Corrigendum: The science behind TCM and Gut microbiota interaction-their combinatorial approach holds promising therapeutic applications
- 1Hospital of Chengdu University of Traditional Chinese Medicine, Chengdu, China
- 2Chengdu University of Traditional Chinese Medicine, Chengdu, China
- 3National Drug Clinical Trial Agency, Teaching Hospital of Chengdu University of Traditional Chinese Medicine (TCM), Chengdu, China
- 4Special Infectious Agents Unit, King Fahd Medical Research Center, King Abdulaziz University, Jeddah, Saudi Arabia
- 5Department of Biotechnology, Abdul Wali Khan University Mardan, Khyber Pakhtunkhwa, Pakistan
The trend toward herbal medicine as an alternative treatment for disease medication is increasing worldwide. However, insufficient pharmacologic information is available about the orally taken medicines. Not only herbal medicine, but also Western drugs, when passing through the gastrointestinal tract, interact with trillions of microbes (known as the gut microbiome [GM]) and their enzymes. Gut microbiome enzymes induce massive structural and functional changes to the herbal products and impact the bioavailability and efficacy of the herbal therapeutics. Therefore, traditional Chinese medicine (TCM) researchers extend the horizon of TCM research to the GM to better understand TCM pharmacology and enhance its efficacy and bioavailability. The study investigating the interaction between herbal medicine and gut microbes utilizes the holistic approach, making landmark achievements in the field of disease prognosis and treatment. The effectiveness of TCM is a multipathway modulation, and so is the GM. This review provides an insight into the understanding of a holistic view of TCM and GM interaction. Furthermore, this review briefly describes the mechanism of how the TCM–GM interaction deals with various illnesses.
1 Introduction
Traditional Chinese medicine (TCM) is a medicinal system that is thousands of years old and has been widely adopted for treating diseases. The precarious therapeutic approach of Western medicine has limited TCM application to the Chinese people only. To make TCM a good competitor with Western medicine, the Chinese government has established 16 TCM centers to modernize TCM application (Feng et al., 2017). Toward the moderation of TCM, OMICS is one of the imperative fields of research that can search for potential targets on which TCM acts and trigger downstream signaling cascades (Joshi et al., 2010).
Unlike Western drugs, TCM holistically improves body physiology against diseases and, therefore, is prescribed for holistic characterization of the patient’s syndrome by following the yin and yang and five elements theory, visceral meridian theory, etiology and pathogenesis theory, diagnosis, and therapy theory (Huang K. et al., 2021). TCM can be available in the form of decoction, powder, pill, and paste. Interestingly, the same ingredients can compose a prescription; however, different dosages can have distinct functions.
A bigger part of TCM is orally taken, which then passes through physiological changes, mainly, through the enzymatic activities that are secreted by trillions of gut-residing microbes (known as gut microbiota [GM]) and host cells. These activities can remodel the functional constituents of TCM. Importantly, GM display a critical role in host health even in the occurrence of disease. It is estimated that GM constitutes about 43% of the human body by cell count and encodes 100 times more genes than our body genes (Knight et al., 2017). According to the latest statistics, the human microbiome encodes 2-20 million genes, surpassing ∼20,000 human genes (Knight et al., 2017). These microbial genes are presented to hosts for various functions, including digestion, metabolism, and immune system maturation (Cani, 2009). The human GM (approximately 99%) is composed of bacteria. And, among the body parts, the gastrointestinal tract is more densely populated. These commensals inhabit the human gut with a magnitude of about 100 billion to 1 trillion bacterial cells in one gram of human stool (Hugon et al., 2015). A balanced GM is key to host health, and any dysbiosis in gut microbial composition could put the host at a risk for obesity, inflammatory bowel disease, diabetes, autism, rheumatoid arthritis, and colorectal cancer (CRC) (Musso et al., 2010; Stefka et al., 2014; Sun and Kato, 2016; Maeda and Takeda, 2017).
Most importantly, TCM–GM research is updating our understanding of disease prognosis and treatment. A growing trend is developing among TCM researchers to define the pharmacology of orally taken TCM by harnessing the potentials of the GM. TCM interacts with trillions of gut-residing microbes and their enzymes that present massive structural and functional changes. These microbes can affect the bioavailability and efficacy of the herbal therapeutics. Not only herbal medicine, but also the efficacy of Western medicines relies on the GM. For instance, the efficacy of cyclophosphamide, an anticancer immune-suppressant drug, is dependent on two intestinal commensals called Enterococcus hirae and Barnesiella intestinihominis (Viaud et al., 2013). This interaction also unmasks the holistic therapeutic approach of TCM and reveals the vital role of GM. With a growing understanding of TCM–GM, various GM-based therapeutic approaches are developing. However, being a new topic, there are questions that still need to be addressed: (a) How does TCM remodel GM diversity and composition? (b) How can the GM remodel TCM constituents and their function? (c) Does TCM function as a growth substrate for the GM? Nevertheless, the study of GM–TCM interaction has opened an exciting avenue for drug discovery and new drug targeting (El Kaoutari et al., 2013) (Chen F. et al., 2016). This review focuses on highlighting key achievements from TCM–GM research.
2 TCM–GM interaction
TCM–GM interaction employs a comprehensive approach and is making groundbreaking achievements in the field of disease prognosis and treatment. Since targeted intervention for remodeling GM composition has shown encouraging results in the field of disease prevention and treatment, in this regard, TCM has become one of the approaches through which GM composition is targeted and remodeled for predetermined therapeutic outcomes. Various TCM components, such as dietary fiber, phenolic compounds, and undigested carbohydrates, are proven to upregulate the growth of beneficial intestinal microbes, improve gut homeostasis, and alleviate disease symptoms (Makki et al., 2018). For example, several studies show GM remodeling effects of saponins, naturally occurring compounds extracted from a Chinese medicinal herb (known as Gynostemma pentaphyllum). Saponins are reported to facilitate the growth of beneficial bacteria and suppress cachexia-like symptoms in mouse models (Chen L. et al., 2016; Huang et al., 2017).
Not only does TCM affect the GM composition and diversity, but TCM therapeutic efficacy is dependent on the presence of certain bacterial species and their enzymes. For instance, PHY906 (derived from four Chinese herbs) is an anticancer medicine that can reduce irinotecan toxicity in advanced-stage CRC patients. However, the efficacy of PHY906 is dependent on β-glucuronidase, an enzyme produced by intestinal bacteria (Lam et al., 2010). Similarly, curcumin supplements to a Il10−/− mouse promote the abundance of Lactobacillales and increase bacterial diversity that is concurrently accompanied by a reduced polyp burden (McFadden et al., 2015). Nonetheless, GM also improves the availability of TCM, which is one of the classic limitations of TCM.
Another classic example of the TCM–GM interaction is the ingestion of indigested polysaccharides. Indigestible polysaccharides undergo biochemical processes in the gastrointestinal tract and are converted into short-chain fatty acids (SCFAs) by gut microbes. SCFAs are indispensable in maintaining our health, especially colon health (Figure 1). Nonetheless, SCFAs are the energy sources for colonocytes to guarantee the activity of the colon (Wong et al., 2006). Besides this, through inhibition of histone deacetylases and activation of G-couple protein receptors (GPRs), SCFAs can regulate the immune system and correct metabolic disorders (Sun et al., 2017). In addition, SCFAs can also contribute to the gut barrier construction via enhancement of the expression of the MUC-2 gene, modulation of oxidative stress, and upregulation of tight junction molecules (Wang et al., 2012; van der Beek et al., 2017). Furthermore, SCFAs also help in alleviating metabolic syndrome in a high-fat-diet mouse model by inhibiting the expression of pro-inflammatory cytokines, such as IL-1β and IL-6 as well as toll like receptor 4 (TLR4) in adipose tissue (Zhai et al., 2019). The nexus of TCM–GM is a relatively new concept, and interests have been developing toward this field since 2015. In the following section of the manuscript, we summarize how TCM and GM influence each other directly and/or indirectly.
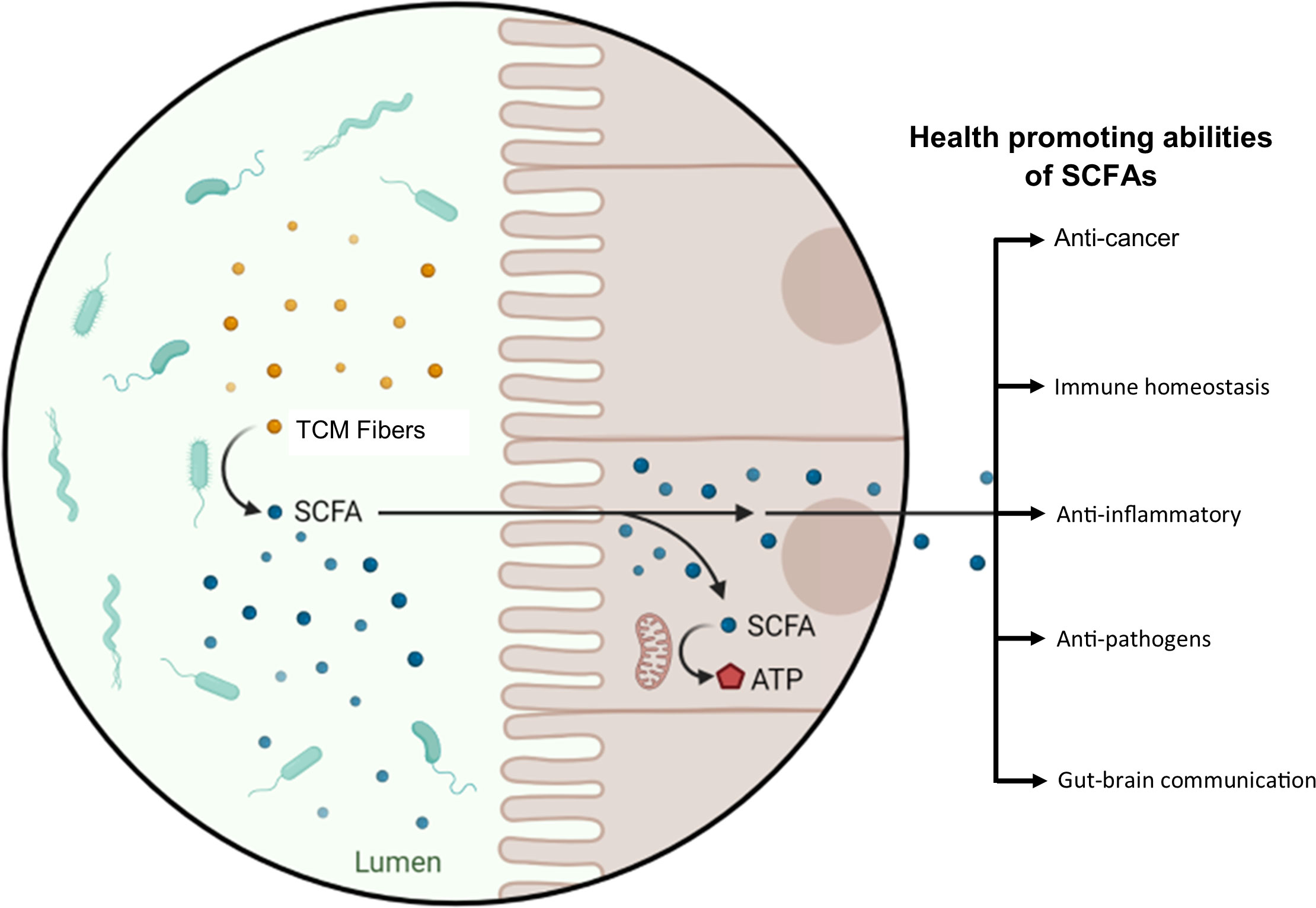
Figure 1 TCM–GM combinatorial therapeutic effect, taking polysaccharides as an example. Dietary and medicinal fibers are converted into SCFAs by the action of the GM. SCFAs are an energy source for colonocytes and possess other health-promoting abilities; a few of these abilities are displayed in the figure.
2.1 Effects of TCM on GM
2.1.1 Direct effects
TCM plays a significant role in promoting the growth of intestinal probiotics and is one of the ways for TCM to exert its curative effect (Table 1). For instance, Pogostemon cablin (Blanco) Benth (PC) is a Chinese medicinal plant, traditionally used for the treatment of gastrointestinal symptoms. Four extractions of PC (i.e., patchouli essential oil, patchouli alcohol, pogostone, and β-patchoulene) were found to promote the abundance of beneficial bacteria, such as Anaerostipes butyraticus, Butytivibrio fibrisolvens, Clostridium jejuense, Eubacterium uniforme, and Lactobacillus lactis (Leong et al., 2019). Other compounds, such as polysaccharide, also have well-known prebiotics. It is reported that the Lycium barbarum polysaccharides reduce the abundance of potential pathogens, such as Allobaculum stercoricanis, Parasutterella excrementihominis, and Tannerella spp., and enhance the abundance of beneficial bacteria, including Clostridium sp., Lachnoclostridium clostridium xylanolyticum, Lachnoclostridium clostridium saccharolyticum, and Lactobacillus reuteri in C57 mice (Xia et al., 2020b). Besides this, mushroom polysaccharides (Ganoderma lucidum and Poria cocos) can also exert beneficial effects by altering GM composition and improving the ratio of beneficial bacteria to potential pathogens (Khan et al., 2018).
Several studies report promoted growth of beneficial bacteria after feeding a host with the saponin of the Gynostemma pentaphyllum. But it was unknown how saponin could promote the growth of bacteria. In a recent study, Liao et al. found out, in an in vitro setup, that saponin promotes growth of the beneficial bacteria (such as Bifidobacterium animalis and Lactobacillus casei) by upregulating several bacterial key genes involve in biogenesis and metabolic pathways (e.g., gatC, rpmH, ruvA, yajC, and rsfS) (Hugon et al., 2015). For a better understanding, Figure 2 shows how TCM can directly impact the composition of the GM. This can also be taken as a guide to investigate the interaction between TCM and GM.
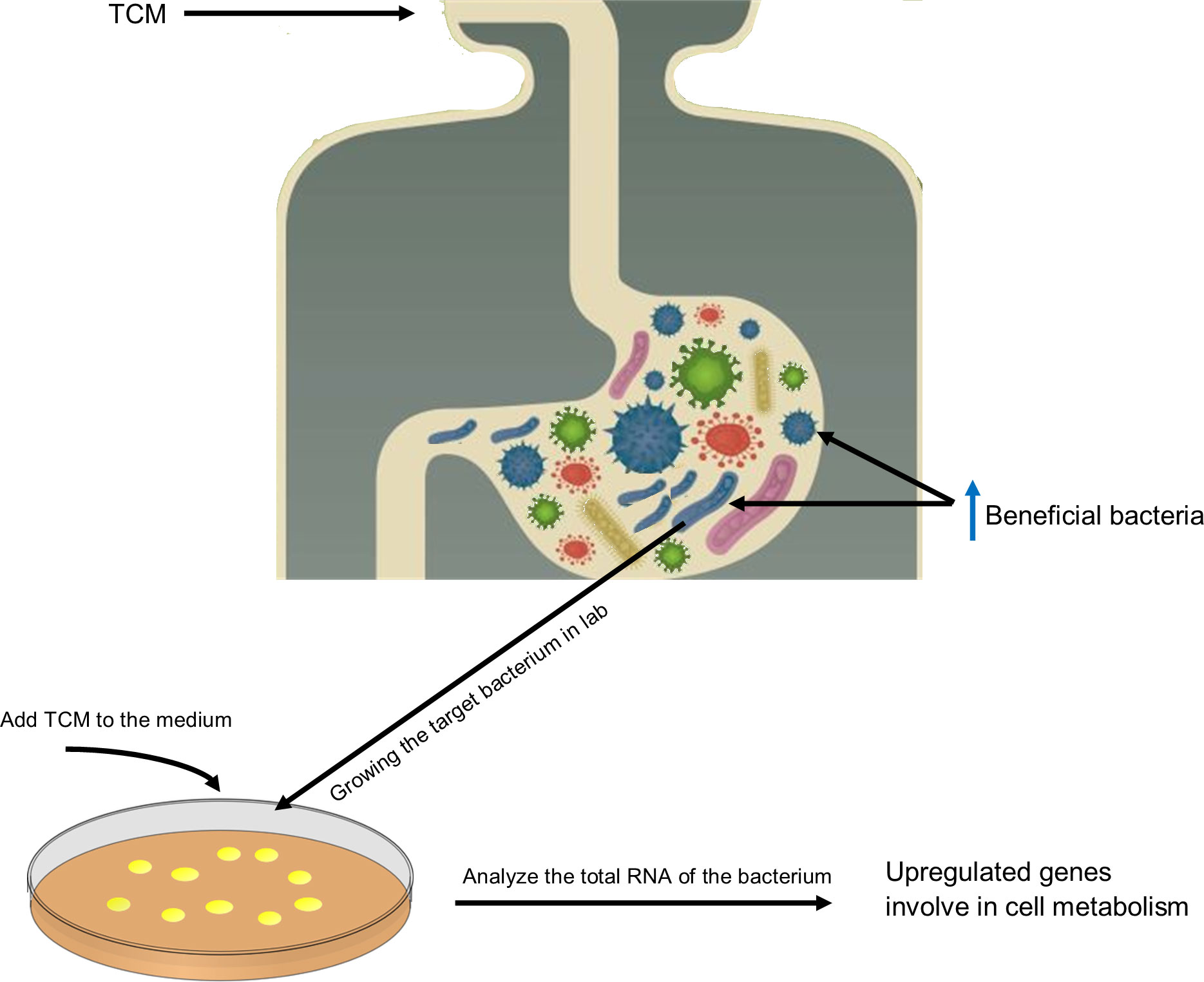
Figure 2 Graphic illustration of the TCM effect on GM composition and gene expression and a guide to investigate the TCM–GM interaction. This illustration shows the direct effect of TCM on GM composition. TCM intake promotes growth of certain bacteria; for instance, in the case of saponins, B animalis and L. reuteri become abundant. To check how TCM modulates the genome expression of microbes, target bacteria should be culture in vitro in the presence of TCM, and their growth kinetics and gene expression should be monitored. In the case of saponins, it is observed that saponins promote the expression of genes involved in metabolism and biogenesis that contribute to promoting growth of these bacteria in a habitat.
2.1.2 Indirect effects
Certainly, the TCM therapeutic mechanism has outcomes far beyond simply changing the composition of the GM. As mentioned above, in health maintenance and disease development, the GM exerts its function in multiple ways. In this regard, the immune system has an impactful role in both health and diseases. TCM affects the host’s immune system to secrete materials that possess GM-modulating properties (Ostaff et al., 2013). For instance, the extracts of Codonopsis pilosula, Saussurea lappa, Imperata cylindrical var. major and Melia toosendan increase the secretion of antimicrobial peptides that markedly impact GM composition (Zhou et al., 2016). In addition, TCM can also regulate GM composition and diversity by affecting the structure of the intestinal barrier. For instance, rhubarb enema is a common TCM medicine that can improve intestinal barrier integrity and consequently regulate GM dysbiosis (Ji et al., 2020). This TCM increases the expression of tight junction (TJ) molecules, thereby promoting the proliferation of gut epithelial cells and significantly enhancing the gut intrinsic mucosal defense, which results in the prevention of harmful substances and sequentially helps to restore GM composition (Tian., 2020). Another way that TCM promotes the growth of beneficial bacteria in the gut is stimulating the gut mucosa, which is a source of nutrients for bacteria such as Lactobacillus and Akkermansia (Tian., 2020). These bacteria have a mutual collaboration in repairing the gut barrier by promoting the growth of goblet cells and mucin 2 (Lu et al., 2021). TCM (such as herbal polysaccharides) can also regulate GM composition through the gut–brain axis (Sun et al., 2020). The direct and indirect effects of TCM on GM composition and diversity is displayed in Figure 3.
2.2 Effects of GM on TCM
2.2.1 GM transforms TCM into functional metabolites
In the gastrointestinal tract, TCM exerts a curative effect through a series of complex processes, such as absorption, transformation, and metabolism. During these processes, the GM plays an important role in the modification, absorption, and detoxication of TCM ingredients and consequently improves its efficacy (Wilson and Nicholson, 2017). The GM can also contribute to the bioavailability of TCM biochemical components (Wu and Tan, 2019; Zhang et al., 2021). Below we explain and display in Figure 3 how the GM can transform TCM into functional metabolites by taking polysaccharides, flavonoids, polyphenols, and saponin (Table 2).
2.2.1.1 Polysaccharides
A considerable portion of TCM comprises polysaccharides, and they are reported to possess prebiotic effects (Khan et al., 2018; Xia et al., 2020b). Polysaccharides possess anticancer properties (Zhang et al., 2018), antiobesity effects (Wu et al., 2019), and anti-inflammatory effects (Niu et al., 2021). Due to the limited digestive enzymes encoded in the human genome, polysaccharides remained undigested until they reach the colon (Baumann and Bisping, 1995), where they degrade into fermentable oligosaccharides, such as β-glucans, and are broken down by microbial saccharolytic machinery (Lin et al., 2021; Niu et al., 2021). Polysaccharides are also converted to SCFAs and other secondary metabolites (Niu et al., 2021). SCFAs are a source of energy for colonocytes, regulate the immune system, and correct metabolic disorders (Sun et al., 2017). In addition, SCFAs contribute to the gut barrier construction via enhancement of the expression of MUC 2, modulation of oxidative stress, and upregulation of TJs (Wang et al., 2012; van der Beek et al., 2017).
2.2.1.2 Flavonoids
Flavonoids are important ingredients of TCM, usually combined with carbohydrates to form glycosides. Inside the gastrointestinal tract, flavonoids are converted into various by-products through the action of the GM and, hence, affect flavonoids’ health-related abilities for the host (Chiou et al., 2014). Bacteria that can convert dietary flavonoids include Bacteroides uniformis, Bacteroides ovatus, Bifidobacterium adolescentis, Enterococcus casseliflavus, Enterococcus avium, Flavonifractor plautii, Lactobacillus plantarum IAM, Parabacteroides distasonis, Eubacterium cellulosolvens, E. coli ATCC BAA-97 and several others (Braune and Blaut, 2016). These bacteria convert dietary flavonoids through several enzymatic reactions that include O-Deglycosylation, C-Ring cleavage, Reduction, O-Desmethylangolensin cleavage, and Dehydroxylation. Most of the bacteria are capable of flavonoid conversion through O-deglycosylation (Braune and Blaut, 2016).
Through GM-secreted enzymes, the glycosyl is removed to form aglycon, which can then be absorbed by the body. Bacterial enzymes, such as α-rhamnosidase, exo-β-glucosidase, and endo-β-glucosidase, transform rutin, hesperidin, naringin, and poncirin into their aglycones, which have more of an antiplatelet effect and cytotoxicity than their parental compounds (Kim et al., 1998). Trinh et al. found that the products of baicalin (a flavone glycoside) when undergoing physiological changes exerted by the GM result in deglycosylated baicalein, methylated aglycon, and oroxylin-A. It is noticed that baicalin and oroxylin-A are more potent than the parental compound (Trinh et al., 2010). Another flavanol, named kaempferol, is reported to lower the tumor burden in the host by promoting the abundance of beneficial bacterial that were involved in secondary bile acid synthesis. These changes were concurrently accompanied with improved expression of the farnesoid X receptor (FXR), a main regulator in bile acid signaling (Li et al., 2022).
2.2.1.3 Polyphenol
Polyphenol, one of the most important secondary metabolites of plants, has received increasing awareness in recent years. Since most polyphenols have lower bioavailability and they reach the colon, a densely inhabited part of the gastrointestinal tract, thus, a bidirectional interaction between the GM and polyphenols commences. GM convert polyphenols to aglycones by removing organic acids, glucuronides, and glycosides (Makarewicz et al., 2021). The GM can convert polyphenols through several enzymatic processes to various metabolites. For instance, enterolactones are produced from lignans, equol is produced from daidzin, and urolithins from ellagitannins (Espín et al., 2017). Contrarily, polyphenols can also affect GM composition by promoting the growth of beneficial bacteria, such as Akkermansia muciniphila, Lactobacillus reuteri, Lactobacillus acidophilus, and Faecalibacterium prausnitzii (Cueva et al., 2017; Espín et al., 2017; Gowd et al., 2019; Liu et al., 2020). In addition to the growth-promoting abilities of polyphenols, these compounds are also antimicrobial in nature and can inhibit the growth of bacteria, mostly potential pathogens (Rodríguez-Daza et al., 2021).
Besides this, microbes also produce phenolic metabolites that possess antioxidant, anti-inflammatory, and antiproliferative activities (Saha et al., 2016). This metabolite production is dependent on the (poly)phenol-associated enzymes produce by the GM. Interindividual differences of the GM are connected to different metabotypes, which are related to different health outcomes in people after taking polyphenol (Cortés-Martín, 2020). In short, among the gut-dwelling bacteria, it is beneficial for the host to inhabit (poly)phenol-degrading bacteria in the gut that could ensure the bioconversion of polyphenols and enhance the host’s health.
2.2.1.4 Saponin
Saponins are markedly studied and practiced in TCM for various therapeutic purposes. More recently, it has been observed that the therapeutic ability of saponin, at least partly, is through improving GM composition (Chen L. et al., 2016; Huang et al., 2017; Khan et al., 2019; Xu et al., 2020). As with several other TCM constituents, the absorption rate of saponin in the human body is exceedingly low, and therefore, the bioconversion of saponins through microbes (such as Aspergillus sp., Bacillus sp., and lactic acid–producing bacteria) is gaining popularity. Microbes mainly hydrolyze the glycosyl group of the saponin. For instance, protopanaxatriol ginsenosides are hydrolyzed into G-Rh1 and G-F1 when interacting with the GM in the gut (Tawab et al., 2003).
The gut-residing microbes harbor a variety of enzymes that metabolize saponin into by-products. For instance, Bifidobacterium, Bacteroides, and Prevotella species encode α-arabinopyranosidase, β-glucosdiase, and α-arabinofuranosidase enzymes that can cleave the sugar moiety and hydrolyze Protopanaxadiol-type ginsenoside into monoglucosylated ginsenoside compound K (Karikura, 1992; Hasegawa et al., 1996; Park et al., 2000; Eun-Ah Bae, 2002). In addition, B. adolescentis and L. rhamnosus are also reported for the bioconversion of saponin in the host gut (Wang et al., 2021). A pharmacokinetic study through an oral treatment with a ginseng saponin fraction confirmed that ginsenosides Rh1, F1, and compound K are the metabolites for parental ginsenosides by GM (Tawab et al., 2003). The metabolites, such as compound K, ginsenoside Rh2, and protopanaxatriol, have potent cytotoxicity against tumor cells, which may suggest the GM has a crucial role in exploiting the advantage of the bioactive compounds of ginsengs in full (Kim, 2018).
Importantly, the differences in GM composition among individuals is related to different metabolite outcomes. For instance, in a control setup, individuals who consume a fat- and protein-rich diet carry different microbes in the gut and, thus, higher concentrations of GF1 and GC-K metabolites were noticed after taking Panax notoginseng saponins. However, people who ate a fibrous diet had more GRh2, PPT, and PPD metabolites after taking Panax notoginseng saponins (Wang et al., 2021).
2.2.2 GM can detoxify lower grade TCM
In “Sheng Nong’s herbal classic,” TCM is divided into three grades. Among them, the lower grade is more toxic, and it is often used after concocting to reduce its toxicity. It is recently reported that the GM can contribute to the detoxification of TCM. For instance, the GM lowers the toxicity of diester diterpene alkaloids by partly converting them to mono-ester aconitum alkaloids and lipo-alkaloids. Diester diterpene alkaloids are the main components of the radix aconiti, Kusnezoff monkshood, and Aconitum carmichali debx (Zhao et al., 2007; Wang et al., 2015; Yang et al., 2018). Another typical case is Baicalin, a glycoside present in Scutellaria baicalensis Georgi, which is converted to baicalein, a flavone with lower cytotoxic side effects (Khanal et al., 2012). However, the GM should be taken carefully in the context of reducing the toxicity of lower grade TCM. A host could inhabit certain bugs that could worsen the toxicity of a compound. The GM role has been suspected in elevating the toxicity of amygdalin that is extracted from Armeniacae Amarae Semen (Carter et al., 1980).
3 A complex interaction and collaboration between TCM–GM and Western medicine
In TCM classic theories, once TCM is combined, some will increase toxicity and some will reduce others’ efficacy (Zhou et al., 2017), which are the so-called 18 incompatible medicaments (ShiBaFan) and 19 medicaments of mutual restraint (ShiJiuWei) principles. To achieve a better curative effect and avoid side effects, combined TCM should be under the guidance of compatibility theories. Modern pharmacology reveals the rationality of the compatibility of TCM, besides this, from another angle, and GM modulation also explains the compatibility theory.
Even in the case of Western drugs, not all of them work for every patient, and a considerable number of patients are nonresponsive; this observation is known as nonresponse bias. And it is a growing consensus that the nonresponders are missing some important bacterial species in their gut. For instance, the efficacy of cyclophosphamide, an anticancer drug, is dependent on the presence of Barnesiella intestinihominis and Enterococcus hirae in the gut of a patient (Daillère et al., 2016). Not only GM but TCM has also been taken for consideration of enhancing the effectiveness of Western medicine. In one such case, a group of researchers found improved efficacy of the antiprogrammed cell death 1/programmed cell death ligand 1 (anti-PD-1/PD-L1) in the presence of ginseng polysaccharides. It was further confirmed that Parabacteroides distasonis and Bacteroides vulgatus were dominating the gut of the treated patient. Those patients who did not respond to the combinatorial treatment of the anti-PD-1/PD-L1 and ginseng polysaccharides had a depleted abundance of the Parabacteroides distasonis and Bacteroides vulgatus (Huang J. et al., 2021).
4 GM-based investigation of medicine’s efficacy – an exciting prospect for TCM–GM research
Nowadays, with the continuous development of culture, multi-omics combination and gene sequencing technologies, the exploration of TCM and microbiota is making exciting discoveries. The mechanism behind TCM theory about Qi, Xue, Ying, Yang, four properties and five flavors have received much attention. TCM regulates the composition and metabolites of intestinal flora, which can be regarded as one of the mechanisms for expounding the efficacy of TCM. We predict that one of the emerging research areas in TCM–GM research will be the investigation of the GM’s role in TCM’s efficacy. Particularly for those therapeutics that are taken orally. By taking the studies carried out on Western drugs as an example, here we try to explain how much the GM could contribute to the efficacy of the medicine. This could help TCM researchers to orient their research.
(1) As an example, we discuss a therapeutic approach that is based upon the development of antibodies to block CTLA-4, known as the cluster of differentiation 152 (a protein receptor working as an immune checkpoint), for the treatment of cancer. This technique is a current hot topic and has shown promising results in clinical trials. During this process, the body’s T cells are recruited against tumors that pose limited damage to the normal cells (Sivan et al., 2015). Preclinical studies have shown that the efficacy of anti-CTLA-4 therapy is dependent upon the composition of the GM. For instance, it has been discovered that anti-PD-L1 (an antibody that blocks CTLA-4) efficacy improves in the presence of Bifidobacterium. Oral administration of anti-PD-1 and Bifidobacterium has been observed with augmented dendritic cell function and improved CD8+ T cell priming in the tumor microenvironment (Sivan et al., 2015). Besides this, anti-CTLA-4 therapies have also shown dependence on the presence of Bacteroides thetaiotaomicron or Bacteroides fragilis. Nonetheless, the anti-CTLA-4 therapy has failed in germ-free mice and those treated with antibiotics (Vétizou et al., 2015). More recently, these preclinical experiments were reproduced in melanoma patients and it was found that patients who responded effectively to anti-CTLA-4 treatments were harboring enriched bacterial diversity belonging to the family Ruminococcaceae (Gopalakrishnan et al., 2018).
(2) As a second example, we discuss the efficacy of cyclophosphamide, an anticancer immune suppressant chemotherapeutic that also remodels the gut microbial composition. During cyclophosphamide treatment, the gram-positive bacteria translocate into the lymphoid organ and mimic the production of pathogenic T helper 17 cells and memory Th1 immune responses C. Especially, cyclophosphamide efficacy is dependent on two intestinal commensals known as Barnesiella intestinihominis and Enterococcus hirae. During cyclophosphamide therapy, Barnesiella intestinihominis accumulates in the colon and infiltrates γδT cells in the cancer lesion, whereas Enterococcus hirae translocates to secondary lymphoid organs and stimulates intratumoral CD8/Treg ratio (Daillère et al., 2016). Similarly, another study reports the interconversion of fluoropyrimidines (the first-line anticancer drug) by gut microbial vitamin B6, B9, and ribonucleotide metabolism (Scott et al., 2017). Microbial influences on the efficacy of chemotherapeutic drugs, 5-fluoro-2′-deoxyuridine, and 5-fluorouracil are also reported (García-González et al., 2017).
It is important to mention that microbiome-based therapies should be tailored to disease types and affected body sites. For example, men with metastatic prostate tumors who responded to checkpoint inhibition have been found to have lower levels of a microbe called Akkermansia muciniphila in their stool than men who did not respond. But the opposite is true of people with lung and kidney cancers: Those with more A. muciniphila in their guts tended to fare better on the therapy (Dolgin, 2020).
5 TCM–GM in mental disease
TCM has been used in treating neuropsychiatric diseases for thousands of years. Synopsis of Golden Chamber (a classic Chinese medicine book written by Zhang Zhongjing in the Han Dynasty) recorded a disease, Zang Zao, in which the main symptoms are depression of spirit, emotional disturbance, weeping and laughing hysterically. Although this kind of disease cannot be solved thoroughly, it can be alleviated effectively by the TCM formula Ganmai Dazao Decoction. Nowadays, lots of evidence uncovers TCM exerting alteration and restoration function of GM. In addition, the altered microbiome, through repairing the gut barrier, regulates the gut permeability, alleviating the inflammation and other potential mechanisms to cure or relieve the symptoms and disease. A gradual but strong link is establishing the connection of TCM, GM, and mental disease. For instance, it is demonstrated that TCM treatment of chronic unpredictable mild stress (CUMS) rats altered the abundance of Ruminococcus and Roseburia and potentially increased the expression of cysteine [83]. Meanwhile, N-acetylcysteine is considered beneficial for brain disorders [84].
In addition, it is reported that GM diversity has a strong association with insular resting state functional connectivity. A higher fecal bacterial microbiota diversity is linked to a higher resting state insular functional connectivity [78]. Interestingly, the fecal microbiota-derived indole metabolites are found to associate with functional and anatomical connectivity of the amygdala and anterior insular nucleus [79]. For example, Bacteroides, Parabacteroides and Escherichia species can promote production of the γ-aminobutyric acid (GABA). Particularly, a lower abundance of Bacteroides was found in depression patients [80]. A series of human mental diseases (such as posttraumatic stress disorder, bipolar disorder, anxiety, and stress, and so on) are associated with altered GM diversity [81].
Recent research shows that gut bacteria are directly sensed by hypothalamic neurons through bacteria muropeptides to regulate host feeding behavior. In this process, muropeptides are recognized by cytosolic Nod-like receptors, which are expressed by a subset of hypothalamic neurons. This subset also responds to muramyl dipeptide from the intestine, thus regulating food intake and associated behaviors [82]. It is revealed that Nod 2 mutation has a strong association with bipolar disorder, schizophrenia, and Parkinson’s disease [82].
6 Conclusion
TCM–GM interaction is a fertile research field for identifying the faulted signaling pathways during disease and finding a treatment for them by manipulating GM, and this could provide a modern framework for evaluation and validation of TCM. It is well-known that TCM exerts therapeutic abilities that are holistic in nature, which could be impractical to comprehend with conventional research tools. Therefore, we propose that the integration of TCM with GM research can target the wholeness of a biological system. In a few cases, the integration of GM with TCM and other natural products have already made landmark achievements in the field of diseases prognosis and treatment.
Author contributions
WX and BL wrong a majjor part of the manuscript, ST collected the information, MY revised the manuscript, IK supervised the data and the manuscript. All authors contributed to the article and approved the submitted version.
Funding
This project was funded by the Deanship of the Scientific Research (DSR), King Abdulaziz University, Jeddah, KSA, under the grant no. DF-244-141-1441.
Conflict of interest
The authors declare that the research was conducted in the absence of any commercial or financial relationships that could be construed as a potential conflict of interest.
Publisher’s note
All claims expressed in this article are solely those of the authors and do not necessarily represent those of their affiliated organizations, or those of the publisher, the editors and the reviewers. Any product that may be evaluated in this article, or claim that may be made by its manufacturer, is not guaranteed or endorsed by the publisher.
Abbreviations
CRC, Colorectal Cancer; FXR, farnesoid X receptor; GABA, g-aminobutyric acid; GM, gut microbiome; PC, Pogostemon cablin; SCFAs, Short-chain fatty acids; TCM, Traditional Chinese Medicine; TJ, Tight Junction; TLR-4, M toll like receptor.
References
Baumann, U., Bisping, B. (1995). Proteolysis during tempe fermentation. Food Microbiol. 12, 39–47. doi: 10.1016/S0740-0020(95)80077-8
Braune, A., Blaut, M. (2016). Bacterial species involved in the conversion of dietary flavonoids in the human gut. Gut Microbes 7, 216–234. doi: 10.1080/19490976.2016.1158395
Cani, P. D. (2009). Gut microbiota and pregnancy, a matter of inner life. Br. J. Nutr. 101, 1579–1580. doi: 10.1017/S0007114508111485
Carter, J. H., McLafferty, M. A., Goldman, P. (1980). Role of the gastrointestinal microflora in amygdalin (laetrile)-induced cyanide toxicity. Biochem. Pharmacol. 29, 301–304. doi: 10.1016/0006-2952(80)90504-3
Chen, L., Brar, M. S., Leung, F. C. C., Hsiao, W. L. W. (2016). Triterpenoid herbal saponins enhance beneficial bacteria, decrease sulfate-reducing bacteria, modulate inflammatory intestinal microenvironment and exert cancer preventive effects in ApcMin/+ mice. Oncotarget 7, 31226–31242. doi: 10.18632/oncotarget.8886
Chen, H., Sang, S. (2014). Biotransformation of tea polyphenols by gut microbiota. J. Funct. Foods 7, 26–42. doi: 10.1016/j.jff.2014.01.013
Chen, F., Wen, Q., Jiang, J., Li, H. L., Tan, Y. F., Li, Y. H., et al. (2016). Could the gut microbiota reconcile the oral bioavailability conundrum of traditional herbs? J. Ethnopharmacol. 179, 253–264. doi: 10.1016/j.jep.2015.12.031
Chiou, Y. S., Wu, J. C., Huang, Q., Shahidi, F., Wang, Y. J., Ho, C. T., et al. (2014). Metabolic and colonic microbiota transformation may enhance the bioactivities of dietary polyphenols. J. Funct. Foods 7, 3–25. doi: 10.1016/j.jff.2013.08.006
Cortés-Martín, A. (2020). Where to look into the puzzle of Polyphenols and health? The postbiotics and gut microbiota associated with human metabotypes. Mol. Nutr. Food Res 64 (9), e1900952. doi: 10.1002/mnfr.201900952
Cueva, C., Gil-Sánchez, I., Ayuda-Durán, B., González-Manzano, S., González-Paramás, A. M., Santos-Buelga, C., et al. (2017). An integrated view of the effects of wine polyphenols and their relevant metabolites on gut and host health. Molecules 22, 1–15. doi: 10.3390/molecules22010099
Daillère, R., Vétizou, M., Waldschmitt, N., Yamazaki, T., Isnard, C., Poirier-Colame, V., et al. (2016). Enterococcus hirae and barnesiella intestinihominis facilitate cyclophosphamide-induced therapeutic immunomodulatory effects. Immunity 45, 931–943. doi: 10.1016/j.immuni.2016.09.009
Dolgin, E. (2020). Microbe fighting with cancer. Nature 577, S16–S18. doi: 10.1038/d41586-020-00199-x
El Kaoutari, A., Armougom, F., Gordon, J. I., Raoult, D., Henrissat, B. (2013). The abundance and variety of carbohydrate-active enzymes in the human gut microbiota. Nat. Rev. Microbiol. 11, 497–504. doi: 10.1038/nrmicro3050
El-Mekkawy, S., Meselhy, M. R., Kawata, Y., Kadota, S., Hattori, M., Namba, T., et al (1993). Metabolism of strychnine N-oxide and brucine N-oxide by human intestinal bacteria. Planta Med. 59 (4), 347–350. doi: 10.1055/s-2006-959698
Espín, J. C., González-Sarrías, A., Tomás-Barberán, F. A. (2017). The gut microbiota: A key factor in the therapeutic effects of (poly)phenols. Biochem. Pharmacol. 139, 82–93. doi: 10.1016/j.bcp.2017.04.033
Eun-Ah Bae, S.-Y. P. (2002). Constitutive beta-glucosidases hydrolyzing gingsenoside rb1 and rb2 from human intestinal bacteria. Chem. Pharm. Bull. 2091, 1481–1485. doi: 10.1248/bpb.23.1481
Feng, S., Han, M., Lai, L., Wang, S. C., Liu, J. P. (2017). Research capacity at traditional Chinese medicine (TCM) centers in China: A survey of clinical investigators. Evidence-Based Complement. Altern. Med. 2017, 1–8. doi: 10.1155/2017/4231680
Feng, R., Shou, J. W., Zhao, Z. X., He, C. Y., Ma, C., Huang, M., et al. (2015). Transforming berberine into its intestine-absorbable form by the gut microbiota. Sci. Rep. 5, 1–15. doi: 10.1038/srep12155
García-González, A. P., Ritter, A. D., Shrestha, S., Andersen, E. C., Yilmaz, L. S., Walhout, A. J. M. (2017). Bacterial metabolism affects the c. elegans response to cancer chemotherapeutics. Cell 169, 431–441.e8. doi: 10.1016/j.cell.2017.03.046
Gopalakrishnan, V., Spencer, C. N., Nezi, L., Reuben, A., Andrews, M. C., Karpinets, T. V., et al. (2018). Gut microbiome modulates response to anti-PD-1 immunotherapy in melanoma patients. Science 359, 97–103. doi: 10.1126/science.aan4236
Gowd, V., Karim, N., Shishir, M. R. I., Xie, L., Chen, W. (2019). Dietary polyphenols to combat the metabolic diseases via altering gut microbiota. Trends Food Sci. Technol. 93, 81–93. doi: 10.1016/j.tifs.2019.09.005
Han, R., Qiu, H., Zhong, J., Zheng, N., Li, B., Hong, Y., et al. (2021). Si Miao formula attenuates non-alcoholic fatty liver disease by modulating hepatic lipid metabolism and gut microbiota. Phytomedicine 85, 153544. doi: 10.1016/j.phymed.2021.153544
Hasegawa, H., Sung, J. H., Matsumiya, S., Uchiyama, M. (1996). Main ginseng saponin metabolites formed by intestinal bacteria. Planta Med. 62, 453–457. doi: 10.1055/s-2006-957938
Hattori, M., Namba, T., Akao, T., Kobashi, K. (1988). Metabolism of sennosides by human intestinal bacteria. Pharmacology 36 (Suppl 1), 172–179. doi: 10.1159/000138437
Huang, G., Khan, I., Li, X., Chen, L., Leong, W., Ho, L. T., et al. (2017). Ginsenosides Rb3 and Rd reduce polyps formation while reinstate the dysbiotic gut microbiota and the intestinal microenvironment in ApcMin/+mice. Sci. Rep. 7, 1–14. doi: 10.1038/s41598-017-12644-5
Huang, J., Liu, D., Wang, Y., Liu, L., Li, J., Yuan, J., et al. (2021). Ginseng polysaccharides alter the gut microbiota and kynurenine/tryptophan ratio, potentiating the antitumour effect of antiprogrammed cell death 1/programmed cell death ligand 1 (anti-PD-1/PD-L1) immunotherapy. Gut 71 (4), 1–12. doi: 10.1136/gutjnl-2020-321031
Huang, K., Zhang, P., Zhang, Z., Youn, J. Y., Wang, C., Zhang, H., et al. (2021). Traditional Chinese medicine (TCM) in the treatment of COVID-19 and other viral infections: Efficacies and mechanisms. Pharmacol. Ther. 225, 107843. doi: 10.1016/j.pharmthera.2021.107843
Hugon, P., Dufour, J. C., Colson, P., Fournier, P. E., Sallah, K., Raoult, D. (2015). A comprehensive repertoire of prokaryotic species identified in human beings. Lancet Infect. Dis. 15, 1211–1219. doi: 10.1016/S1473-3099(15)00293-5
Ji, C., Deng, Y., Yang, A., Lu, Z., Chen, Y., Liu, X., et al. (2020). Rhubarb enema improved colon mucosal barrier injury in 5/6 nephrectomy rats may associate with gut microbiota modification. Front. Pharmacol. 11. doi: 10.3389/fphar.2020.01092
Joshi, K., Ghodke, Y., Shintre, P. (2010). Traditional medicine and genomics. J. Ayurveda Integr. Med. 1, 26–32. doi: 10.4103/0975-9476.59824
Kang, M. J., Khanal, T., Kim, H. G., Lee, D. H., Yeo, H. K., Lee, Y. S., et al. (2012). Role of metabolism by human intestinal microflora in geniposideinduced toxicity in HepG2 cells. Arch. Pharm. Res. 35, 733–738. doi: 10.1007/s12272-012-0418-y
Karikura, M. (1992). Study on absorption distribution,excretion and metabolism of gingseng sapoins.isotope labeling of gingseng rb2. Chem. Pharm. Bull. 2091, 2458–2460. doi: 10.1248/cpb.40.2458
Khanal, T., Kim, H. G., Choi, J. H., Park, B. H., Do, M. T., Kang, M. J., et al. (2012). Protective role of intestinal bacterial metabolism against baicalin-induced toxicity in HepG2 cell cultures. J. Toxicol. Sci. 37, 363–371. doi: 10.2131/jts.37.363
Khan, I., Huang, G., Li, X., Leong, W., Xia, W., Hsiao, W. L. W. (2018). Mushroom polysaccharides from ganoderma lucidum and poria cocos reveal prebiotic functions. J. Funct. Foods 41, 191–201. doi: 10.1016/j.jff.2017.12.046
Khan, I., Huang, G., Li, X., Liao, W., Leong, W. K., Xia, W., et al. (2019). Mushroom polysaccharides and jiaogulan saponins exert cancer preventive effects by shaping the gut microbiota and microenvironment in apc Min / + mice. Pharmacol. Res. 148, 104448. doi: 10.1016/j.phrs.2019.104448
Kim, D. H. (2018). Gut microbiota-mediated pharmacokinetics of ginseng saponins. J. Ginseng Res. 42, 255–263. doi: 10.1016/j.jgr.2017.04.011
Kim, D. H., Jung, E. A., Sohng, I. S., Han, J. A., Kim, T. H., Han, M. J. (1998). Intestinal bacterial metabolism of flavonoids and its relation to some biological activities. Arch. Pharm. Res. 21, 17–23. doi: 10.1007/BF03216747
Knight, R., Callewaert, C., Marotz, C., Hyde, E. R., Debelius, J. W., McDonald, D., et al. (2017). The microbiome and human biology. Annu. Rev. Genomics Hum. Genet. 18, 65–86. doi: 10.1146/annurev-genom-083115-022438
Lam, W., Bussom, S., Guan, F., Jiang, Z., Zhang, W., Gullen, E. A., et al. (2010). The four-herb Chinese medicine PHY906 reduces chemotherapy-induced gastrointestinal toxicity. Sci. Transl. Med. 2, 45ra59 LP–45ra59. doi: 10.1126/scitranslmed.3001270
Leong, W., Huang, G., Khan, I., Xia, W., Li, Y., Liu, Y., et al. (2019). Patchouli essential oil and its derived compounds revealed prebiotic-like effects in C57BL/6J mice. Front. Pharmacol. 10. doi: 10.3389/fphar.2019.01229
Liang, Y., Zhang, Y., Deng, Y., Liang, S., He, Y., Chen, Y., et al. (2018). Chaihu-Shugan-San decoction modulates intestinal microbe dysbiosis and alleviates chronic metabolic inflammation in NAFLD rats via the NLRP3 inflammasome pathway. Evidence-Based Complement. Altern. Med. 2018, 9390786. doi: 10.1155/2018/9390786
Li, X., Khan, I., Huang, G., Lu, Y., Wang, L., Liu, Y., et al. (2022). Kaempferol acts on bile acid signaling and gut microbiota to attenuate the tumor burden in ApcMin/+ mice. Eur. J. Pharmacol. 918, 174773. doi: 10.1016/j.ejphar.2022.174773
Li, Y., Liao, Q., Lin, M., Zhong, D., Wei, L., Han, B., et al. (2015). An integrated metabonomics and microbiology analysis of host-microbiota metabolic interactions in rats with coptis chinensis-induced diarrhea. RSC Adv. 5, 79329–79341. doi: 10.1039/c5ra16338b
Li, M. Y., Luo, H. J., Wu, X., Liu, Y. H., Gan, Y. X., Xu, N., et al. (2020). Anti-inflammatory effects of huangqin decoction on dextran sulfate sodium-induced ulcerative colitis in mice through regulation of the gut microbiota and suppression of the ras-PI3K-Akt-HIF-1a and NF-κB pathways. Front. Pharmacol. 10. doi: 10.3389/fphar.2019.01552
Lin, T. L., Lu, C. C., Lai, W. F., Wu, T. S., Lu, J. J., Chen, Y. M., et al. (2021). Role of gut microbiota in identification of novel TCM-derived active metabolites. Protein Cell 12, 394–410. doi: 10.1007/s13238-020-00784-w
Liu, D., Lin, L., Lin, Y., Zhong, Y., Zhang, S., Liu, W., et al. (2019). Zengye decoction induces alterations to metabolically active gut microbiota in aged constipated rats. Biomed. Pharmacother. 109, 1361–1371. doi: 10.1016/j.biopha.2018.11.013
Liu, Y. C., Li, X. Y., Shen, L. (2020). Modulation effect of tea consumption on gut microbiota. Appl. Microbiol. Biotechnol. 104, 981–987. doi: 10.1007/s00253-019-10306-2
Lu, F., Li, Y., Wang, X., Hu, X., Liao, X., Zhang, Y. (2021). Early-life polyphenol intake promotes akkermansia growth and increase of host goblet cells in association with the potential synergistic effect of lactobacillus. Food Res. Int. 149, 110648. doi: 10.1016/j.foodres.2021.110648
Luo, S., Wen, R., Wang, Q., Zhao, Z., Nong, F., Fu, Y., et al. (2019). Rhubarb peony decoction ameliorates ulcerative colitis in mice by regulating gut microbiota to restoring Th17/Treg balance. J. Ethnopharmacol. 231, 39–49. doi: 10.1016/j.jep.2018.08.033
Maeda, Y., Takeda, K. (2017). Role of gut microbiota in rheumatoid arthritis. J. Clin. Med. 6, 1–7. doi: 10.3390/jcm6060060
Makarewicz, M., Drożdż, I., Tarko, T., Duda-Chodak, A. (2021). The interactions between polyphenols and microorganisms, especially gut microbiota. Antioxidants 10, 1–70. doi: 10.3390/antiox10020188
Makki, K., Deehan, E. C., Walter, J., Bäckhed, F. (2018). The impact of dietary fiber on gut microbiota in host health and disease. Cell Host Microbe 23, 705–715. doi: 10.1016/j.chom.2018.05.012
McFadden, R.-M. T., Larmonier, C. B., Shehab, K. W., Midura-Kiela, M., Ramalingam, R., Harrison, C. A., et al. (2015). The role of curcumin in modulating colonic microbiota during colitis and colon cancer prevention. Inflamm. Bowel Dis. 21, 2483–2494. doi: 10.1097/MIB.0000000000000522
Moco, S., Martin, F. P. J., Rezzi, S. (2012). Metabolomics view on gut microbiome modulation by polyphenol-rich foods. J. Proteome Res. 11, 4781–4790. doi: 10.1021/pr300581s
Musso, G., Gambino, R., Cassader, M. (2010). Obesity, diabetes, and gut microbiota: The hygiene hypothesis expanded? Diabetes Care 33, 2277–2284. doi: 10.2337/dc10-0556
Niu, W., Chen, X., Xu, R., Dong, H., Yang, F., Wang, Y., et al. (2021). Polysaccharides from natural resources exhibit great potential in the treatment of ulcerative colitis: A review. Carbohydr. Polym. 254, 117189. doi: 10.1016/j.carbpol.2020.117189
Ostaff, M. J., Stange, E. F., Wehkamp, J. (2013). Antimicrobial peptides and gut microbiota in homeostasis and pathology. EMBO Mol. Med. 5, 1465–1483. doi: 10.1002/emmm.201201773
Park, J. D., Lee, Y. H., Kim, S. I. I. (2000). Ginsenoside Rf2, a new dammarane glycoside from Korean red ginseng (Panax ginseng. Arch. Pharm. Res. 21, 615–617. doi: 10.1007/BF02975384
Rodríguez-Daza, M. C., Pulido-Mateos, E. C., Lupien-Meilleur, J., Guyonnet, D., Desjardins, Y., Roy, D. (2021). Polyphenol-mediated gut microbiota modulation: Toward prebiotics and further. Front. Nutr. 8. doi: 10.3389/fnut.2021.689456
Saha, P., Yeoh, B. S., Singh, R., Chandrasekar, B., Vemula, P. K., Haribabu, B., et al. (2016). Gut microbiota conversion of dietary ellagic acid into bioactive phytoceutical urolithin a inhibits heme peroxidases. PloS One 11, 1–21. doi: 10.1371/journal.pone.0156811
Scott, T. A., Quintaneiro, L. M., Norvaisas, P., Lui, P. P., Wilson, M. P., Leung, K. Y., et al. (2017). Host-microbe Co-metabolism dictates cancer drug efficacy in c. elegans. Cell 169, 442–456.e18. doi: 10.1016/j.cell.2017.03.040
Sivan, A., Corrales, L., Hubert, N., Williams, J. B., Aquino-Michaels, K., Earley, Z. M., et al. (2015). Commensal bifidobacterium promotes antitumor immunity and facilitates anti-PD-L1 efficacy. Science 350, 1084–1089. doi: 10.1126/science.aac4255
Stefka, A. T., Feehley, T., Tripathi, P., Qiu, J., McCoy, K., Mazmanian, S. K., et al. (2014). Commensal bacteria protect against food allergen sensitization. Proc. Natl. Acad. Sci. U. S. A. 111, 13145–13150. doi: 10.1073/pnas.1412008111
Sun, Q., Cheng, L., Zeng, X., Zhang, X., Wu, Z., Weng, P. (2020). The modulatory effect of plant polysaccharides on gut flora and the implication for neurodegenerative diseases from the perspective of the microbiota-gut-brain axis. Int. J. Biol. Macromol. 164, 1484–1492. doi: 10.1016/j.ijbiomac.2020.07.208
Sun, J., Kato, I. (2016). Gut microbiota, inflammation and colorectal cancer. Genes Dis. 3, 130–143. doi: 10.1016/j.gendis.2016.03.004
Sun, M., Wu, W., Liu, Z., Cong, Y. (2017). Microbiota metabolite short chain fatty acids , GPCR , and inflammatory bowel diseases. J. Gastroenterol. 52, 1–8. doi: 10.1007/s00535-016-1242-9
Takayama, K., Tsutsumi, H., Ishizu, T., Okamura, N. (2012). The influence of rhein 8-O-β-D-glucopyranoside on the purgative action of sennoside a from rhubarb in mice. Biol. Pharm. Bull. 35, 2204–2208. doi: 10.1248/bpb.b12-00632
Tawab, M. A., Bahr, U., Karas, M., Wurglics, M., Schubert-Zsilavecz, M. (2003). Degradation of ginsenosides in humans after oral administration. Drug Metab. Dispos. 31, 1065–1071. doi: 10.1124/dmd.31.8.1065
Tian., B., Liu, M., An, W., Yu, L., Zhang, J., Liu, Y., et al (2020). Lycium barbarum relieves gut microbiota dysbiosis and improves colonic barrier function in mice following antibiotic perturbation. J. Funct. Foods 71, 1756–4646. doi: 10.1016/j.jff.2020.103973
Trinh, H. T., Joh, E. H., Kwak, H. Y., Baek, N. I., Kim, D. H. (2010). Anti-pruritic effect of baicalin and its metabolites, baicalein and oroxylin a, in mice. Acta Pharmacol. Sin. 31, 718–724. doi: 10.1038/aps.2010.42
van der Beek, C. M., Dejong, C. H. C., Troost, F. J., Masclee, A. A. M., Lenaerts, K. (2017). Role of short-chain fatty acids in colonic inflammation, carcinogenesis, and mucosal protection and healing. Nutr. Rev. 75, 286–305. doi: 10.1093/nutrit/nuw067
Vétizou, M., Pitt, J. M., Daillère, R., Lepage, P., Waldschmitt, N., Flament, C., et al. (2015). Anticancer immunotherapy by CTLA-4 blockade relies on the gut microbiota. Science 350, 1079–1084. doi: 10.1126/science.aad1329
Viaud, S., Saccheri, F., Mignot, G., Yamazaki, T., Daillère, R., Hannani, D., et al. (2013). The intestinal microbiota modulates the anticancer immune effects of cyclophosphamide. Science 342, 971–976. doi: 10.1126/science.1240537
Wang, L., Chen, M. Y., Shao, L., Zhang, W., Li, X. P., Huang, W. H. (2021). Personalized bioconversion of panax notoginseng saponins mediated by gut microbiota between two different diet-pattern healthy subjects. Chin. Med. (United Kingdom) 16, 1–14. doi: 10.1186/s13020-021-00476-5
Wang, Y., Shou, J., Jiang, J. (2015). Metabolism of Chinese materia medica in gut microbiota and its biological effects. Chin. Herb. Med. 7, 109–115. doi: 10.1016/s1674-6384(15)60027-2
Wang, H. B., Wang, P. Y., Wang, X., Wan, Y. L., Liu, Y. C. (2012). Butyrate enhances intestinal epithelial barrier function via up-regulation of tight junction protein claudin-1 transcription. Dig. Dis. Sci. 57, 3126–3135. doi: 10.1007/s10620-012-2259-4
Wei, X., Tao, J., Xiao, S., Jiang, S., Shang, E., Zhu, Z., et al. (2018). Xiexin tang improves the symptom of type 2 diabetic rats by modulation of the gut microbiota. Sci. Rep. 8, 1–12. doi: 10.1038/s41598-018-22094-2
Wilson, I. D., Nicholson, J. K. (2017). Gut microbiome interactions with drug metabolism , efficacy and toxicity Europe PMC funders author manuscripts the gut microbiota have the capability of preforming a wide range of metabolic reactions on. Transl. Res. 179, 204–222. doi: 10.1016/j.trsl.2016.08.002.Gut
Wong, J. M. W., de Souza, R., Kendall, C. W., Emam, A., Jenkins, D. J. A. (2006). Colonic health: Fermentation and short chain fatty acids. J. Clin. Gastroenterol. 40, 235–243. doi: 10.3319/TAO.1998.9.3.395(TAICRUST
Wu, T. R., Lin, C. S., Chang, C. J., Lin, T. L., Martel, J., Ko, Y. F., et al. (2019). Gut commensal parabacteroides goldsteinii plays a predominant role in the anti-obesity effects of polysaccharides isolated from hirsutella sinensis. Gut 68, 248–262. doi: 10.1136/gutjnl-2017-315458
Wu, X. M., Tan, R. X. (2019). Interaction between gut microbiota and ethnomedicine constituents. Nat. Prod. Rep. 36, 788–809. doi: 10.1039/c8np00041g
Xia, W., Khan, I., Li, X., Huang, G., Yu, Z., Kit, W., et al. (2020a). Adaptogenic fl ower buds exert cancer preventive e ff ects by enhancing the SCFA-producers , strengthening the epithelial tight junction complex and immune responses. Pharmacol. Res. 159, 104809. doi: 10.1016/j.phrs.2020.104809
Xia, W., Li, X., Khan, I., Yin, L., Su, L., Leong, W. K., et al. (2020b). Lycium berry polysaccharides strengthen gut microenvironment and modulate gut microbiota of the mice. Evidence-Based Complement. Altern. Med. 2020, 1–10. doi: 10.1155/2020/8097021
Xu, J., Lian, F., Zhao, L., Zhao, Y., Chen, X., Zhang, X., et al. (2015). Structural modulation of gut microbiota during alleviation of type 2 diabetes with a Chinese herbal formula. ISME J. 9, 552–562. doi: 10.1038/ismej.2014.177
Xu, Y., Wang, N., Tan, H. Y., Li, S., Zhang, C., Zhang, Z., et al. (2020). Panax notoginseng saponins modulate the gut microbiota to promote thermogenesis and beige adipocyte reconstruction via leptin-mediated AMPKα/STAT3 signaling in diet-induced obesity. Theranostics 10, 11302–11323. doi: 10.7150/thno.47746
Yang, M., Ji, X., Zuo, Z. (2018). Relationships between the toxicities of radix aconiti lateralis preparata (Fuzi) and the toxicokinetics of its main diester-diterpenoid alkaloids. Toxins (Basel) 10, 1–18. doi: 10.3390/toxins10100391
Zhai, S., Qin, S., Li, L., Zhu, L., Zou, Z., Wang, L. (2019). Dietary butyrate suppresses inflammation through modulating gut microbiota in high-fat diet-fed mice. FEMS Microbiol. Lett. 366, fnz153. doi: 10.1093/femsle/fnz153
Zhang, S., Zeng, B., Chen, Y., Yang, M., Kong, F., Wei, L., et al. (2021). Gut microbiota in healthy and unhealthy long-living people. Gene 779, 145510. doi: 10.1016/j.gene.2021.145510
Zhang, X., Zhao, S., Song, X., Jia, J., Zhang, Z., Zhou, H., et al. (2018). Inhibition effect of glycyrrhiza polysaccharide (GCP) on tumor growth through regulation of the gut microbiota composition. J. Pharmacol. Sci. 137, 324–332. doi: 10.1016/j.jphs.2018.03.006
Zhao, Y. F., Song, F. R., Yue, H., Guo, X. H., Li, H. L., Liu, Z. Q., et al. (2007). Biotransformation of deoxyaconitine of metabolite of aconitine by human intestinal bacteria and electrospray ionization tandem mass spectrometry. Gaodeng Xuexiao Huaxue Xuebao/Chemical J. Chin. Univ. 28, 2051–2055.
Zhou, M., Hong, Y., Lin, X., Shen, L., Feng, Y. (2017). Recent pharmaceutical evidence on the compatibility rationality of traditional Chinese medicine. J. Ethnopharmacol. 206, 363–375. doi: 10.1016/j.jep.2017.06.007
Keywords: Chinese medicine, TCM, gut microbiota, prebiotics, TCM-microbiota interaction, TCM-bacteria interaction, medicine
Citation: Xia W, Liu B, Tang S, Yasir M and Khan I (2022) The science behind TCM and Gut microbiota interaction—their combinatorial approach holds promising therapeutic applications. Front. Cell. Infect. Microbiol. 12:875513. doi: 10.3389/fcimb.2022.875513
Received: 15 February 2022; Accepted: 08 August 2022;
Published: 13 September 2022.
Edited by:
Steven Gill, University of Rochester, United StatesReviewed by:
Tongtong Li, Zhejiang University of Technology, ChinaYuqian Feng, Zhejiang Chinese Medical University, China
Copyright © 2022 Xia, Liu, Tang, Yasir and Khan. This is an open-access article distributed under the terms of the Creative Commons Attribution License (CC BY). The use, distribution or reproduction in other forums is permitted, provided the original author(s) and the copyright owner(s) are credited and that the original publication in this journal is cited, in accordance with accepted academic practice. No use, distribution or reproduction is permitted which does not comply with these terms.
*Correspondence: Imran Khan, cnVzdGFta2hhbjMxQHlhaG9vLmNvbQ==; Muhammad Yasir, eWFzaXJwaHJAZ21haWwuY29t
†These authors have contributed equally to this work