- Department of Parasitology, School of Basic Medical Sciences, Zunyi Medical University, Zunyi, China
Cysticercus cellulosae (C. cellulosae) excretes and secretes antigens during the parasitic process to regulate the host immune response; however, resulting immune response and cytokine production in the host during infection still remains unclear. We used C. cellulosae crude antigens (CAs) as controls to explore the effect of excretory secretory antigens (ESAs) on T-cell immune responses in piglets. C. cellulosae ESAs induced imbalanced CD4+/CD8+ T-cell proportions, increased the CD4+Foxp3+ and CD8+Foxp3+ T-cell frequencies, and induced lymphocytes to produce interleukin-10, which was mainly attributed to CD4+ and CD4−CD8− T cells. The ESAs also induced Th2-type immune responses. The results showed that the ability of C. cellulosae to escape the host immune attacks and establish a persistent infection may be related to host immune response regulation by the ESAs.
Introduction
Taenia solium (T. solium) is a cestode parasite that has zoonotic importance and is harmful to human health. Its developmental stages include four life cycles: eggs, oncospheres, larvae, and adults (Arora et al., 2017). Both larval form of this parasite, termed as Cysticercus cellulosae and the adult worms, are responsible for human disease. Adult parasites of T. solium infected the human intestine and caused taeniasis, whereas, C. cellulosae infected the subcutaneous tissues, muscle, brain, eyes, and other sites of intermediate host and caused cysticercosis, and neurocysticercosis (NCC) is the most serious form of the disease (Gonzales et al., 2016). Patients with NCC exhibit varying symptoms; these can be asymptomatic or have mild clinical manifestations (e.g., headache, dizziness, and occasional seizures) to a severe neurological syndrome (e.g., seizures, intracranial hypertension, hydrocephalus, cerebrospinal fluid blockage, cognitive deficit, and other features), which is chronic with a very high mortality rate (Fleury et al., 2016; Gonzales et al., 2016).
Parasitic infections are an important public health problem worldwide (Gazzinelli-Guimaraes and Nutman, 2018). Helminth parasites can regulate and evade host immune attacks through various means (Maizels and McSorley, 2016). Regulatory T cells (Tregs) could produce interleukin (IL)-10 and transforming growth factor (TGF)-β to suppress host immune responses (Arce-Sillas et al., 2016); thus, immune escape by parasites may be attributed to Tregs production. Host immunity determines parasite susceptibility (Tharmalingam et al., 2016), and CD4+ T cells play important roles in host immunoregulation (Zhu et al., 2010). Dendritic cells (DCs) are the most efficient antigen-presenting cells (Clotilde and Amigorena, 2001), which can present foreign antigens to naive CD4+ T cells, which then activate and differentiate into Tregs and helper T cells (Th), including Th1, Th2, and Th17. Th1-type immune responses inhibit infections, and Th2-type immune responses aggravate infections (Tharmalingam et al., 2016).
Both larval and adult form of T. solium excrete and secrete various products into the host; these products make direct contact with the hosts’ immune system and interfere with immunoregulation (Hewitson et al., 2009; White and Artavanis-Tsakonas, 2012; Sun et al., 2019). Among these products, excretory secretory antigens (ESAs) include many antigenic substances, which can be obtained in vitro via cultivation technology (Iddawela et al., 2007; Stefan et al., 2011; Wang et al., 2017). Compared with parasitic crude antigens (CAs) and soluble antigens (SAs), ESAs are involved in parasitic pathogenesis and immune evasion and are considered diagnostic and treatment targets (Hayam et al., 2013; Gazzinelli-Guimaraes and Nutman, 2018). For example, CD4+ and CD8+ T-cell proliferation are decreased and the mice’s immunoreaction to the worms is suppressed when mice are infected with ESAs from adult hookworms, thus increasing the worm’s ability to survive in the host (Nicholas and Blaise, 2017). ESAs from adult nematodes can selectively inhibit interferon (IFN)-γ and IL-2 production, but not IL-4 or IL-10 production, in CD4+ and CD8+ T cells of rat mesenteric lymph nodes. It shows that Th2 immune responses are closely related to persistent parasitic infections (Uchikawa et al., 2000).
C. cellulosae ESAs from T. solium induced angiogenesis in the brains of rat models of NCC, which may be related to the NCC pathology (Carmen-Orozco et al., 2019). Studies have shown that the sensitivity and specificity of enzyme-linked immunoelectrotransfer blots (EITB) for detecting anti-ESA antibodies in the serum of patients with NCC were higher than the lower molecular mass (LMM) antigenic fractions of C. cellulosae. Moreover, detecting anti-ESA antibodies via enzyme-linked immunosorbent assay (ELISA) enables evaluating the treatment responses of these patients. C. cellulosae survival is directly related to the existence of anti-ESA antibodies, and these antibodies can be used in new diagnostic tests and subsequent treatments (Molinari et al., 2002;Atluri et al., 2010; Atluri et al., 2014; Paredes et al., 2016). Thus, we conducted this study to explore the effects of C. cellulosae ESAs from T. solium on the immunoresponses of T lymphocytes.
Materials and Methods
Animals and Infection
A whole adult T. solium worm was obtained from a patient with taeniasis from a taeniasis-endemic area in Yajiang, Ganzi, Sichuan Province. To generate T. solium larvae, each healthy piglet was infected by feeding 5 pieces mature gravid proglottids from the worm at the Animal Experimental Center of Zunyi Medical University. There were 12 piglets in this infection experiment, including 10 piglets in the infection group and 2 piglets in the control group. The healthy piglets were confirmed to be pathogen-free before infection and raised under standard conditions. The Animal Care and Use Committee of Zunyi Medical University approved the experiments.
Preparation of C. cellulosae ESAs and CAs
To prepare the ESAs and CAs of the T. solium larvae, the infected piglets were euthanized 2–3 months postinfection, and C. cellulosae were harvested from the muscle tissue. The C. cellulosae were washed three times in physiological saline and sterile phosphate-buffered saline (PBS: 0.02 M Na2HPO4/NaH2PO4, 0.15 M NaCl, pH 7.2), then cultivated at 37°C and 5% CO2 in RPMI 1640 medium (cat. #31800, Solarbio, Beijing, China) containing penicillin G (100 U/ml) and streptomycin (100 μg/ml). Then the culture supernatants were collected aseptically after 72 h. The supernatants were filtered through 0.22 μm sterile filters, then concentrated by transferring them into a 3 kDa ultrafiltration tube (cat. #UFC900396, Millipore, MA, USA). The liquid after concentration was C. cellulosae ESAs, and its protein concentration was determined using a Bradford protein assay kit (cat. #UFC0010, Solarbio, Beijing, China) per the manufacturer’s instructions and stored at −80°C until used.
The C. cellulosae CAs were prepared as previously described with some modifications (Verastegui et al., 2001). The C. cellulosae were collected and washed three times with physiological saline and PBS, then homogenized in a homogenizer (70 Hz/s, 10 s/time) at 4°C until completely lysed. The supernatants were collected via centrifugation as C. cellulosae CAs, and its protein concentration was measured using a Bradford protein assay kit per the manufacturer’s instructions and stored at −80°C until further use.
Peripheral Blood Mononuclear Cell Isolation
Peripheral blood mononuclear cells (PBMCs) were isolated as previously described with minor modifications (Valent et al., 2017). Briefly, under sterile conditions, 10 ml of heparinized anticoagulated blood was collected from the healthy piglets via jugular venipuncture and diluted 1:1 with sample diluent according to the lymphocyte separation solution kit (cat. #LTS1110, TBD Sciences, Tianjin, China) as per manufacturer’s instructions. The diluted blood was added to the surface of an equal volume of separation liquid. The solutions were centrifuged at 2,500 rpm for 20 min according to the density gradient centrifugation to obtain the PBMCs layer. PBMCs were collected and washed twice with cleaning solution. Erythrocyte lysis buffer (cat. #R1010, Solarbio, Beijing, China) was used to eliminate erythrocyte contamination. PBMCs were suspended in RPMI 1640 medium with 10% inactivated fetal calf serum (cat. #11011-8611, Sijiqing, Zhejiang, China) after ensuring that the cell viability exceeded 95% via trypan blue staining.
Cell Cultures
To analyze the effects of ESAs and CAs on mitogen-induced lymphocyte proliferation, PBMCs were cultured in 96-well plates at 1×106 cells/well at 37°C and 5% CO2 and separately incubated with control medium, concanavalin A (ConA, 10 μg/ml) (cat. #C5275, Sigma, Saint Louis, MO, USA), ESAs (40 μg/ml), or CAs (40 μg/ml). Phytohemagglutinin (cat. #P8090, Solarbio, Beijing, China) was added to a final concentration of 2.5 μg/ml after cultivation for 3 h. Cells were harvested on day 2, stained with CD4 and CD8, and analyzed via flow cytometry.
To further analyze whether ESAs and CAs can induce Treg production, PBMCs were incubated in 96-well plates at 1×106 cells/well and treated with control medium, ConA, ESAs, or CAs for 48 h under standard conditions. The cells were then collected and analyzed for Foxp3, CD4, and CD8 expression by flow cytometry. Foxp3 and Helios mRNA expression levels were calculated via quantitative real-time PCR (qRT-PCR).
To investigate whether ESAs and CAs can induce IL-10 production, PBMCs were cultured in 96-well plates at 1×106/well and added with ESAs, CAs, 2 μg/ml lipopolysaccharide (LPS: cat. #L2880, Solarbio, Beijing, China), ESA+LPS, CA+LPS, or control medium. The culture supernatants were harvested after 24 h, and IL-10 expression was measured via ELISA.
The lymphocyte subsets expressing IL-10 were evaluated via intracellular cytokine staining. PBMCs were cultured in 96-well plates at 1×106/well and stimulated with ESAs, CAs, LPS, ESA+LPS, CA+LPS, or control medium. During the last 12 h of culturing, 5 μg/ml of Brefeldin A (cat. #abs810012, Absin, Shanghai, China) was added to block intracellular cytokine secretion into the extracellular area. After 24 h, the cells were stained with IL-10, CD4, CD8, IL-10, and Foxp3 and analyzed via flow cytometry.
T-Lymphocyte Polarization
Healthy piglets were anesthetized, 20 ml bone marrow was extracted, and the bone marrow precursor cells were isolated under sterile conditions. The cells were cultured under standard conditions, and the immature DCs were induced. The purity of immature DCs was more than 80% by flow cytometry. To determine the influence of C. cellulosae ESAs and CAs on T-cell polarization, CD4+ T cells were enriched from the spleens of the healthy piglets via positive selection using anti-CD4 magnetic beads (cat. #130-091-652, Miltenyi Biotec, Bergisch Gladbach, Germany) and a lymphocyte separation solution kit (cat. #LTS1110, TBD Sciences, Tianjin, China) as per manufacturer’s instructions. CD4+ T cells at 1×107 were co-cultivated with 1×106 immature DCs in 96-well pates and added with ESAs (40 μg/ml), CAs (40 μg/ml), ConA (10 μg/ml), or control medium stimulation. The medium was removed via centrifugation, and 10 ng/ml pig recombinant IL-2 (cat. #ab238302, ABCAM, Cambridge, UK) was added to promote polarization and maintain T-lymphocyte activity when 24 h of culture. After 48 h, the culture supernatants were collected, and the expression levels of IFN-γ (Th1), IL-4 (Th2), and IL-17 (Th17) were analyzed via ELISA.
Flow Cytometry and Antibodies
For cell surface staining, cells were washed twice in precooling staining buffer, then resuspended in staining buffer and incubated with primary antibodies for 30 min on ice in the dark. Cells were then washed once with staining buffer and resuspended in it. The antibodies were used as CD4a:PE-Cy7 (clone #561473, BD Pharmingen, Franklin Lakes, NJ, USA) and CD8a:AF647 (clone #561475, BD Pharmingen, Franklin Lakes, NJ, USA). To detect the intracellular antigens, cells were first incubated for 30 min with surface-labeled antibodies, then washed and resuspended in staining buffer. Cells were then fixed and permeabilized for 45 min with transcription factor buffer (cat. #562574, BD Pharmingen, Franklin Lakes, NJ, USA) as per manufacturer’s instructions, then incubated with intracellular antigens fluorescent antibodies for 45 min on ice in the dark. The antibodies were used as CD4a:PE-Cy7, CD8a:AF647, Foxp3:FITC (clone #11577382, Thermo Fisher, Waltham, MA, USA), and IL-10:PE (clone #12710841, Thermo Fisher, Waltham, MA, USA).
ELISA for Cytokine Secretion
Cytokine secretion was detected using pig IFN-γ, IL-4, and IL-17 ELISA kits (cat. #SEA056Po, Cloud-Clone Corp., Wuhan, China). The culture supernatants were collected, and then the cytokine production levels were analyzed as per manufacturer’s instructions.
qRT-PCR
The relative changes of Foxp3 and Helios mRNA expression levels in the PBMCs were measured via qRT-PCR. The primer sequences used were pig GAPDH (F: 5′-TCGGAGTGAACGGATTTGGC-3′, R: 5′-TGACAAGCTTCCCGTTCTCC-3′), pig Foxp3 (F: 5′-GGTGCAGTCTCTGGAACCAAC-3′, R: 5′-GGTGCCAGTGGCTACAATAC-3′), and pig Helios (F: 5′-AGGAGGTACATGGTGACTCA-3′, R: 5′-CTCACAGGACACCTCAGGAC-3′). TRIzol universal total RNA extraction reagent (cat. #D424, Tiangen) was used to extract total RNA from the stimulated cells per the manufacturer’s instructions. Reverse transcription and qRT-PCR were performed using RevertAid First Strand cDNA synthesis kit (cat. #K1622, Thermo Fisher) and FastStart Universal SYBR Green Master (Rox) (cat. #04 913 914 001, Roche, Basel, Switzerland). The cycle conditions were one cycle at 95°C for 15 s, 56°C for 30 s, and 72°C for 20 s for 44 cycles. The relative Foxp3 and Helios mRNA expression levels were determined via the 2−ΔΔCT method.
Statistical Analysis
One-way analysis of variance was used for multiple-group comparisons, and the least significant differences test was used for pairwise comparisons between groups. P<0.05 was considered the lowest statistically significant; P<0.01 was considered moderately statistically significant; P<0.001 was considered the highest statistically significant.
Results
C. cellulosae ESAs and CAs Induced CD4+ and CD8+ T-Lymphocyte Responses
Piglet PBMCs were cultured with ESAs and CAs for 48 h, then the CD4+ and CD8+ T-cell subset expressions were detected via flow cytometry. The ESAs-induced CD8+ T cells increased to levels near those of ConA. Compared with the normal controls, the CAs-induced CD8+ T cells were elevated, but CD4+ T cells were decreased, and both levels were lower than those of ConA (Figure 1). Compared with CAs, ESAs induced a significant increase in CD4+ and CD8+ T cells (Figures 1A, B). Thus, both C. cellulosae ESAs and CAs can stimulate an immune imbalance in the T-lymphocyte subsets.
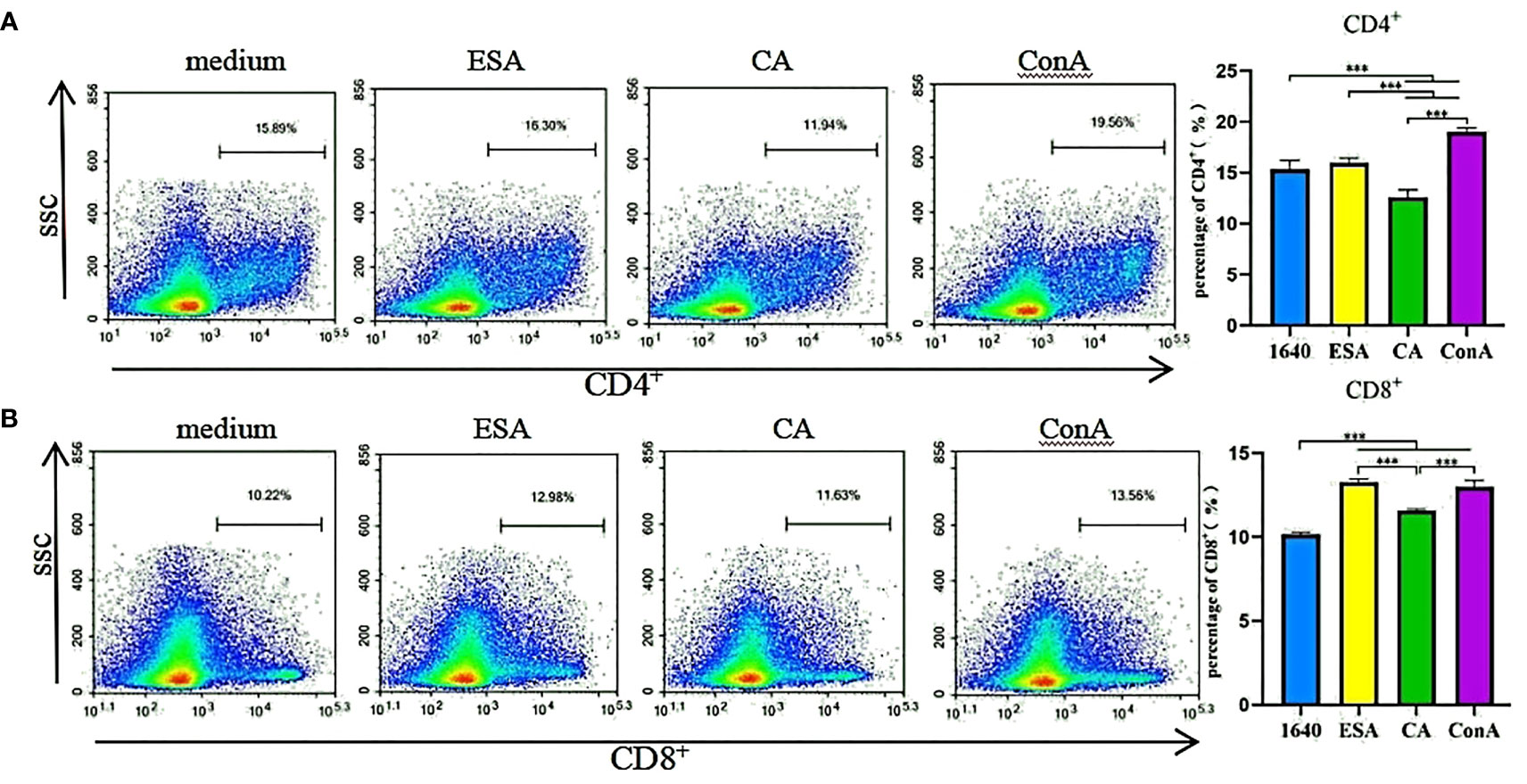
Figure 1 T cells immune response assay. The frequencies of CD4+ and CD8+ T lymphocytes were detected by flow cytometry. (A) Cysticercus cellulosae CA inhibited the expression of CD4+ T lymphocytes but ESA had no significant effect on them. Cysticercus cellulosae ESA significantly induced the expression of CD4+ T lymphocytes compared with CA. (B) Both Cysticercus cellulosae ESA and CA induced the production of CD8+ T lymphocytes, and compared with CA, ESA significantly stimulated the expression of CD8+ T lymphocytes. All dates were represented by means ± SD, ***P < 0.001.
C. cellulosae ESAs and CAs Stimulated Tregs Subsets Expression
The effects of C. cellulosae ESAs and CAs on Treg production were checked. We analyzed the expressions of Treg subsets via flow cytometry. C. cellulosae ESAs increased Foxp3+ lymphocyte expression and had a statistical significance. The CAs also slightly increased the Foxp3+ lymphocytes, but the increase was not statistically significant compared with the controls (Figure 2A). Tricolor flow cytometry showed that C. cellulosae ESAs stimulated CD4+ and CD8+ lymphocytes, which are the main source of Foxp3 expression. CAs stimulated only the production of CD4+Foxp3+ Tregs (Figures 2B, C). Neither the ESAs nor the CAs of C. cellulosae significantly affected the expressions of CD4+CD8+Foxp3+ or CD4−CD8−Foxp3+ Tregs (Figures 2D, E).
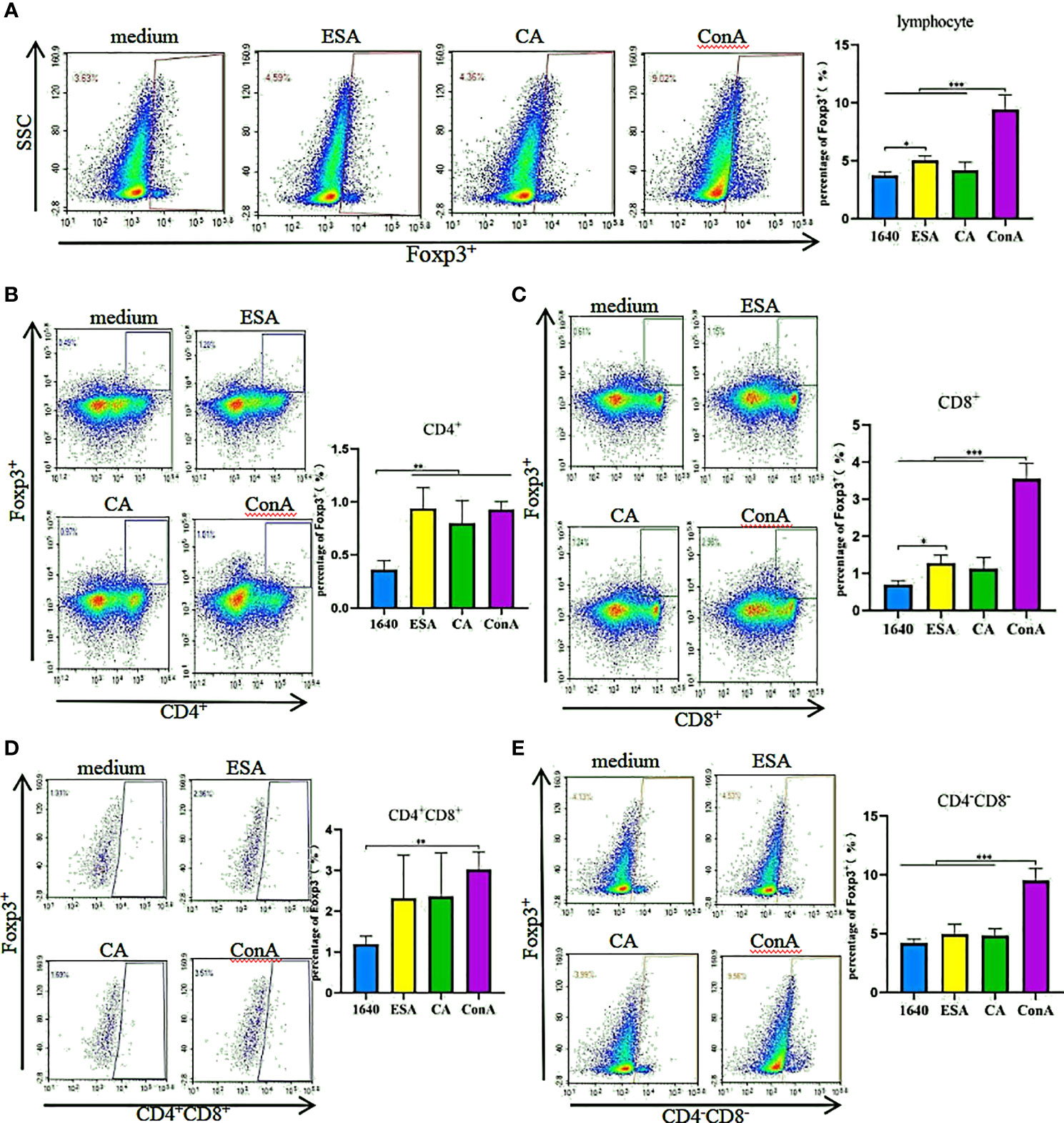
Figure 2 Tregs inducing assay. PBMCs were stimulated for 48 hours with Cysticercus cellulosae ESA and CA respectively, then the cells were collected and measured the expression of Tregs subsets via flow cytometry. (A) Cysticercus cellulosae ESA significantly induced the expression of Foxp3+ lymphocytes, while, CA had no obvious impacts on the expression. (B) Both Cysticercus cellulosae ESA and CA could promote the frequency of CD4+Foxp3+Tregs. (C) The frequency of CD8+Foxp3+ Tregs was increased only by Cysticercus cellilosae ESA. (D, E) Both Cysticercus cellulosae ESA and CA had no effects on the expression of CD4+CD8+Foxp3+ and CD4-CD8-Foxp3+Tregs. Dates were expressed as the means ± SD, *P < 0.05, **P < 0.001, ***P < 0.001.
C. cellulosae ESAs and CAs Decreased the Relative Foxp3 and Helios mRNA Expression Levels
Because Foxp3+ Tregs also express Helios, we preliminarily detected the mRNA expression levels of Foxp3 and Helios in PBMCs stimulated with C. cellulosae ESAs and CAs via qRT-PCR. Compared with those of the normal controls, C. cellulosae ESAs and CAs significantly reduced the Foxp3 and Helios mRNA expression levels in the PBMCs (Figures 3A, B).
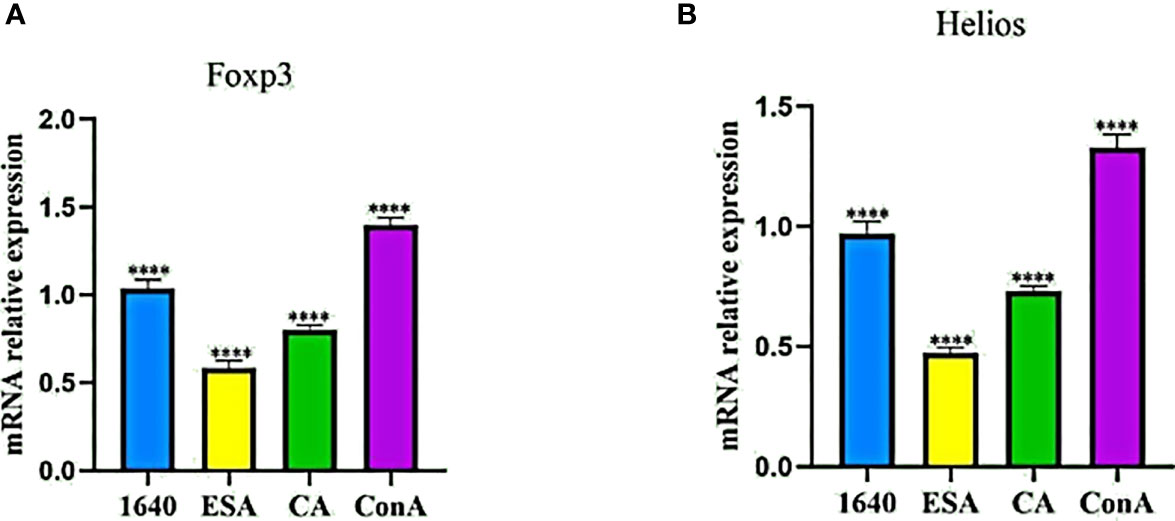
Figure 3 qRT-PCR revealed the mRNA expression levels of Foxp3 and Helios in PBMCs. (A) The Foxp3 mRNA expression quantity was inhibited by Cysticercus cellulosae ESA and CA compered with 1640 group. (B) Similiar to the change of Foxp3, ESA and CA also reduced the mRNA expression level of Helios inPBMCs. Results were expressed as the mean ± SD, ****P < 0.0001.
IL-10 Secretion in PBMCs
PBMCs were stimulated with ESAs and CAs for 24 h, then the culture supernatants were collected, and the IL-10 secretion levels were detected via ELISA. In the absence of LPS, both C. cellulosae ESAs and CAs increased the IL-10 secretion in the PBMCs compared with that of the controls. Additionally, C. cellulosae ESAs induced lower IL-10 secretion levels than did the CAs. However, stimulating the ESAs and CAs with LPS significantly reduced the IL-10 secretion levels. Compared with CAs+LPS, ESAs+LPS decreased the IL-10 secretion (Figure 4).
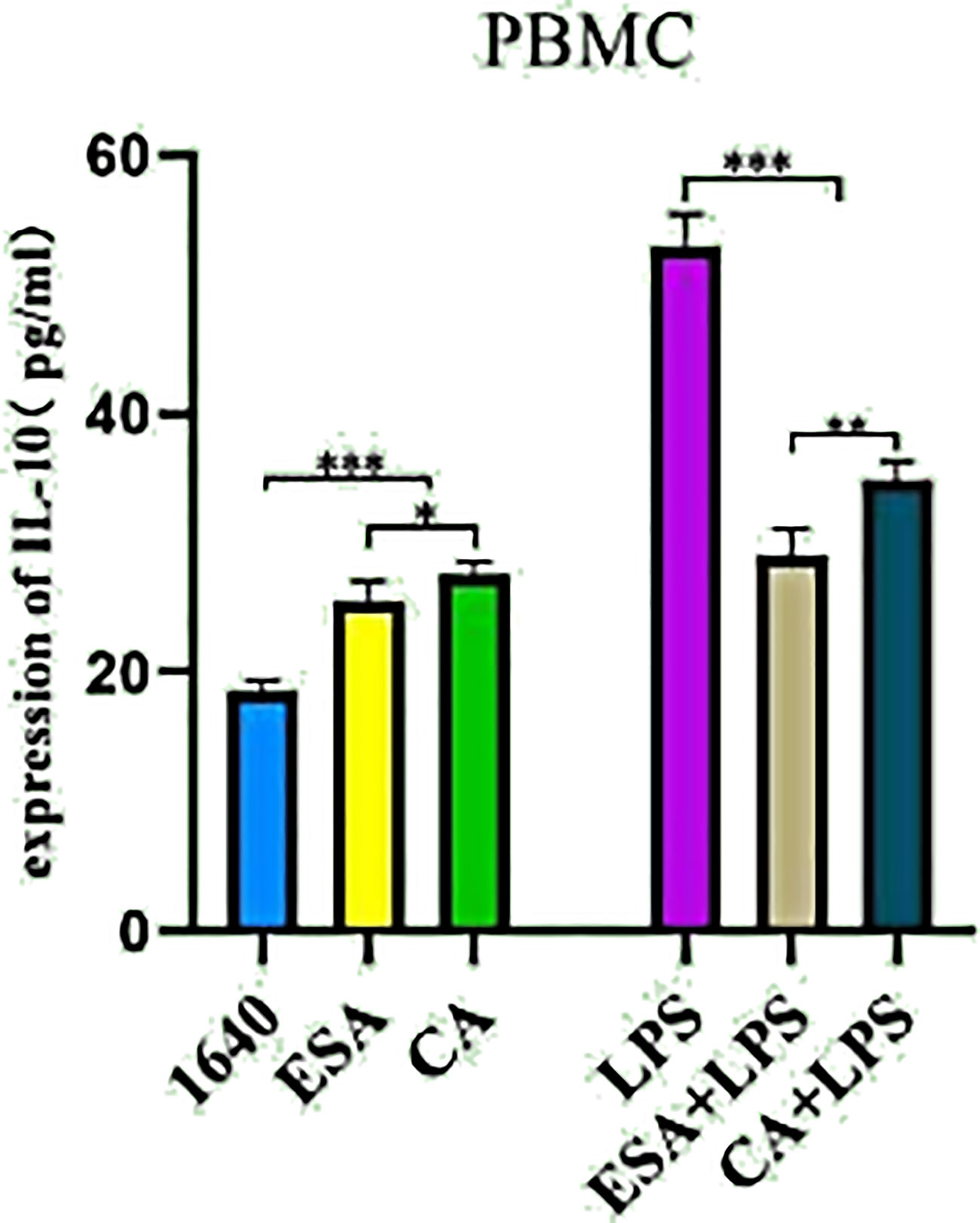
Figure 4 IL-10 secretion. The secretion level of IL-10 in cultured supernatant was measured by ELISA. Cysticercus cellulosae ESA and CA treated PBMCs for 24 hours without LPS, ELISA showed both ESA and CA could be capable to induce IL-10 secretion. But, under the presence of LPS, the secretion of IL-10 was inhibited by ESA and CA in PBMCs. Dates were represented by means ± SD, *P < 0.05, **P < 0.01, ***P < 0.001.
C. cellulosae ESAs and CAs Induced IL-10 Expression in Lymphocytes
To further investigate IL-10 expression in lymphocytes, we used tricolor flow cytometry to detect the IL-10+, CD4+IL-10+, CD8+IL-10+, and CD4+/−CD8+/−IL-10+ lymphocyte frequencies. Without LPS, both C. cellulosae ESAs and CAs significantly increased the IL-10+ lymphocyte frequencies. Additionally, the induction effects of the ESAs and CAs on IL-10+ lymphocytes were stronger in the presence LPS (Figure 5A). CD4+ T lymphocytes were the main sources of C. cellulosae ESA- and CA-induced IL-10 secretion (Figure 5B). ESAs also induced CD4−CD8−IL-10+ lymphocyte expression (Figure 5D). Conversely, IL-10 expression was significantly inhibited in the CD8+ and CD4+CD8+ T lymphocytes (Figures 5C–E). When cells were co-cultured with C. cellulosae ESA+LPS and CA+LPS, the CD4+IL-10+ lymphocyte frequencies increased markedly, but the CD8+IL-10+ lymphocyte expression decreased significantly (Figures 5B, C). C. cellulosae ESAs stimulated with LPS significantly inhibited the frequency of CD4+CD8+IL-10+ cells but increased the expression frequency of CD4−CD8−IL-10+ cells. However, C. cellulosae CAs stimulated with LPS decreased the CD4−CD8−IL-10+ cells production (Figures 5D, E).
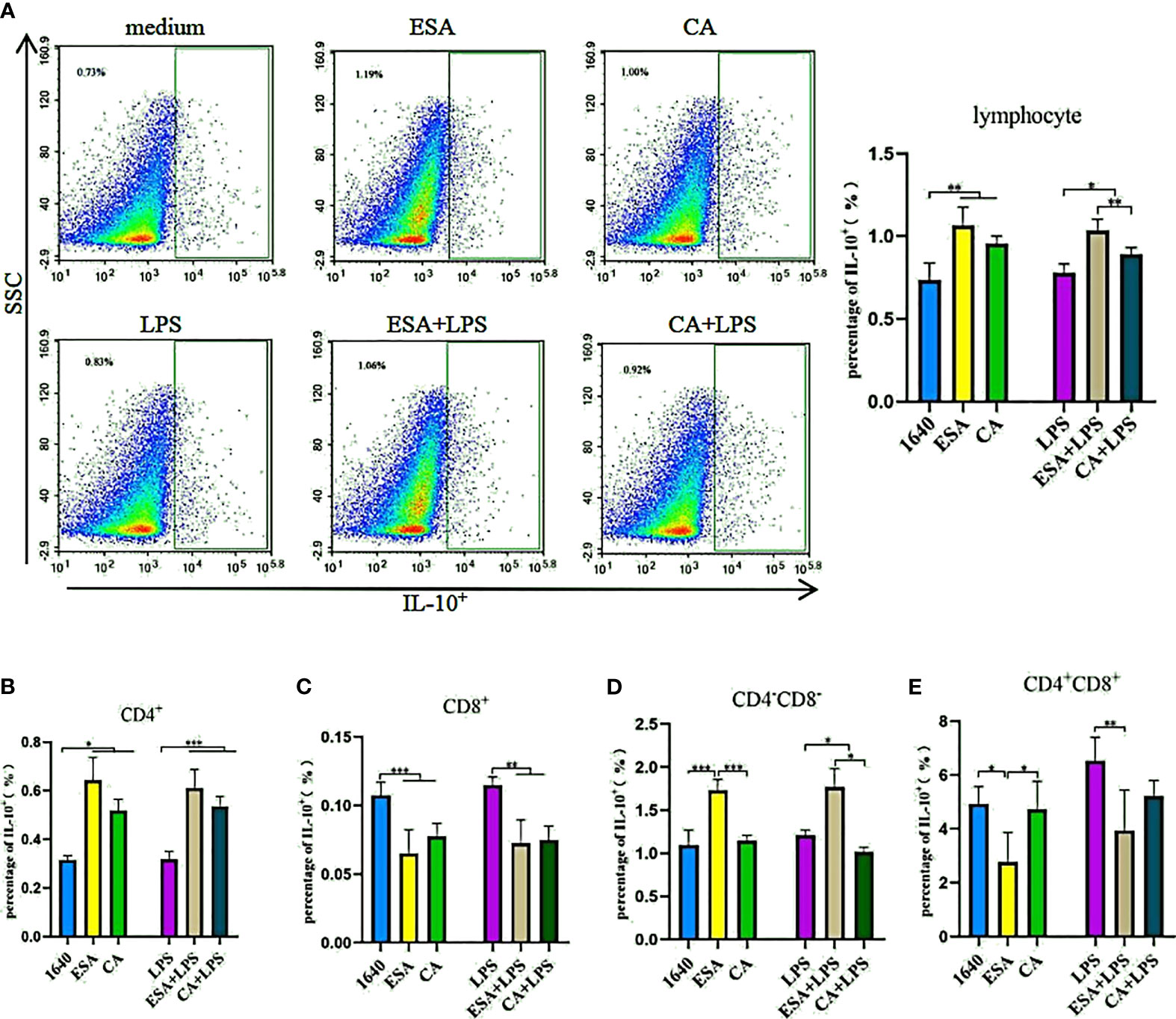
Figure 5 IL-10+ lymphocyte assay. The production of IL-10+ lymphocyte subsets by flow cytometry. (A) Both Cysticercus cellulosae ESA and CA induced the production of IL-10+ lymphocyte. (B) CD4+T lymphocyte was the main source Cysticercus cellulosae ESA and CA-induced IL-10 expression. (C) In the presence or absence of LPS, Cysticercus cellulosae ESA and CA significantly inhibited the expression frequency of CD8+IL-10+ lymphocyte. (D) Cysticercus cellulosae ESA could be induced the expression of CD4-CD8-IL-10+ cell under the presence or absence of LPS. While, CA only decreased the expression in the presence of LPS. (E) Similar to (C), the production of IL-10 in CD4+CD8+ lymphocyte was inhibited by Cysticercus cellulosae ESA. All dates were shown as the means ± SD, *P < 0.05, **P < 0.01, ***P < 0.001.
Co-staining IL-10 and Foxp3 without LPS revealed that C. cellulosae ESAs inhibited the Foxp3+IL-10+ lymphocyte production, but no significant differences were noted between the CAs and the controls. Interestingly, only C. cellulosae CAs reduced the Foxp3+IL-10+ lymphocytes expression when combined with LPS (Figure 6).
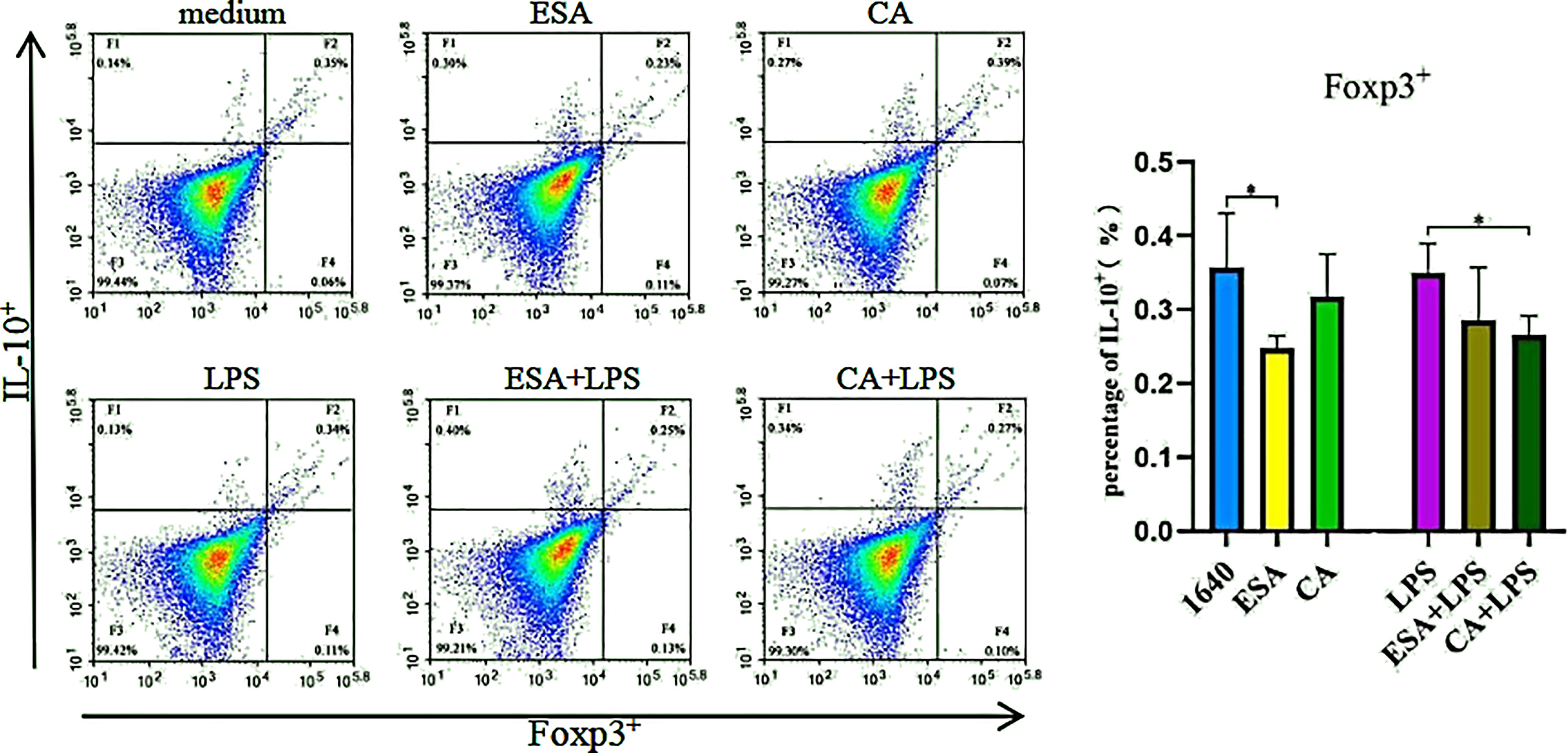
Figure 6 The production of Foxp3+IL-10+lymphocyte. The co-expressing Foxp3 and IL-10 cell were detected via tricolor flow cytometry, which shown that the production of Foxp3+IL-10+ lymphocyte was inhibited by Cysticercus cellulosae ESA. Besides, CA had significantly decreased the production only under the presence of LPS. Results were expressed as the mean± SD, *P < 0.05.
Th1, Th2, and Th17 Cell Differentiation
To investigate the modulatory effects of C. cellulosae ESAs and CAs on Th subset production, IFN-γ, IL-4, and IL-17 secretion levels were examined via ELISA. DC-CD4+ T cells exposed to C. cellulosae ESAs and CAs produced different cytokine levels (Figures 7A–C). Stimulation with C. cellulosae CAs significantly induced IL-4, IL-17, and IFN-γ secretion, and the IL-4 and IL-17 secretion levels were higher than those of the positive controls. However, C. cellulosae ESAs strongly promoted IL-4 and IL-17 secretion, but it was lower than that of the CAs. Importantly, both ESAs and CAs dramatically induced IL-4 production (Figure 7D). Therefore, C. cellulosae ESAs and CAs stimulation played strong regulatory roles in Th cell immune responses by altering the cytokine secretion.
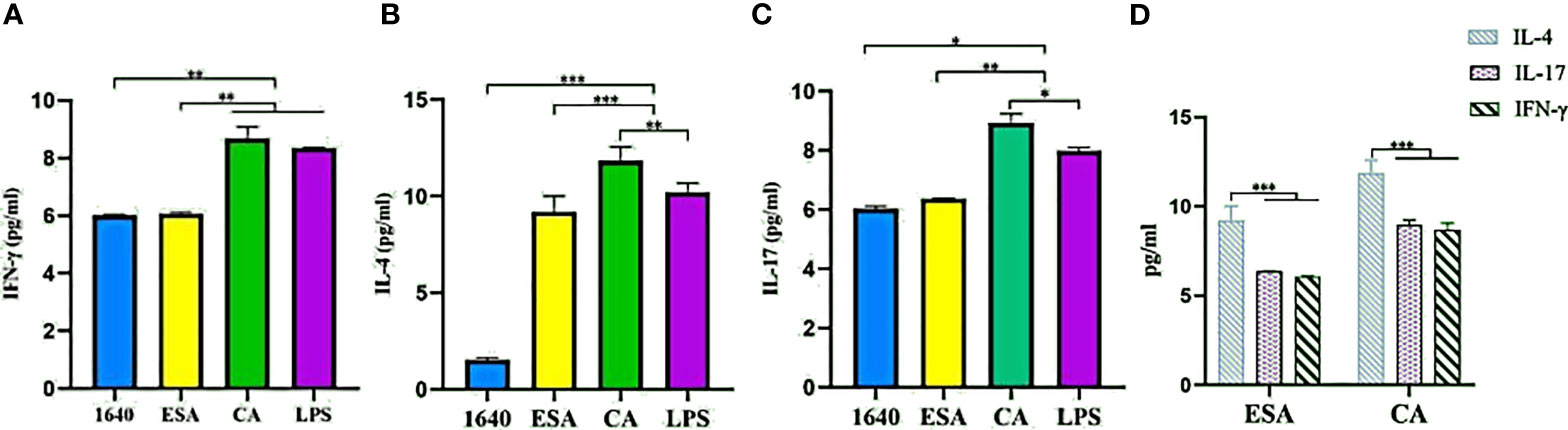
Figure 7 Th cytokines secretion. The secretion levels of IFN-γ, IL-4 and IL-17 were measured by ELISA. (A) Only Cysticercus cellulosae CA induced IFN-γ secretion, ESA had no effect on it. (B) Both Cysticercus cellulosea ESA and CA significantly induced the production of IL-4 and the latter was higher than the former. (C) Cysticercus cellulosae CA obviously up-regulated the secretion level of IL-17 compared with ESA. (D) Both Cysticercus cellulosae ESA and CA dramatically induced IL-4 secretion. All dates were represented by means ± SD, *P < 0.05, **P < 0.01, ***P < 0.001.
Discussion
Cysticercosis is a complex disease involving host-parasite interactions. The extent and nature of the host immune response, as well as the active immunomodulatory mechanisms produced by the parasite, determine the disease occurrence and development (Garcia et al., 2014). Under normal conditions, T-cell subsets regulate each other and maintain a balanced immune function. In this study, C. cellulosae ESAs and CAs increased the number of CD8+ T cells without significantly changing or decreasing the number of CD4+ T cells, thus decreasing the CD4+/CD8+ T-cell ratios. Thus, the immune imbalance induced by cysticercosis may be related to regulation of the T-cell immune responses by C. cellulosae ESAs and CAs, indicating that these two have potential regulatory roles in lymphocytes homeostasis.
Similar to human Tregs, CD4+Foxp3+ T cells are markers of porcine Tregs, and porcine CD8+Foxp3+ Tregs have also been identified (Wang et al., 2016). Tregs can exert suppressive functions by prompting secretion of the immunosuppressive cytokines, IL-10 and TGF-β, as well as play important roles in immunotolerance and immune homeostasis (Georgiev et al., 2019). The transcription factor, Foxp3, a dominant regulator and highly specific marker of Tregs, is the prerequisite for Treg cells to produce and exert their immunosuppressive effects (Deng et al., 2019). Foxp3-defective Tregs have decreased IL-10 and TGF-β production levels and weaker suppression abilities (Jia et al., 2015). During parasitic infections, Tregs are increased and exert immunosuppressive functions, enabling the parasites to escape immune attack and survive for long periods in the host. Here, C. cellulosae ESAs induced Foxp3 expression in piglet lymphocytes, but the CAs showed no significant differences compared with those of the controls. These results are similar to those of a previous study on Toxocara canis ESAs in canine Foxp3+ Tregs (Junginger et al., 2017). The results showed that C. cellulosae ESAs may induce Treg production and exert immunosuppressive effects in natural hosts. Further analysis of the lymphocyte subsets expressing Foxp3 showed that ESAs and CAs promoted Foxp3 expression in CD4+T lymphocytes, which are typical immunosuppressive cells. Additionally, C. cellulosae ESAs also induced production of CD8+Foxp3+ Tregs, which inhibited T-cell activation and proliferation and induced de novo generation of CD4+Foxp3+ Tregs (Erika et al., 2012). These results were similar to those of previous studies in that induction of Tregs by parasitic ESAs was more significant than that of CAs (Wang et al., 2015; Grainger et al., 2020). The differences in Treg subsets induced by ESAs and CAs may be related to the different components of the two antigens. In summary, ESAs and CAs could induce Treg expression, which may be one mechanism by which C. cellulosae evades the host immune attacks.
Foxp3+ Tregs can be divided into Helios+ and Helios− Tregs in mice. In human Tregs, Helios knockout downregulated Foxp3 expression, thereby weakening Treg inhibition (Eyad et al., 2015). qRT-PCR was used to detect relative mRNA expression levels of Foxp3 and Helios in ESA- and CA-stimulated cells to preliminarily evaluate the change trend of Foxp3 and Helios in these cells. The relative expression trend of the Helios mRNA coincided well with the Foxp3 mRNA in PBMCs stimulated with T. solium larva ESAs and CAs and were significantly lower than those of the controls. One study found reduced Foxp3 mRNA levels in splenocytes and placental cells in mice infected with Toxoplasma gondii (Ge et al., 2010). Helios was originally thought to be expressed only in Tregs, but studies have shown that it is also expressed in CD8+ cells, NK cells, B cells, and other human and mouse cells, but its function in these cells is unclear (Eyad et al., 2015; Thornton and Shevach, 2019). In summary, whether porcine Foxp3 and Helios are expressed in immune cells other than CD4+ and CD8+ T cells and whether play immunosuppressive roles in these immune cells require further study.
IL-10 is an inhibitory anti-inflammatory factor that inhibits T-cell proliferation by inhibiting the production of proinflammatory factors and downregulating the expression of MHC-II and costimulatory molecules on the surface of antigen-presenting cells and directly acts on T cells to induce an unresponsive state (Kumar et al., 2019; Ouyang and O’Garra, 2019; Saraiva et al., 2019). Clinical malaria models and human studies have shown that IL-10 could inhibit antiparasitic immunity (Kumar et al., 2019). In our study, C. cellulosae ESAs and CAs stimulated IL-10 secretion in PBMCs, which is similar to the findings of previous (Javaid et al., 2016). In summary, increased IL-10 secretion may inhibit the host’s immune responses against cysticercosis, leading to a persistent infection. One study showed that LPS induced IL-10 synthesis and release (Chanteux et al., 2007). In addition to Treg cells, IL-10 is also derived from Th2, CD4, and CD8 T lymphocytes (Weiss et al., 2011). Additionally, C. cellulosae ESAs and CAs significantly inhibited IL-10 secretion in the presence of LPS. This result may be related to the interregulation of IL-10 among different cells.
Three-color flow cytometry showed that CD4+ T lymphocytes were mainly responsible for ESA- and CA-induced IL-10 expression. Their production can inhibit nitrous oxide pathway-mediated insecticidal immunity and protect parasites from host immune attacks (Zhou et al., 2014). Additionally, ESAs can also induce production of CD4−CD8−IL-10+ T lymphocytes, which can express IL-10 in vitro and inhibit CD4+ T-cell proliferation (Passos et al., 2017). Thus, CD4+IL-10+ and CD4−CD8−L-10+ T cells play essential roles in immunoregulation of parasitic infections, which may be one mechanism of immune evasion by C. cellulosae. ESAs and CAs can also regulate the production of IL-10+ lymphocytes via LPS-related pathways, but the specific molecular mechanism remains unclear. Although ESAs and CAs can induce the IL-10+, CD4+IL-10+ and CD4−CD8−IL-10+T lymphocyte production, it is unknown whether these cells represent Tregs. Further found ESAs inhibited Foxp3+IL-10+ cell production, but CAs did not. In summary, IL-10 secretion in lymphocytes induced by C. cellulosae ESAs and CAs may be unrelated to Foxp3 expression; the two were likely independent of each other and exerted inhibitory effects.
Th cells specialize in producing effector cytokines and play significant roles in adaptive immune responses. Activated CD4+ T cells can obtain different effector phenotypes, such as those of Th1, Th2, and Th17 (Bianchi et al., 2000). IFN-γ produced by Th1 and IL-4 produced by Th2 can amplify themselves but antagonize each other (Wan and Flavell, 2009). Once infected, the pathogens will actively regulate the immune balance of Th1/Th2 cells and escape from host immune attacks. Both C. cellulosae CA and ESA stimulation led to imbalanced Th immune responses and dominated by Th2-type immunity, which could be interpreted as the manifestation of cytokine cross-regulation. Th2-type immune responses are related to parasite susceptibility (Mendlovic et al., 2015), which may be one reason for the persistent development of cysticercosis. C. cellulosae CAs induced Th2-type immune responses more significantly than did the ESAs, which may be related to the different abilities of DCs antigen presentation induced by the two antigens and the change in Th-type responses with the infection course. The specific immunoregulatory mechanism requires further study (Xu et al., 2016; Musumeci et al., 2019).
Conclusion
In summary, both C. cellulosae ESAs and CAs induced T-cell immune imbalances, which in turn induced immunosuppressive Treg cells, IL-10, and Th2-type immune response production. These may be related to the ability of C. cellulosae to evade the host immune attacks. Our research provides an experimental basis for developing anticysticercosis treatments and disease control strategies, but the cellular and molecular mechanisms involved in regulation of the host immune responses by C. cellulosae require further study.
Data Availability Statement
The raw data supporting the conclusions of this article will be made available by the authors, without undue reservation.
Ethics Statement
The animal study was reviewed and approved by the Ethics Committee of Zunyi Medical University. Written informed consent was obtained from the owners for the participation of their animals in this study.
Author Contributions
XF, YZ, RO, BL, and FY conducted the experiments. XF, YZ, and BZ designed the experiments. XF analyzed the data. LL, WH, ML, NJ, LW, and BZ assisted with the experiments. XF wrote the manuscript. BZ revised the manuscript. All authors contributed to the article and approved the submitted version.
Funding
This work was funded by the National Natural Science Foundation of China (81960378).
Conflict of Interest
The authors declare that the research was conducted in the absence of any commercial or financial relationships that could be construed as a potential conflict of interest.
Publisher’s Note
All claims expressed in this article are solely those of the authors and do not necessarily represent those of their affiliated organizations, or those of the publisher, the editors and the reviewers. Any product that may be evaluated in this article, or claim that may be made by its manufacturer, is not guaranteed or endorsed by the publisher.
Supplementary Material
The Supplementary Material for this article can be found online at: https://www.frontiersin.org/articles/10.3389/fcimb.2021.728222/full#supplementary-material
References
Arce-Sillas, A., Álvarez-Luquín, D. D., Cárdenas, G., Casanova-Hernández, D., Fragoso, G., Hernández, M., et al. (2016). Interleukin 10 and Dendritic Cells are the Main Suppression Mediators of Regulatory T Cells in Human Neurocysticercosis. Clin. Exp. Immunol. 183, 271–279. doi: 10.1111/cei.12709
Arora, N., Tripathi, S., Kumar, P., Mondal, P., Mishra, A., Prasad, A. (2017). Recent Advancements and New Perspectives in Animal Models for Neurocysticercosis Immunopathogenesis. Parasite Immunol. 39, e12439. doi: 10.1111/pim.12439
Atluri, V. S. R., Gogulamudi, V. R., Singhi, P., Khandelwal, N., Parasa, L. S., Malla, N. (2014). Pediatric Neurocysticercosis: Usefulness of Antibody Response in Cysticidal Treatment Follow-Up. Biomed. Res. Int. 2014, 904046. doi: 10.1155/2014/904046
Atluri, S. R. V., Singhi, P., Khandelwal, N., Malla, N. (2010). Evaluation of Excretory Secretory and 10-30 kDa Antigens of Taenia Solium Cysticerci by EITB Assay for the Diagnosis of Neurocysticercosis. Parasite Immunol. 31, 151–155. doi: 10.1111/j.1365-3024.2008.01085.x
Bianchi, G., D’Amico, G., Varone, L., Sozzani, S., Mantovani, A., Allavena, P. (2000). In Vitro Studies on the Trafficking of Dendritic Cells Through Endothelial Cells and Extra-Cellular Matrix. Dev. Immunol. 7, 143–153. doi: 10.1155/2000/39893
Carmen-Orozco, R. P., Dávila-Villacorta, D. G., Cauna, Y., Bernal-Teran, E. G., Bitterfeld, L., Sutherland, G. L., et al. (2019). Blood-Brain Barrier Disruption and Angiogenesis in a Rat Model for Neurocysticercosis. J. Neurosci. Res. 97, 137–148. doi: 10.1002/jnr.24335
Chanteux, H., Guisset, A. C., Pilette, C., Sibille, Y. (2007). LPS Induces IL-10 Production by Human Alveolar Macrophages via MAPKinases- and Sp1-Dependent Mechanisms. Respir. Res. 8, 71–80. doi: 10.1186/1465-9921-8-71
Clotilde, T., Amigorena, S. (2001). The Cell Biology of Antigen Presentation in Dendritic Cells. Curr. Opin. Immunol. 13, 45–51. doi: 10.1016/s0952-7915(00)00180-1
Deng, G. P., Song, X. M., Fujimoto, S., Ciriaco, A. P., Yasuhiro, N., Mark, I. G. (2019). Foxp3 Post-Translational Modifications and Treg Suppressive Activity. Front. Immunol. 10, 2486. doi: 10.3389/fimmu.2019.02486
Erika, S. C., Verónica, M. H., Enric, M., Jesús, H. (2012). Porcine Reproductive and Respiratory Syndrome Virus Induces CD4+CD8+CD25+Foxp3+regulatory T Cells (Tregs). Virology 430, 73–80. doi: 10.1016/j.virol.2012.04.009
Eyad, E., May, A. A., Belal, C. (2015). Helios, and Not FoxP3, Is the Marker of Activated Tregs Expressing GARP/LAP. Oncotarget 6, 20026–20036. doi: 10.18632/oncotarget.4771
Fleury, A., Cardenas, G., Adalid-Peralta, L., Fragoso, G., Sciutto, E. (2016). Immunopathology in Taenia Solium Neurocysticercosis. Parasite Immunol. 38, 147–157. doi: 10.1111/pim.12299
Garcia, H. H., Rodriguez, S., Friedland, J. S. (2014). Immunology of Taenia Solium Taeniasis and Human Cysticercosis. Parasite Immunol. 36, 388–396. doi: 10.1111/pim.12126
Gazzinelli-Guimaraes, P. H., Nutman, T. B. (2018). Helminth Parasites and Immune Regulation. F1000 Res. 7. doi: 10.12688/f1000research.15596.1
Georgiev, P., Charbonnier, L. M., Chatila, T. A. (2019). Regulatory T Cells: The Many Faces of Foxp3. J. Clin. Immunol. 39, 623–640. doi: 10.1007/s10875-019-00684-7
Ge, Y. Y., Zhang, L., Zhang, G., Wu, J. P., Tan, M. J., Hu, E., et al. (2010). In Pregnant Mice, the Infection of Toxoplasma Gondii Causes the Decrease of CD4+CD25+ -Regulatory T Cells. Parasite Immunol. 30, 471–481. doi: 10.1111/j.1365-3024.2008.01044.x
Gonzales, I., Rivera, J. T., Garcia, H. H. (2016). Pathogenesis of Taenia Solium Taeniasis and Cysticercosis. Parasite Immunol. 38, 136–146. doi: 10.1111/pim.12307
Grainger, J. R., KSmith, K. A., Hewitson, J. P., McSorley, H. J., Harcus, Y., Filbey, K. J., et al. (2020). Helminth Secretions Induce De Novo T Cell Foxp3 Expression and Regulatory Function Through the TGF-β Pathway. J. Exp. Med. 207, 2331–2341. doi: 10.1084/jem.20101074
Hayam, M. E. E., Hanan, H. K., Abeer, F. B., Lobna, S. S. (2013). A Comparative Study Between Excretory/Secretory and Autoclaved Vaccines Against RH Strain of Toxoplasma Gondii in Murine Models. J. Parasitol. Dis. 39, 526–535. doi: 10.1007/s12639-013-0390-6
Hewitson, J. P., Grainger, J. R., Maizels, R. M. (2009). Helminth Immunoregulation: The Role of Parasite Secreted Proteins in Modulating Host Immunity. Mol. Biochem. Parasitol. 167, 1–11. doi: 10.1016/j.molbiopara.2009.04.008
Iddawela, R. D., Rajapakse, R. P. V. J., Perera, N. A. N. D., Agatsuma, T. (2007). Characterization of a Toxocara Canis Species-Specific Excretory-Secretory Antigen (TcES-57) and Development of a Double Sandwich ELISA for Diagnosis of Visceral Larva Migrans. Korean J. Parasitol. 45, 19–26. doi: 10.3347/kjp.2007.45.1.19
Javaid, A. G., Bu, Y. Q., Muhammad, E., Zhang, Z. C., Wang, S., Yan, R. F., et al. (2016). Haemonchus Contortus Excretory and Secretory Proteins (HcESPs) Suppress Functions of Goat PBMCs In Vitro. Oncotarget 7, 35670–35679. doi: 10.18632/oncotarget.9589
Jia, N., Yang, L. Y., Guo, Z. S., Andy, T., Bin, L. (2015). Foxp3+ Treg Cells and Gender Bias in Autoimmune Diseases. Front. Immunol. 6. doi: 10.3389/fimmu
Junginger, J., Raue, K., Wolf, K., Janecek, E., Stein, V. M., Tipold, A., et al. (2017). Zoonotic Intestinal Helminths Interact With the Canine Immune System by Modulating T Cell Responses and Preventing Dendritic Cell Maturation. Sic. Rep. 7, 10310. doi: 10.1038/s41598-017-10677-4
Kumar, R., Ng, S., Engwerda, C. (2019). The Role of IL-10 in Malaria: A Double Edged Sword. Front. Immunol. 10, 229. doi: 10.3389/fimmu.2019.00229
Maizels, R. M., McSorley, H. J. (2016). Regulation of the Host Immune System by Helminth Parasites. J. Allergy Clin. Immunol. 138, 666–675. doi: 10.1016/j.jaci.2016.07.007
Mendlovic, F., Cruz-Rivera, M., Ávila, G., Vaughan, G., Flisser, A. (2015). Cytokine, Antibody and Proliferative Cellular Responses Elicited by Taenia Solium Calreticulin Upon Experimental Infection in Hamsters. PloS One 10, e0141177. doi: 10.1371/journal.pone.0121321
Molinari, J. L., García-Mendoza, E., Garza, Y. D. L., Ramírez, J. A., Sotelo, J., Tato, P. (2002). Discrimination Between Active and Inactive Neurocysticercosis by Metacestode Excretory/Secretory Antigens of Taenia Solium in an Enzyme-Linked Immunosorbent Assay. Am. J. Trop. Med. Hyg. 66, 777–781. doi: 10.4269/ajtmh.2002.66.777
Musumeci, A., Lutz, K., Winheim, E., Krug, A. B. (2019). What Makes a pDC: Recent Advances in Understanding Plasmacytoid DC Development and Heterogeneity. Front. Immunol. 10, 1222. doi: 10.3389/fimmu.2019.01222
Nicholas, D., Blaise, D. (2017). Hookworm Excretory/Secretory Products Modulate Immune Responses to Heterologous and Species -Specific Antigens. Parasite Immunol. 39. doi: 10.1111/pim.12459
Ouyang, W. J., O’Garra, A. (2019). IL-10 Family Cytokines IL-10 and IL-22: From Basic Science to Clinical Translation. Immunity 50, 871–891. doi: 10.1016/j.immuni.2019.03.020
Paredes, A., Sáenz, P., Marzal, M. W., Orrego, M. A., Castillo, Y., Rivera, A., et al. (2016). Anti-Taenia Solium Monoclonal Antibodies for the Detection of Parasite Antigens in Body Fluids From Patients With Neurocysticercosis. Exp. Parasitol. 166, 37–43. doi: 10.1016/j.exppara
Passos, L. S. A., Magalhães, L. M. D., Soares, R. P., Marques, A. F., Nunes, M., do, C. P., et al. (2017). Specific Activation of CD4-CD8- Double Negative T Cells by Trypanosoma Cruzi-Derived Glycolipids Induces a Pro-Inflammatory Profile Associated With Cardiomyopathy in Chagas Patients. Clin. Exp. Immunol. 190, 122–132. doi: 10.1111/cei.12992
Saraiva, M., Vieira, P., O’Garra, A. (2019). Biology and Therapeutic Potential of Interleukin-10. J. Exp. Med. 21, e20190418. doi: 10.1084/jem.20190418
Stefan, M. G., Ricardo, T. F., Paula, A. F., Massara, C. L., Carvalho, O. D. S., Corrêa-Oliveira, R., et al. (2011). Excretory-Secretory Products From Hookworm L3 and Adult Worms Suppress Proinflammatory Cytokines in Infected Individuals. J. Parasitol. Res. 2011, 512154. doi: 10.1155/2011/512154
Sun, X. M., Guo, K., Hao, C. Y., Zhan, B., Huang, J. J., Zhu, X., et al. (2019). Trichinella Spiralis Excretory–Secretory Products Stimulate Host Regulatory T Cell Differentiation Through Activating Dendritic Cells. Cells 8, 1404. doi: 10.3390/cells8111404
Tharmalingam, J., Prabhakar, A. T., Gangadaran, P., Dorny, P., Vercruysse, J., Geldhof, P., et al. (2016). Host Th1/Th2 Immune Response to Taenia Solium Cyst Antigens in Relation to Cyst Burden of Neurocysticercosis. Parasite Immunol. 38, 628–634. doi: 10.1111/pim.12351
Thornton, A. M., Shevach, E. M. (2019). Helios: Still Behind the Clouds. Immunology 158, 161–170. doi: 10.1111/imm.13115
Uchikawa, R., Matsuda, S., Arizono, N. (2000). Suppression of Gamma Interferon Transcription and Production by Nematode Excretory-Secretory Antigen During Polyclonal Stimulation of Rat Lymph Node T Cells. Infect. Immun. 68, 6233–6239. doi: 10.1128/IAI.68.11.6233-6239.2000
Valent, D., Arroyo, L., Peña, R., Yu, K., Carreras, R., Mainau, E., et al. (2017). Effects on Pig Immunophysiology, PBMC Proteome and Brain Neurotransmitters Caused by Group Mixing Stress and Human-Animal Relationship. PloS One 12, e0176928. doi: 10.1371/journal.pone.0176928
Verastegui, M., Gilman, R. H., Gonzales, A., Garcia, H. H., Gavidia, C., Falcon, N., et al. (2001). Taenia Solium Oncosphere Antigens Induce Immunity in Pigs Against Experimental Cysticercosis. Vet. Parasitol. 108, 49–62. doi: 10.1016/s0304-4017(02)00182-6
Wan, Y. Y., Flavell, R. A. (2009). How Diverse–CD4 Effector T Cells and Their Functions. J. Mol. Cell. Bio. 1, 20–36. doi: 10.1093/jmcb/mjp001
Wang, Z. H., Nalu, N. A., Jigesh, A. S., Zhang, H. P., Huang, Q., Zheng, Q., et al. (2016). Porcine Treg Depletion With a Novel Diphtheria Toxin-Based Anti-Human CCR4 Immunotoxin. Vet. Immunol. Immunopathol. 182, 150–158. doi: 10.1016/j.vetimm.2016.10.014
Wang, S., Zhang, Z. C., Wang, Y. J., Gadahi, J. A., Xie, Q., Xu, L., et al. (2017). Toxoplasma Gondii Excretory/Secretory Antigens (TgESAs) Suppress Pro-Inflammatory Cytokine Secretion by Inhibiting TLR-Induced NF-κb Activation in LPS-Stimulated Murine Macrophages. Oncotarget 8, 88351–88359. doi: 10.18632/oncotarget.19362
Wang, Y., Zhou, H. J., Shen, Y. J., Wang, Y. J., Wu, W. P., Liu, H. P., et al. (2015). Impairment of Dendritic Cell Function and Induction of CD4+CD25+Foxp3+ T Cells by Excretory-Secretory Products: A Potential Mechanism of Immune Evasion Adopted by Echinococcus Granulosus. BMC Immunol. 16, 44. doi: 10.1186/s12865-015-0110-3
Weiss, K. A., Christiaansen, A. F., Fulton, R. B., Meyerholz, D. K., Varga, S. M. (2011). Multiple CD4+ T Cell Subsets Produce Immunomodulatory IL-10 During Respiratory Syncytial Virus Infection. J. Immunol. 187, 3145–3154. doi: 10.4049/jimmunol.1100764
White, R. R., Artavanis-Tsakonas, K. (2012). How Helminths Use Excretory Secretory Fractions to Modulate Dendritic Cells. Virulence 3, 668–677. doi: 10.4161/viru.22832
Xu, X. Q., Wang, R., Su, Q., Huang, H. Y., Zhou, P., Luan, J. W., et al. (2016). Expression of Th1- Th2- and Th17-Associated Cytokines in Laryngeal Carcinoma. Oncol. Lett. 12, 1941–1948. doi: 10.3892/ol.2016.4854
Zhou, H. Y., Wang, Y. M., Lian, Q. S., Yang, B., Ma, Y. L., Wu, X. D., et al. (2014). Differential IL-10 Production by DCs Determines the Distinct Adjuvant Effects of LPS and PTX in EAE Induction. E. J. Immunol. 44, 1352–1362. doi: 10.1002/eji.201343744
Keywords: Taenia solium, Cysticercus cellulosae, excretory secretory antigens, cellular immune response, T cells
Citation: Fan X, Zhang Y, Ouyang R, Luo B, Li L, He W, Liu M, Jiang N, Yang F, Wang L and Zhou B (2021) Cysticercus cellulosae Regulates T-Cell Responses and Interacts With the Host Immune System by Excreting and Secreting Antigens. Front. Cell. Infect. Microbiol. 11:728222. doi: 10.3389/fcimb.2021.728222
Received: 21 June 2021; Accepted: 06 August 2021;
Published: 03 September 2021.
Edited by:
Qingfeng Zhang, Tongji University, ChinaReviewed by:
Yujian Wang, Huizhou University, ChinaJavaid Ali Gadahi, Sindh Agriculture University, Pakistan
Copyright © 2021 Fan, Zhang, Ouyang, Luo, Li, He, Liu, Jiang, Yang, Wang and Zhou. This is an open-access article distributed under the terms of the Creative Commons Attribution License (CC BY). The use, distribution or reproduction in other forums is permitted, provided the original author(s) and the copyright owner(s) are credited and that the original publication in this journal is cited, in accordance with accepted academic practice. No use, distribution or reproduction is permitted which does not comply with these terms.
*Correspondence: Biying Zhou, MTQ1ODAzMDg3MUBxcS5jb20=