in the Sequence Read Archive (https://www.ncbi.nlm.nih.gov/sra), under accession number PRJNA937169 (https://www.ncbi.nlm.nih.gov/bioproject/PRJNA937169/).
Ethics statement
The studies involving human participants were reviewed and approved by The IRB of School of Basic Medical Science of Central South University (No: 2021-KT75). The patients/participants provided their written informed consent to participate in this study.The animal study was reviewed and approved by The IRB of School of Basic Medical Science of Central South University (No: 2021-KT25).
Author contributions
JH and SH conceived the study. JW isolated and characterized B. amyloliquefaciens. HC and SY performed the experiments. HC and RS analyzed the data. HC and ZY visualized the statistical results. HC wrote the draft. HC, SH, and JH wrote and edited the final manuscript. SB improved the language and grammar of the manuscript. All authors contributed to the article and approved the submitted version.
Funding
This work was supported by the Natural Science Foundation of Hunan Province (2020JJ5700), the National Natural Science Foundation of China (82102428), and the Natural Science Foundation of Hunan Province (2022JJ40663).
Acknowledgments
We would like to convey our thanks to Professor Zuping Zhang for her instruction in identification of S. japonicum eggs, Miss Ying Xiao and Miss Qingqun Wang for assistance when sacrificing mice, and Miss Xuan Peng for help in data analyzing.
Conflict of interest
The authors declare that the research was conducted in the absence of any commercial or financial relationships that could be construed as a potential conflict of interest.
Publisher’s note
All claims expressed in this article are solely those of the authors and do not necessarily represent those of their affiliated organizations, or those of the publisher, the editors and the reviewers. Any product that may be evaluated in this article, or claim that may be made by its manufacturer, is not guaranteed or endorsed by the publisher.
Supplementary material
The Supplementary Material for this article can be found online at: https://www.frontiersin.org/articles/10.3389/fcimb.2023.1172298/full#supplementary-material
References
Abriouel, H., Franz, C. M., Ben Omar, N., Galvez, A. (2011). Diversity and applications of bacillus bacteriocins. FEMS Microbiol. Rev. 35 (1), 201–232. doi: 10.1111/j.1574-6976.2010.00244.x
Alboukadel Kassambara, M. K., Przemyslaw, B. (2021) Survminer: drawing survival curves using 'ggplot2'. Available at: https://CRAN.R-project.org/package=survminer.
Bastian M., Heymann S., Jacomy, M. (2009). Gephi: an open source software for exploring and manipulating networks. Int. AAAI Conf. Weblogs Soc. Media. 324(2):C573–C587. doi: 10.1609/icwsm.v3i1.13937
Berry, D., Kuzyk, O., Rauch, I., Heider, S., Schwab, C., Hainzl, E., et al. (2015). Intestinal microbiota signatures associated with inflammation history in mice experiencing recurring colitis. Front. Microbiol. 6, 1408. doi: 10.3389/fmicb.2015.01408
Bloom, S. M., Bijanki, V. N., Nava, G. M., Sun, L., Malvin, N. P., Donermeyer, D. L., et al. (2011). Commensal bacteroides species induce colitis in host-genotype-specific fashion in a mouse model of inflammatory bowel disease. Cell Host Microbe 9 (5), 390–403. doi: 10.1016/j.chom.2011.04.009
Burke, M. L., Jones, M. K., Gobert, G. N., Li, Y. S., Ellis, M. K., McManus, D. P. (2009). Immunopathogenesis of human schistosomiasis. Parasite Immunol. 31 (4), 163–176. doi: 10.1111/j.1365-3024.2009.01098.x
Cai, Y., Li, X., Han, Q., Bai, J., Zheng, Q., Sun, R., et al. (2023). Si-Ni-San improves experimental colitis by favoring akkermensia colonization. J. Ethnopharmacol 305, 116067. doi: 10.1016/j.jep.2022.116067
Cao, G., Wang, K., Li, Z., Tao, F., Xu, Y., Lan, J., et al. (2018). Bacillus amyloliquefaciens ameliorates dextran sulfate sodium-induced colitis by improving gut microbial dysbiosis in mice model. Front. Microbiol. 9, 3260. doi: 10.3389/fmicb.2018.03260
Choi, S., Hwang, Y. J., Shin, M. J., Yi, H. (2017). Difference in the gut microbiome between ovariectomy-induced obesity and diet-induced obesity. J. Microbiol. Biotechnol. 27 (12), 2228–2236. doi: 10.4014/jmb.1710.10001
Colley, D. G., Bustinduy, A. L., Secor, W. E., King, C. H. (2014). Human schistosomiasis. Lancet 383 (9936), 2253–2264. doi: 10.1016/S0140-6736(13)61949-2
Di Lorenzo, F., De Castro, C., Silipo, A., Molinaro, A. (2019). Lipopolysaccharide structures of gram-negative populations in the gut microbiota and effects on host interactions. FEMS Microbiol. Rev. 43 (3), 257–272. doi: 10.1093/femsre/fuz002
Dziarski, R., Park, S. Y., Kashyap, D. R., Dowd, S. E., Gupta, D. (2016). Pglyrp-regulated gut microflora prevotella falsenii, parabacteroides distasonis and bacteroides eggerthii enhance and alistipes finegoldii attenuates colitis in mice. PloS One 11 (1), e0146162. doi: 10.1371/journal.pone.0146162
F, H. J. (2022) Hmisc: harrell miscellaneous. Available at: https://CRAN.R-project.org/package=Hmisc.
Fairfax, K., Nascimento, M., Huang, S. C., Everts, B., Pearce, E. J. (2012). Th2 responses in schistosomiasis. Semin. Immunopathol. 34 (6), 863–871. doi: 10.1007/s00281-012-0354-4
FitzGerald, S. F., Moloney, A. C., Maurer, B. J., Hall, W. W. (2006). Gemella endocarditis: consider the colon. J. Heart Valve Dis. 15 (6), 833–835.
Gabor Csardi, T. N. (2016). The igraph software package for complex network research. InterJournal. Complex Syst., 1695.
Galaxy, C. (2022). The galaxy platform for accessible, reproducible and collaborative biomedical analyses: 2022 update. Nucleic Acids Res. 50 (W1), W345–W351. doi: 10.1093/nar/gkac247
Gao, Q., Sun, G., Duan, J., Luo, C., Yangji, C., Zhong, R., et al. (2022). Alterations in gut microbiota improve SCFA production and fiber utilization in Tibetan pigs fed alfalfa diet. Front. Microbiol. 13, 969524. doi: 10.3389/fmicb.2022.969524
Gao, Q., Liu, Z., Li, K., Bai, G., Liu, L., Zhong, R., et al. (2023). Time-course effects of different fiber-rich ingredients on energy values, microbiota composition and SCFA profile in growing pigs. Anim. Nutr. 12, 263–275. doi: 10.1016/j.aninu.2022.10.003
Garrett, W. S., Gallini, C. A., Yatsunenko, T., Michaud, M., DuBois, A., Delaney, M. L., et al. (2010). Enterobacteriaceae act in concert with the gut microbiota to induce spontaneous and maternally transmitted colitis. Cell Host Microbe 8 (3), 292–300. doi: 10.1016/j.chom.2010.08.004
Guarino, A., Guandalini, S., Lo Vecchio, A. (2015). Probiotics for prevention and treatment of diarrhea. J. Clin. Gastroenterol. 49, S37–S45. doi: 10.1097/MCG.0000000000000349
Gunda, D. W., Kilonzo, S. B., Manyiri, P. M., Peck, R. N., Mazigo, H. D. (2020). Morbidity and mortality due to schistosoma mansoni related periportal fibrosis: could early diagnosis of varices improve the outcome following available treatment modalities in Sub Saharan Africa? a scoping review. Trop. Med. Infect. Dis. 5 (1), 20. doi: 10.3390/tropicalmed5010020
Han, B., Zhai, Y., Li, X., Zhao, H., Sun, C., Zeng, Y., et al. (2023). Total flavonoids of tetrastigma hemsleyanum diels et gilg inhibits colorectal tumor growth by modulating gut microbiota and metabolites. Food Chem. 410, 135361. doi: 10.1016/j.foodchem.2022.135361
Hu, R. (2022). Grifola frondosa may play an anti-obesity role by affecting intestinal microbiota to increase the production of short-chain fatty acids. Front. Endocrinol. (Lausanne) 13, 1105073. doi: 10.3389/fendo.2022.1105073
Hu, Y., Chen, J., Xu, Y., Zhou, H., Huang, P., Ma, Y., et al. (2020). Alterations of gut microbiome and metabolite profiling in mice infected by schistosoma japonicum. Front. Immunol. 11, 569727. doi: 10.3389/fimmu.2020.569727
Jiang, Z., Li, W., Su, W., Wen, C., Gong, T., Zhang, Y., et al. (2021). Protective effects of bacillus amyloliquefaciens 40 against clostridium perfringens infection in mice. Front. Nutr. 8, 733591. doi: 10.3389/fnut.2021.733591
Kojima, A., Nakano, K., Wada, K., Takahashi, H., Katayama, K., Yoneda, M., et al. (2012). Infection of specific strains of streptococcus mutans, oral bacteria, confers a risk of ulcerative colitis. Sci. Rep. 2, 332. doi: 10.1038/srep00332
Kolde, R. (2019) Pheatmap: pretty heatmaps. Available at: https://CRAN.R-project.org/package=pheatmap.
Leibovitzh, H., Lee, S. H., Xue, M., Raygoza Garay, J. A., Hernandez-Rocha, C., Madsen, K. L., et al. (2022). Altered gut microbiome composition and function are associated with gut barrier dysfunction in healthy relatives of patients with crohn's disease. Gastroenterology 163 (5), 1364–1376. doi: 10.1053/j.gastro.2022.07.004
Li, H. J., Liang, Y. S., Dai, J. R., Wang, W., Qu, G. L., Li, Y. Z., et al. (2011). Studies on resistance of schistosoma to praziquantel XIV experimental comparison of susceptibility to praziquantel between PZQ-resistant isolates and PZQ-susceptible isolates of schistosoma japonicum in stages of adult worms, miracidia and cercariae. Zhongguo Xue Xi Chong Bing Fang Zhi Za Zhi 23 (6), 611–619.
Li, J., Sung, C. Y., Lee, N., Ni, Y., Pihlajamaki, J., Panagiotou, G., et al. (2016). Probiotics modulated gut microbiota suppresses hepatocellular carcinoma growth in mice. Proc. Natl. Acad. Sci. U.S.A. 113 (9), E1306–E1315. doi: 10.1073/pnas.1518189113
Li, X. H., DeMarco, R., Neves, L. X., James, S. R., Newling, K., Ashton, P. D., et al. (2018). Microexon gene transcriptional profiles and evolution provide insights into blood processing by the schistosoma japonicum esophagus. PloS Negl. Trop. Dis. 12 (2), e0006235. doi: 10.1371/journal.pntd.0006235
Lin, D., Song, Q., Zhang, Y., Liu, J., Chen, F., Du, S., et al. (2021). Bacillus subtilis attenuates hepatic and intestinal injuries and modulates gut microbiota and gene expression profiles in mice infected with schistosoma japonicum. Front. Cell Dev. Biol. 9, 766205. doi: 10.3389/fcell.2021.766205
Liu, Y. X., Qin, Y., Chen, T., Lu, M., Qian, X., Guo, X., et al. (2021). A practical guide to amplicon and metagenomic analysis of microbiome data. Protein Cell 12 (5), 315–330. doi: 10.1007/s13238-020-00724-8
Liu Y, W. T., Chen, T. (2021) Amplicon: statistics and visualization for microbiome data. Available at: https://github.com/microbiota/amplicon.
Lopez-Dupla, M., Creus, C., Navarro, O., Raga, X. (1996). Association of gemella morbillorum endocarditis with adenomatous polyps and carcinoma of the colon: case report and review. Clin. Infect. Dis. 22 (2), 379–380. doi: 10.1093/clinids/22.2.379
McManus, D. P., Gray, D. J., Li, Y., Feng, Z., Williams, G. M., Stewart, D., et al. (2010). Schistosomiasis in the people's republic of China: the era of the three gorges dam. Clin. Microbiol. Rev. 23 (2), 442–466. doi: 10.1128/CMR.00044-09
McManus, D. P., Dunne, D. W., Sacko, M., Utzinger, J., Vennervald, B. J., Zhou, X. N. (2018). Schistosomiasis. Nat. Rev. Dis. Primers 4 (1), 13. doi: 10.1038/s41572-018-0013-8
Mo, J., Ni, J., Zhang, M., Xu, Y., Li, Y., Karim, N., et al. (2022). Mulberry anthocyanins ameliorate DSS-induced ulcerative colitis by improving intestinal barrier function and modulating gut microbiota. Antioxidants (Basel) 11 (9), 1674. doi: 10.3390/antiox11091674
Ni, J., Shen, T. D., Chen, E. Z., Bittinger, K., Bailey, A., Roggiani, M., et al. (2017). A role for bacterial urease in gut dysbiosis and crohn's disease. Sci. Transl. Med. 9 (416). doi: 10.1126/scitranslmed.aah6888
Niu, M. M., Guo, H. X., Cai, J. W., Meng, X. C. (2022). Bifidobacterium breve alleviates DSS-induced colitis in mice by maintaining the mucosal and epithelial barriers and modulating gut microbes. Nutrients 14 (18), 3671. doi: 10.3390/nu14183671
Oksanen J, S. G., Blanchet, F., Kindt, R., Legendre, P., Minchin, P., O'Hara, R., et al. (2022) Community ecology. R package version 2.6-4. Available at: https://CRAN.R-project.org/package=vegan.
Pearce, E. J., MacDonald, A. S. (2002). The immunobiology of schistosomiasis. Nat. Rev. Immunol. 2 (7), 499–511. doi: 10.1038/nri843
Qi, C., Li, Y., Yu, R. Q., Zhou, S. L., Wang, X. G., Le, G. W., et al. (2017). Composition and immuno-stimulatory properties of extracellular DNA from mouse gut flora. World J. Gastroenterol. 23 (44), 7830–7839. doi: 10.3748/wjg.v23.i44.7830
Qian, X. B., Chen, T., Xu, Y. P., Chen, L., Sun, F. X., Lu, M. P., et al. (2020). A guide to human microbiome research: study design, sample collection, and bioinformatics analysis. Chin. Med. J. 133 (15), 1844–1855. doi: 10.1097/CM9.0000000000000871
R Core Team (2022). R: a language and environment for statistical computing (Vienna, Austria: R Foundation for Statistical Computing). Available at: https://www.R-project.org/.
Sanders, M. E., Heimbach, J. T., Pot, B., Tancredi, D. J., Lenoir-Wijnkoop, I., Lahteenmaki-Uutela, A., et al. (2011). Health claims substantiation for probiotic and prebiotic products. Gut Microbes 2 (3), 127–133. doi: 10.4161/gmic.2.3.16174
Terry, M., Therneau, P. M. G. (2000). Modeling survival data: extending the cox model (New York: Springer).
Vazquez-Mendoza, P., Elghandour, M. M. M., Alaba, P. A., Sanchez-Aparicio, P., Alonso-Fresan, M. U., Barbabosa-Pliego, A., et al. (2018). Antimicrobial and bactericidal impacts of bacillus amyloliquefaciens CECT 5940 on fecal shedding of pathogenic bacteria in dairy calves and adult dogs. Microb. Pathog. 114, 458–463. doi: 10.1016/j.micpath.2017.11.040
Wang, Y., Wu, Y., Wang, B., Xu, H., Mei, X., Xu, X., et al. (2019). Bacillus amyloliquefaciens SC06 protects mice against high-fat diet-induced obesity and liver injury via regulating host metabolism and gut microbiota. Front. Microbiol. 10, 1161. doi: 10.3389/fmicb.2019.01161
Wang, N., Peng, H. Q., Gao, C. Z., Cheng, Y. H., Sun, M. T., Qu, G. L., et al. (2022). In vivo efficiency of praziquantel treatment of single-sex schistosoma japonicum aged three months old in mice. Int. J. Parasitol. Drugs Drug Resist. 20, 129–134. doi: 10.1016/j.ijpddr.2022.11.002
Wen, X., Wang, H. G., Zhang, M. N., Zhang, M. H., Wang, H., Yang, X. Z. (2021). Fecal microbiota transplantation ameliorates experimental colitis via gut microbiota and T-cell modulation. World J. Gastroenterol. 27 (21), 2834–2849. doi: 10.3748/wjg.v27.i21.2834
WoldemariamYohannes, K., Wan, Z., Yu, Q., Li, H., Wei, X., Liu, Y., et al. (2020). Prebiotic, probiotic, antimicrobial, and functional food applications of bacillus amyloliquefaciens. J. Agric. Food Chem. 68 (50), 14709–14727. doi: 10.1021/acs.jafc.0c06396
Wu, Y., Wang, Y., Zou, H., Wang, B., Sun, Q., Fu, A., et al. (2017). Probiotic bacillus amyloliquefaciens SC06 induces autophagy to protect against pathogens in macrophages. Front. Microbiol. 8, 469. doi: 10.3389/fmicb.2017.00469
Xue, J., Hu, X., Hao, Y., Gong, Y., Wang, X., Huang, L., et al. (2022). Transmission risk predicting for schistosomiasis in mainland China by exploring ensemble ecological niche modeling. Trop. Med. Infect. Dis. 8 (1), 24. doi: 10.3390/tropicalmed8010024
Yadav, M. K., Kumari, I., Singh, B., Sharma, K. K., Tiwari, S. K. (2022). Probiotics, prebiotics and synbiotics: safe options for next-generation therapeutics. Appl. Microbiol. Biotechnol. 106 (2), 505–521. doi: 10.1007/s00253-021-11646-8
Yahya, N. A., Lanham, J. K., Sprague, D. J., Palygin, O., McCorvy, J. D., Marchant, J. S. (2023). Molecular and cellular basis of praziquantel action in the cardiovascular system. Am. J. Physiol. Cell Physiol. 324 (2), C573–C587. doi: 10.1152/ajpcell.00520.2022
Yang, X., Ding, W., Qian, X., Jiang, P., Chen, Q., Zhang, X., et al. (2021). Schistosoma japonicum infection leads to the reprogramming of glucose and lipid metabolism in the colon of mice. Front. Vet. Sci. 8, 645807. doi: 10.3389/fvets.2021.645807
Zhang, B., Wu, X., Song, Q., Ning, A., Liang, J., Song, L., et al. (2020). Gut microbiota modulates intestinal pathological injury in schistosoma japonicum-infected mice. Front. Med. (Lausanne) 7, 588928. doi: 10.3389/fmed.2020.588928
Zhao, Y., Yang, S., Li, B., Li, W., Wang, J., Chen, Z., et al. (2019). Alterations of the mice gut microbiome via schistosoma japonicum ova-induced granuloma. Front. Microbiol. 10, 352. doi: 10.3389/fmicb.2019.00352
Zhao, H., Cheng, N., Zhou, W., Chen, S., Wang, Q., Gao, H., et al. (2019). Honey polyphenols ameliorate DSS-induced ulcerative colitis via modulating gut microbiota in rats. Mol. Nutr. Food Res. 63 (23), e1900638. doi: 10.1002/mnfr.201900638
Zhou, H., Zeng, X., Sun, D., Chen, Z., Chen, W., Fan, L., et al. (2020). Monosexual cercariae of schistosoma japonicum infection protects against DSS-induced colitis by shifting the Th1/Th2 balance and modulating the gut microbiota. Front. Microbiol. 11, 606605. doi: 10.3389/fmicb.2020.606605
Keywords: Bacillus amyloliquefaciens, Schistosoma japonicum, pathological damage, intestinal microbiome, dynamic change
Citation: Chen H, Sun R, Wang J, Yao S, Batool SS, Yu Z, Huang S and Huang J (2023) Bacillus amyloliquefaciens alleviates the pathological injuries in mice infected with Schistosoma japonicum by modulating intestinal microbiome. Front. Cell. Infect. Microbiol. 13:1172298. doi: 10.3389/fcimb.2023.1172298
Received: 23 February 2023; Accepted: 24 April 2023;
Published: 17 May 2023.
Edited by:
Eugenia Bezirtzoglou, Democritus University of Thrace, GreeceReviewed by:
Jipeng Wang, Fudan University, ChinaNikhilesh Joardar, Washington University in St. Louis, United States
Robert Fultz, Brightseed, United States
Copyright © 2023 Chen, Sun, Wang, Yao, Batool, Yu, Huang and Huang. This is an open-access article distributed under the terms of the Creative Commons Attribution License (CC BY). The use, distribution or reproduction in other forums is permitted, provided the original author(s) and the copyright owner(s) are credited and that the original publication in this journal is cited, in accordance with accepted academic practice. No use, distribution or reproduction is permitted which does not comply with these terms.
*Correspondence: Jing Huang, jing_huang@csu.edu.cn; Shuaiqin Huang, sqhuang@csu.edu.cn