- 1State Key Laboratory of Oral Diseases & National Clinical Research Center for Oral Disease, West China Hospital of Stomatology, Sichuan University, Chengdu, China
- 2Department of Stomatology, Chengdu Second People’s Hospital, Chengdu, China
- 3Division of Oral Ecology and Biochemistry, Graduate School of Dentistry, Tohoku University, Sendai, Japan
Dental caries is associated with caries-related streptococci and antimicrobial agents have been widely used for caries control, but troubled by antibiotic resistance. This study aimed to investigate the intrinsic and acquired resistance of caries-related streptococci to antimicrobial peptide GH12, which was proven promising for caries control, and preliminarily explore the phenotypic changes and whole genome of stable acquired resistant strains. In this study, susceptibility assays and resistance assays were performed, followed by stability assays of resistance, to evaluate the intrinsic resistance and the potential resistance of caries-related streptococci. Then, the phenotypic changes of the stable acquired resistant strain were explored. The whole genome of the resistant strain was sequenced and analyzed by second-generation and third-generation high-throughput sequencing technologies. Streptococcus gordonii and Streptococcus sanguinis were intrinsically resistant to GH12 compared to cariogenic Streptococcus mutans. Acquired GH12 resistance in one S. sanguinis and four S. mutans clinical strains was transient but stable in one S. mutans strain (COCC33-14). However, acquired resistance to daptomycin (DAP) and chlorhexidine in all strains was stable. Furthermore, the COCC33-14 showed cross-resistance to DAP and delayed growth rates and a lower population. However, no drug-resistant gene mutation was detected in this strain, but 6 new and 5 missing genes were found. Among them, annotation of one new gene (gene 1782|COCC33-14R) is related to the integral component of the membrane, and one missing gene rpsN is associated with the metabolism and growth of bacteria. The results indicate that stable resistant mutants of caries-related streptococci could hardly be selected by exposure to consecutive sublethal GH12, but the risk still existed. Resistance in COCC33-14R is mainly related to changes in the cell envelope.
Introduction
Dental caries affects approximately 3.9 billion people, causing huge health and economic burdens (Pitts et al., 2017), and is directly associated with biofilm communities, in which several oral streptococci, such as Streptococcus mutans, Streptococcus gordonii and Streptococcus sanguinis, are involved in the caries process (Takahashi and Nyvad, 2011).Among them, S. gordonii and S. sanguinis are considered more related to symbiotic or health-associated oral conditions, while S. mutans is considered more cariogenic especially in the progress of caries (Takahashi and Nyvad, 2011; Wright, 2011).
As dental caries is closely associated with oral bacteria, antimicrobial agents are widely used and studied in the prevention and treatment of caries. Fluoride has been introduced to caries control since 1980s (Buzalafa et al., 2011), and made huge contribution to the decline of caries in recent decades. It has been used in community water fluoridation, toothpaste in general population (Walsh et al., 2019; Whelton et al., 2019) and varnish in high caries risk children (Carey, 2014). According to World Oral Health report 2003 (Petersen and Lennon, 2004), fluoride made extraordinary effectiveness in caries prevention of all ages. One community-randomized controlled trial in dental caries among Australian Aboriginal children demonstrated that F-varnish application once every 6 months for 2 years significantly reduce adjusted d3mfs increment in the intervention group (Slade et al., 2011). CHX was one of the first antiseptic agents proposed for dental caries and has proved to be effective for retardation of smooth caries with dentifrice containing 1% chlorhexidine (Gjermo, 1974; Johansen et al., 1975). CHX varnish could reduce the mean number of new white spot lesions of orthodontic patients (Lipták et al., 2018), and improve oral hygiene of high caries risk patients such as geriatric and xerostomia patients and post head and neck radiation patients (Slot et al., 2011; Hong et al., 2018). Hong et al. (Hong et al., 2018) showed that once daily regimens of 0.12% chlorhexidine in post head and neck radiation patients contributed to significant reduction in plaque index and decrease in salivary S. mutans count. Quaternary ammonium salts (QAS) was added into mouthwash in 1970s and was proved effective to reduce dental plaques (Ciancio et al., 1975). Nowadays, different forms of QAS were successfully added into dental materials, such as dental adhensive (Melo et al., 2013) and resin composites (Cheng et al., 2016) to prevent secondary caries. An adhesive containing dimethylaminododecyl methacrylate (DMADDM) significantly reduced both lesion depth and mineral loss, and also lowered the Keyes scores in vivo in a secondary caries animal model (Wu et al., 2018).
However, there are some arguments that resistant strains could emerge during frequent use and drug misuse. Antimicrobial resistance (AMR) in pathogenic bacteria has been a worldwide problem, causing enormous economic loss and a global health crisis (Wright, 2011). Bacteria can be intrinsically resistant to certain antibiotics and can obtain acquired resistance through long-term selective pressure. Intrinsic resistance of bacteria to a particular antibiotic is the ability to resist the action of that antibiotic due to inherent structural or functional properties (Blair et al., 2015). Acquired resistance is generally mediated by genetic mutation in chromosomes or via horizontal gene transfer (Munita and Arias, 2016). Acquired resistance with the acquisition of genetic determinants has the highest risk of horizontal spread and the most unacceptable harm.
Oral streptococci have shown an increased frequency of AMR, such as clindamycin (Loyola-Rodriguez et al., 2018), tetracycline (Diaz-Torres et al., 2006), amoxicillin (Diaz-Torres et al., 2006), penicillin (Pasquantonio et al., 2012), fluoroquinolones (De Lastours and Fantin, 2015) and lipopeptide daptomycin (DAP) (Garcia-de-la-Maria et al., 2013). In addition, AMR have been found under topical antimicrobial agent applications, such as fluoride (Liao et al., 2017) and CHX (Suppiger et al., 2020). Since oral streptococci are the dominant part of dental plaques and initially attach to the tooth surface (Lee and Lee, 2019), the resistance of caries-related streptococci needs to be studied preferentially.
Antimicrobial peptides (AMPs) are attracting interest worldwide and are generally considered as alternative therapy of conventional antibiotics. The bactericidal mechanisms of AMP are considered to involve cationic AMP that is adsorbed on the anionic bacterial outer membrane and pore formation resulting in general membrane disruption (Lee et al., 2016). However, AMP resistance has arisen through their clinical use, such as polymycin (Moffatt et al., 2019), colistin (Fernandez and Hancock, 2012) and DAP. DAP is an antimicrobial lipopeptide that has been applied in the clinic since 2003 (Blair et al., 2015) for multiresistant gram-positive bacteria (Larioza et al., 2011). Unfortunately, some bacteria developed resistance toward DAP during these years, including Mitis group streptococci (Garcia-de-la-Maria et al., 2013; Parrett et al., 2020) and Staphylococcus aureus (Kaatz et al., 2006). In the field of caries prevention and control, synthetic or natural AMPs, such as defensins and derivatives (Aida et al., 2018), histatins and derivatives (Mai et al., 2017), nisin (Su et al., 2018), LL-37 (da Silva et al., 2012), L-K6 (Sztukowska et al., 2019), and Bac8c (Ding et al., 2014), have been proven to show anti-caries effects without cytotoxicity. In our previous studies, the cationic AMP GH12 was proven to be effective as an anti-caries agent, which killed both planktonic and biofilms of cariogenic bacteria quickly and efficiently (Tu et al., 2016; Wang Y. et al., 2017; Jiang et al., 2018), suppressed the virulence factors of S. mutans (Wang et al., 2018a), and inhibited caries in a rat caries model (Wang et al., 2018b). GH12 could be a promising antimicrobial agent in oral application, but whether oral streptococci would be resistant to GH12 has not been evaluated.
The aim of this study was to explore the potential resistance of caries-related streptococci against de novo AMP GH12 through susceptibility assays to evaluate the intrinsic resistance of oral streptococci to GH12 and resistance assays with continuous GH12 selective pressure to explore the acquired resistance. As far as we know, although studies on anti-caries AMP are increasing, there have been few investigations into resistance to AMP until now. The present study, for the first time, evaluated the potential resistance of caries-related streptococci to GH12 before its clinical use in advance to prevent acquired GH12 resistance from emerging and transferring, which helps prolong the useful time of GH12 and provides adequate directions for its further clinical use.
Materials and methods
Peptides, chemicals, and assay kits
The peptide GH12 (Gly-Leu-Leu-Trp-His-Leu-Leu-His-His-Leu-Leu-His-NH2) was synthesized, identified, and purified to 98% by GL Biochem (Shanghai, China) as described previously (Wang Y. et al., 2017). The peptide was dissolved in sterile deionized water (DDW) and stored at −20 °C. Unless otherwise stated, the chemicals and assay kits were purchased from Sigma−Aldrich (St. Louis, MO, US).
Bacterial strains and growth conditions
Caries-related streptococci (Supplementary Table 1) which included S. mutans, S. gordonii and S. sanguinis, were obtained from American Type Culture Collection (ATCC, Manassas, VA), Guangdong Culture Collection Center (GS, Guangzhou, China), and Japan Collection of Microorganisms (JCM, Tokyo, Japan). In addition, clinical strains of S. mutans (Supplementary Table 1) were included in the assay and were collected, isolated and identified according to a previous study from West China Hospital of Stomatology, Chengdu, China (Lu et al., 2015). All the strains were grown in brain-heart infusion broth (BHI; Oxoid, Basingstoke, Hampshire, UK) anaerobically (85% N2, 10% H2 and 5% CO2) at 37°C.
Bacterial susceptibility assay
To evaluate the intrinsic resistance of different caries-related streptococci to antimicrobial agents, the minimal inhibitory concentration (MIC) was determined using a modified broth microdilution method (Wang Y. et al., 2017). BHI broth (80 µL), twofold serial dilutions of tested agents (20 μL), and bacteria suspension (100 µL) were placed in 96-well plates with a final concentration of bacteria of 1.0 × 108 CFU/mL. The final concentrations of GH12 ranged from 4.0 to 128.0 mg/L. CHX and DAP (Marklin Biochemical Co., Ltd., Shanghai, China) served as controls. Negative and blank controls were incubated with DDW or fresh BHI broth, respectively. Incubation steps were based on the CLSI guidelines (CLSI, 2018). Absorbance at 600 nm (A600) was recorded using a microplate spectrophotometer (Multiskan GO; Thermo Scientific, USA). MIC was defined as the lowest concentration where no visible growth was observed (A600(test)/A600(control) < 0.1). The tests were conducted and repeated for ten times on different days.
Bacterial drug resistance assay
To further investigate whether caries-related streptococci could develop drug resistance to the tested antimicrobial agents, MIC measurements were performed following repeated serial passages, according to a previous method (Ikai et al., 2013). Aliquots (100 μL) of the bacterial suspensions in the sub-MIC well were taken and inoculated into 10 mL of fresh culture medium broth at 37°C anaerobically. The overnight bacterial suspensions were diluted to a concentration of approximately 1.0 × 108 CFU/mL for the next passage MIC test. All MIC tests were repeatedly performed for 10 passages. After repeated exposures to one antimicrobial agent, an increase in the MIC value compared to the initial MIC indicated the acquisition of drug resistance by the bacterial cells. The tests were conducted and repeated for three times on different days.
Assay of the stability of resistance
To evaluate the stability of acquired resistance, the assay was performed as previously described (Hong et al., 2016). Aliquots of resistant strain suspensions (100 µL) were taken and incubated in fresh 10 mL of BHI broth without antimicrobial agent under anaerobic conditions overnight, which was repeated for 10 continuous passages. And the bacterial susceptibility assay was performed at each passage to determine the MIC value. The tests were conducted and repeated for three times on different days.
Cross-resistance assay
The susceptibility of the resistant strain to other antimicrobial agents was determined. The GH12-resistant S. mutans COCC33-14 isolate was selected and confirmed through the aforementioned assays. The resistant strain and parent strain were incubated anaerobically as mentioned before. Twofold serial dilutions of DAP and CHX were prepared to final concentrations ranging from 4.0 mg/L to 128.0 mg/L and 0.5 mg/L to 16.0 mg/L, respectively. The overnight suspension was adjusted to 2.0×108 CFU/mL. Then, 100 μL of suspension, 80 μL of BHI broth, and 20 μL of prepared antimicrobial dilutions were mixed in each well of 96-well U-bottom microtiter plates. Negative and blank controls were incubated with DDW or fresh BHI broth, respectively. Incubation and determination are according to MIC measurement mentioned above in Bacterial susceptibility assay. The tests were conducted and repeated for three times on different days.
Measurement of bacterial growth curves
Bacterial growth curves were generated following modified previous methods (Castillo et al., 2006) to evaluate the growth and metabolism changes of acquired resistant bacteria after being challenged with antibacterial agents. The initial concentrations of the bacteria (S. mutans UA159, S. mutans COCC33-14 parental strain, and GH12-resistant S. mutans COCC33-14 strain) were adjusted to 1 × 106 CFU/mL. The inoculums were incubated at 37 °C anaerobically. A600 was detected over 24 h, and the growth inhibition kinetics were drawn based on the correspondence between A600 and incubation time. The tests were conducted and repeated for three times on different days.
Whole genome sequencing and bioinformatics analysis
Genomic DNA was sequenced using a combination of Illumina NovaSeq6000 and Nanopore PromethION sequencing platforms. All of the analyses were performed using the online platform of Majorbio Cloud Platform (http://cloud.majorbio.com).
Glimmer Version 3.02 (Delcher et al., 2007) and GeneMarks (Besemer and Borodovsky, 2005) was used for codon sequence (CDS) prediction of chromosome and plasmid respectively. tRNA-scan-SE v2.0 (Chan and Lowe, 2019) was used for tRNA prediction, and Barrnap v0.9 was used for rRNA prediction. The predicted CDSs were annotated from NCBI’s nonredundant (NR), Swiss-Prot, Pfam, Gene Ontology (GO), Clusters of Orthologous Groups of proteins (COG), Kyoto Encyclopedia of Genes and Genomes (KEGG), Resfinder and Comprehensive Antibiotic Resistance Database (CARD). The detailed methods were presented in Supplementary S1.
Statistical analysis
GraphPad Prism 8.0 (GraphPad, USA) was used to evaluate quantitative data statistically using one-way ANOVA and Fisher’s LSD tests, and differences were considered significant when P < 0.05.
Results
The resistance of caries-related streptococci and the stability of acquired resistance
To evaluate the spectrum of intrinsic resistance of caries-related streptococci, the total MIC values are shown in Table 1. The MICs of GH12 against S. gordonii and S. sanguinis were significantly higher than those against S. mutans (P < 0.05), indicating that S. gordonii and S. sanguinis possessed intrinsic resistance to GH12. Similarly, the MICs of DAP against S. mutans and S. gordonii were higher than those against S. sanguinis (P < 0.05), which indicated that DAP was more effective against S. sanguinis. However, the intrinsic resistance to CHX did not vary totally depending on species, and the MICs of two strains of S. gordonii (ATCC35105, ATCC33399) and S. sanguinis (JCM5708, SK36) against CHX were higher than those of S. mutans and S. sanguinis ATCC29667 (P < 0.05).
To evaluate the spectrum of GH12 acquired resistance in three different species of streptococci, the serial MICs of S. mutans UA159, S. gordonii ATCC10558 and S. sanguinis JCM5708 are presented in Figure 1A. MIC values of GH12 did not increase except S. sanguinis, from 16.0 mg/L to 32.0 mg/L at passage 7. In addition, only S. gordonii ATCC10558 developed resistance to CHX through continuous passages, and the MIC value increased from 4.0 mg/L to 8.0 mg/L at passage 5. Unexpectedly, the MIC of DAP against all of the tested strains increased and even to high-level drug resistance for S. gordonii (from 32.0 mg/L to 128.0 mg/L) and S. sanguinis (from 4.0 mg/L to 64.0 mg/L).
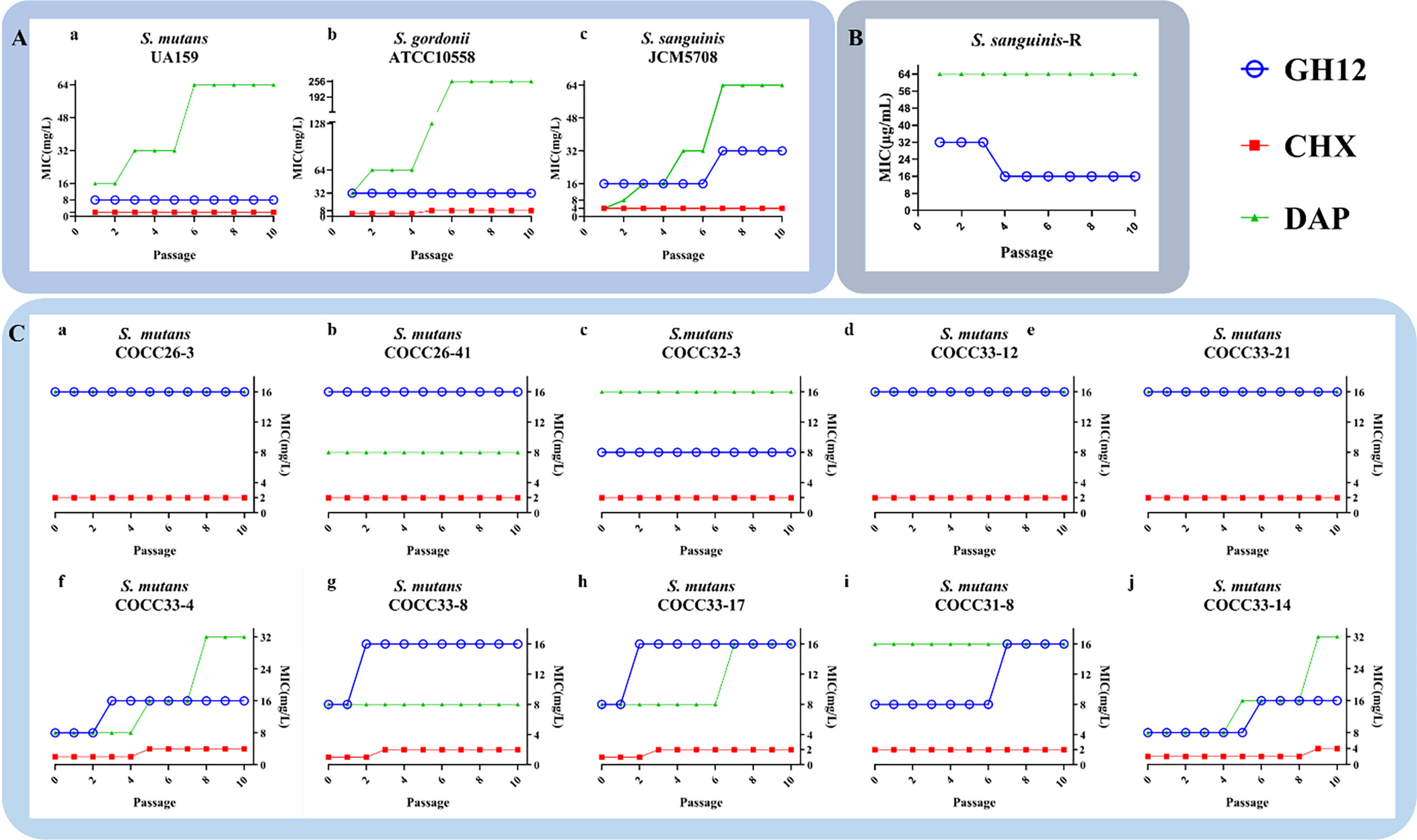
Figure 1 (A) MICs of GH12, CHX and DAP against S. mutans UA159 (a), S. gordonii ATCC10558 (b) and S. sanguinis JCM5708 (c) from passages 1 to 10. (B) Evaluation of the stability of acquired resistance of S. sanguinis-R strain. S. sanguinis-R: resistant S. sanguinis strain toward GH12 and DAP. (C) MICs of GH12, CHX and DAP against S. mutans clinical strains (passage 0) and MICs after each passage under exposure to sub-MIC concentration (passage 1 to 10). The data are represented as mean ± standard deviation (n=3).
Nevertheless, the MIC of GH12-resistant S. sanguinis declined back to the initial MIC value of the parental strain after continuous passages in fresh BHI broth (Figure 1B). In contrast, the DAP-resistant S. sanguinis strain maintained an increased MIC value (Figure 1B). These results indicated that S. sanguinis developed stable resistance to DAP but transient resistance to GH12.
The resistance of S. mutans clinical strains and the stability of acquired resistance
The total MIC values of ten S. mutans clinical strains of are shown in Figure 1C (passage 0). The different clinical isolates of S. mutans showed varied susceptibilities, which demonstrated different potential drug resistances. To further evaluate the spectrum of GH12 acquired resistance in different isolates of S. mutans, MICs against ten S. mutans clinically isolated strains from passages 1 to 10 were monitored. As shown in Figure 1C (passage 1-10), among all ten tested strains, five clinically isolated strains (S. mutans COCC26-3, COCC26-41, COCC32-3, COCC33-12, COCC33-21) maintained the same MIC values under the continuous selective burden of all antimicrobial agents. For the other clinical isolates, a tendency of acquired resistance was observed through the phenomenon of increased MIC of GH12 along the repeated passages. The MIC values of S. mutans COCC33-8, COCC33-4, and COCC33-17 increased twofold at passage 2 or 3, and COCC31-8 and COCC33-14 increased at passage 6 or 7. On the other hand, the MICs of CHX increased twofold against four isolates of S. mutans (COCC33-8, COCC33-4, COCC33-17 and COCC33-14). In addition, the MICs of DAP against three isolates (COCC33-4, COCC33-17 and COCC33-14) increased by 2-4 times.
To test the stability of acquired resistance, resistant strains were regrown without antimicrobial agents for 10 passages, and the MIC values were determined. As shown in Figure 2, although the MICs of the five isolates decreased, only GH12-resistant S. mutans COCC33-14 maintained an increased MIC value. In contrast, all of the CHX- or DAP-resistant strains retained the increased MIC values, which were 2-4 times higher than those of the parental strains. The unchanged MICs demonstrated that these isolates developed stable resistance to the corresponding antimicrobial agent.
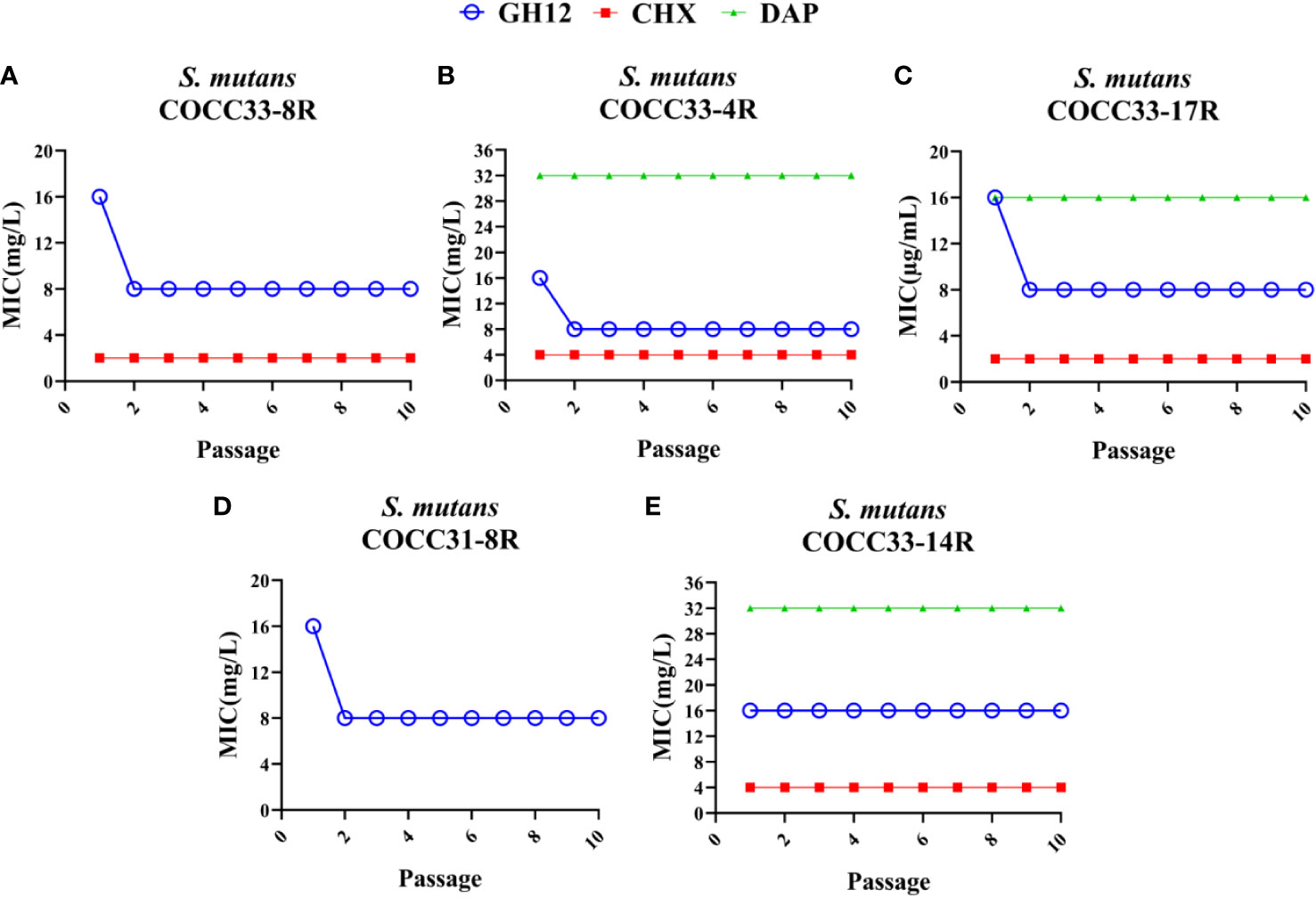
Figure 2 Evaluation of the stability of acquired resistance of S. mutans clinical strains. MIC values of GH12, CHX and DAP to S. mutans COCC33-8R (A), COCC33-4R (B), COCC33-17R (C), COCC31-8R (D) and COCC33-14R (E) after each passage regrown in the lack of antimicrobial agent, respectively (mean ± SD; n = 3). R, resistant strain.
The cross-resistance and kinetic growth curves of the GH12-resistant strain
The MIC values of other antimicrobial agents against GH12-resistant S. mutans COCC33-14 strain (COCC33-14R) are shown in Table 2. For CHX, the MIC value was 2.0 mg/L and equal to the parental strain, which indicated that COCC33-14R was susceptible to CHX. However, the MIC of DAP against COCC33-14R was four times higher than the that of parental strain. The results demonstrated that COCC33-14R showed cross-resistance to DAP but no cross-resistance to CHX.
To compare the growth differences between UA159, COCC33-14, and COCC33-14R, 24-h representative growth curves are shown in Figure 3. UA159 and COCC33-14 showed similar lag phases and log phases, of which lag phase ended at the fourth hour, and the log phase continued from the fifth to eighth hour. Otherwise, COCC33-14R had a much longer lag phase, which sustained almost 11 h. Additionally, although with no statistical significance, the stationary phase of COCC33-14R was slightly lower than that of the other two strains. The results indicated that the growth metabolism of COCC33-14R was delayed compared to that of the parental strain.
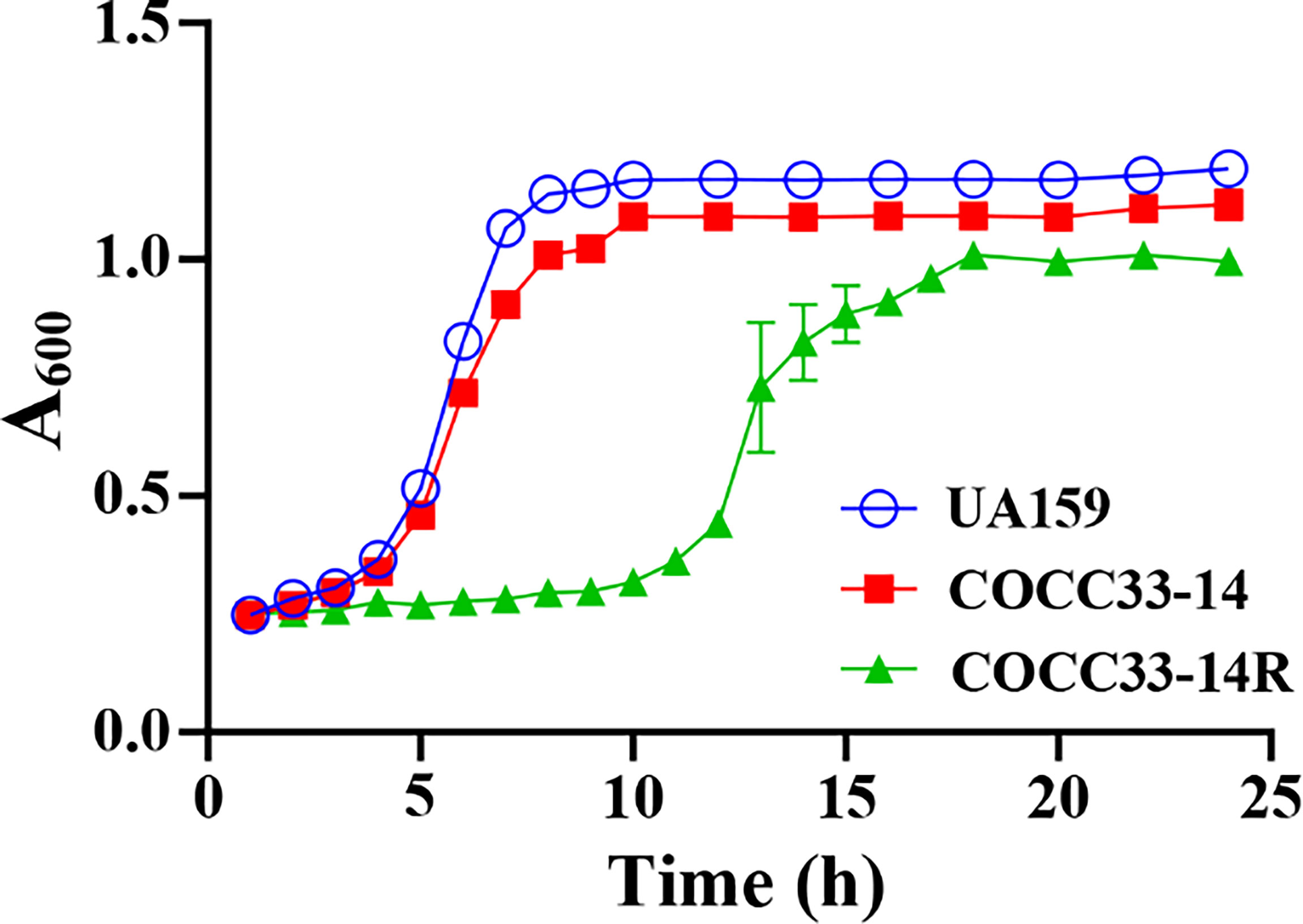
Figure 3 Kinetic growth curves (mean ± SD; n = 3). UA159: S. mutans UA159; COCC33-14: S. mutans COCC33-14 parental strain; COCC33-14R: GH12-resistant S. mutans COCC33-14 strain.
Whole genome sequencing analysis
The whole genomes of COCC33-14R, COCC33-14 and UA159 were sequenced, assembled, predicted and annotated, followed by comparison and analysis. All three strains have only one chromosomal DNA, and no plasmid, of which COCC33-14R possesses the longest sequences and most CDSs (Supplementary Table 2). Resfinder database analysis showed that no resistance genes were found in any strain. CARD annotations and predictions of probable resistance genes of COCC33-14R and COCC33-14 are shown in Figure 4A, and no differences were found between them.
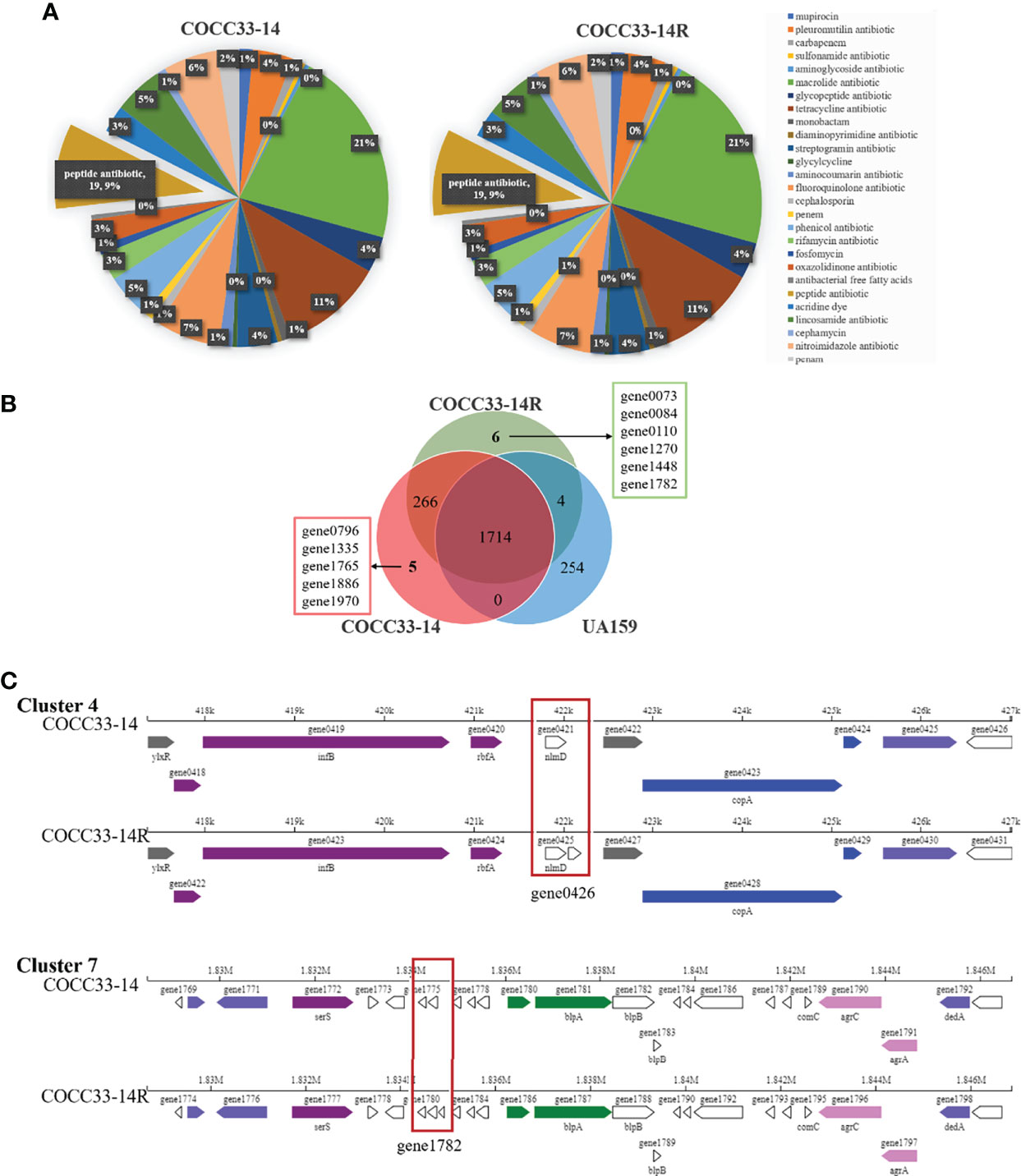
Figure 4 Analysis of genome of COCC33-14R. (A) CARD annotations and prediction of probable resistant genes. (B) Differential genes between COCC33-14R and COCC33-14. (C) Comparison of linear plot of secondary metabolite gene Cluster 4 and Cluster 7.
The differential genes between COCC33-14R and COCC33-14 are shown in a Venn diagram (Figure 4B). COCC33-14R acquires 6 new genes and misses 5 genes compared with COCC33-14. According to the annotations of the 11 differential genes (Supplementary Table 3), most of them encode hypothetical proteins. Only GO annotation of one new gene (gene1782|COCC33-14R) shows that it’s related to integral component of membrane. One missing gene of COCC33-14R (gene1886|COCC33-14) is related to the biogenesis and function of ribosomal protein S14.
The CDSs of carbohydrate-active enzymes (CAZymes) and secondary metabolites were analyzed. No difference in CAZymes was found between COCC33-14R and COCC33-14. However, there was one more gene in secondary metabolite gene Cluster 4 and Cluster 7 of COCC33-14R than COCC33-14, which were gene0426 and gene1782, respectively (Figure 4C). The annotation of gene1782 is as mentioned above, and gene0426 has no annotation.
Discussion
AMP has been used widely in the clinic, and systemic administration of AMP causes resistance in oral streptococci (Fernandez and Hancock, 2012; Moffatt et al., 2019). According to the Center for Disease Control of the USA, novel antibiotics are far from sufficient, and appropriate use of antibiotics could reduce the emergence of resistance (CDC, 2019). As a result, it’s necessary to study the potential resistance of oral bacteria to GH12 in advance to improve its clinical use. The present study explored the intrinsic and acquired drug resistance of caries-related streptococci toward the de novo AMP GH12 for the first time, and further investigated the probable mechanism of acquired resistance to GH12. The results of the current study demonstrated that different species of oral streptococci showed different intrinsic and acquired resistance to GH12. More health-associated strains, S. gordonii and S. sanguinis were intrinsically resistant to GH12, while more cariogenic strain, S. mutans showed different susceptibilities to GH12. In addition, the frequency of GH12 resistance in these oral streptococci was low. Among all thirteen tested caries-related streptococci in this study, only one S. mutans clinical strain (COCC33-14) developed stable resistance to GH12. GH12 induced transient resistance in S. sanguinis JCM5708 and four isolates of S. mutans clinical strains and no resistance in the other seven streptococci. However, DAP and CHX always selected stable resistant isolates. Furthermore, the stability of acquired resistance was tested after resistance assays. In addition, the new gene in GH12-resistant strain COCC33-14R might be related to integral component of membrane.
Streptococci are one of the most predominant bacteria in oral biofilms, and are associated with biofilm colonization and plaque formation (Lee and Lee, 2019). S. mutans is considered a representative cariogenic bacterium, with the virulence factors of acidurance, acidogenicity, and the ability to produce glucans to adhere to tooth surface (Suppiger et al., 2020). On the other hand, S. sanguinis and S. gordonii have the ability not only to produce acid but also to produce alkali (Huang et al., 2012) and H2O2 (Cheng et al., 2020), which are antagonistic with S. mutans (Huang et al., 2018) and partly linked to protection against dental caries. The results in the present study demonstrate that these bacteria are intrinsically resistant to GH12 (Table 1), so GH12 could have the advantage of being able to suppress S. mutans more selectively in vivo. This is consistent with our previous study, where GH12 could selectively inhibit S. mutans in a three-species biofilm (Jiang et al., 2018). However, the previous study neither looked into the aspect of intrinsic resistance nor further studied the acquired resistance. Remarkably, one S. sanguinis strain JCM5708 and four S. mutans clinical strains (COCC33-4, COCC33-8, COCC33-17, COCC31-8) showed transient resistance to GH12, which was reversed after several passages without GH12 (Figures 1B, C, 2A–D). A similar phenomenon was also observed in nisin-resistant Listeria monocytogenes, and its meaning was unclear (Gravesen et al., 2002). Our hypothesis was that the phenomenon of transient resistance might indicate temporary phenotypic changes rather than genetic mutation, which help bacteria resist the antibiotic effect for a short time (Andersson and Hughes, 2010). However, further studies are needed.
Since S. mutans is associated with increased caries risk and poor caries outcomes (Lima et al., 2020), and the emergence of S. mutans resistance is undesirable for caries treatment and prevention, further studies on different S. mutans strains were conducted. Different strains of the same bacterium have shown a great degree of heterogeneity. It has been reported that different clinical isolates have diverse susceptibilities to the same antimicrobial agent, such as silver nanoparticles (Espinosa-Cristóbal et al., 2012) and chitosan (Pasquantonio et al., 2008). In the present study, S. mutans clinical isolates were collected and identified by morphological identification, VITEK automatic microorganism identification and 16S rRNA gene sequencing and were named according to human oral microbiome database and EzTaxon server 2.1 (Lu et al., 2015). The clinical strains of S. mutans showed various intrinsic and acquired resistance to GH12 (Figure 1C), consistent with the results of other studies (Espinosa-Cristóbal et al., 2012). The mechanism may be not only species-dependent but also serotype-dependent, but further studies are needed.
CHX resistance in streptococci was found early in the 1970s (Schiøtt and Löe, 1972), but it has not raised much attention. Afterwards, there were several reports about CHX resistance in oral streptococci, such as S. sanguinis (Westergren and Emilson, 1980), S. gordonii (Wang S. et al., 2017) and S. mutans ATCC20523 (Verspecht et al., 2019). The present study also showed increased MIC values in S. gordonii ATCC35105 and four clinical isolates of S. mutans to CHX (Figure 1A, b, Figure 1C, f-h, j). The CHX resistance would be detrimental to infectious control and a unique clone of community-acquired MRSA was found to expand according to the acquisition of a plasmid consisting of genes (qacA/B) conferring resistance to CHX (Copin et al., 2019). CHX resistance could induce resistance in clinical Klebsiella pneumoniae isolates and cross-resistance to colistin (Wand et al., 2017). The cross resistance of CHX-resistant strains has also been well documented (Kampf, 2018a; Kampf, 2019). The present study could provide more evidence for acquired CHX resistance in S. mutans clinical strains in vitro. DAP is a cyclic lipopeptide antibiotic that has bactericidal activity against staphylococci and streptococci and is less renal toxicity than vancomycin (Larioza et al., 2011). Thus, DAP has been a best alternative for the therapy of multidrug-resistant streptococci infectious diseases for nearly 20 years. In our study, DAP showed the ability to select for resistant strains in three tested species of caries-related streptococci (Figure 1A), and three of clinical isolates of S. mutans (Figure 1C, f, h, j). In addition, S. gordonii and S. sanguinis rapidly developed high-level resistance to DAP. This was the same situation as other studies, in which streptococci acquired DAP resistance in vitro and in vivo after exposure to DAP (Garcia-de-la-Maria et al., 2013; Parrett et al., 2020). Another study also observed that stable and high-level DAP resistant mutants in S. mitis-oralis were produced by serial passages in sublethal DAP in vitro (Mishra et al., 2020). A variety of bacteria colonize the oral cavity and infect not only oral health but also general health. Oral bacteria can enter the bloodstream through ulcerated inflamed crevices and pocket epithelium and adjacent gingival microcirculation during surgery or during routine brushing and chewing, leading to systemic disease (Dhotre et al., 2016). Both previous studies and our results indicated that GH12 is advantageous over the controls because of its lower frequency of stable resistance.
The mechanism of CHX resistance is not clear, but it is widely believed to be related to changes in efflux pumps and cell membranes (Kampf, 2018b). As an AMP, the mechanism of DAP resistance in Gram-positive bacteria is generally considered to be associated with important adaptive changes in the cell wall and cell membrane homeostasis (Tran et al., 2015). However, based on CARD annotations and prediction of probable resistance genes (Figure 4A), the GH12-resistant strain did not possess any known resistance genes, and Resfinder database analysis did not find any resistance gene in any strain, suggesting that there is no similarity between the GH12-resistant and other known resistance genomes. As a result, the mechanism of GH12 resistance has to be discussed in the other aspects as follows.
The phenotypic consequences of GH12 resistance were evaluated with particular reference to antibiotic growth curves and cross-resistance, which, together with the results of gene sequencing and analysis, provide clues to elucidate the mechanism of GH12 resistance. First, GH12-resistant S. mutans COCC33-14 showed cross-resistance to DAP but was still susceptible to CHX (Table 2), suggesting that the mechanism of GH12 resistance is related to similarities and differences in the antimicrobial mechanisms of GH12, DAP and CHX. The first step of the antimicrobial mechanisms of GH12, DAP and CHX is thought to be binding to bacterial surface by the action of electric charge in all cases. However, the next interaction of disruption of bacterial cells followed by binding is different, which may determine the potential for resistance development (Gilbert and Moore, 2005). CHX consists of two cationic groups and one hydrophobic bridging structure, and the hydrophobic region is 6 carbons long and inflexible and incapable of folding sufficiently to interdigitate into the bilayer (Gilbert and Moore, 2005). In contrast, GH12 and DAP are both AMPs and have similar antimicrobial targets, phospholipid bilayers, and their second step of bactericidal action is believed to form pores on the cell membrane (Wang Y. et al., 2017; Heidary et al., 2018). On the other hand, WGS analysis also demonstrated that the new gene of COCC33-14R (gene1782) is related to integral components of the membrane. According to the two pieces of evidence above, the resistance of COCC33-14R was probably associated with changes in the cell envelope, and major differences in antimicrobial mechanisms between AMPs and CHX. Second, the metabolism of the GH12-resistant strain was reduced after continuous exposure to GH12. Antibiotic resistance is always associated with fitness cost, which is typically observed as a reduced bacterial growth rate (Andersson and Hughes, 2010), which is consistent with the present study. In a recent study, DAP-resistant S. mitis also possessed a decreased growth rate and a decreased growth biomass compared to DAP-susceptible S. mitis (Parrett et al., 2020). WGS demonstrated that the missing gene in COCC33-14R (rpsN) was related to bacterial metabolism and growth and commonly existed in many bacteria, including streptococci (Makthal et al., 2020), and the absence of rpsN could result in a defective 30S subunit of the ribosome and then affect translation (Nanamiya and Kawamura, 2010). Although the CDS, which encodes a hypothetical protein, needs further evaluation, based on this explicable evidence, we deduced that the consecutive sublethal GH12 pressure selected for the gene1782 mutation, to change the cell envelope of COCC33-14R to induce its resistance, and in the meantime, the rpsN was missing in COCC33-14R’s genome to increase its fitness cost. However, further investigations are needed, such as thickness, permeability and specific cell membrane components of COCC33-14R. Additionally, further studies on metabolomics, transcriptomics, and genomics are necessary to understand the connection between this type of resistance and metabolic changes.
In conclusion, stable resistant mutants of caries-related streptococci could hardly be selected by exposure to consecutive sublethal GH12, but the risk still exists. The resistance mechanism of COCC33-14R is probably related to changes in the cell envelope. To avoid the emergence of GH12 resistance, more studies with different variables, such as selection methods, concentrations and drug treatment times are needed.
Data availability statement
The data presented in the study are deposited in the Genome Database of NCBI (https://www.ncbi.nlm.nih.gov/genome/) repository, and are under BioProject ID PRJNA862258 with accession numbers: CP101984 (Streptococcus mutans UA159), CP101985 (Streptococcus mutans COCC33-14R) and CP101986 (Streptococcus mutans COCC33-14).
Author contributions
XL: The conception and design of the study, acquisition of data, analysis and interpretation of data, drafting the article. YW: The conception and design of the study, acquisition of data, analysis and interpretation of data. XJ: Acquisition of data. YZ: Acquisition of data. XZ: Analysis and interpretation of data. JW: Analysis and interpretation of data. NT: Analysis and interpretation of data. LZ: The conception and design of the study, analysis and interpretation of data. All authors contributed to revise it critically for important intellectual content and approve the final version of the manuscript. All authors contributed to the article and approved the submitted version.
Funding
This work was supported by the National Natural Science Foundation of China (grant number 81970931).
Conflict of interest
The authors declare that the research was conducted in the absence of any commercial or financial relationships that could be construed as a potential conflict of interest.
Publisher’s note
All claims expressed in this article are solely those of the authors and do not necessarily represent those of their affiliated organizations, or those of the publisher, the editors and the reviewers. Any product that may be evaluated in this article, or claim that may be made by its manufacturer, is not guaranteed or endorsed by the publisher.
Supplementary material
The Supplementary Material for this article can be found online at: https://www.frontiersin.org/articles/10.3389/fcimb.2022.991938/full#supplementary-material
References
Aida, K. L., Kreling, P. F., Caiaffa, K. S., Calixto, G. M. F., Chorilli, M., Spolidorio, D. M., et al. (2018). Antimicrobial peptide-loaded liquid crystalline precursor bioadhesive system for the prevention of dental caries. Int. J. Nanomed 13, 3081–3091. doi: 10.2147/IJN.S155245
Andersson, D. I., Hughes, D. (2010). Antibiotic resistance and its cost: is it possible to reverse resistance? Nat. Rev. Microbiol. 8 (4), 260–271. doi: 10.1038/nrmicro2319
Besemer, J., Borodovsky, M. (2005). GeneMark: web software for gene finding in prokaryotes, eukaryotes and viruses. Nucleic Acids Res. 33, W451–W454. doi: 10.1093/nar/gki487
Blair, J. M., Webber, M. A., Baylay, A. J., Ogbolu, D. O., Piddock, L. J. (2015). Molecular mechanisms of antibiotic resistance. Nat. Rev. Microbiol. 13 (1), 42–51. doi: 10.1038/nrmicro3380
Buzalafa, M. A. R., Pessanc, J. P., Honóriob, H. M., Cated, J. (2011). Mechanisms of action of fluoride for caries control. Monogr. Oral. Sci. 22, 97–114. doi: 10.1159/000325151
Carey, C. M. (2014). Focus on fluorides: update on the use of fluoride for the prevention of dental caries. J. Evid Based Dent. Pract. 14 Suppl, 95–102. doi: 10.1016/j.jebdp.2014.02.004
Castillo, A., Liebana, J., Lopez, E., Baca, P., Liebana, J. M., Liebana, M. J., et al. (2006). Interference of antibiotics in the growth curves of oral streptococci. Int. J. Antimicrob. Agents 27 (3), 263–266. doi: 10.1016/j.ijantimicag.2005.10.017
CDC (2019). Antibiotic resistance threats in the united states (Atlanta, GA: U.S: Department of Health and Human Services, CDC).
Chan, P. P., Lowe, T. M. (2019). tRNAscan-SE: Searching for tRNA Genes in Genomic Sequences. Methods Mol. Biol. 1962, 1–14. doi: 10.1007/978-1-4939-9173-0_1
Cheng, X., Redanz, S., Treerat, P., Qin, H., Choi, D., Zhou, X., et al. (2020). Magnesium-dependent promotion of H2O2 production increases ecological competitiveness of oral commensal streptococci. J. Dent. Res. 99 (7), 847–854. doi: 10.1177/0022034520912181
Cheng, L., Zhang, K., Zhou, C. C., Weir, M. D., Zhou, X. D., Xu, H. H. (2016). One-year water-ageing of calcium phosphate composite containing nano-silver and quaternary ammonium to inhibit biofilms. Int. J. Oral. Sci. 8 (3), 172–181. doi: 10.1038/ijos.2016.13
Ciancio, S. G., Mather, M. L., Bunnell, H. L. (1975). Clinical evaluation of a quaternary ammonium-containing mouthrinse. J. Periodontol 46 (7), 397–401. doi: 10.1902/jop.1975.46.7.397
CLSI (2018). “Methods for antimicrobial susceptibility testing of anaerobic bacteria,” in Approved standard-ninth edition (Pennsylvania (USA: Clinical and Laboratory Standards Institute).
Copin, R., Sause, W. E., Fulmer, Y., Balasubramanian, D., Dyzenhaus, S., Ahmed, J. M., et al. (2019). Sequential evolution of virulence and resistance during clonal spread of community-acquired methicillin-resistant staphylococcus aureus. Proc. Natl. Acad. Sci. U.S.A. 116 (5), 1745–1754. doi: 10.1073/pnas.1814265116
da Silva, B. R., de Freitas, V. A., Nascimento-Neto, L. G., Carneiro, V. A., Arruda, F. V., de Aguiar, A. S., et al. (2012). Antimicrobial peptide control of pathogenic microorganisms of the oral cavity: a review of the literature. Peptides 36 (2), 315–321. doi: 10.1016/j.peptides.2012.05.015
De Lastours, V., Fantin, B. (2015). Impact of fluoroquinolones on human microbiota. focus on the emergence of antibiotic resistance. Future Microbiol. 10 (7), 1241–1255. doi: 10.2217/fmb.15.40
Delcher, A. L., Bratke, K. A., Powers, E. C., Salzberg, S. L. (2007). Identifying bacterial genes and endosymbiont DNA with glimmer. Bioinformatics 23 (6), 673–679. doi: 10.1093/bioinformatics/btm009
Dhotre, S. V., Jahagirdar, V. L., Davane, M. S., Nagoba, B. S. (2016). Susceptibility, resistance and treatment strategy for infections caused by viridans group streptococci - a review. JKIMSU 5 (4), 1–9.
Diaz-Torres, M. L., Villedieu, A., Hunt, N., McNab, R., Spratt, D. A., Allan, E., et al. (2006). Determining the antibiotic resistance potential of the indigenous oral microbiota of humans using a metagenomic approach. FEMS Microbiol. Lett. 258 (2), 257–262. doi: 10.1111/j.1574-6968.2006.00221.x
Ding, Y., Wang, W., Fan, M., Tong, Z., Kuang, R., Jiang, W., et al. (2014). Antimicrobial and anti-biofilm effect of Bac8c on major bacteria associated with dental caries and streptococcus mutans biofilms. Peptides 52, 61–67. doi: 10.1016/j.peptides.2013.11.020
Espinosa-Cristóbal, L. F., Martínez-Castañón, G. A., Martínez-Martínez, R. E., Loyola-Rodríguez, J. P., Patiño-Marín, N., Reyes-Macías, J. F., et al. (2012). Antimicrobial sensibility of streptococcus mutans serotypes to silver nanoparticles. Mater Sci. Eng. C-Mater 32 (4), 896–901. doi: 10.1016/j.msec.2012.02.009
Fernandez, L., Hancock, R. E. (2012). Adaptive and mutational resistance: role of porins and efflux pumps in drug resistance. Clin. Microbiol. Rev. 25 (4), 661–681. doi: 10.1128/CMR.00043-12
Garcia-de-la-Maria, C., Pericas, J. M., Del Rio, A., Castaneda, X., Vila-Farres, X., Armero, Y., et al. (2013). Early in vitro and in vivo development of high-level daptomycin resistance is common in mitis group streptococci after exposure to daptomycin. Antimicrob. Agents Chemother. 57 (5), 2319–2325. doi: 10.1128/AAC.01921-12
Gilbert, P., Moore, L. E. (2005). Cationic antiseptics: diversity of action under a common epithet. J. Appl. Microbiol. 99 (4), 703–715. doi: 10.1111/j.1365-2672.2005.02664.x
Gjermo, P. (1974). Chlorhexidine in dental practice. J. Clin. Periodontol 1 (3), 143–152. doi: 10.1111/j.1600-051x.1974.tb01250.x
Gravesen, A., Jydegaard Axelsen, A. M., Mendes da Silva, J., Hansen, T. B., Knochel, S. (2002). Frequency of bacteriocin resistance development and associated fitness costs in listeria monocytogenes. Appl. Environ. Microbiol. 68 (2), 756–764. doi: 10.1128/aem.68.2.756-764.2002
Heidary, M., Khosravi, A. D., Khoshnood, S., Nasiri, M. J., Soleimani, S., Goudarzi, M. (2018). Daptomycin. J. Antimicrob. Chemother. 73 (1), 1–11. doi: 10.1093/jac/dkx349
Hong, C. H. L., Hu, S., Haverman, T., Stokman, M., Napeñas, J. J., Braber, J. B., et al. (2018). A systematic review of dental disease management in cancer patients. Support Care Cancer 26 (1), 155–174. doi: 10.1007/s00520-017-3829-y
Hong, J., Hu, J., Ke, F. (2016). Experimental induction of bacterial resistance to the antimicrobial peptide tachyplesin I and investigation of the resistance mechanisms. Antimicrob. Agents Chemother. 60 (10), 6067–6075. doi: 10.1128/AAC.00640-16
Huang, X., Browngardt, C. M., Jiang, M., Ahn, S. J., Burne, R. A., Nascimento, M. M. (2018). Diversity in antagonistic interactions between commensal oral streptococci and streptococcus mutans. Caries Res. 52 (1-2), 88–101. doi: 10.1159/000479091
Huang, X., Exterkate, R. A. M., ten Cate, J. M. (2012). Factors associated with alkali production from arginine in dental biofilms. J. Dent. Res. 91 (12), 1130–1134. doi: 10.1177/0022034512461652
Ikai, H., Odashima, Y., Kanno, T., Nakamura, K., Shirato, M., Sasaki, K., et al. (2013). In vitro evaluation of the risk of inducing bacterial resistance to disinfection treatment with photolysis of hydrogen peroxide. PloS One 8 (11), e81316. doi: 10.1371/journal.pone.0081316
Jiang, W., Wang, Y., Luo, J., Li, X., Zhou, X., Li, W., et al. (2018). Effects of antimicrobial peptide GH12 on the cariogenic properties and composition of a cariogenic multispecies biofilm. Appl. Environ. Microbiol. 84 (24), e01423–e01418. doi: 10.1128/aem.01423-18
Johansen, J. R., Gjermo, P., Eriksen, H. M. (1975). Effect of 2-years’ use of chlorhexidine-containing dentifrices on plaque, gingivitis, and caries. Scand. J. Dent. Res. 83 (5), 288–292. doi: 10.1111/j.1600-0722.1975.tb00440.x
Kaatz, G. W., Lundstrom, T. S., Seo, S. M. (2006). Mechanisms of daptomycin resistance in Staphylococcus aureus. Int. J. Antimicrob. Agents 28 (4), 280–287. doi: 10.1016/j.ijantimicag.2006.05.030
Kampf, G. (2018a). Biocidal agents used for disinfection can enhance antibiotic resistance in gram-negative species. Antibiotics 7 (4), 110. doi: 10.3390/antibiotics7040110
Kampf, G. (2018b). “Chlorhexidine digluconate,” in Antiseptic Stewardship: Biocide Resistance and Clinical Implications. (Switzerland: Springer Nature), 429–534. doi: 10.1007/978-3-319-98785-9_13
Kampf, G. (2019). Antibiotic resistance can be enhanced in gram-positive species by some biocidal agents used for disinfection. Antibiotics 8 (1), 13. doi: 10.3390/antibiotics8010013
Larioza, J., Brown, R. B., Girard, A. (2011). Clinical experience with daptomycin for outpatient parenteral antibiotic therapy. Am. J. Med. Sci. 342 (6), 486–488. doi: 10.1097/MAJ.0b013e31821e1e6b
Lee, T.-H., Hall, K. N., Aguilar, M.-I. (2016). Antimicrobial peptide structure and mechanism of action: A focus on the role of membrane structure. Curr. Top. Med. Chem. 16, 25–39. doi: 10.2174/1568026615666150703121700
Lee, S. Y., Lee, S. Y. (2019). Susceptibility of oral streptococci to chlorhexidine and cetylpyridinium chloride. Biocontrol Sci. 24 (1), 13–21. doi: 10.4265/bio.24.13
Liao, Y., Brandt, B. W., Li, J. Y., Crielaard, W., Van Loveren, C., Deng, D. M. (2017). Fluoride resistance in streptococcus mutans: a mini review. J. Oral. Microbiol. 9 (1), 1344509. doi: 10.1080/20002297.2017.1344509
Lima, A. R., Ganguly, T., Walker, A. R., Acosta, N., Francisco, P. A., Pileggi, R., et al. (2020). Phenotypic and genotypic characterization of streptococcus mutans strains isolated from endodontic infections. J. Endod. 46 (12), 1876–1883. doi: 10.1016/j.joen.2020.09.002
Lipták, L., Szabó, K., Nagy, G., Márton, S., Madléna, M. (2018). Microbiological changes and caries-preventive effect of an innovative varnish containing chlorhexidine in orthodontic patients. Caries Res. 52 (4), 272–278. doi: 10.1159/000486140
Loyola-Rodriguez, J. P., Ponce-Diaz, M. E., Loyola-Leyva, A., Garcia-Cortes, J. O., Medina-Solis, C. E., Contreras-Ramire, A. A., et al. (2018). Determination and identification of antibiotic-resistant oral streptococci isolated from active dental infections in adults. Acta Odontol Scand. 76 (4), 229–235. doi: 10.1080/00016357.2017.1405463
Lu, W., Wu, F., Zhou, X., Wu, L., Li, M., Ren, B., et al. (2015). Isolation and identification of aerobic and facultative anaerobic bacteria in the oral cavity. Nan Fang Yi Ke Da Xue Xue Bao 35, 1710–1714. doi: 10.3969/j.issn.1673-4254.2015.12.09
Mai, S., Mauger, M. T., Niu, L. N., Barnes, J. B., Kao, S., Bergeron, B. E., et al. (2017). Potential applications of antimicrobial peptides and their mimics in combating caries and pulpal infections. Acta Biomater 49, 16–35. doi: 10.1016/j.actbio.2016.11.026
Makthal, N., Do, H., Wendel, B. M., Olsen, R. J., Helmann, J. D., Musser, J. M., et al. (2020). Group a streptococcus AdcR regulon participates in bacterial defense against host-mediated zinc sequestration and contributes to virulence. Infect. Immun. 88 (8), e00097–e00020. doi: 10.1128/IAI.00097-20
Melo, M. A., Cheng, L., Weir, M. D., Hsia, R. C., Rodrigues, L. K., Xu, H. H. (2013). Novel dental adhesive containing antibacterial agents and calcium phosphate nanoparticles. J. BioMed. Mater Res. B Appl. Biomater 101 (4), 620–629. doi: 10.1002/jbm.b.32864
Mishra, N. N., Tran, T. T., Arias, C. A., Seepersaud, R., Sullam, P. M., Bayer, A. S. (2020). Strain-specific adaptations of streptococcus mitis-oralis to serial in vitro passage in daptomycin (DAP): genotypic and phenotypic characteristics. Antibiotics 9 (8), 520. doi: 10.3390/antibiotics9080520
Moffatt, J. H., Harper, M., Boyce, J. D. (2019). “Mechanisms of polymyxin resistance,” in Polymyxin antibiotics: From laboratory bench to bedside. Eds. Li, J., Nation, R. L., Kaye, K.S. (Switzerland: Springer Nature), 55–71. doi: 10.1007/978-3-030-16373-0_5
Munita, J. M., Arias, C. A. (2016). Mechanisms of antibiotic resistance. Microbiol. Spectr. 4 (2), VMBF-0016-2015. doi: 10.1128/microbiolspec.VMBF-0016-2015
Nanamiya, H., Kawamura, F. (2010). Towards an elucidation of the roles of the ribosome during different growth phases in bacillus subtilis. Biosci. Biotechnol. Biochem. 74 (3), 451–461. doi: 10.1271/bbb.90859
Parrett, A., Reed, J. M., Gardner, S. G., Mishra, N. N., Bayer, A. S., Powers, R., et al. (2020). Metabolic changes associated with adaptive resistance to daptomycin in streptococcus mitis-oralis. BMC Microbiol. 20 (1), 162. doi: 10.1186/s12866-020-01849-w
Pasquantonio, G., Condo, S., Cerroni, L., Bikiqu, L., Nicoletti, M., Prenna, M., et al. (2012). Antibacterial activity and various antibiotics against oral streptocci isolated in the oral cavity. Int. J. Immunopathol. Pharmacol. 25 (3), 805–809. doi: 10.1177/039463201202500331
Pasquantonio, G., Greco, C., Prenna, M., Ripa, C., Vitali, L. A., Petrelli, D., et al. (2008). Antibacterial activity and anti-biofilm effect of chitosan against strains of streptococcus mutans isolated in dental plaque. Int. J. Immunopathol. Pharmacol. 21 (4), 993–997. doi: 10.1177/039463200802100424
Petersen, P. E., Lennon, M. A. (2004). Effective use of fluorides for the prevention of dental caries in the 21st century: the WHO approach. Community Dent Oral. Epidemiol. 32 (5), 319–321. doi: 10.1111/j.1600-0528.2004.00175.x
Pitts, N. B., Zero, D. T., Marsh, P. D., Ekstrand, K., Weintraub, J. A., Ramos-Gomez, F., et al. (2017). Dental caries. Nat. Rev. Dis. Primers 3, 1–16. doi: 10.1038/nrdp.2017.30
Schiøtt, C. R., Löe, H. (1972). The sensitivity of oral streptococci to chlorhexidine. J. Periodontol Res. 7, 192–194. doi: 10.1111/j.1600-0765.1972.tb00645.x
Slade, G. D., Bailie, R. S., Roberts-Thomson, K., Leach, A. J., Raye, I., Endean, C., et al. (2011). Effect of health promotion and fluoride varnish on dental caries among Australian aboriginal children: results from a community-randomized controlled trial. Community Dent. Oral. Epidemiol. 39 (1), 29–43. doi: 10.1111/j.1600-0528.2010.00561.x
Slot, D. E., Vaandrager, N. C., Van Loveren, C., Van Palenstein Helderman, W. H., Van der Weijden, G. A. (2011). The effect of chlorhexidine varnish on root caries: a systematic review. Caries Res. 45 (2), 162–173. doi: 10.1159/000327374
Suppiger, S., Astasov-Frauenhoffer, M., Schweizer, I., Waltimo, T., Kulik, E. M. (2020). Tolerance and persister formation in oral streptococci. Antibiotics 9 (4), 167. doi: 10.3390/antibiotics9040167
Su, M., Yao, S., Gu, L., Huang, Z., Mai, S. (2018). Antibacterial effect and bond strength of a modified dental adhesive containing the peptide nisin. Peptides 99, 189–194. doi: 10.1016/j.peptides.2017.10.003
Sztukowska, M. N., Roky, M., Demuth, D. R. (2019). Peptide and non-peptide mimetics as potential therapeutics targeting oral bacteria and oral biofilms. Mol. Oral. Microbiol. 34 (5), 169–182. doi: 10.1111/omi.12267
Takahashi, N., Nyvad, B. (2011). The role of bacteria in the caries process: ecological perspectives. J. Dent. Res. 90 (3), 294–303. doi: 10.1177/0022034510379602
Tran, T. T., Munita, J. M., Arias, C. A. (2015). Mechanisms of drug resistance: daptomycin resistance. Ann. NY Acad. Sci.1354, 32–53. doi: 10.1111/nyas.12948
Tu, H., Fan, Y., Lv, X., Han, S., Zhou, X., Zhang, L. (2016). Activity of synthetic antimicrobial peptide GH12 against oral streptococci. Caries Res. 50 (1), 48–61. doi: 10.1159/000442898
Verspecht, T., Rodriguez Herrero, E., Khodaparast, L., Khodaparast, L., Boon, N., Bernaerts, K., et al. (2019). Development of antiseptic adaptation and cross-adapatation in selected oral pathogens in vitro. Sci. Rep. 9 (1), 8326. doi: 10.1038/s41598-019-44822-y
Walsh, T., Worthington, H. V., Glenny, A. M., Marinho, V. C., Jeroncic, A. (2019). Fluoride toothpastes of different concentrations for preventing dental caries. Cochrane Database Syst. Rev. 3, CD007868. doi: 10.1002/14651858.CD007868.pub3
Wand, M. E., Bock, L. J., Bonney, L. C., Sutton, J. M. (2017). Mechanisms of increased resistance to chlorhexidine and cross-resistance to colistin following exposure of klebsiella pneumoniae cinical iolates to clorhexidine. Antimicrob. Agents Chemother. 61 (1), e01162–e01116. doi: 10.1128/AAC.01162-16
Wang, Y., Fan, Y., Zhou, Z., Tu, H., Ren, Q., Wang, X., et al. (2017). De novo synthetic short antimicrobial peptides against cariogenic bacteria. Arch. Oral. Biol. 80, 41–50. doi: 10.1016/j.archoralbio.2017.03.017
Wang, Y., Wang, X., Jiang, W., Wang, K., Luo, J., Li, W., et al. (2018a). Antimicrobial peptide GH12 suppresses cariogenic virulence factors of streptococcus mutans. J. Oral. Microbiol. 10 (1), 1442089. doi: 10.1080/20002297.2018.1442089
Wang, S., Wang, H., Ren, B., Li, H., Weir, M. D., Zhou, X., et al. (2017). Do quaternary ammonium monomers induce drug resistance in cariogenic, endodontic and periodontal bacterial species? Dent. Mater 33 (10), 1127–1138. doi: 10.1016/j.dental.2017.07.001
Wang, Y., Zeng, Y., Wang, Y., Li, H., Yu, S., Jiang, W., et al. (2018b). Antimicrobial peptide GH12 targets streptococcus mutans to arrest caries development in rats. J. Oral. Microbiol. 11 (1), 1549921. doi: 10.1080/20002297.2018.1549921
Westergren, G., Emilson, C.-G. (1980). In vitro development of chlorhexidine resistance in streptococcus sanguis and its transmissibility by genetic transformation. Scand. J. Dent. Res. 88, 236–243. doi: 10.1111/j.1600-0722.1980.tb01220.x
Whelton, H. P., Spencer, A. J., Do, L. G., Rugg-Gunn, A. J. (2019). Fluoride revolution and dental caries: Evolution of policies for global use. J. Dent. Res. 98 (8), 837–846. doi: 10.1177/0022034519843495
Wright, G. D. (2011). Molecular mechanisms of antibiotic resistance. Chem. Commun. (Camb) 47 (14), 4055–4061. doi: 10.1039/c0cc05111j
Keywords: antimicrobial peptide, drug resistance, dental caries, streptococci, whole genome sequencing
Citation: Li X, Wang Y, Jiang X, Zeng Y, Zhao X, Washio J, Takahashi N and Zhang L (2022) Investigation of drug resistance of caries-related streptococci to antimicrobial peptide GH12. Front. Cell. Infect. Microbiol. 12:991938. doi: 10.3389/fcimb.2022.991938
Received: 12 July 2022; Accepted: 22 August 2022;
Published: 08 September 2022.
Edited by:
Steven Gill, University of Rochester, United StatesReviewed by:
Dorota Kopycka-Kedzierawski, University of Rochester, United StatesAninda Mazumdar, Academy of Sciences of the Czech Republic (ASCR), Czechia
Copyright © 2022 Li, Wang, Jiang, Zeng, Zhao, Washio, Takahashi and Zhang. This is an open-access article distributed under the terms of the Creative Commons Attribution License (CC BY). The use, distribution or reproduction in other forums is permitted, provided the original author(s) and the copyright owner(s) are credited and that the original publication in this journal is cited, in accordance with accepted academic practice. No use, distribution or reproduction is permitted which does not comply with these terms.
*Correspondence: Linglin Zhang, emhsbF9zY0AxNjMuY29t