- 1Department of Biochemistry, Genetics and Microbiology, University of Pretoria, Pretoria, South Africa
- 2Institute for Sustainable Malaria Control, School of Health Systems and Public Health, University of Pretoria, Pretoria, South Africa
Malaria elimination is dependent on the ability to target both the pathogenic and transmissible stages of the human malaria parasite, Plasmodium falciparum. These forms of the parasite are differentiated by unique developmental stages, each with their own biological mechanisms and processes. These individual stages therefore also respond differently to inhibitory compounds, and this complicates the discovery of multistage active antimalarial agents. The search for compounds with transmission-blocking activity has focused on screening for activity on mature gametocytes, with only limited descriptions available for the activity of such compounds on immature stage gametocytes. This therefore poses a gap in the profiling of antimalarial agents for pan-reactive, multistage activity to antimalarial leads. Here, we optimized an effective and robust strategy for the simple and cost-effective description of the stage-specific action of gametocytocidal antimalarial compounds.
Introduction
Increased resistance to currently available antimalarials continues to pose a threat to worldwide malaria elimination efforts and motivates the continued discovery of new antimalarial agents (World Health Organisation, 2020). Target profiling of candidate antimalarial agents now routinely requires evidenced killing of multiple life cycle stages of malaria parasites (Burrows et al., 2017). As such, antimalarial lead candidates are firstly profiled for activity against asexual blood stage (ABS) parasites, addressing the therapeutic necessity of killing parasite stages associated with morbidity and mortality. However, to be useful in and contribute to malaria elimination strategies, antimalarial candidates with such established ABS activity are typically profiled for additional abilities, including being able to block human-to-mosquito transmission of the parasite. This would thereby curb the spread of the disease, with various strategies proposed to exploit such transmission-blocking agents (Birkholtz et al., 2022).
In the human malaria parasite, Plasmodium falciparum, human-to-mosquito parasite transmission is dependent on the differentiation and development of mature gametocyte forms of the parasite. In this species, gametocyte development is a uniquely prolonged process (~12 days) characterized by five morphologically distinct stages (I–V) (Young et al., 2005), each of which is associated with distinct biological processes (Sinden, 2015; Ngotho et al., 2019; Van der Watt et al., 2022). After the stochastic conversion of ≤10% of P. falciparum ABS parasites to gametocytogenesis, immature gametocytes (stages I–IV) develop and sequester in extravascular environments including the bone marrow (Day et al., 1998; Aguilar et al., 2014). Terminally differentiated, mature falciform gametocytes (stage V) can re-enter the circulatory system and are the only gametocyte stage that can be transmitted back to the mosquito (Sinden, 2015; Meibalan and Marti, 2017; Ngotho et al., 2019). Mature gametocytes can be maintained in circulation for up to 55 days [mean life span, ~5.5 days (Bousema et al., 2010)] and are thereby continuously able to ensure transmission of the parasite in the human population, with only ~103 mature gametocytes required for transmission to occur. Therefore, blocking human-to-mosquito parasite transmission is conceptually associated with the ease of targeting such low numbers of long-lasting mature gametocytes. Additionally, as they are circulating in the blood compartment, they are accessible to pharmacological intervention (Birkholtz et al., 2022).
Profiling of antimalarial candidates with ABS activity for additional transmission-blocking activity is frequently only performed on mature stage V gametocytes (D'alessandro et al., 2016; Plouffe et al., 2016; Van Voorhis et al., 2016; Miguel-Blanco et al., 2017; Paquet et al., 2017; Van Der Watt et al., 2018) or male and female gametes (Miguel-Blanco et al., 2017; Delves et al., 2018; Delves et al., 2019). In both instances, a reduction of oocyst numbers (and by implication sporozoite formation) is used to validate transmission-blocking activity (Vos et al., 2015; Birkholtz et al., 2016). However, such a screening pipeline would be fragmented and would not consider the different gametocyte stages of P. falciparum, each stage of which is associated with differential biological activities (Delves, 2012; Meibalan and Marti, 2017; Ngotho et al., 2019; Schneider and Reece, 2021; Usui and Williamson, 2021). This translates to compounds having variant activity profiles against these different stages of P. falciparum gametocytes (Duffy and Avery, 2013; Miguel-Blanco et al., 2017; Delves et al., 2018; Delves et al., 2019; Abraham et al., 2020). Although the majority of ABS actives do retain activity against immature gametocytes, very few are active against mature gametocytes (Duffy and Avery, 2013). Importantly, not all ABS actives are able to target immature gametocytes. For example, atovaquone, which is potent against ABS parasites, has no activity against immature and mature gametocytes (Plouffe et al., 2016). In these instances, if the entire ABS population is not rapidly cleared, any gametocytes that are formed and not targeted in their immature stages will continuously seed formation of mature gametocytes that will then be transmitted. In such scenarios, transmission will not be blocked, and the parasite will continue to be spread. Moreover, this is of particular concern given the presence of same cycle sexual conversion, i.e., induction of gametocytogenesis within the first cycle of ABS proliferation (Bancells et al., 2019) and evidence that some ABS actives (chloroquine, antifolates, and mefloquine) can shift the parasite population into sexual differentiation and increase the gametocytaemia of immature stages in vivo (Buckling et al., 1999; Price et al., 1999; Sowunmi and Fateye, 2003; Sowunmi et al., 2006; Fehintola et al., 2012; Thommen et al., 2022).
Evaluation of the stage-specific gametocytocidal activity of small molecules has been sparse (Duffy and Avery, 2013; Miguel-Blanco et al., 2017; Delves et al., 2018; Delves et al., 2019; Abraham et al., 2020) and, where these assays have been performed, they made use of extensive, complicated, and expensive manipulation of the gametocyte populations to enrich for specific stages (Adjalley et al., 2011; Lucantoni et al., 2013; Lucantoni et al., 2016; Plouffe et al., 2016). These processes run the risk of compromising gametocyte viability and negate the use of such assays in resource-constrained settings and for robust and reproducible high-throughput applications. Consequently, a more streamlined method is required to allow the stage-specific production of gametocytes with minimal manipulation, to accurately assess the activity of small molecules against immature and mature gametocyte populations. Additionally, the application of such an optimized, streamlined “whole-gametocytocidal” assay beyond proof of principle is required (Lucantoni et al., 2016). Here, we report an optimization of such whole-gametocytocidal assay to allow straightforward, robust, and routine investigation of stage-specific gametocytocidal activity of antimalarial candidates. We validate this strategy with key antimalarial lead candidates with known ABS activity and confirm the importance of characterising any new antimalarial lead compound for stage-specific action against various gametocyte stages including immature gametocytes. With this, we complete the gap in the current profiling of antimalarial candidates with potential transmission-blocking ability by including evaluation of stage-specific gametocytocidal activity.
Materials and Methods
Ethics Statement
Parasitology work and volunteer human blood donation at the University of Pretoria are covered under ethical approval from the Health Sciences Ethics Committee (506/2018) and Natural and Agricultural Sciences Ethics Committee (180000094).
In Vitro Parasite Culturing
NF54-Pfs16-GFP-luc P. falciparum asexual parasites [kind gift from David Fidock, Columbia University, USA (Adjalley et al., 2011)] were maintained at 37°C in A+/O+ human erythrocytes at 5% hematocrit in complete culture medium under hypoxic conditions as previously described (Reader et al., 2015). Asexual parasites were synchronized at least twice at 12 h intervals with 5% (w/v) D-sorbitol. This staggered synchronization resulted in a parasite population containing >97% ring-stage parasites that were all detected and quantified morphologically to be within a tight developmental window of 5- to 10-h post-invasion. Such age binning and compartmentalization of these ring-stage parasites were performed as described before (Van Biljon et al., 2018; Van Biljon et al., 2019). All cultures were maintained with daily medium changes and synchronicity evaluated with Giemsa-stained thin smear stage binning under 1,000× magnification.
Stage-Specific Gametocyte Production
Gametocytogenesis was induced on a tightly synchronised (>97% rings) asexual parasite culture (0.5% parasitemia and 6% hematocrit) with a combination of nutrient starvation and a decrease in hematocrit, as previously described (Reader et al., 2015). Gametocyte development was allowed to occur in culture medium prepared as for growth of asexual parasites but without additional glucose supplementation (day −3) under hypoxic conditions, stationary growth, with daily media replacement (from day −1) and parasites/gametocytes were morphologically monitored as per recent molecular descriptors (Brancucci et al., 2018; Dixon and Tilley, 2021). The hematocrit was reduced to 4% after 72 h (day 0), mimicking anemic conditions in the patient. For immature gametocytes (stage II/III), cultures were exposed to 50 mM N-acetyl glucosamine (NAG) on days 1–4 to eliminate residual asexual parasites and harvested at days 5–6 (Figure 1). For mature (stage V) gametocytes, NAG treatment only occurred from days 3–7 and harvested at day 13 (Figure 1).
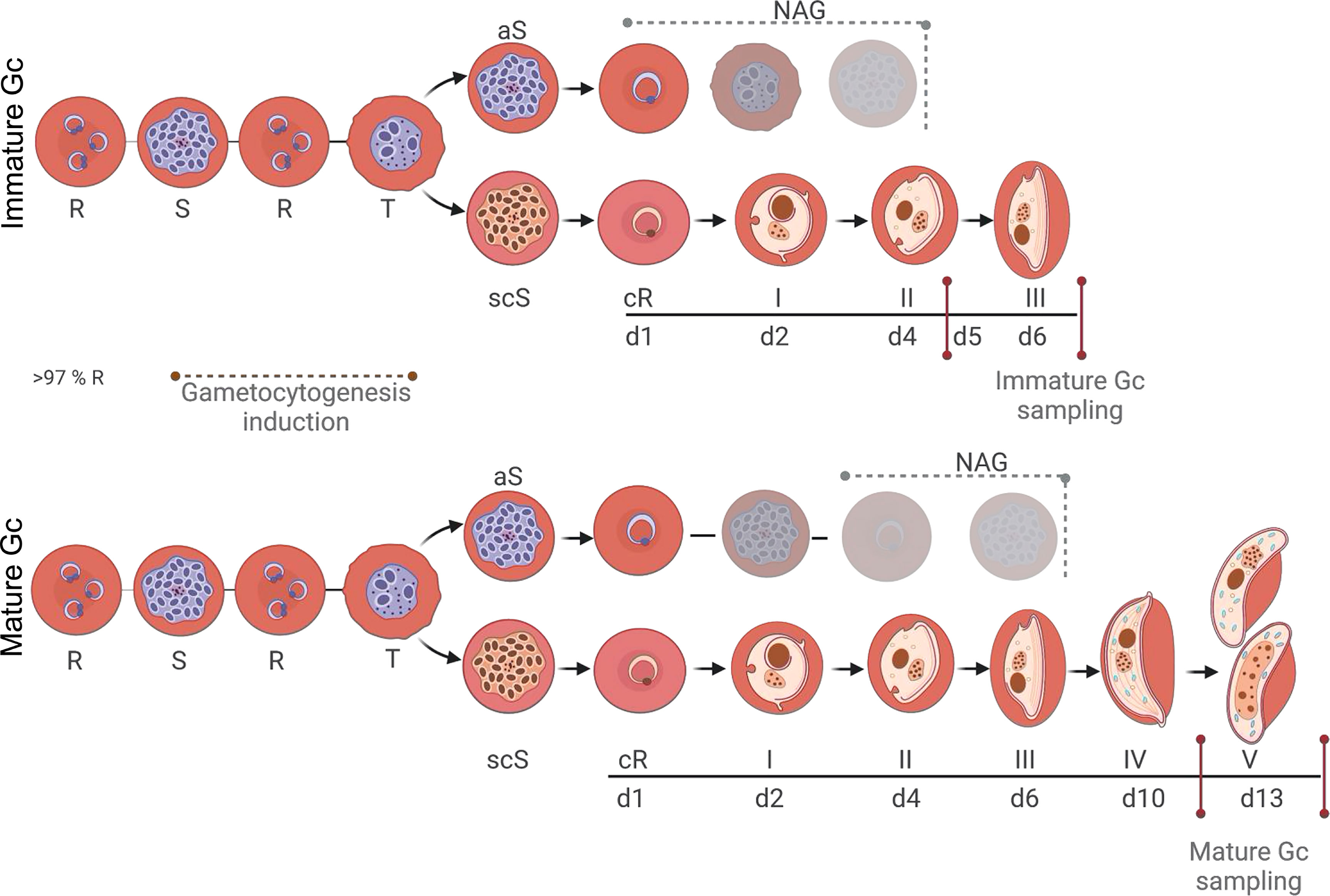
Figure 1 P. falciparum stage-specific gametocyte production and development. Asexual parasites (0.5% parasitemia, 6% hematocrit) were D-sorbitol synchronized for two consecutive cycles to ensure a highly synchronized (>97%) ring population. Initiation of gametocytogenesis (same cycle conversion) was induced on day 0 by a decrease in hematocrit, mimicking anemic conditions. Asexual parasites were eliminated with N-acetyl glucosamine treatment from days 1 to 4 for immature gametocytes and days 3 to 7 for mature gametocytes. Assays were performed on days 5–6 or 13 for immature and mature gametocytes, respectively. R-ring, S-schizont, T-trophozoite, aS-asexual schizont, scS-sexual committed schizont, cR-committed ring, Gc-gametocyte. Created and modified with BioRender.com.
Male Gamete Formation Assay
The male exflagellation inhibition assay (EIA) was performed as described previously (Coetzee et al., 2020; Reader et al., 2021) by capturing the temporal movement of exflagellation centers by video microscopy. Mature PfNF54 gametocytes (>95% stage V) were treated with 2 µM compound [with methylene blue (MB) as inhibition control at 10 µM] in complete culture media [final dimethyl sulfoxide (DMSO) concentration of <0.1% (v/v), 50% (v/v) A+ male human serum] for 48 h at 37°C under hypoxic conditions. Male gametogenesis was induced on these populations with 100 µM xanthurenic acid at room temperature for 16 min and exflagellation was measured in a Neubauer chamber. Movement was recorded by video microscopy (Carl Zeiss NT 6V/10 W Stab microscope, MicroCapture camera, 10× magnification) and quantified semiautomatically on 16 videos of 8–10 s each, captured between 16 and 24 min after incubation at 30 s intervals. The total exflagellating centers per treatment were quantified using ICY (open-source imaging software GPLv3) normalized to an untreated control.
Viability Determination With Hydroethidine or Determining Adenosine Triphosphate Levels
The viability of both immature and mature gametocytes was evaluated microscopically by staining treated (DHA, 1× IC50 which equates to 20 nM and 5 µM on immature and mature gametocyte, respectively) and untreated gametocytes with 5 µM hydroethidine (HE) for 2 h at room temperature. After removal of excess dye with 1× PBS washes, parasites were fixed in 0.025% (v/v) paraformaldehyde onto a poly-L-lysine–coated cover slide, overnight at 4°C. Micrographs were generated with a Zeiss 510-Meta confocal laser scanning microscope and a HeNe laser with a 543 nm wavelength to excite HE.
Viability was also confirmed by determining ATP levels as a proxy for metabolic activity as previously described (Reader et al., 2015; Reader et al., 2021). Mature stage gametocytes (>90% stage V) were enriched using density gradient centrifugation in NycoPrep (Axis Shield) and magnetic separation using LS columns and a MidiMacs magnet. Approximately 62,000 gametocytes, in glucose-supplemented complete medium, were added to the well in a final volume of 100 µl. Plates were incubated for periods for 0, 12, 24, or 48 h in a humidified gas chamber (90% N2, 5% O2, and 5% CO2) at 37°C. Subsequently, the BacTiter-Glo™ assay (Promega) was performed according to the manufacturer’s instructions at room temperature in the dark, with assay substrate incubated for 10 min, to detect ATP levels. Bioluminescence was detected at an integration constant of 0.5 s with the GloMax®-Multi Detection System with Instinct® software. MB (20 μM) was included as control.
Stage-Specific Gametocytocidal Assay
Compounds [supplied by the Medicine for Malaria Venture (www.mmv.org), in DMSO] were evaluated after 48 h drug pressure on either immature (stage II/III) or mature (stage V) gametocytes (2% gametocytemia, 1.5% hematocrit) under hypoxic conditions at 37°C. Drug pressure of 48 h allowed standardization and comparison of activity of compounds if measured on additional transmission-blocking assays such as gamete and oocyst formation (Almela et al., 2015; Miguel-Blanco et al., 2017; Coetzee et al., 2020; Reader et al., 2021). Luciferase activity was determined in 30 μl of parasite lysate by adding 30 μl of luciferin substrate (Promega Luciferase Assay System) at room temperature and detection of bioluminescence at an integration constant of 10 s with a GloMax®-Multi Detection System with Instinct® software. All single point screens were performed at 5 µM of the compound. All dose-response analyses were performed for 9–18 concentrations of 2-fold dilutions to determine the inhibitory concentration at which 50% of the gametocyte population was no longer viable (IC50). In both these assays, MB and the P. falciparum phosphatidylinositol-4-kinase inhibitor, MMV390048, were used as internal controls (at 5 µM each). Inhibition was enumerated as luciferase levels compared to control viable gametocytes and the IC50 with non-linear curve fitting and four parametric equations (GraphPad Prism). All assays were performed on three independent biological repeats, each in technical triplicates.
Genotyping of the PfNF54 Reporter Line
ABS parasites obtained from the NF54-Pfs16-GFP-luc reporter line expressing green fluorescent protein luciferase (Adjalley et al., 2011) were harvested and genomic DNA was isolated using Quick-DNA™ MiniPrep kit (ZYMO, Research, USA) according to the manufacturer’s instructions. Correct integration of reporter construct into the cg6 locus was analyzed by PCR amplification of the fragments on each side of the attB integration site. The PCR fragments were amplified using KAPA Taq Ready Mix (Roche).
Results
Streamlined Production of Stage-Stratified, Viable Gametocytes
An essential requirement for the stage-specific evaluation of gametocytocidal activity of new antimalarial compounds is the compartmentalized production of immature (stage II/III) and mature (stage V) gametocytes (Figure 1). Our protocol relied on the use of highly synchronized ring-stage asexual parasites as a starting population (97.1 ± 0.6% synchronicity, all with <6-h age window, n = 50), from which gametocytes were induced (Figure 1). This allowed manipulation of the asexual population, which is less prone to adverse effects due to this treatment, rather than manipulation of the gametocyte population. For instance, magnetic purification of gametocytes, as is typically used in other protocols (Lelievre et al., 2012; Lucantoni et al., 2013; Lucantoni et al., 2016; Plouffe et al., 2016), results in a significant >2-fold drop in viability (P = 0.0004, paired, two-tailed t-test, n = 3) as rapidly as 12-h post-enrichment on stage IV/V gametocytes but is more pronounced at 24 and 48 h (P < 000.1, paired, two-tailed t-test, n = 3) (Figure S1). Importantly, to further ensure pure gametocyte populations, asexual parasites were continuously eliminated from the gametocyte population after induction at optimized exposure times for either immature or mature gametocyte populations (Figure 1), with <1 and 0.2% asexual parasites remaining in the immature (on days 5–6) or mature (day 13) gametocytes, respectively.
Immature gametocytes were harvested only when stringent morphological stage binning (Figure S2) indicated >80% enrichment to stage II/III gametocytes (Figure 2A; 16.5 ± 1.7% stage I, 62.3 ± 3.6% stage II, and 21.2 ± 3.3%, stage III; 826 gametocytes evaluated), with routine gametocytaemias of 3.3 ± 0.16% obtained (n = 81 independent inductions). These stage distributions were highly comparative to those obtained previously but with additional purification steps required (Lucantoni et al., 2013; Plouffe et al., 2016). Immature gametocyte populations containing any stage IV gametocytes were not used in downstream assays to ensure that drug efficacy was evaluated only on stage II/III gametocytes, even after 48-h drug pressure where the typical stage distribution remained at ~82% stage II/III gametocytes. By comparison, a more homogeneous population of mature stage gametocytes was obtained (2.7 ± 0.16% gametocytaemia, n = 50 independent inductions) consisting of >90% stage V gametocytes (10 ± 1.9% stage IV and 90 ± 2.3% stage V; 270 gametocytes counted; Figure 2B) due to continued removal of asexual parasites from day 3 and the natural attainment of endpoint differentiation (Figure 1). This stage distribution in the mature gametocyte population again correlates well with that obtained for other protocols, but without needing the additional purification as used in other protocols, e.g., through magnetic separation (Lucantoni et al., 2013; Plouffe et al., 2016), exposing committed rings to sorbitol treatment (Plouffe et al., 2016) or gradient separations (Lelievre et al., 2012), both during the gametocytogenesis process or downstream during the actual use of these gametocytes in gametocytocidal assays.
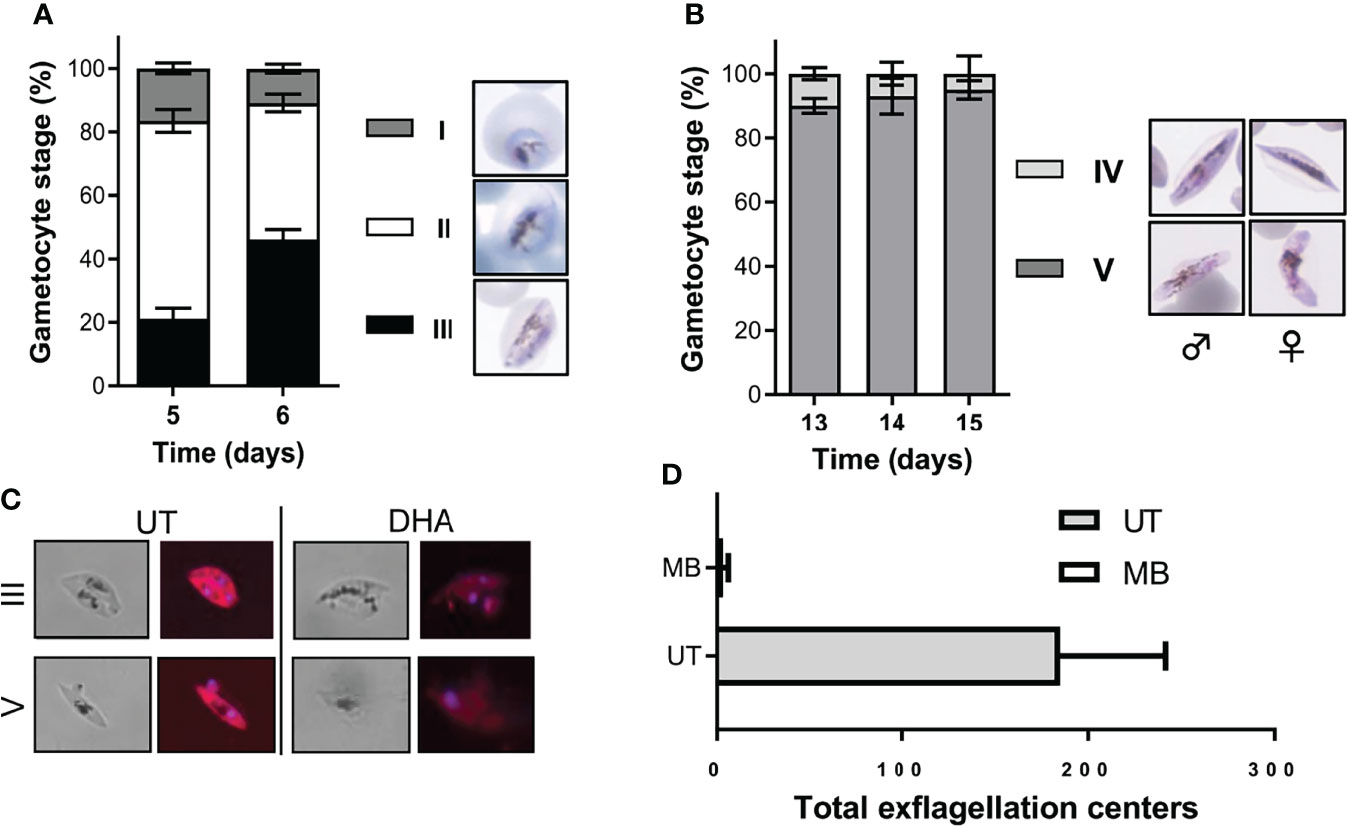
Figure 2 Morphological stage distribution and viability confirmation of immature and mature gametocytes. Quantification of the stage distribution of (A) immature gametocytes indicated at >80% enrichment to stage II/III gametocytes and (B) >90% stage V mature gametocytes on the day of assay. N > 30 individual exp, ~500 red blood cell counted/exp ± S.E. (C) Hydroethidine-stained confocal images confirmed the viability of untreated gametocytes and the “dead” phenotype observed following DHA treatment on both immature and mature gametocytes (at 1× IC50 of 20 nM and 5 µM, respectively). Magnification, ×1,000. (D) Viability of mature gametocytes was further evaluated by confirming male exflagellation before initiating any assays (N > 25, ± S.E.). MB, methylene blue (10 µM) treated gametocytes; UT, untreated gametocytes.
Stage-stratified gametocyte populations were produced here at low cost from a single parasite line and, in contrast to previous protocols, required minimal manipulation and no additional purification or enrichment steps. Our gametocyte populations were viable as measured with live-dead staining (Figure 2C) without any detectable morphological abnormalities. Additionally, mature gametocytes produced in this manner were fully functional and able to produce high numbers of exflagellating male gametes (15–20 exflagellation centers per field, 16 fields counted, average of 184.8 ± 56.7 total exflagellation centers per experiment; Figure 2D), comparable to previous work (13–50 exflagellation centers per field) (Delves et al., 2013).
Evaluating Gametocytocidal Action on Different Stages of Gametocytes
The immature and mature gametocyte populations produced above were subsequently used to evaluate gametocytocidal action of compounds by detecting differences in luciferase signal after a 48 h incubation period. This signal remained detectable in the NF54-Pfs16-GFP-luc line used to produce the different stages of gametocytes for more than 10 generations (Figure S3). Luciferase expression could be detected throughout gametocytogenesis (Figure 3A) but was expectedly higher in immature gametocytes compared to mature gametocytes, as this is associated with Pfs16 promotor activity that is higher in the immature stages but still active in mature stages (Bruce et al., 1994; Van Biljon et al., 2019). For both the immature and mature gametocytes, a linear relationship between gametocyte numbers and luciferase expression was present (R2 [immature] = 0.9991 and R2 [mature] = 0.9927; Figures 3B, C) with sensitive detection of signal in as little as ~3,000 gametocytes per well (in a 96-well format) for both immature and mature gametocytes. Saturation in the luciferase readout could not be observed at even the highest cell count tested (15 × 103 immature gametocytes and ~50 × 103 mature gametocytes per well), similar to previous reports with luciferase expression (D'alessandro et al., 2016). Overall assay performance indicated high reproducibility with Z′-factors of 0.83 ± 0.02 (immature gametocyte assay; n = 81) and 0.84 ± 0.02 (mature gametocyte assay, n = 50) routinely obtained (Figures 3D, E).
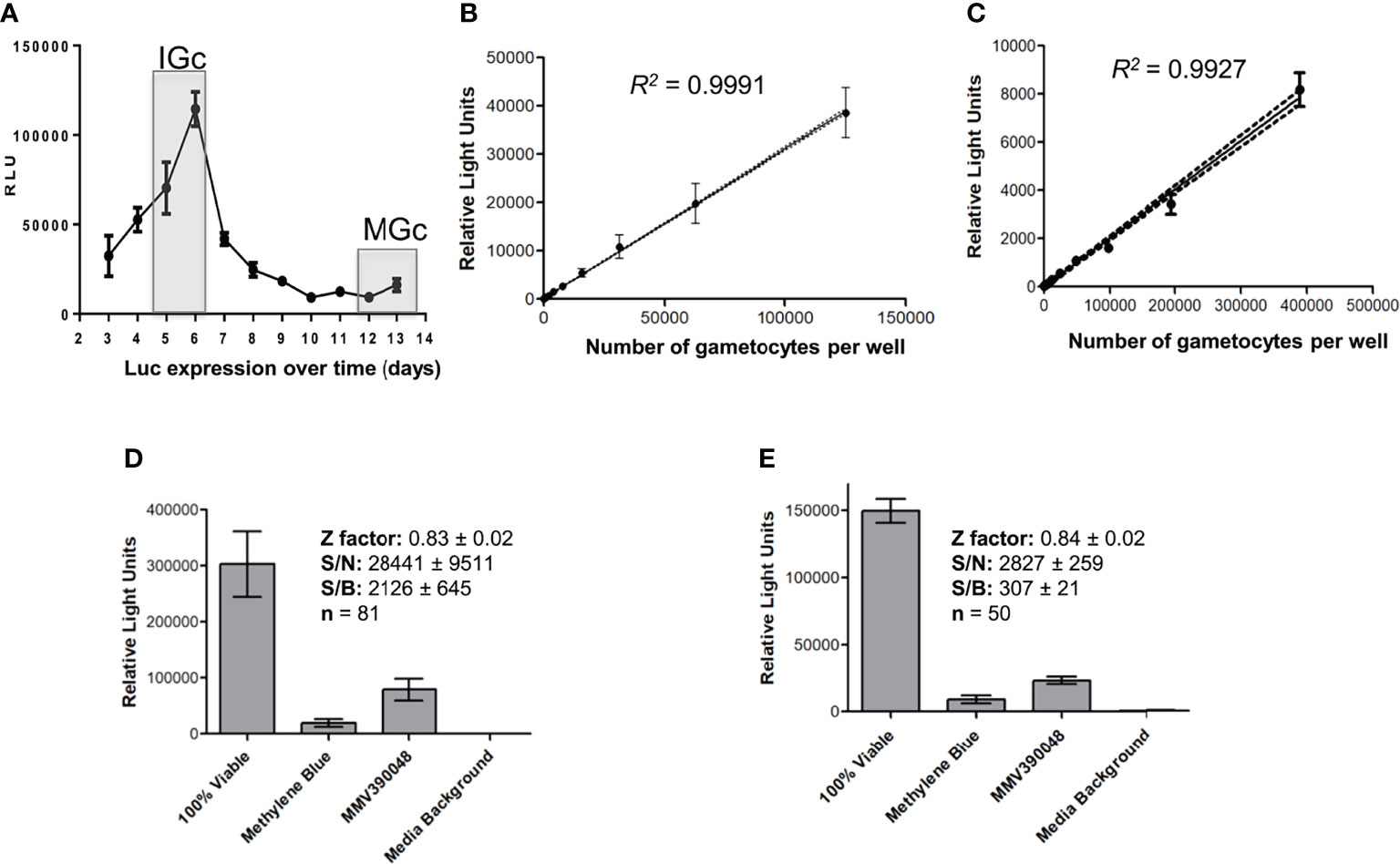
Figure 3 Luciferase reporter assay metrics. (A) Assessment of luciferase expression (RLU) throughout gametocytogenesis for the transgenic line NF54-pfs16-GFP-Luc on immature gametocytes (IGc, days 5–6), and the mature gametocytes (days 12–14, MGc). The linearity of luminescence readout compared to number of gametocytes per well for both the (B) immature and the (C) mature gametocytes. Data are from three independent biological experiments each performed in technical triplicates, ± SEM, 95% confidence intervals on each point indicated as ribbons. Assay metrics, including signal-to-noise (S/N), signal-to-background (S/B), and Z′-factor values for the luminescence readout (relative light units) on both the (D) immature and the (E) mature gametocyte assays, with 5 µM of either MMV390048 or MB.
To benchmark and validate the use of the stage-stratified gametocytes produced in gametocytocidal assays, a set of 12 known antimalarial compounds were evaluated against both immature and mature gametocytes and compared to published data (Table S1). The gametocytocidal activity of the compounds against our immature gametocyte population strongly correlated with that previously observed for both stage II (Pearson R2 = 0.98) and stage III gametocytes (Pearson R2 = 0.82; Figure 4A) (Plouffe et al., 2016) supporting the prevalence of stage II/III gametocytes in our immature gametocyte population and the absence of stage IV gametocytes (Pearson R2 = 0.48 with stage IV gametocytocidal action as reported (Plouffe et al., 2016)). Moreover, the gametocytocidal action of the compounds against immature gametocytes correlated weakly (Pearson R2 = 0.55; Figure 4B) with their activity against ABS parasites. Particularly, pyrimethamine and atovaquone that are potent ABS actives have no (>5,000 nM) activity against immature gametocytes (D'alessandro et al., 2016; Plouffe et al., 2016). The gametocytocidal action of the compounds against the mature gametocyte population confirmed the enrichment of stage V gametocytes, with a stronger correlation (Pearson R2 = 0.82) with the stage V population from the “Plouffe” data set (Plouffe et al., 2016), compared to the stage IV population (Pearson R2 = 0.48; Figure 4C). Comparison of individual IC50 values validated our data compared to previous reports [Table S1, (Lucantoni et al., 2013; Lucantoni et al., 2016; Plouffe et al., 2016)] and included, for instance, comparable IC50s of 20 and 0.9–7 nM for DHA on immature gametocytes (Table S1), while our mature assay accurately indicated the loss of activity of this compound on mature gametocytes (>2,000 nM), similar to data from Plouffe et al. (Plouffe et al. (2016)) and more accurate than those reported on late-stage gametocytes (Lucantoni et al., 2016; Coertzen et al., 2018).
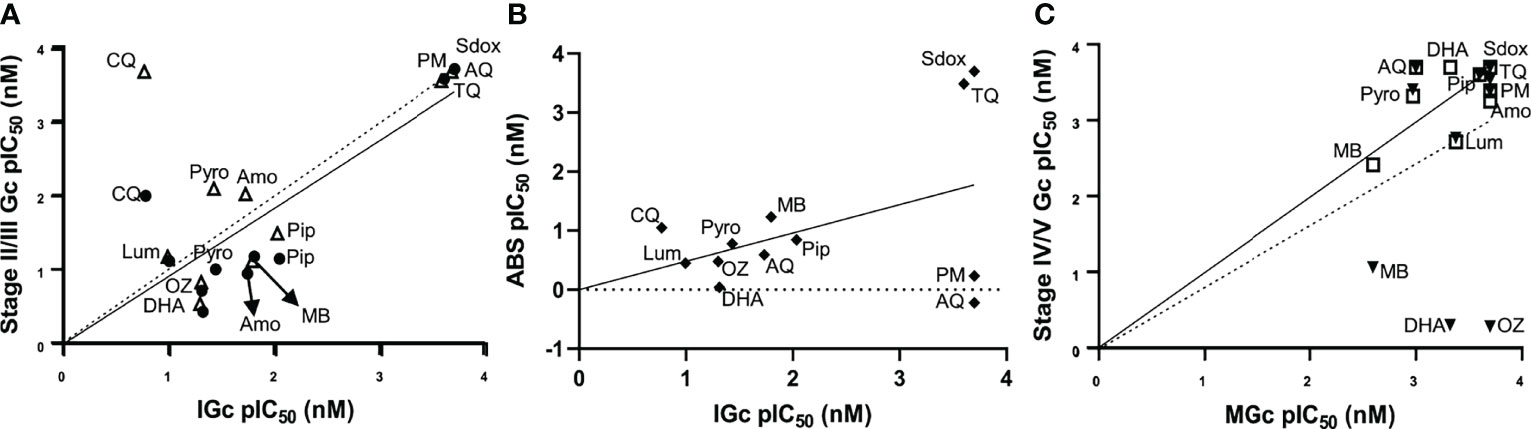
Figure 4 Stage-specific gametocytocidal action evaluation with known antimalarials. Correlation of the gametocytocidal activities of known antimalarials between the luciferase assay on (A) immature gametocytes and data from stage II (filled black circle; solid line) and stage III gametocytes (open triangle; dashed line) from a previous report (Plouffe et al., 2016). (B) Comparison between activity on immature gametocytes and ABS parasites. (C) Mature gametocyte activity compared to stage V (squares; solid line) and stage IV gametocytes (filled black triangles; dashed line) from a previous report (Plouffe et al., 2016). Data are from three independent biological experiments (n = 3), each performed in technical triplicates. DHA, dihydroartemisinin; OZ, artefenomel (OZ439); CQ, chloroquine; Amo, amodiaquine; Pip, piperaquine; Pyro, pyronaridine; Lum, lumefantrine; MB, methylene blue; AQ, atovaquone; PM, pyrimethamine; Sdox, sulfadoxine; TQ, tafenoquine.
When the stage-specific evaluation of gametocytocidal activity was extended to include additional investigative and frontrunner reference compounds from the Medicines for Malaria Venture (https://www.mmv.org/), we could confirm the presence of unique profiles associated with the susceptibility of different stages of gametocytes for different chemical classes (Figure 5). Amino alcohols (e.g., lumefantrine, halofantrine, and mefloquine), 4-aminoquinolines (such as amodiaquine, chloroquine, piperaquine, and pyronaridine), and the endoperoxides, which are all active against ABS parasites in a nanomolar range (Duffy and Avery, 2013), retain comparative activity in immature gametocytes with an average loss in activity of only ~3-fold observed for these classes. These compounds are inactive against mature stage V gametocytes (IC50 > 5 µM). The 8-aminoquinolines such as NPC-1161B and pamaquine show little activity on ABS (Duffy and Avery, 2013), which is also observed here (~60% inhibition for NPC-1161B and <50% inhibition for pamaquine at 5 µM; Figure 5), but the inactivity of primaquine in these in vitro assays is associated with its requirement of metabolic activation in vivo, as primaquine has validated transmission-blocking activity (Goncalves et al., 2016). Some activity for the above compounds is only reported when stage IV gametocytes were present (e.g., DHA with an IC50 of 88 nM in a stage IV/V population, but not active against stage V gametocytes; Figure 5), due to their known ability of targeting haem detoxification in the food vacuole, a process only active until stages III–IV of development and not important in mature, differentiated gametocytes (Lucantoni et al., 2013; Lucantoni et al., 2016; Plouffe et al., 2016). Only derivatives such as artemisone, artesunate, and artefenomel (OZ439) have some activity against mature gametocytes, associated with general disruption of redox homeostasis, as these processes are known to be downregulated in the mature gametocytes, enhancing the effect of increased reactive oxygen species production (Coertzen et al., 2018). Pyronaridine displays submicromolar activity against mature gametocytes (IC50 = 938 nM), in contrast to earlier reports (Duffy and Avery, 2013; Plouffe et al., 2016). The sulfonamides and antifolates (e.g., P218) have no activity against any stage of gametocytes, although antifolates are potently active against ABS (Duffy and Avery, 2013; Pethrak et al., 2022). The antibiotic, thiostrepton, retains activity against all stages, possibly through targeting general processes such as protein degradation. Importantly, more specific protein targets include the protein synthesis inhibitor with M5717 that inhibits elongation factor PfEF2 (Baragana et al., 2015), equipotently active (IC50s of 3.57 ± 0.7 and 0.25 ± 0.09 nM), against immature and mature gametocytes, respectively (P = 0.04, unpaired t-test, n = 3). This confirms its importance as frontrunner antimalarial candidate with ABS and gametocytocidal activity and its potential use in transmission-blocking strategies.
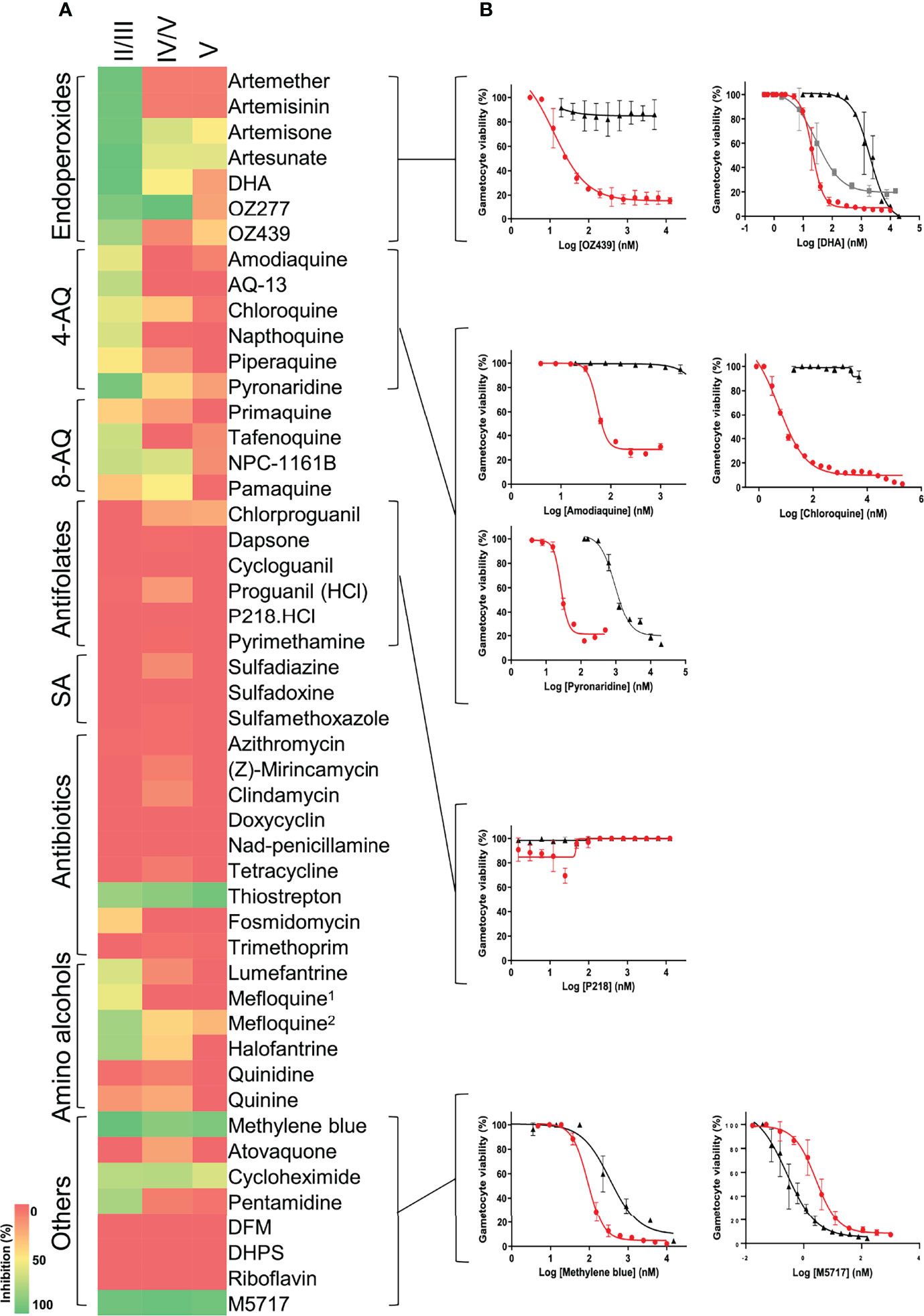
Figure 5 Evaluation of gametocytocidal activity of key MMV compounds. (A) Gametocytocidal profiling of the compounds (5 µM for 48 h) against immature (stage II/III), a mixture of stage IV and V and mature (stage V) gametocytes. (B) Dose-response curves of selected compounds indicating the IC50 shift between immature (red), mature (black), and mixed stage IV and V gametocyte populations (gray). 1Racemic, 2(+RS); DFM, deferoxamine mesylate salt; DHPS, dehydroepiandrosterone sulphate. Data are from three independent biological repeats (n = 3), performed in technical triplicates, mean ± S.E indicated.
Discussion
The recent detailed descriptors of the differential biology associated with the different stages of gametocytogenesis in P. falciparum have provided biological support and clarification for the stage-specific action of antimalarial compounds. It has become clear that profiling of compounds on immature gametocytes, in addition to mature gametocytes, is therefore of importance to progress leads and frontrunner clinical candidates as multistage active compounds. Such activities will ensure that sexual stages are targeted from the onset of gametocytogenesis and this will decrease the chances of mature gametocytes forming. In addition, if used in combination with an ABS active, but where the compound with gametocytocidal activity targets a different biological process, it will prolong the life span of ABS active as ABS-resistant parasites will not be able to spread (Birkholtz et al., 2022).
For stage-specific gametocyte assays to be routinely included to profile antimalarial candidates, we optimized a platform to allow robust, sensitive, and cost-efficient assays for stage-specific gametocytocidal activity to address the current caveat in the profiling of antimalarial candidates. This included the use of luciferase as viability indicator, produced from a single cell line and assayed in specific gametocyte stages, thereby proving previous proposals (Lucantoni et al., 2016) that such a whole-gametocytocidal assay is possible and can be widely applied to various antimalarial screening applications. Importantly, we prove that our platform allowed interrogation of the stage-specific gametocytocidal activity of frontrunner antimalarial candidates after 48 h drug pressure and therefore provide the first set of data to compare the activity of these compounds to data where they were evaluated on other life cycle stages after 48 h. Although longer incubation times (e.g., 72 h employed before) have improved compound activity against mature gametocytes (Lucantoni et al., 2017), this may indicate artificial potency as has been the case with DHA, where this compound could only be shown to be active on mature gametocytes upon longer incubation times (D'alessandro et al., 2016; Coertzen et al., 2018), inconsistent with the consensus that mature gametocytes are resistant to endoperoxides (Plouffe et al., 2016), as we confirm here. The reduced incubation period (compared to 72 h employed before) did not detrimentally affect assay performance, with high assay reproducibility still maintained (Z′-factors of 0.83 and 0.84 obtained on our platform with 48 h assay compared to previous reports of Z′-factors of 0.79–0.85 for a 72 h incubation on immature and mature gametocytes (Lucantoni et al., 2016). Additionally, using only a 48 h incubation on immature gametocytes ensures that stage-specific action is measured only on stage II/III gametocytes, while longer incubation periods run the risk of also measuring activity on developing stage IV gametocytes.
Additionally, our platform streamlined previous processes where either multiple P. falciparum luciferase expressing lines were required (Adjalley et al., 2011; Siciliano et al., 2017; Marin-Mogollon et al., 2019) or expensive instrumentation was needed, such as high-content imaging screening assays requiring expensive robotic imaging systems or monoclonal antibodies (Duffy and Avery, 2013; Lucantoni et al., 2015; Plouffe et al., 2016; Miguel-Blanco et al., 2017) rendering them impractical in developing countries or as routine profiling platforms. Similar to previous reports using the same cell line (Lucantoni et al., 2013; Lucantoni et al., 2016), the use of luciferase as viability indicator remains superior to other indicators including fluorescent signals (e.g., tdTomato), which may not be gametocyte- specific (Mclean et al., 2019) and requires expensive flow cytometry approaches (Matthews et al., 2020). Furthermore, luciferase is not influenced by assay differences as experienced with metabolic readouts (Reader et al., 2015; Reader et al., 2021). Importantly, expression of luciferase under the pfs16 promotor is gametocyte-specific and not influenced by the presence of asexual parasites (Lucantoni et al., 2013; Lucantoni et al., 2016). The latter is a major concern during drug screens particularly on immature gametocyte to distinguish activity of compounds from killing ABS parasites and therefore being seemingly active (Marin-Mogollon et al., 2019).
Previous stage-specific gametocyte assays made use of extensive, complicated manipulation of the gametocyte populations to enrich for specific stages (Adjalley et al., 2011; Lucantoni et al., 2013; Lucantoni et al., 2016; Plouffe et al., 2016), which compromises gametocyte viability and negates the use of such assays in resource constrained settings and in reproducible high-throughput applications. Furthermore, none of these methods result in any improved enrichment of specific stages above what we report here. Currently, stage stratification of gametocytes remains a mostly subjective process, dependent on expertise in morphological analyses of gametocyte stages. However, recent reports have guided this process as more detailed mechanistic data, and stage-specific features have been revealed (Dixon and Tilley, 2021), associated with the individual gametocyte stages in P. falciparum. Moreover, with the description of gametocyte-specific transcripts present exclusively in certain stages of gametocyte development (Van Biljon et al., 2019), these could be explored to generate lines with stage-specific expression of multiple reporter genes.
One of the main outcomes of this work is the unique gametocyte stage-specific profiles observed for an investigative and frontrunner reference set of compounds. This highlights the importance to investigate the chemosensitivity of the immature gametocytes to compounds in the developmental pipeline. Compounds that are unable to target immature gametocytes could result in seeding of mature gametocytes and could compromise their efficacy against these mature stages. The streamlined and robust process of gametocyte production and stage-specific evaluation of gametocytocidal activity of antimalarial compounds described here therefore provides affordable and sustainable enabling technology, which is immediately useful to studies performed in developing countries.
Data Availability Statement
The original contributions presented in the study are included in the article/Supplementary Material. Further inquiries can be directed to the corresponding author.
Author Contributions
L-MB conceived the study with JR and MvdW performing all assays and doing data analyses. JR and L-MB wrote the paper with input from MvdW. All authors contributed to the article and approved the submitted version.
Funding
L-MB acknowledges the South African Medical Research Council Strategic Health Innovation Partnership and the Department of Science and Innovation South African Research Chairs Initiative, administered through the South African National Research Foundation (UID 84627). Research is supported by a BMGF Grand Challenges Africa grant (GCA/DD2/Round10/021/001) and by the Medicines for Malaria Venture as Global Test center for stage-specific gametocytocidal assays to L-MB. The UP ISMC acknowledges the South African Medical Research Council (SA MRC) as Collaborating Centre for Malaria Research.
Conflict of Interest
The authors declare that the research was conducted in the absence of any commercial or financial relationships that could be construed as a potential conflict of interest.
Publisher’s Note
All claims expressed in this article are solely those of the authors and do not necessarily represent those of their affiliated organizations, or those of the publisher, the editors and the reviewers. Any product that may be evaluated in this article, or claim that may be made by its manufacturer, is not guaranteed or endorsed by the publisher.
Acknowledgments
We thank Seike Garny and Elisha Mugo for technical assistance. We furthermore thank the MMV for the compounds provided.
Supplementary Material
The Supplementary Material for this article can be found online at: https://www.frontiersin.org/articles/10.3389/fcimb.2022.926460/full#supplementary-material
References
Abraham, M., Gagaring, K., Marino, M. L., Vanaerschot, M., Plouffe, D., Calla, J., et al. (2020). Probing the Open Global Health Chemical Diversity Library for Multistage-Active Starting Points for Next-Generation Antimalarials. ACS Infect. Dis 6 (4), 613–628. doi: 10.1021/acsinfecdis.9b00482
Adjalley, S. H., Johnston, G. L., Li, T., Eastman, R. T., Ekland, E. H., Eappen, A. G., et al. (2011). Quantitative Assessment of Plasmodium Falciparum Sexual Development Reveals Potent Transmission-Blocking Activity by Methylene Blue. Proc. Natl. Acad. Sci. U.S.A. 108, E1214–E1223. doi: 10.1073/pnas.1112037108
Aguilar, R., Magallon-Tejada, A., Achtman, A. H., Moraleda, C., Joice, R., Cisteró, P., et al. (2014). Molecular Evidence for the Localization of Plasmodium Falciparum Immature Gametocytes in Bone Marrow. Blood J. Am. Soc. Hematol. 123, 959–966. doi: 10.1182/blood-2013-08-520767
Almela, M. J., Lozano, S., Lelievre, J., Colmenarejo, G., Coteron, J. M., Rodrigues, J., et al. (2015). A New Set of Chemical Starting Points With Plasmodium Falciparum Transmission-Blocking Potential for Antimalarial Drug Discovery. PloS One 10, e0135139. doi: 10.1371/journal.pone.0135139
Bancells, C., Llora-Batlle, O., Poran, A., Notzel, C., Rovira-Graells, N., Elemento, O., et al. (2019). Revisiting the Initial Steps of Sexual Development in the Malaria Parasite Plasmodium Falciparum. Nat. Microbiol. 4, 144–154. doi: 10.1038/s41564-018-0291-7
Baragana, B., Hallyburton, I., Lee, M. C., Norcross, N. R., Grimaldi, R., Otto, T. D., et al. (2015). A Novel Multiple-Stage Antimalarial Agent That Inhibits Protein Synthesis. Nature 522, 315–320. doi: 10.1038/nature14451
Birkholtz, L. M., Alano, P., Leroy, D. (2022). Transmission-Blocking Drugs for Malaria Elimination. Trends Parasitol. 38, 390–403. doi: 10.1016/j.pt.2022.01.011
Birkholtz, L. M., Coetzer, T. L., Mancama, D., Leroy, D., Alano, P. (2016). Discovering New Transmission-Blocking Antimalarial Compounds: Challenges and Opportunities. Trends Parasitol. 32, 669–681. doi: 10.1016/j.pt.2016.04.017
Bousema, T., Okell, L., Shekalaghe, S., Griffin, J. T., Omar, S., Sawa, P., et al. (2010). Revisiting the Circulation Time of Plasmodium Falciparum Gametocytes: Molecular Detection Methods to Estimate the Duration of Gametocyte Carriage and the Effect of Gametocytocidal Drugs. Malar. J. 9, 136. doi: 10.1186/1475-2875-9-136
Brancucci, N. M. B., De Niz, M., Straub, T. J., Ravel, D., Sollelis, L., Birren, B. W., et al. (2018). Probing Plasmodium Falciparum Sexual Commitment at the Single-Cell Level. Wellcome Open Res. 3, 70. doi: 10.12688/wellcomeopenres.14645.4
Bruce, M. C., Carter, R. N., Nakamura, K., Aikawa, M., Carter, R. (1994). Cellular Location and Temporal Expression of the Plasmodium Falciparum Sexual Stage Antigen Pfs16. Mol. Biochem. Parasitol. 65, 11–22. doi: 10.1016/0166-6851(94)90111-2
Buckling, A., Ranford-Cartwright, L., Miles, A., Read, A. (1999). Chloroquine Increases Plasmodium Falciparum Gametocytogenesis In Vitro. Parasitology 118, 339–346. doi: 10.1017/S0031182099003960
Burrows, J. N., Duparc, S., Gutteridge, W. E., Hooft Van Huijsduijnen, R., Kaszubska, W., Macintyre, F., et al. (2017). New Developments in Anti-Malarial Target Candidate and Product Profiles. Malar. J. 16, 26. doi: 10.1186/s12936-016-1675-x
Coertzen, D., Reader, J., van der Watt, M., Nondaba, S. H., Gibhard, L., Wiesner, L., et al. (2018). Artemisone and Artemiside Are Potent Panreactive Antimalarial Agents That Also Synergize Redox Imbalance in Plasmodium Falciparum Transmissible Gametocyte Stages. Antimicrob. Agents Chemother. 62, e02214-17. doi: 10.1128/AAC.02214-17
Coetzee, N., Von Gruning, H., Opperman, D., van der Watt, M., Reader, J., Birkholtz, L. M. (2020). Epigenetic Inhibitors Target Multiple Stages of Plasmodium Falciparum Parasites. Sci. Rep. 10, 2355. doi: 10.1038/s41598-020-59298-4
D'alessandro, S., Camarda, G., Corbett, Y., Siciliano, G., Parapini, S., Cevenini, L., et al. (2016). A Chemical Susceptibility Profile of the Plasmodium Falciparum Transmission Stages by Complementary Cell-Based Gametocyte Assays. J. Antimicrob. Chemother. 71, 1148–1158. doi: 10.1093/jac/dkv493
Day, K. P., Hayward, R. E., Dyer, M. (1998). The Biology of Plasmodium Falciparum Transmission Stages. Parasitology 116, 95–109. doi: 10.1017/S0031182000084985
Delves, M. J. (2012). Plasmodium Cell Biology Should Inform Strategies Used in the Development of Antimalarial Transmission-Blocking Drugs. Future Med. Chem. 4, 2251–2263. doi: 10.4155/fmc.12.182
Delves, M., Lafuente-Monasterio, M. J., Upton, L., Ruecker, A., Leroy, D., Gamo, F. J., et al. (2019). Fueling Open Innovation for Malaria Transmission-Blocking Drugs: Hundreds of Molecules Targeting Early Parasite Mosquito Stages. Front. Microbiol. 10, 2134. doi: 10.3389/fmicb.2019.02134
Delves, M. J., Miguel-Blanco, C., Matthews, H., Molina, I., Ruecker, A., Yahiya, S., et al. (2018). A High Throughput Screen for Next-Generation Leads Targeting Malaria Parasite Transmission. Nat. Commun. 9, 3805. doi: 10.1038/s41467-018-05777-2
Delves, M. J., Ruecker, A., Straschil, U., Lelievre, J., Marques, S., Lopez-Barragan, M. J., et al. (2013). Male and Female Plasmodium Falciparum Mature Gametocytes Show Different Responses to Antimalarial Drugs. Antimicrob. Agents Chemother. 57, 3268–3274. doi: 10.1128/AAC.00325-13
Dixon, M. W. A., Tilley, L. (2021). Plasmodium Falciparum Goes Bananas for Sex. Mol. Biochem. Parasitol. 244, 111385. doi: 10.1016/j.molbiopara.2021.111385
Duffy, S., Avery, V. M. (2013). Identification of Inhibitors of Plasmodium Falciparum Gametocyte Development. Malar. J. 12, 408. doi: 10.1186/1475-2875-12-408
Fehintola, F., Balogun, S., Adeoye, S. (2012). Intermittent Preventive Treatment During Pregnancy With Sulphadoxine-Pyrimethamine may Promote Plasmodium Falciparum Gametocytogenesis. Med. Principles Pract. 21, 63–67. doi: 10.1159/000332405
Goncalves, B. P., Tiono, A. B., Ouedraogo, A., Guelbeogo, W. M., Bradley, J., Nebie, I., et al. (2016). Single Low Dose Primaquine to Reduce Gametocyte Carriage and Plasmodium Falciparum Transmission After Artemether-Lumefantrine in Children With Asymptomatic Infection: A Randomised, Double-Blind, Placebo-Controlled Trial. BMC Med. 14, 40. doi: 10.1186/s12916-016-0581-y
Lelievre, J., Almela, M. J., Lozano, S., Miguel, C., Franco, V., Leroy, D., et al. (2012). Activity of Clinically Relevant Antimalarial Drugs on Plasmodium Falciparum Mature Gametocytes in an ATP Bioluminescence "Transmission Blocking" Assay. PloS One 7, e35019. doi: 10.1371/journal.pone.0035019
Lucantoni, L., Duffy, S., Adjalley, S. H., Fidock, D. A., Avery, V. M. (2013). Identification of MMV Malaria Box Inhibitors of Plasmodium Falciparum Early-Stage Gametocytes Using a Luciferase-Based High-Throughput Assay. Antimicrob. Agents Chemother. 57, 6050–6062. doi: 10.1128/AAC.00870-13
Lucantoni, L., Fidock, D. A., Avery, V. M. (2016). Luciferase-Based, High-Throughput Assay for Screening and Profiling Transmission-Blocking Compounds Against Plasmodium Falciparum Gametocytes. Antimicrob. Agents Chemother. 60, 2097–2107. doi: 10.1128/AAC.01949-15
Lucantoni, L., Loganathan, S., Avery, V. M. (2017). The Need to Compare: Assessing the Level of Agreement of Three High-Throughput Assays Against Plasmodium Falciparum Mature Gametocytes. Sci. Rep. 7, 45992. doi: 10.1038/srep45992
Lucantoni, L., Silvestrini, F., Signore, M., Siciliano, G., Eldering, M., Dechering, K. J., et al. (2015). A Simple and Predictive Phenotypic High Content Imaging Assay for Plasmodium Falciparum Mature Gametocytes to Identify Malaria Transmission Blocking Compounds. Sci. Rep. 5, 16414. doi: 10.1038/srep16414
Marin-Mogollon, C., Salman, A. M., Koolen, K. M. J., Bolscher, J. M., Van Pul, F. J. A., Miyazaki, S., et al. (2019). A P. Falciparum NF54 Reporter Line Expressing Mcherry-Luciferase in Gametocytes, Sporozoites, and Liver-Stages. Front. Cell Infect. Microbiol. 9, 96. doi: 10.3389/fcimb.2019.00096
Matthews, K. A., Senagbe, K. M., Notzel, C., Gonzales, C. A., Tong, X., Rijo-Ferreira, F., et al. (2020). Disruption of the Plasmodium Falciparum Life Cycle Through Transcriptional Reprogramming by Inhibitors of Jumonji Demethylases. ACS Infect. Dis. 6, 1058–1075. doi: 10.1021/acsinfecdis.9b00455
Mclean, K. J., Straimer, J., Hopp, C. S., Vega-Rodriguez, J., Small-Saunders, J. L., Kanatani, S., et al. (2019). Generation of Transmission-Competent Human Malaria Parasites With Chromosomally-Integrated Fluorescent Reporters. Sci. Rep. 9, 13131. doi: 10.1038/s41598-019-49348-x
Meibalan, E., Marti, M. (2017). Biology of Malaria Transmission. Cold Spring Harb. Perspect. Med. 7. doi: 10.1101/cshperspect.a025452
Miguel-Blanco, C., Molina, I., Bardera, A. I., Diaz, B., De Las Heras, L., Lozano, S., et al. (2017). Hundreds of Dual-Stage Antimalarial Molecules Discovered by a Functional Gametocyte Screen. Nat. Commun. 8, 15160. doi: 10.1038/ncomms15160
Ngotho, P., Soares, A. B., Hentzschel, F., Achcar, F., Bertuccini, L., Marti, M. (2019). Revisiting Gametocyte Biology in Malaria Parasites. FEMS Microbiol. Rev. 43, 401–414. doi: 10.1093/femsre/fuz010
Paquet, T., Le Manach, C., Cabrera, D. G., Younis, Y., Henrich, P. P., Abraham, T. S., et al. (2017). Antimalarial Efficacy of MMV390048, an Inhibitor of Plasmodium Phosphatidylinositol 4-Kinase. Sci. Transl. Med. 9. doi: 10.1126/scitranslmed.aad9735
Pethrak, C., Posayapisit, N., Pengon, J., Suwanakitti, N., Saeung, A., Shorum, M., et al. (2022). New Insights Into Antimalarial Chemopreventive Activity of Antifolates. Antimicrob. Agents Chemother. 66, e0153821. doi: 10.1128/aac.01538-21
Plouffe, D. M., Wree, M., Du, A. Y., Meister, S., Li, F., Patra, K., et al. (2016). High-Throughput Assay and Discovery of Small Molecules That Interrupt Malaria Transmission. Cell Host Microbe 19, 114–126. doi: 10.1016/j.chom.2015.12.001
Price, R., Nosten, F., Simpson, J. A., Luxemburger, C., Phaipun, L., Ter Kuile, F., et al. (1999). Risk Factors for Gametocyte Carriage in Uncomplicated Falciparum Malaria. Am. J. Trop. Med. Hyg. 60, 1019–1023. doi: 10.4269/ajtmh.1999.60.1019
Reader, J., Botha, M., Theron, A., Lauterbach, S. B., Rossouw, C., Engelbrecht, D., et al. (2015). Nowhere to Hide: Interrogating Different Metabolic Parameters of Plasmodium Falciparum Gametocytes in a Transmission Blocking Drug Discovery Pipeline Towards Malaria Elimination. Malar. J. 14, 213. doi: 10.1186/s12936-015-0718-z
Reader, J., van der Watt, M. E., Taylor, D., Le Manach, C., Mittal, N., Ottilie, S., et al. (2021). Multistage and Transmission-Blocking Targeted Antimalarials Discovered From the Open-Source MMV Pandemic Response Box. Nat. Commun. 12, 269. doi: 10.1038/s41467-020-20629-8
Schneider, P., Reece, S. E. (2021). The Private Life of Malaria Parasites: Strategies for Sexual Reproduction. Mol. Biochem. Parasitol. 244, 111375. doi: 10.1016/j.molbiopara.2021.111375
Siciliano, G., Santha Kumar, T. R., Bona, R., Camarda, G., Calabretta, M. M., Cevenini, L., et al. (2017). A High Susceptibility to Redox Imbalance of the Transmissible Stages of Plasmodium Falciparum Revealed With a Luciferase-Based Mature Gametocyte Assay. Mol. Microbiol. 104, 306–318. doi: 10.1111/mmi.13626
Sinden, R. (2015). The Cell Biology of Malaria Infection of Mosquito: Advances and Opportunities. Cell. Microbiol. 17, 451–466. doi: 10.1111/cmi.12413
Sowunmi, A., Adedeji, A., Gbotosho, G., Fateye, B., Happi, T. (2006). Effects of Pyrimethamine-Sulphadoxine, Chloroquine Plus Chlorpheniramine, and Amodiaquine Plus Pyrimethamine-Sulphadoxine on Gametocytes During and After Treatment of Acute, Uncomplicated Malaria in Children. Memórias do Instituto. Oswaldo Cruz 101, 887–893. doi: 10.1590/S0074-02762006000800011
Sowunmi, A., Fateye, B. (2003). Plasmodium Falciparum Gametocytaemia in Nigerian Children: Before, During and After Treatment With Antimalarial Drugs. Trop. Med. Int. Health 8, 783–792. doi: 10.1046/j.1365-3156.2003.01093.x
Thommen, B. T., Passecker, A., Buser, T., Hitz, E., Voss, T. S., Brancucci, N. M. B. (2022). Revisiting the Effect of Pharmaceuticals on Transmission Stage Formation in the Malaria Parasite Plasmodium Falciparum. Front. Cell Infect. Microbiol. 12, 802341. doi: 10.3389/fcimb.2022.802341
Usui, M., Williamson, K. C. (2021). Stressed Out About Plasmodium Falciparum Gametocytogenesis. Front. Cell Infect. Microbiol. 11, 790067. doi: 10.3389/fcimb.2021.790067
Van Biljon, R., Niemand, J., Van Wyk, R., Clark, K., Verlinden, B., Abrie, C., et al. (2018). Inducing Controlled Cell Cycle Arrest and Re-Entry During Asexual Proliferation of Plasmodium Falciparum Malaria Parasites. Sci. Rep. 8, 16581. doi: 10.1038/s41598-018-34964-w
Van Biljon, R., Van Wyk, R., Painter, H. J., Orchard, L., Reader, J., Niemand, J., et al. (2019). Hierarchical Transcriptional Control Regulates Plasmodium Falciparum Sexual Differentiation. BMC Genomics 20, 920. doi: 10.1186/s12864-019-6322-9
Van Der Watt, M. E., Reader, J., Birkholtz, L. (2022). Adapt or Die: Targeting Unique Transmission-Stage Biology for Malaria Elimination. Front. Cell Infect. Microbiol. doi: 10.3389/fcimb.2022.901971
Van Der Watt, M. E., Reader, J., Churchyard, A., Nondaba, S. H., Lauterbach, S. B., Niemand, J., et al. (2018). Potent Plasmodium Falciparum Gametocytocidal Compounds Identified by Exploring the Kinase Inhibitor Chemical Space for Dual Active Antimalarials. J. Antimicrob. Chemother. 73, 1279–1290. doi: 10.1093/jac/dky008
Van Voorhis, W. C., Adams, J. H., Adelfio, R., Ahyong, V., Akabas, M. H., Alano, P., et al. (2016). Open Source Drug Discovery With the Malaria Box Compound Collection for Neglected Diseases and Beyond. PloS Pathog. 12, e1005763. doi: 10.1371/journal.ppat.1005763
Vos, M. W., Stone, W. J., Koolen, K. M., Van Gemert, G. J., Van Schaijk, B., Leroy, D., et al. (2015). A Semi-Automated Luminescence Based Standard Membrane Feeding Assay Identifies Novel Small Molecules That Inhibit Transmission of Malaria Parasites by Mosquitoes. Sci. Rep. 5, 18704. doi: 10.1038/srep18704
Young, J. A., Fivelman, Q. L., Blair, P. L., de la Vega, P., Le Roch, K. G., Zhou, Y., et al. (2005). The Plasmodium Falciparum Sexual Development Transcriptome: A Microarray Analysis Using Ontology-Based Pattern Identification. Mol. Biochem. Parasitol. 143, 67–79. doi: 10.1016/j.molbiopara.2005.05.007
Keywords: gametocyte, luciferase, malaria, Plasmodium, transmission-blocking, drug discovery
Citation: Reader J, van der Watt ME and Birkholtz L-M (2022) Streamlined and Robust Stage-Specific Profiling of Gametocytocidal Compounds Against Plasmodium falciparum. Front. Cell. Infect. Microbiol. 12:926460. doi: 10.3389/fcimb.2022.926460
Received: 22 April 2022; Accepted: 30 May 2022;
Published: 30 June 2022.
Edited by:
Tania F. De Koning-Ward, Deakin University, AustraliaReviewed by:
Rapatbhorn Patrapuvich, Mahidol University, ThailandChe Julius Ngwa, RWTH Aachen University, Germany
Copyright © 2022 Reader, van der Watt and Birkholtz. This is an open-access article distributed under the terms of the Creative Commons Attribution License (CC BY). The use, distribution or reproduction in other forums is permitted, provided the original author(s) and the copyright owner(s) are credited and that the original publication in this journal is cited, in accordance with accepted academic practice. No use, distribution or reproduction is permitted which does not comply with these terms.
*Correspondence: Lyn-Marié Birkholtz, bGJpcmtob2x0ekB1cC5hYy56YQ==