- 1EA 7380 Dynamyc, Université Paris-Est Créteil, Ecole nationale vétérinaire d'Alfort, USC Anses, Créteil, France
- 2Unité de Bactériologie-Hygiène, Département de prévention, diagnostic et traitement des infections, Hôpital Henri Mondor, AP-HP, Créteil, France
- 3LABGeM, Génomique Métabolique, CEA, Genoscope, Institut François Jacob, Université d'Evry, Université Paris-Saclay, CNRS, Evry, France
- 4Unité de Parasitologie-Mycologie, Ecole nationale vétérinaire d'Alfort, Maisons-Alfort, France
- 5Unité de Parasitologie-Mycologie, Service de Microbiologie, Hôpital Européen Georges Pompidou, AP-HP, Université Paris-Descartes, Paris, France
- 6Unité de Parasitologie-Mycologie, Département de prévention, diagnostic et traitement des infections, Hôpital Henri Mondor, AP-HP, Créteil, France
Introduction: The complexity of biofilms constitutes a therapeutic challenge and the antimicrobial susceptibility of fungal-bacterial biofilms remains poorly studied. The filamentous fungus Aspergillus fumigatus (Af) and the Gram-negative bacillus Stenotrophomonas maltophilia (Sm) can form biofilms and can be co-isolated from the airways of cystic fibrosis (CF) patients. We previously developed an in vitro biofilm model which highlighted the antibiosis effect of Sm on Af, which was dependent on the bacterial fitness. The aim of the present study was to investigate the in vitro susceptibility of Af and Sm in mono- or polymicrobial biofilms to five antimicrobial agents alone and in two-drug combinations.
Methods: Af and Sm clinical reference strains and two strains from CF sputa were tested through a planktonic and biofilm approaches. Af, Sm, or Af-Sm susceptibilities to amphotericin B (AMB), itraconazole (ITC), voriconazole (VRC), levofloxacin (LVX), and rifampicin (RFN) were evaluated by conventional planktonic techniques, crystal violet, XTT, qPCR, and viable plate count.
Results: Af planktonic cells and biofilms in formation were more susceptible to AMB, ITC, and VRC than Af mature biofilms. Af mature biofilms were susceptible to AMB, but not to ITC and VRC. Based on viable plate count, a lower concentration of LVX and RFN was required to reduce Sm cell numbers on biofilms in formation compared with mature biofilms. The antibiosis effect of Sm on Af growth was more pronounced for the association of CF strains that exhibited a higher fitness than the reference strains. In Af-Sm biofilms, the fungal susceptibility to AMB was increased compared with Af biofilms. In contrast, the bacterial susceptibility to LVX decreased in Af-Sm biofilms and was fungal biomass-dependent. The combination of AMB (64 μg/mL) with LVX or RFN (4 μg/mL) was efficient to impair Af and Sm growth in the polymicrobial biofilm.
Conclusion: Sm increased the Af susceptibility to AMB, whereas Af protected Sm from LVX. Interactions between Af and Sm within biofilms modulate susceptibility to antimicrobial agents, opening the way to new antimicrobial strategies in CF patients.
Introduction
Biofilm-embedded cells have specific characteristics, which distinguish them from planktonic cells, namely their lower susceptibility to drugs explained in particular by the presence of self-produced protective matrix and the reduction of microbial metabolic activity in the biofilm (Desai et al., 2014; Lebeaux et al., 2014; Flemming et al., 2016).
Many microorganisms are commonly co-isolated from the airways of cystic fibrosis (CF) patients (Botterel et al., 2018; Granchelli et al., 2018), including the filamentous fungus Aspergillus fumigatus (Burgel et al., 2016) and the Gram-negative bacillus Stenotrophomonas maltophilia (Esposito et al., 2017). Approximatively, 10% of the French CF patients carry in their airways S. maltophilia, and 30 % carry Aspergillus (French, 2019), with common co-infections, as recently shown in a very large cohort of CF patients (Granchelli et al., 2018). Treatment of these pathogens in chronic respiratory diseases is often difficult due to their multidrug-resistant nature, especially for S. maltophilia, and to their biofilm-forming ability (Flores-Treviño et al., 2019). The presence of microbial aggregates and biofilms have already been observed in the respiratory tract of CF patients, which is a favorable environment for biofilm formation (Bjarnsholt et al., 2009; Ramage et al., 2011; Kragh et al., 2014). S. maltophilia biofilm was documented in the sputum of CF patients (Høiby et al., 2017), although there is no direct evidence supporting the presence of Aspergillus biofilm in vivo in CF patients.
The complexity of biofilm structure constitutes a therapeutic challenge since infections are often treated with drugs selected according to the results of susceptibility testing of microorganisms in planktonic form. Furthermore, Keays et al. (2009) showed a better clinical outcome when CF patients were treated with efficient biofilm-targeting agents. The in vitro antifungal tolerance of A. fumigatus in biofilm has already been reported (Mowat et al., 2007; Seidler et al., 2008; Bugli et al., 2013; Luo et al., 2018). Mowat et al. (2007) showed that amphotericin B, itraconazole, voriconazole, and caspofungin were 1,000 times less efficient on biofilm-life form than on planktonic form. Several studies showed that levofloxacin could be an alternative to treat S. maltophilia infections (King et al., 2010; Wu et al., 2013; Herrera-Heredia et al., 2017; Pompilio et al., 2020). This molecule could reduce biofilm biomass (Di Bonaventura et al., 2004; Passerini de Rossi et al., 2009), but some levofloxacin-resistant strains emerge (Wang et al., 2020). Another study suggested the combination of old alternatives, such as rifampicin, with newer agents for critically ill patients infected with S. maltophilia (Savini et al., 2010). Rifampicin is known for its anti-biofilm activity and synergistic effect with several antibiotics targeting Gram-positive bacteria (Tang et al., 2013; Yan et al., 2018).
Regarding microbial intra-kingdom interactions, the antimicrobial susceptibility of different bacterial species growing inside polymicrobial biofilms has been investigated (Pompilio et al., 2015; Cendra et al., 2019; Rodríguez-Sevilla et al., 2019). Cross-kingdom interactions impact on antimicrobial susceptibility is still poorly studied, but some authors have described the antimicrobial susceptibility of Candida albicans yeast in polymicrobial biofilm with Staphylococcus aureus (Harriott and Noverr, 2009; Kong et al., 2016; Rogiers et al., 2018) or Cutibacterium acnes (Bernard et al., 2018). To our knowledge, only (Manavathu et al., 2014; Manavathu and Vazquez, 2015) described the antimicrobial susceptibility of an in vitro filamentous fungal (A. fumigatus) and bacterial (Pseudomonas aeruginosa) biofilm. In that model, A. fumigatus had the same antifungal susceptibility in mono- and polymicrobial biofilms. Regarding the susceptibility of P. aeruginosa, cefepime and imipenem were significantly less efficient in the polymicrobial biofilm than in the monomicrobial biofilm.
We previously showed that S. maltophilia inhibited A. fumigatus growth and modified hyphae development in a polymicrobial biofilm (Melloul et al., 2016), with strain-dependent manner (Melloul et al., 2018). The aim of the present study was to investigate the in vitro antimicrobial response of A. fumigatus and S. maltophilia in our polymicrobial biofilm in comparison with monomicrobial biofilm.
Materials and Methods
Strains and Standardization of Inocula
Aspergillus fumigatus (Af) ATCC 13073-GFP (Wasylnka and Moore, 2002) expressing a constitutive Green Fluorescent Protein (AF_REF), and S. maltophilia (Sm) ATCC 13637 (SM_REF) were the clinical reference strains used in this study. Two other clinical strains obtained from sputa of CF patients, named AF_CF and SM_CF, were used. AF_CF is A. fumigatus sensu stricto as identified by molecular technique using sequence analysis of beta-tubulin gene as previously described (Loeffert et al., 2017). The genomic phylogeny of Sm was recently updated (Vinuesa et al., 2018) and S. maltophilia complex was defined, including Sm sensu stricto and several related genospecies. SM_CF is S. maltophilia sensu stricto through whole genome sequencing and phylogenomic analysis (Mercier-Darty et al., 2020). Both SM_REF and SM_CF belong to genogroup 6 (Hauben et al., 1999; Mercier-Darty et al., 2020), and no major difference in resistance genes was found. Af strains were cultured on 2% malt agar containing 0.05% chloramphenicol at 37°C for 5 days. The fungal suspensions were prepared as previously described to obtain an inoculum of 105 conidia/mL in 3-(N-morpholino) propanesulfonic acid (MOPS) - buffered RPMI 1640 [pH 7.0] with 2% glucose (G) + 10 % fetal bovine serum (FBS) (Sigma-Aldrich, France) (Melloul et al., 2016). Sm strains were streaked out on Luria-Bertani (LB) agar plate at 37°C for 24 h. The bacterial suspensions were also prepared as previously reported to obtain an inoculum of 106 bacteria/mL (Melloul et al., 2016). These inocula were used to test antimicrobial susceptibilities of planktonic cells and biofilms.
Fungal, Bacterial, and Polymicrobial Biofilm Formation
The in vitro biofilm formation in the 96-well plates (Thermo Fisher Scientific Inc, France) was adapted from the protocol previously described (Melloul et al., 2016). Briefly, 50 μL of the fungal (105 conidia/mL) or bacterial (106 bacteria/mL) inoculum was added to 50 μL of MOPS–RPMI (2 % G) + 10 % FBS to form Af or Sm monomicrobial biofilm. The Af-Sm polymicrobial biofilm was produced by simultaneous inoculation of 50 μL of each inoculum per well. The tested microbial associations were AF_REF + SM_REF and AF_CF + SM_CF. Plates were incubated at 37°C in static condition for 24 h to obtain mature biofilms (biofilm-embedded cells), then washed twice with PBS to remove planktonic cells.
Antimicrobial Agents
Pure antimicrobial powders were obtained from Sigma-Aldrich, France. The antifungal stock solutions of amphotericin B (AMB), itraconazole (ITC), and voriconazole (VRC) were prepared at 10 mg/mL in dimethylsulfoxide (DMSO). The antibiotic stock solutions of levofloxacin (LVX) and rifampicin (RFN) were prepared at 6.4 mg/mL in sterile distilled water and DMSO, respectively. Stock solutions were kept at −20°C until used. Working solutions were then adjusted in MOPS–RPMI (2% G).
Determination of Minimum Inhibitory Concentration (MIC) and Minimum Bactericidal Concentration (MBC) of Planktonic Cells
The MICs of AMB, ITC, and VRC were determined by the EUCAST reference microdilution broth technique (Arendrup et al., 2020). The MICs of LVX and RFN were determined following the recommendations from the International Standards Organization (ISO, 2019). MIC was defined as the lowest concentration of drug required for complete growth inhibition with a visual endpoint for Af and a spectrophotometric endpoint for Sm using a microplate reader set at 550 nm (Multiskan FC®, Thermo Fisher Scientific Inc, France). To further compare planktonic and biofilm-embedded cells susceptibilities, the culture conditions of planktonic cells were adjusted to 105 conidia/mL or 106 bacteria/mL and were prepared in MOPS–RPMI (2% G) + 10% FBS medium. In such conditions, the minimum inhibitory concentration (MICb) was determined after 24 h of culture using same endpoints as above (Figure 1A).
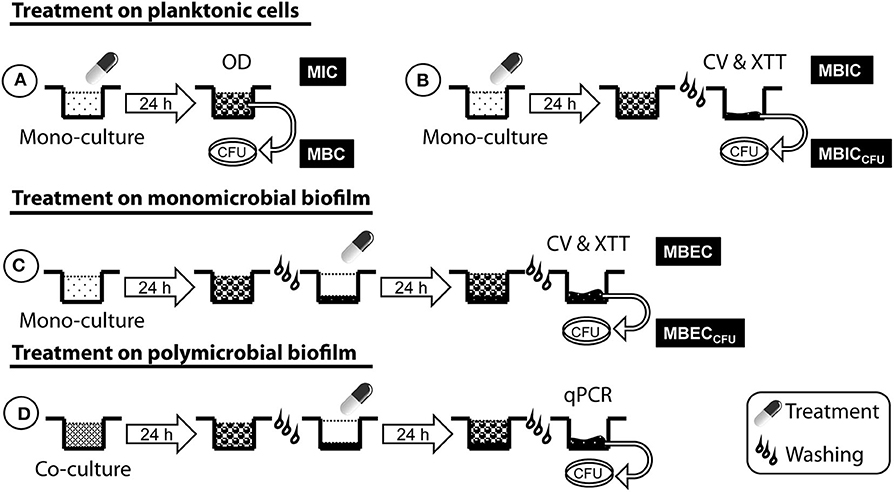
Figure 1. Antimicrobial susceptibility testing of planktonic cells and biofilm forms. (A,B) Mono-cultures with conidia or bacteria were simultaneously inoculated with antimicrobial agents for 24 h. Then, the optical density (OD) was measured to obtain the MIC (in planktonic cells), and the wells were washed before crystal violet (CV) and XTT analyses to determine the drug concentration that inhibited the biofilm formation (MBIC). (C,D) Mono- and co-cultures were incubated for 24 h to obtain mature mono- and polymicrobial biofilms, and then treated with antimicrobial agents for another 24 h. (C) CV and XTT analyses on monomicrobial biofilm enabled determining the drug concentration that eradicated the mature biofilm (MBEC). The viability of Sm following antibacterial treatment was assessed on planktonic cells (MBC), adherent cells of biofilm in formation (MBICCFU), and mature biofilm (MBECCFU). (D) Antimicrobial susceptibility of mono- and polymicrobial biofilms was compared using qPCR and viable plate count.
MBC was evaluated and defined as the lowest concentration of antibiotic required to reduce Sm CFU by 99.9% as compared with the initial inoculum. MBC was determined by plating 200 μL from each well that showed no visible growth on cation-adjusted Mueller-Hinton (CAMH) (Sigma-Aldrich, France) agar plates incubated at 37°C for 48 h, and CFUs were enumerated. Each experiment was performed in triplicate.
Determination of Minimum Biofilm Inhibitory Concentration (MBIC) and Viability of S. maltophilia Cells Extracted From Biofilm in Formation
To determine the effects of antimicrobial agents on Af and Sm biofilm formation, 50 μL of the fungal (105 conidia/mL) or bacterial (106 bacteria/mL) inoculum was mixed with 50 μL of the antimicrobial agent (2X final concentration) into wells of the 96-well plates and incubated at 37°C in static condition for 24 h. Then, the supernatant was removed, and plates were washed twice with PBS. The final concentration range of the antimicrobial agent was 0.06–8 μg/mL. The lowest concentration of drug required to inhibit at least 90% of the biofilm formation (MBIC) was assessed by the crystal violet (CV) staining method (biomass measurement), and by the 2,3-bis(2-methoxy-4-nitro-5-sulfo-phenyl)-2H-tetrazolium-5carboxanilide (XTT) reduction method (metabolic activity measurement) (Figure 1B). Wells were stained with 200 μL of CV (0.02% for Af, 0.1% for Sm) for 30 min at room temperature, then washed thrice with PBS before adding 200 μL of 30% acetic acid for 10 min. The XTT reduction method was used according to Pierce et al. (2008) with minor modifications. Briefly, a final solution containing 0.5 mg/mL of XTT (Invitrogen, France) + 50 μM (Af) or 10 μM (Sm) of menadione (Merck, Germany) was added into wells and plates were incubated in the dark at 37°C for 2 h. The optical density values of blank wells were subtracted from the test wells. Each test was run in triplicates and three independent experiments were performed.
The viability of adherent bacterial cells was checked on agar plates. The MBIC based on CFU enumeration (MBICCFU) was defined as the lowest concentration of antibiotic required to reduce Sm cell number by 90% on biofilm in formation. Following antibiotic exposure of Sm inoculum, wells were washed, and adherent cells were scraped with swab and plated on LB agar for 24 h at 37°C. All assays were performed in triplicate and repeated three times.
Determination of Minimum Biofilm Eradication Concentration (MBEC) and Viability of S. maltophilia Cells Extracted From Mature Biofilm
Monomicrobial (Af or Sm) mature biofilms were exposed to a range of concentrations of drugs (100 μL) at 37°C for 24 h. The highest concentrations were 256 μg/mL for antifungal and 32 μg/mL for antibacterial agents. For each experiment, some biofilms were not treated with drugs (untreated biofilms). Following washing, the susceptibility testing was performed using CV and XTT (Figure 1C) as described above. Both methods helped determine the MBEC, which was defined as the lowest concentration of drug required to eradicate at least 90% of mature biofilm.
The MBEC based on CFU enumeration (MBECCFU) was evaluated and defined as the lowest concentration of antibiotic required to reduce Sm cell number by 90% on mature biofilm. Following antibiotic treatment of Sm biofilm, wells were washed, and adherent cells were scraped with swab and plated on LB agar for 24 h at 37°C. All assays were performed in triplicate and repeated three times.
Determination of Polymicrobial Biofilm Susceptibility by Quantitative PCR
Polymicrobial (Af-Sm) mature biofilms were exposed to a range of concentrations of AMB or LVX at 37°C for 24 h. Two-drug combination experiments were performed with AMB at 64 μg/mL and LVX or RFN at 4 μg/mL. The quantity of Af and Sm DNAs was investigated in the drug-treated or drug-free biofilms by qPCR (Figure 1D). Following washing, biofilms were frozen at −20°C for 24 h. After thawing, biofilms were covered with 250 μL of tissue lysis buffer (ATL, Qiagen GmbH, Germany) and prepared as previously described (Melloul et al., 2016). DNA extraction using QIAamp DNA Mini Kit (Qiagen GmbH, Germany) was performed and the qPCR test was conducted following the protocol previously described (Melloul et al., 2016). Data were analyzed using LightCycler software V3.5 and results were expressed in conidial equivalent (CE) or bacterial equivalent (BE) in comparison with a standard curve plotted on DNA samples extracted from co-inoculated solutions with different concentrations of conidia (1–108 conidia) and bacteria (10–109 bacteria). Results obtained from treated biofilms were expressed in percentage of biomass inhibition compared with untreated biofilms. Each testing condition was performed in duplicate for three independent experiments.
Effect of A. fumigatus on S. maltophilia Biofilm Susceptibility
Comparative Analysis of Viable Bacterial Counts in Monomicrobial and Polymicrobial Biofilms
The effect of Af biomass on the susceptibility of Sm was assessed by a comparative analysis of viable bacterial counts extracted from mono- and polymicrobial biofilms (Figure 1D). One strain association (AF_REF + SM_REF) was used, and the response of SM_REF to LVX (1, 4, and 32 μg/mL) or AMB (64 μg/mL) + LVX (4 μg/mL) was assessed. Biofilms were thoroughly scraped with 200 μL of PBS and collected into tubes, and that was repeated twice for vigorous agitation using MagNA Lyser Instrument (Roche, France). Serial 10-fold dilutions up to 10−5 in PBS were performed and 100 μL from each dilution was plated on LB agar supplemented with 16 μg/mL of ITC to prevent Af growth. The number of CFUs was determined after 24 h of incubation at 37°C. Results were expressed in percentage of survival compared with untreated biofilms. All assays were repeated three times for three independent experiments.
Effect of Fungal Matrix Degradation on S. maltophilia Biofilm Susceptibility
To investigate the effect of AF_REF on the response of SM_REF to LVX, bacterial viability was assessed after enzymatic pretreatment intended to degrade the fungal biofilm extracellular matrix (ECM). The enzymatic degradation protocol was based on a previous research (De Brucker et al., 2015). For such, following Sm and Af-Sm biofilm formation, samples were washed and covered with MOPS–RPMI (2% G) containing 50 μg/mL proteinase K (Qiagen GmbH, Germany) for 2 h at 37°C or with MOPS–RPMI (2% G) as control. Then, biofilms were exposed to LVX at 1 μg/mL for 24 h at 37°C. Bacterial viability was determined using viable plate count as described above. The experiment was performed in triplicate and repeated three times.
Confocal Laser Scanning Microscopy (CLSM) Observations
For microscopic analyses, biofilms were developed on Lab-TekTM slides (Thermo Fisher Scientific Inc, France) in a damp chamber. After 16 h of incubation, wells were washed with PBS. AF_REF, which expresses GFP, was visualized with FITC filter. AF_CF was visualized after Calcofluor-white staining (Invitrogen, France) using DAPI filter. Phenotypic modifications of Af in polymicrobial biofilm were investigated and compared with Af phenotype in monomicrobial biofilm by CLSM. Images of biofilms were obtained by Zeiss LSM 510 META confocal (Zeiss, Germany).
Transmission Electron Microscopy (TEM) Observations
Effects of LVX (8 μg/mL) on polymicrobial biofilms were investigated by TEM. Biofilms were prepared as previously described (Melloul et al., 2016). Briefly, biofilms were first fixed with 2.5% glutaraldehyde-cacodylate (pH 6.5) and then with 2% osmium tetroxide buffer. The fixed samples were dehydrated using a graded ethanol series and embedded in EPON resin for at least 72 h. Ultra-fine sections were cut via ultramicrotome (Leica EM UC7), gently collected on grids, and stained with lead-citrate and uranyl-acetate solutions before observation under TEM (JEOL 100 CX II instrument, Japan).
Data Analyses
Linear regression and Spearman's rank correlation helped determine the relationship between CV and XTT results. Data failed the normality test (Shapiro–Wilk), hence the use of non-parametric tests. Comparisons of responses of mono- and polymicrobial biofilms to antimicrobial concentrations were performed using multiple linear regressions. Pairwise comparisons relied on Wilcoxon test. Statistical analyses were conducted using JMP 14.0 software. P ≤ 0.05 were considered statistically significant.
Results
Comparison of Colorimetric Methods
The effects of various drug concentrations on AF_REF and SM_REF biofilms depending on the used colorimetric method (i.e., CV for biomass measurement or XTT for metabolic activity measurement) are shown in Figure 2. The linear regression and Spearman's rank correlation showed that percentages of inhibition evaluated by CV and XTT methods gave consistent results for antimicrobial susceptibility testing of biofilms in formation (R2 = 0.83, p < 0.0001; Spearman's ρ = 0.8816, p < 0.0001) and mature biofilms (R2 = 0.89, p < 0.0001; Spearman's ρ = 0.8562, p < 0.0001). Therefore, the effects of antimicrobial agents on the other strains (AF_CF and SM_CF) were evaluated only by the XTT reduction method.
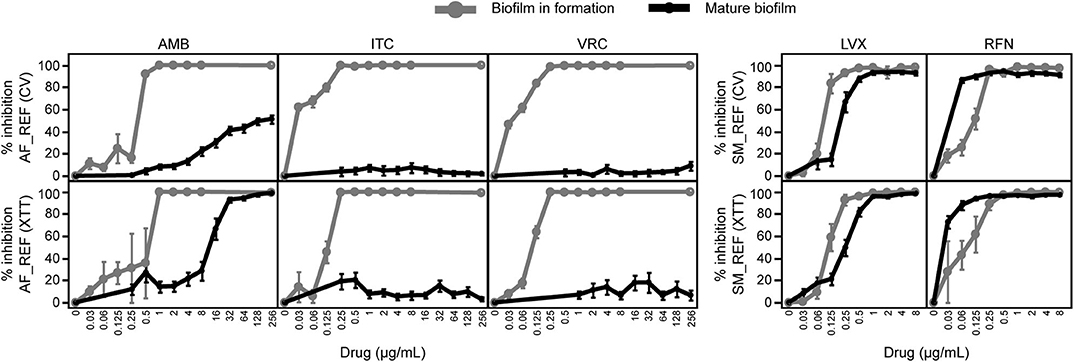
Figure 2. Susceptibilities of biofilms in formation and mature biofilms to drugs. The biomasses and the metabolic activities of biofilms were measured by CV and XTT methods, respectively. Results were expressed in percentages of AF_REF or SM_REF inhibition after antimicrobial treatment compared with untreated controls. AMB, amphotericin B; ITC, itraconazole; VRC, voriconazole; LVX, levofloxacin; RFN, rifampicin.
Effect of Antimicrobial Agents on Planktonic Cells and Biofilm in Formation
MICb helped to compare planktonic and biofilm-embedded cells susceptibilities. The susceptibility values of planktonic Af and Sm strains are listed in Table 1, which displays the mode for each condition amongst the three performed replicates. MICb values of the three antifungals were one dilution lower than MIC values, and this was not considered as a major discrepancy. MIC and MICb of LVX were similar, but showed discrepancy of up to two dilutions for RFN, indicating that Sm strains tended to be more susceptible to RFN in our culture conditions.
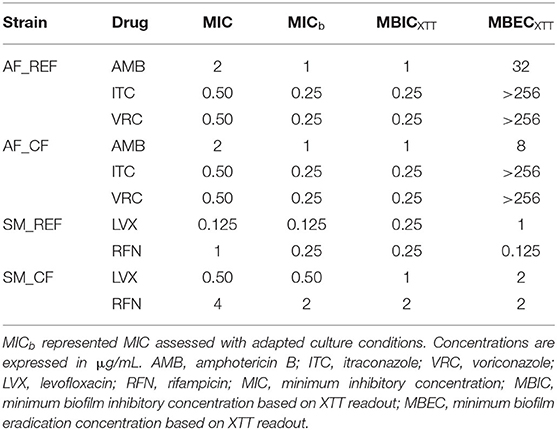
Table 1. Efficacy of drugs to inhibit growth of planktonic cells and to reduce metabolic activity of biofilm cells.
AF_REF and AF_CF were equally susceptible to AMB, ITC, and VRC (MICbs 1, 0.25, and 0.25 μg/mL, respectively). SM_REF and SM_CF were both susceptible to LVX (MICbs ≤ 0.50 μg/mL), and MICb of RFN for SM_CF (2 μg/mL) was 8-fold higher than that for SM_REF (0.25 μg/mL).
Overall, the susceptibility of planktonic cells (MICb) measured by turbidity was similar to the susceptibility of biofilm in formation (MBICXTT) measured by XTT (Table 1).
Decrease of Antimicrobial Susceptibility on Monomicrobial Mature Biofilm Compared With Planktonic Cells and Biofilm in Formation
Antifungal and antibacterial agents exhibited concentration-dependent activities against AF_REF and SM_REF biofilms (Figure 2). The lowest drug concentrations required to reduce metabolic activity of biofilm in formation (MBICXTT) and mature biofilm (MBECXTT) are shown in Table 1. AMB exhibited a greater effect on biofilm formation of Af since MBICXTTs (1 μg/mL) were 8 and 32-fold lower than MBECXTTs for AF_CF and AF_REF, respectively. ITC and VRC were efficient to inhibit biofilm formation of both Af strains (MBICXTTs = 0.25 μg/mL), but not to eradicate mature biofilms (MBECXTTs > 256 μg/mL). Only AMB was efficient on mature biofilms with a slightly greater effect on AF_CF (MBECXTT = 8 μg/mL) compared with AF_REF (MBECXTT = 32 μg/mL). The MBICXTTs of LVX (0.25 and 1 μg/mL for SM_REF and SM_CF, respectively) were lower than MBECXTTs (1 and 2 μg/mL). LVX exhibited a slightly greater effect to reduce metabolic activity of Sm biofilms in formation than Sm mature biofilms. For RFN, MBICXTTs, and MBECXTTs were similar, indicating that RFN had a similar effect on biofilm formation and mature biofilm according to the XTT results.
The viability of antibiotic-treated Sm cells in planktonic cultures (MBC), biofilm in formation (MBICCFU), and mature biofilm (MBECCFU) is shown in Table 2. The MBC of LVX was similar to its MICb, whereas the MBC of RFN was at least 16-fold higher than MICb for both Sm strains (Tables 1, 2). LVX reached its bactericidal effect on Sm strains at lower concentrations than RFN.
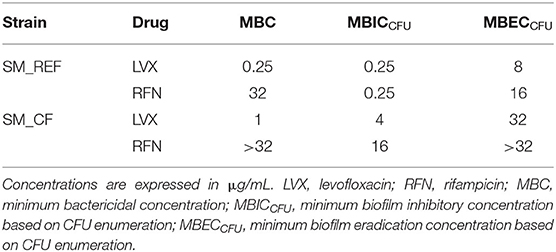
Table 2. Efficacy of drugs to reduce cell numbers on planktonic cultures and biofilm forms of S. maltophilia.
The efficacy of LVX to reduce cell numbers on planktonic cultures (MBC) and biofilm in formation (MBICCFU) was higher than on mature biofilm (MBECCFU), for both Sm strains (Table 2). LVX exhibited a slightly greater effect on SM_REF (MBECCFU = 8 μg/mL) compared with SM_CF (MBECCFU = 32 μg/mL). The MBC of RFN was similar to its MBECCFU for both strains, but the MBECCFU was up to 64-fold higher than MBICCFU. Thus, RFN only seems to reduce the number of adherent Sm cell by inhibiting the biofilm formation.
Fungal Growth Inhibition and Fungal Phenotype Modification in the Presence of S. maltophilia
In AF_REF + SM_REF biofilm, the fungal growth was significantly reduced (p < 0.0001) but not the bacterial growth (p = 0.0520), in comparison with the corresponding monomicrobial biofilms (Figures 3A,B). We obtained the same trend for CF strains: AF_CF in mono- vs. polymicrobial biofilm (p < 0.0001) and SM_CF in mono- vs. polymicrobial biofilm (p = 0.1706; Figures 3A,B). The fungal growth inhibition was higher for the association of CF strains than for REF strains. Specifically, the growth of AF_REF and AF_CF in polymicrobial biofilms was respectively reduced by 2 and 10 compared with that in fungal biofilms. This difference can be attributed to the difference of Sm fitness (Figure 3B). SM_CF grew significantly faster than SM_REF in polymicrobial biofilm (p < 0.0001) and thus induced a larger inhibition of fungal growth. Moreover, the fungal phenotype was modified and showed highly branched hyphae in the presence of bacteria for both associations (Figures 3C,D) in comparison with the corresponding Af monomicrobial biofilm (Figures 3E,F).
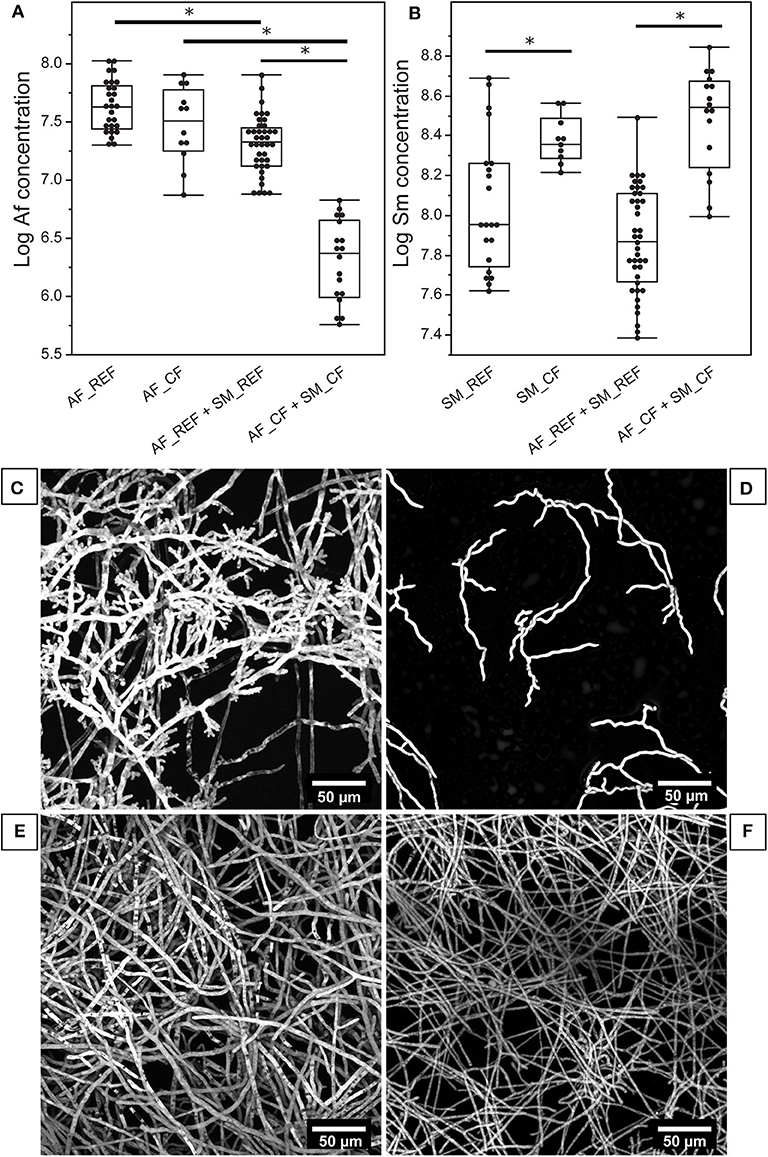
Figure 3. Quantification of fungal and bacterial concentrations in biofilms and phenotype modifications of A. fumigatus in the presence of S. maltophilia. (A,B) Assessment of Af or Sm growth in mono- and polymicrobial biofilms after 24 h of culture by qPCR. *p < 0.05. (C) AF_REF phenotype in polymicrobial biofilm with SM_REF. (D) AF_CF phenotype in polymicrobial biofilm with SM_CF. (E) AF_REF phenotype in monomicrobial biofilm. (F) AF_CF phenotype in monomicrobial biofilm. Af, A. fumigatus; Sm, S. maltophilia.
Modification of A. fumigatus Susceptibility to AMB in Polymicrobial Biofilm
Since no ITC and VRC activities on Af monomicrobial biofilms were found (Table 1), we focused on AMB activity for the following experiments. Af susceptibility to AMB in mono- and polymicrobial biofilms was tested for both strain associations: AF_REF + SM_REF and AF_CF + SM_CF. The percentage of fungal biomass inhibition measured in AMB-treated biofilms was significantly higher in polymicrobial biofilms for both Af strains (multiple linear regressions, biofilm * AMB concentration effect: p < 0.001; Figure 4); i.e., the fungus was more susceptible to AMB in the presence of Sm. The AMB concentrations required to obtain 90% of AF_REF or AF_CF biomass inhibition were at least 32 μg/mL in fungal biofilm and 0.5 or ≤ 0.06 μg/mL in polymicrobial biofilm. The difference in Af susceptibility between fungal and polymicrobial biofilms was observed from 0.25 and ≤ 0.06 μg/mL of AMB for AF_REF and AF_CF, respectively. Overall, Af susceptibility to AMB in polymicrobial biofilm was at least 64-fold higher compared with fungal biofilm (Figure 4), whereas the fungal growth was reduced by 2 times with SM_REF and 10 times with SM_CF (Figure 3A).
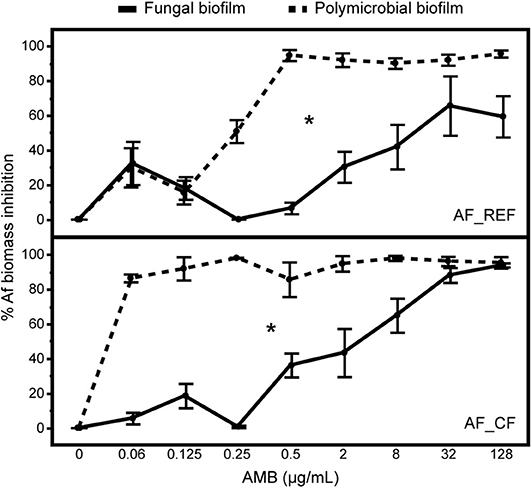
Figure 4. Effects of amphotericin B on A. fumigatus in fungal and polymicrobial biofilms. Assessment of AMB activity on AF_REF or AF_CF in fungal (solid line) and polymicrobial (broken line) biofilms by qPCR. Percentages of biomass inhibition were calculated based on untreated biofilms results. *p < 0.05; Af, A. fumigatus; AMB, amphotericin B.
Modification of S. maltophilia Susceptibility to LVX in Polymicrobial Biofilm
The susceptibility of Sm in bacterial and polymicrobial biofilms was compared in response to LVX. Figure 5A results showed that LVX had a greater effect on SM_REF in bacterial biofilm than in polymicrobial biofilm (multiple linear regressions, p < 0.0001). In the bacterial biofilm, the inhibition level raised gradually and proportionally with the increase in LVX concentration until 90% at 1 μg/mL. This result was consistent with that of MBECXTT (1 μg/mL, Table 1). Concerning SM_REF in polymicrobial biofilm, LVX had a limited effect (20% inhibition, regardless of LVX concentration) (Figure 5A). In addition to these results, the bacterial survival was assessed by subculturing the LVX-treated biofilms (Figure 5B). The results showed a significantly higher survival rate of SM_REF in polymicrobial biofilm than in bacterial biofilm following LVX treatment at 1 μg/mL (p = 0.0003), 4 μg/mL (p = 0.0002), and 32 μg/mL (p = 0.0025). In the bacterial biofilm, 2% (~106 bacteria/mL) and 0.001% (103 bacteria/mL) of survival rates were recorded following exposure to 1 and 32 μg/mL of LVX, respectively. In contrast, in the polymicrobial biofilm, almost 40 and 6% of Sm were still alive after exposure to 1 and 32 μg/mL of LVX, respectively. For SM_CF, qPCR results showed a similar antibacterial effect of LVX on the bacterial and polymicrobial biofilms (multiple linear regressions, p = 0.8550; Figure 6). Regardless of the LVX concentration used, the presence of AF_CF had no effect on SM_CF susceptibility to LVX, while the presence of AF_REF decreased the susceptibility of SM_REF to LVX. This difference was probably due to the higher inhibition of Af growth exhibited in the association of CF strains (Figure 3A). In addition, TEM experiments were performed to visualize effects of LVX (8 μg/mL) on polymicrobial biofilms. SM_REF cells grown with AF_REF did not show any signs of severe damage after LVX treatment (Figures 7A,B), whereas SM_CF cells appeared broken and emptied of their contents in LVX-treated polymicrobial biofilm (Figures 7C,D).
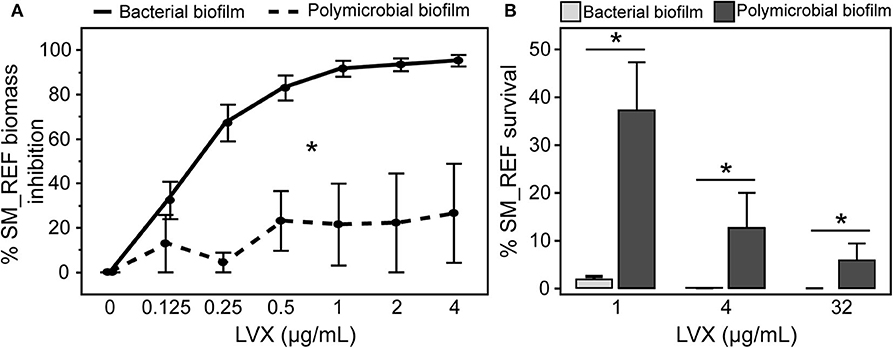
Figure 5. Effects of levofloxacin on SM_REF in bacterial and polymicrobial biofilms. (A) Assessment of LVX activity on SM_REF in bacterial (solid line) and polymicrobial (broken line) biofilms by qPCR. (B) Percentages of SM_REF survival following LVX (1, 4, and 32 μg/mL) treatment in bacterial (light gray) and polymicrobial (dark gray) biofilms using viable plate count. *p < 0.05; LVX, levofloxacin.
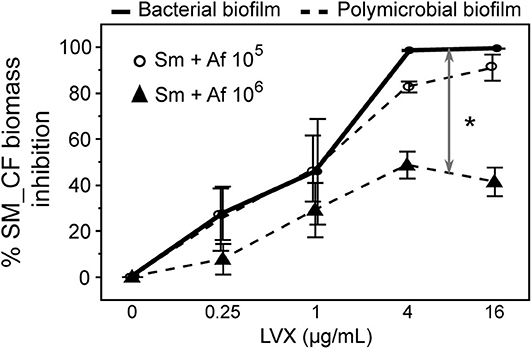
Figure 6. Effects of levofloxacin on SM_CF in bacterial and polymicrobial biofilms. Susceptibility of SM_CF to LVX was assessed in bacterial (solid line) and polymicrobial biofilms (broken lines) with an initial AF_CF inoculum of 105 conidia/mL (circle) or 106 conidia/mL (triangle) by qPCR. *p < 0.05; LVX, levofloxacin.
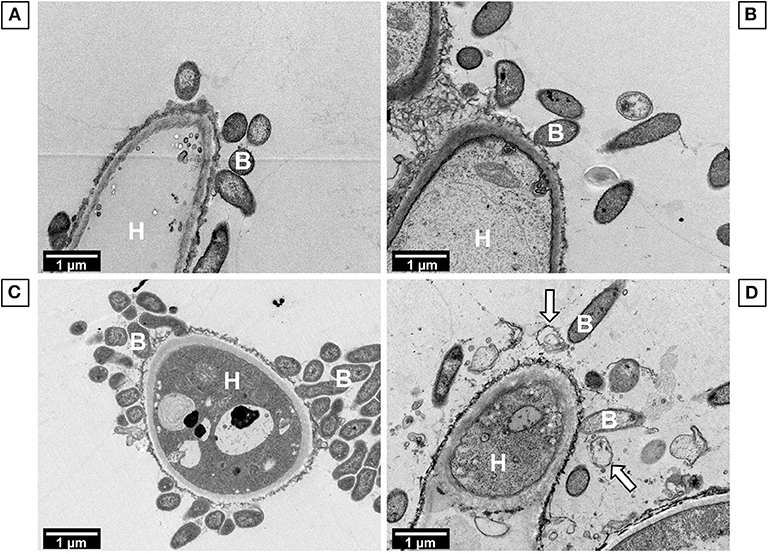
Figure 7. TEM observations of A. fumigatus-S. maltophilia biofilms exposed to levofloxacin. (A) AF_REF + SM_REF untreated biofilm. (B) AF_REF + SM_REF biofilm treated with 8 μg/mL LVX. (C) AF_CF + SM_CF untreated biofilm. (D) AF_CF + SM_CF biofilm treated with 8 μg/mL LVX. H, hypha; B, bacteria. The white arrows show damages to bacterial cells.
Decrease of S. maltophilia Susceptibility to LVX in Polymicrobial Biofilm Is Related to the A. fumigatus Biomass
Since the fungal biomass in the AF_CF + SM_CF biofilm was lower than in the AF_REF + SM_REF biofilm (Figure 3A), we hypothesized that the fungal biomass was responsible for the significant decrease of SM_REF susceptibility to LVX observed in polymicrobial biofilm (Figure 5A), in contrast to SM_CF (Figure 6). To test this hypothesis, we carried out experiments using different concentrations of AF_CF (105 or 106 conidia/mL) for the same SM_CF concentration (106 bacteria/mL) in order to reduce the fungal growth inhibition caused by the bacteria. There was a bigger fungal biomass (+ 0.5 log CE/mL, data not shown) in the polymicrobial biofilm formed with 106 conidia/mL than that observed in the polymicrobial biofilm with 105 conidia/mL. In the bacterial biofilm, 4 μg/mL of LVX was enough to achieve 90% SM_CF inhibition, which was not the case in the polymicrobial biofilm (performed with 106 conidia/mL) where 16 μg/mL of LVX could not exceed 50% inhibition. The presence of higher AF_CF biomass helped to protect SM_CF from LVX (multiple linear regressions, p = 0.0121; Figure 6).
Role of the Fungal ECM in S. maltophilia Protection From LVX
Fungal ECM may have a role in decreasing Sm susceptibility to LVX in polymicrobial biofilm. To support this hypothesis, Sm and Af-Sm biofilms were pretreated with proteinase K to degrade proteins of ECM before analyzing the bacterial survival following LVX treatment at 1 μg/mL. Proteinase K did not affect the SM_REF response to LVX in bacterial biofilm (p = 0.8563; Figure 8). However, a significant decrease of SM_REF survival following LVX treatment was observed in polymicrobial biofilm pretreated with Proteinase K compared with unpretreated biofilm (p = 0.0333; Figure 8), suggesting that AF_REF ECM is involved in the protection of SM_REF from LVX.
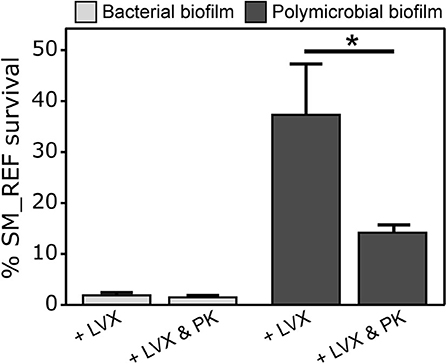
Figure 8. Effects of A. fumigatus matrix on S. maltophilia biofilm susceptibility to levofloxacin. Bacterial and polymicrobial biofilms were treated with or without proteinase K (50 μg/mL) for 2 h before LVX treatment at 1 μg/mL. Percentages of SM_REF survival was assessed using viable plate count. *p < 0.05; LVX, levofloxacin; PK, proteinase K.
Antifungal and Antibacterial Combination Strategies to Treat Polymicrobial Biofilm
Antifungal-antibacterial combinations were tested on Af-Sm biofilm in order to impair both pathogens and to provide more evidence that Af plays a role in protecting Sm from the effects of antibiotics. Bacterial and polymicrobial biofilms of REF strains were exposed to AMB at 64 μg/mL combined with LVX or RFN at 4 μg/mL, and to each drug alone.
In polymicrobial biofilm, the susceptibility of AF_REF to AMB alone or in combination with antibiotics did not differ (AMB vs. AMB + LVX: p = 0.2027; AMB vs. AMB + RFN: p = 0.3801) (data not shown). These results suggested that the antibiotics did not affect the antifungal efficacy of AMB on Af in polymicrobial biofilm.
When the polymicrobial biofilm was treated with AMB (64 μg/mL), the growth of SM_REF was significantly increased compared with untreated biofilms (p < 0.0001, data not shown). SM_REF biomass was ~5 × 107 BE/mL in the untreated polymicrobial biofilm vs. 3 × 108 BE/mL in the AMB-treated. For such, the results of the two-drug combination were expressed in percentages of growth inhibition and survival compared with AMB-treated biofilm. The qPCR analysis demonstrated that AMB in combination with LVX or RFN significantly improved the antibacterial effect against Sm in polymicrobial biofilm in comparison with LVX or RFN alone (LVX vs. AMB + LVX: p < 0.0001; RFN vs. AMB + RFN: p < 0.0001; Figure 9A). Remarkably, the susceptibility of Sm in polymicrobial biofilm to antifungal-antibacterial combination was not significantly different from the susceptibility of Sm in bacterial biofilm to each antibacterial alone (Sm-LVX vs. Af-Sm-AMB + LVX: p = 0.8668; Sm-RFN vs. Af-Sm-AMB + RFN: p = 0.3963; Figure 9A). AMB + LVX combination was significantly more efficient against Sm in polymicrobial biofilm (90% of Sm growth inhibition) than AMB + RFN (75% of Sm growth inhibition; p = 0.0002). Therefore, survival experiments were performed with AMB in combination with LVX (Figure 9B). A higher reduction of Sm survival in polymicrobial biofilm following AMB + LVX treatment compared with LVX alone (p = 0.0006) was obtained by viable plate count. Moreover, AMB + LVX combination against Sm in polymicrobial biofilm was as efficient as LVX alone against Sm in bacterial biofilm (p = 0.1096; Figure 9B). These results suggest that the inhibition of Af with AMB prompted Sm susceptibility to antibiotics.
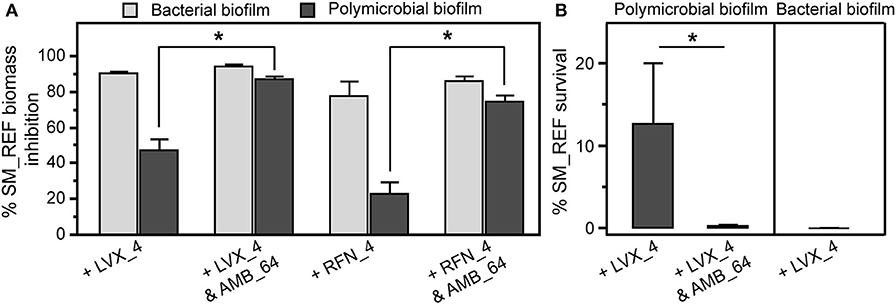
Figure 9. Effects of antifungal-antibacterial combination on A. fumigatus-S. maltophilia biofilm. (A) Susceptibility of SM_REF in bacterial (light gray) and polymicrobial (dark gray) biofilms to LVX and RFN alone or in two-drug combination with AMB assessed by qPCR. (B) Percentages of SM_REF survival following LVX alone or in two-drug combination with AMB treatment in bacterial and polymicrobial biofilms using viable plate count. *p < 0.05; AMB_64, amphotericin B (64 μg/mL); LVX_4, levofloxacin (4 μg/mL); RFN_4, rifampicin (4 μg/mL).
Discussion
Managing cross-kingdoms polymicrobial infections, especially of biofilm-forming microbes, remains an outstanding challenge to overcome resistance to a wide range of clinical antimicrobial agents. Conventional treatment of infectious diseases relies on standard susceptibility testing of planktonic cells, which does not take into account microbial interactions. For such, this approach becomes unsuitable against polymicrobial biofilm with sessile cells embedded in ECM. We previously showed A. fumigatus-S. maltophilia interactions in polymicrobial biofilm (Melloul et al., 2016, 2018), and from there we sought to determine whether the antimicrobial response of both pathogens would be modified in their polymicrobial biofilm.
The present study highlights the modulated antimicrobial response of a filamentous fungal-bacterial biofilm. We showed that A. fumigatus monomicrobial biofilms were susceptible to amphotericin B, but not to itraconazole, and voriconazole. Amphotericin B susceptibility of A. fumigatus increased when it was embedded in polymicrobial biofilm with S. maltophilia. Levofloxacin and rifampicin were efficient in inhibiting S. maltophilia monomicrobial biofilms, but much higher concentrations were needed to eradicate it. S. maltophilia susceptibility to levofloxacin decreased in the polymicrobial biofilm and was fungal biomass-dependent. The inhibited effect of S. maltophilia on A. fumigatus growth was more pronounced for the association of CF strains than the reference strains. The combination of amphotericin B with levofloxacin or rifampicin was efficient to impair both pathogens in the polymicrobial biofilm.
Antifungal susceptibility of A. fumigatus biofilms was assessed by the XTT reduction method, already used several years ago (Mowat et al., 2007; Seidler et al., 2008). We focused on viability test to assess antibacterial susceptibility of S. maltophilia biofilms, which have been used in previous research (Di Bonaventura et al., 2004; Pompilio et al., 2016). The antibiosis effect of S. maltophilia on A. fumigatus growth was revealed by qPCR. This technique was also used to compare the antimicrobial susceptibility of species in mono- and polymicrobial biofilms.
Our results revealed clear differences in antifungal susceptibilities between biofilms in formation and mature biofilms of A. fumigatus (i.e., MBICXTT vs. MBECXTT, Table 1). The formation of A. fumigatus biofilm was prevented by the three antifungal agents (MBICXTTs ≤ 1 μg/mL). A. fumigatus mature biofilm was inhibited by amphotericin B (MBECXTT range = 8–32 μg/mL), but not by the two azoles (MBECXTTs > 256 μg/mL), as already shown in the work of Mowat et al. (2007). These results suggest that azoles could be useful in preventing biofilm formation rather than in treating mature biofilm. To date, the reasons for the decreased susceptibility of A. fumigatus biofilm to drugs have not been fully elucidated (Latgé and Chamilos, 2019).
Considering the results with azoles, we focused on amphotericin B activity on A. fumigatus in polymicrobial biofilms with S. maltophilia and we found an increase susceptibility compared with their corresponding A. fumigatus monomicrobial biofilms (Figure 4). A possible explanation is the modification of fungal phenotype due to the presence of bacteria. In our model, the modification of A. fumigatus cell wall by S. maltophilia (Melloul et al., 2018) resembles the one induced by caspofungin or dirhamnolipids (diRhls) secreted by P. aeruginosa which specifically inhibit the fungal 1,3-glucan synthase activity (Briard et al., 2017). We suppose that some diRhls-like molecules secreted by S. maltophilia would modify A. fumigatus phenotype similarly to echinocandins. This could justify the impairment of A. fumigatus response to amphotericin B, as showed for clinical Aspergillus spp. strains treated with a combination of amphotericin B and echinocandins (Panackal et al., 2014). Iron could be another important parameter in this interaction between S. maltophilia and A. fumigatus. The role of two siderophores secreted by P. aeruginosa (pyoverdine and pyochelin) involved in the reduction of A. fumigatus growth has been analyzed (Sass et al., 2018; Briard et al., 2019) and the production of catecholate siderophores from S. maltophilia clinical strains has been shown (García et al., 2012; Nas and Cianciotto, 2017). In our polymicrobial model, we can hypothesize that siderophores secreted by S. maltophilia could deprive A. fumigatus from iron. In turn, iron deficiency could increase A. fumigatus susceptibility, as reported by Zarember et al. (2009) who showed that iron deprivation gave better A. fumigatus response to amphotericin B treatment.
The viability of antibiotic-treated S. maltophilia in planktonic cultures (MBC), biofilm in formation (MBICCFU), and mature biofilm (MBECCFU) is shown in Table 2. Results showed a reduced levofloxacin susceptibility of biofilm-embedded bacteria. This finding is consistent with the results of previous studies (Passerini de Rossi et al., 2009; Pompilio et al., 2016). The in vitro activity of other fluoroquinolones against biofilm-embedded S. maltophilia cells has already been reported (Di Bonaventura et al., 2004; Passerini de Rossi et al., 2009; Wu et al., 2013; Wang et al., 2016). However, no study analyzed their effect on S. maltophilia in polymicrobial biofilms with a filamentous fungus. Our study demonstrated that S. maltophilia response to levofloxacin was impacted by the presence of A. fumigatus. Manavathu et al. (2014) documented a decrease of bacterial susceptibility in A. fumigatus-P. aeruginosa polymicrobial biofilm with no explanation of the underlying mechanism. Our work demonstrated that levofloxacin effect on S. maltophilia in polymicrobial biofilms, of both associations of strains, decreased and was fungal biomass-dependent. The network of A. fumigatus hyphae could protect S. maltophilia from levofloxacin. The higher fitness of SM_CF, in comparison with SM_REF, could account for the larger antibiosis effect of the CF bacterial strain on the CF fungal growth in polymicrobial biofilm. Alike, in our latest study, we showed that S. maltophilia antibiosis on A. fumigatus was dependent on the bacterial fitness (Melloul et al., 2018). The fungal biomass of AF_CF + SM_CF biofilm was insufficient to protect the bacteria from levofloxacin, but upon increasing the fungal biomass (using a larger initial inoculum), the polymicrobial biofilm provided SM_CF with a better protection from levofloxacin (Figure 6). We further suggested that A. fumigatus ECM could prevent drug diffusion by acting as a physical barrier and enhance S. maltophilia tolerance to levofloxacin. This hypothesis was partially validated using proteinase K pretreatment to damage the ECM structure of the polymicrobial biofilm, similarly to what De Brucker et al. (2015) did for C. albicans and Escherichia coli to obtain a lower bacterial tolerance to ofloxacin. Moreover, matrix components released by A. fumigatus could alter the physiology of S. maltophilia by restricting penetration of nutrients or oxygen into the aggregates. Indeed, Stewart et al. (2015) showed that a low oxygen level seems to be the primary mechanism for tolerance of biofilms to quinolones. Further investigations are warranted to identify the fungal ECM components, which could promote antibacterial protection of S. maltophilia in A. fumigatus-S. maltophilia biofilm.
Some studies put forwards the use of rifampicin to treat S. maltophilia infections (Savini et al., 2010; Betts et al., 2014), but to our knowledge, we are the first to explore its activity against S. maltophilia biofilm. Our experiments needed high doses of rifampicin to eradicate planktonic cells (MBC, Table 2) and mature biofilms (MBECCFU, Table 2) of both S. maltophilia strains. Rifampicin was only efficient to eradicate the SM_REF cells of biofilm in formation (MBICCFU, Table 2).
To date, few studies explored in vitro effects of antifungal agents in combination with antibacterial agents against polymicrobial biofilms. We demonstrated that levofloxacin or rifampicin combined with a high dose of amphotericin B was significantly more efficient to eradicate S. maltophilia in polymicrobial biofilm than the antibiotic alone (Figure 9). This result corroborates the protective effect of A. fumigatus on S. maltophilia in polymicrobial biofilm, since once the fungus was destroyed by amphotericin B, the antibiotic had a stronger effect on the bacteria.
Most CF patients with A. fumigatus infection are put on azole therapies though their efficacy is discussed (Burgel et al., 2016). In the same way, the success of management of S. maltophilia infections in CF patients remains unclear (Amin and Waters, 2016). Finally, treatment failure could be attributed in some cases to the in vivo presence of biofilm forms, as it was proposed for A. fumigatus (Ramage et al., 2011). Our in vitro results demonstrated that the microbial interactions between A. fumigatus and S. maltophilia mutually modulate their responses to antimicrobial agents. This could be particularly relevant in CF patients where lungs can be characterized by decreased oxygen pressure. Also, these findings add a toll on therapeutic decision-making, since the microbial interactions and the biofilm-forming ability are not usually taken into account to design treatment options.
In conclusion, microbial interactions within polymicrobial biofilms can modulate the antimicrobial response of pathogens. In polymicrobial biofilms, S. maltophilia increased the antifungal susceptibility of A. fumigatus to amphotericin B, whereas A. fumigatus protected S. maltophilia from levofloxacin. Further work analyzing the underlying mechanisms of antimicrobial combinations on polymicrobial biofilms would be valuable in the future.
Data Availability Statement
The raw data supporting the conclusions of this article will be made available by the authors, without undue reservation.
Author's Note
This work was presented in part at the 6th European Congress on Biofilms (EUROBIOFILMS) (Glasgow, Scotland, September 2019).
Author Contributions
EM and FB conceived the design of the study. EM supervised the experiments. LR performed the experiments and the statistical analysis. LR and EM designed the figures. LR, EM, and FB participated in results analysis. P-LW and ED helped in the interpretation of antibacterial and antifungal activities, respectively. J-WD and GR analyzed S. maltophilia strains. LR, EM, JG, and FB drafted the manuscript. All authors read and approved the final manuscript.
Funding
This work was supported by a National Research Grant Leg Poix 2017 and by the Pharmaceutical Division of MSD France and Astellas France. The funder bodies were not involved in the study design, collection, analysis, interpretation of data, the writing of this article or the decision to submit it for publication.
Conflict of Interest
During the past 5 years, ED has received research grants from Gilead and MSD; travel grants from Gilead, MSD, Pfizer, and Astellas, and speaker's fee from Gilead, MSD, and Astellas. FB has received research grants from MSD; travel grants from Gilead, MSD, Pfizer, and speaker's fee from Gilead, MSD, and Pfizer.
The remaining authors declare that the research was conducted in the absence of any commercial or financial relationships that could be construed as a potential conflict of interest.
The handling editor declared a past co-authorship with the authors ED and FB.
Acknowledgments
We thank Xavier Decrouy for his work on the microscopy platform. We are also grateful to Suhad Assad for her critical linguistic reviewing and editing.
Abbreviations
Af, A. fumigatus; Sm, S. maltophilia; AMB, amphotericin B; ITC, itraconazole; VRC, voriconazole; LVX, levofloxacin; RFN, rifampicin.
References
Amin, R., and Waters, V. (2016). Antibiotic treatment for stenotrophomonas maltophilia in people with cystic fibrosis. Cochrane Database Syst. Rev. 7:CD009249. doi: 10.1002/14651858.CD009249.pub4
Arendrup, M. C., Meletiadis, J., Mouton, J. W., Lagrou, K., Hamal, P., Guinea, J., et al. (2020). Method for the Determination of Broth Dilution Minimum Inhibitory Concentrations of Antifungal Agents for Conidia Forming Moulds. Available online at: https://www.eucast.org/astoffungi/methodsinantifungalsusceptibilitytesting/ast_of_moulds/ (accessed April 22, 2020).
Bernard, C., Renaudeau, N., Mollichella, M.-L., Quellard, N., Girardot, M., and Imbert, C. (2018). Cutibacterium acnes protects candida albicans from the effect of micafungin in biofilms. Int. J. Antimicrob. Agents 52, 942–946. doi: 10.1016/j.ijantimicag.2018.08.009
Betts, J. W., Phee, L. M., Woodford, N., and Wareham, D. W. (2014). Activity of colistin in combination with tigecycline or rifampicin against multidrug-resistant Stenotrophomonas maltophilia. Eur. J. Clin. Microbiol. Infect. Dis. 33, 1565–1572. doi: 10.1007/s10096-014-2101-3
Bjarnsholt, T., Jensen, P. Ø., Fiandaca, M. J., Pedersen, J., Hansen, C. R., Andersen, C. B., et al. (2009). Pseudomonas aeruginosa biofilms in the respiratory tract of cystic fibrosis patients. Pediatr. Pulmonol. 44, 547–558. doi: 10.1002/ppul.21011
Botterel, F., Angebault, C., Cabaret, O., Stressmann, F. A., Costa, J.-M., Wallet, F., et al. (2018). Fungal and bacterial diversity of airway microbiota in adults with cystic fibrosis: concordance between conventional methods and ultra-deep sequencing, and their practical use in the clinical laboratory. Mycopathologia 183, 171–183. doi: 10.1007/s11046-017-0185-x
Briard, B., Mislin, G. L. A., Latgé, J.-P., and Beauvais, A. (2019). Interactions between aspergillus fumigatus and pulmonary bacteria: current state of the field, new data, and future perspective. J Fungi 5:48. doi: 10.3390/jof5020048
Briard, B., Rasoldier, V., Bomme, P., ElAouad, N., Guerreiro, C., Chassagne, P., et al. (2017). Dirhamnolipids secreted from pseudomonas aeruginosa modify anjpegungal susceptibility of Aspergillus fumigatus by inhibiting β1,3 glucan synthase activity. ISME J. 11, 1578–1591. doi: 10.1038/ismej.2017.32
Bugli, F., Posteraro, B., Papi, M., Torelli, R., Maiorana, A., Paroni Sterbini, F., et al. (2013). In vitro interaction between alginate lyase and amphotericin B against Aspergillus fumigatus biofilm determined by different methods. Antimicrob. Agents Chemother. 57, 1275–1282. doi: 10.1128/AAC.01875-12
Burgel, P.-R., Paugam, A., Hubert, D., and Martin, C. (2016). Aspergillus fumigatus in the cystic fibrosis lung: pros and cons of azole therapy. Infect. Drug Resist. 9, 229–238. doi: 10.2147/IDR.S63621
Cendra, M. D. M., Blanco-Cabra, N., Pedraz, L., and Torrents, E. (2019). Optimal environmental and culture conditions allow the in vitro coexistence of Pseudomonas aeruginosa and Staphylococcus aureus in stable biofilms. Sci. Rep. 9:16284. doi: 10.1038/s41598-019-52726-0
De Brucker, K., Tan, Y., Vints, K., De Cremer, K., Braem, A., Verstraeten, N., et al. (2015). Fungal β-1,3-glucan increases ofloxacin tolerance of Escherichia coli in a polymicrobial E. coli/Candida albicans biofilm. Antimicrob. Agents Chemother. 59, 3052–3058. doi: 10.1128/AAC.04650-14
Desai, J. V., Mitchell, A. P., and Andes, D. R. (2014). Fungal biofilms, drug resistance, and recurrent infection. Cold Spring Harb. Perspect. Med. 4:a019729. doi: 10.1101/cshperspect.a019729
Di Bonaventura, G., Spedicato, I., D'Antonio, D., Robuffo, I., and Piccolomini, R. (2004). Biofilm formation by Stenotrophomonas maltophilia: modulation by quinolones, trimethoprim-sulfamethoxazole, and ceftazidime. Antimicrob. Agents Chemother. 48, 151–160. doi: 10.1128/AAC.48.1.151-160.2004
Esposito, A., Pompilio, A., Bettua, C., Crocetta, V., Giacobazzi, E., Fiscarelli, E., et al. (2017). Evolution of Stenotrophomonas maltophilia in cystic fibrosis lung over chronic infection: a genomic and phenotypic population study. Front. Microbiol. 8:1590. doi: 10.3389/fmicb.2017.01590
Flemming, H.-C., Wingender, J., Szewzyk, U., Steinberg, P., Rice, S. A., and Kjelleberg, S. (2016). Biofilms: an emergent form of bacterial life. Nat. Rev. Microbiol. 14, 563–575. doi: 10.1038/nrmicro.2016.94
Flores-Treviño, S., Bocanegra-Ibarias, P., Camacho-Ortiz, A., Morfín-Otero, R., Salazar-Sesatty, H. A., and Garza-González, E. (2019). Stenotrophomonas maltophilia biofilm: its role in infectious diseases. Expert Rev. Anti. Infect. Ther. 17, 877–893. doi: 10.1080/14787210.2019.1685875
French, C. F register. (2019). Registre Français de la Mucoviscidose – Bilan des Données 2017. Available online at: https://www.vaincrelamuco.org/sites/default/files/rapport_du_registre_-_donnees_2017.pdf (accessed March 15, 2020).
García, C. A., Passerini De Rossi, B., Alcaraz, E., Vay, C., and Franco, M. (2012). Siderophores of Stenotrophomonas maltophilia: detection and determination of their chemical nature. Rev. Argent. Microbiol. 44, 150–154.
Granchelli, A. M., Adler, F. R., Keogh, R. H., Kartsonaki, C., Cox, D. R., and Liou, T. G. (2018). Microbial interactions in the cystic fibrosis airway. J. Clin. Microbiol. 56, e00354–18. doi: 10.1128/JCM.00354-18
Harriott, M. M., and Noverr, M. C. (2009). Candida albicans and Staphylococcus aureus form polymicrobial biofilms: effects on antimicrobial resistance. Antimicrob. Agents Chemother. 53, 3914–3922. doi: 10.1128/AAC.00657-09
Hauben, L., Vauterin, L., Moore, E. R., Hoste, B., and Swings, J. (1999). Genomic diversity of the genus stenotrophomonas. Int. J. Syst. Bacteriol. 49 (Pt. 4), 1749–1760. doi: 10.1099/00207713-49-4-1749
Herrera-Heredia, S. A., Pezina-Cantú, C., Garza-González, E., Bocanegra-Ibarias, P., Mendoza-Olazarán, S., Morfín-Otero, R., et al. (2017). Risk factors and molecular mechanisms associated with trimethoprim-sulfamethoxazole resistance in Stenotrophomonas maltophilia in Mexico. J. Med. Microbiol. 66, 1102–1109. doi: 10.1099/jmm.0.000550
Høiby, N., Bjarnsholt, T., Moser, C., Jensen, P. Ø., Kolpen, M., Qvist, T., et al. (2017). Diagnosis of biofilm infections in cystic fibrosis patients. APMIS 125, 339–343. doi: 10.1111/apm.12689
ISO (2019). Susceptibility Testing of Infectious Agents and Evaluation of Performance of Antimicrobial Susceptibility Test Devices — Part 1: Broth Micro-Dilution Reference Method for Testing the in vitro Activity of Antimicrobial Agents Against Rapidly Growing Aerobic Bacteria Involved in Infectious Diseases. Available online at: https://www.iso.org/cms/render/live/en/sites/isoorg/contents/data/standard/07/04/70464.html (accessed June 2, 2020).
Keays, T., Ferris, W., Vandemheen, K. L., Chan, F., Yeung, S.-W., Mah, T.-F., et al. (2009). A retrospective analysis of biofilm antibiotic susceptibility testing: a better predictor of clinical response in cystic fibrosis exacerbations. J. Cyst. Fibros. 8, 122–127. doi: 10.1016/j.jcf.2008.10.005
King, P., Lomovskaya, O., Griffith, D. C., Burns, J. L., and Dudley, M. N. (2010). In vitro pharmacodynamics of levofloxacin and other aerosolized antibiotics under multiple conditions relevant to chronic pulmonary infection in cystic fibrosis. Antimicrob. Agents Chemother. 54, 143–148. doi: 10.1128/AAC.00248-09
Kong, E. F., Tsui, C., Kucharíková, S., Andes, D., Van Dijck, P., and Jabra-Rizk, M. A. (2016). Commensal protection of Staphylococcus aureus against antimicrobials by Candida albicans biofilm matrix. mBio 7:e01365–16. doi: 10.1128/mBio.01365-16
Kragh, K. N., Alhede, M., Jensen, P. Ø., Moser, C., Scheike, T., Jacobsen, C. S., et al. (2014). Polymorphonuclear leukocytes restrict growth of Pseudomonas aeruginosa in the lungs of cystic fibrosis patients. Infect. Immun. 82, 4477–4486. doi: 10.1128/IAI.01969-14
Latgé, J.-P., and Chamilos, G. (2019). Aspergillus fumigatus and aspergillosis in 2019. Clin. Microbiol. Rev. 33, e00140–18. doi: 10.1128/CMR.00140-18
Lebeaux, D., Ghigo, J.-M., and Beloin, C. (2014). Biofilm-related infections: bridging the gap between clinical management and fundamental aspects of recalcitrance toward antibiotics. Microbiol. Mol. Biol. Rev. 78, 510–543. doi: 10.1128/MMBR.00013-14
Loeffert, S. T., Melloul, E., Dananché, C., Hénaff, L., Bénet, T., Cassier, P., et al. (2017). Monitoring of clinical strains and environmental fungal aerocontamination to prevent invasive aspergillosis infections in hospital during large deconstruction work: a protocol study. BMJ Open 7:e018109. doi: 10.1136/bmjopen-2017-018109
Luo, J., Wang, K., Li, G. S., Lei, D. Q., Huang, Y. J., Li, W. D., et al. (2018). 3,5-Dicaffeoylquinic acid disperses Aspergillus fumigatus biofilm and enhances fungicidal efficacy of voriconazole and amphotericin B. Med. Sci. Monit. 24, 427–437. doi: 10.12659/MSM.908068
Manavathu, E. K., Vager, D. L., and Vazquez, J. A. (2014). Development and antimicrobial susceptibility studies of in vitro monomicrobial and polymicrobial biofilm models with Aspergillus fumigatus and Pseudomonas aeruginosa. BMC Microbiol. 14:53. doi: 10.1186/1471-2180-14-53
Manavathu, E. K., and Vazquez, J. A. (2015). Effects of antimicrobial combinations on Pseudomonas aeruginosa-Aspergillus fumigatus mixed microbial biofilm. J. Microbiol. Exp. 2, 126–136. doi: 10.15406/jmen.2015.02.00057
Melloul, E., Luiggi, S., Anaïs, L., Arné, P., Costa, J.-M., Fihman, V., et al. (2016). Characteristics of Aspergillus fumigatus in association with Stenotrophomonas maltophilia in an in vitro model of mixed biofilm. PLoS ONE 11:e0166325. doi: 10.1371/journal.pone.0166325
Melloul, E., Roisin, L., Durieux, M.-F., Woerther, P.-L., Jenot, D., Risco, V., et al. (2018). Interactions of Aspergillus fumigatus and Stenotrophomonas maltophilia in an in vitro mixed biofilm model: does the strain matter? Front. Microbiol. 9:2850. doi: 10.3389/fmicb.2018.02850
Mercier-Darty, M., Royer, G., Lamy, B., Charron, C., Lemenand, O., Gomart, C., et al. (2020). Comparative whole-genome phylogeny of animal, environmental, and human strains confirms the genogroup organization and diversity of the Stenotrophomonas maltophilia complex. Appl. Environ. Microbiol. 86, e02919–19. doi: 10.1128/AEM.02919-19
Mowat, E., Butcher, J., Lang, S., Williams, C., and Ramage, G. (2007). Development of a simple model for studying the effects of antifungal agents on multicellular communities of Aspergillus fumigatus. J. Med. Microbiol. 56, 1205–1212. doi: 10.1099/jmm.0.47247-0
Nas, M. Y., and Cianciotto, N. P. (2017). Stenotrophomonas maltophilia produces an EntC-dependent catecholate siderophore that is distinct from enterobactin. Microbiology 163, 1590–1603. doi: 10.1099/mic.0.000545
Panackal, A. A., Parisini, E., and Proschan, M. (2014). Salvage combination antifungal therapy for acute invasive aspergillosis may improve outcomes: a systematic review and meta-analysis. Int. J. Infect. Dis. 28, 80–94. doi: 10.1016/j.ijid.2014.07.007
Passerini de Rossi, B., García, C., Calenda, M., Vay, C., and Franco, M. (2009). Activity of levofloxacin and ciprofloxacin on biofilms and planktonic cells of Stenotrophomonas maltophilia isolates from patients with device-associated infections. Int. J. Antimicrob. Agents 34, 260–264. doi: 10.1016/j.ijantimicag.2009.02.022
Pierce, C. G., Uppuluri, P., Tristan, A. R., Wormley, F. L., Mowat, E., Ramage, G., et al. (2008). A simple and reproducible 96-well plate-based method for the formation of fungal biofilms and its application to antifungal susceptibility testing. Nat. Protoc. 3, 1494–1500. doi: 10.1038/nprot.2008.141
Pompilio, A., Crocetta, V., De Nicola, S., Verginelli, F., Fiscarelli, E., and Di Bonaventura, G. (2015). Cooperative pathogenicity in cystic fibrosis: Stenotrophomonas maltophilia modulates Pseudomonas aeruginosa virulence in mixed biofilm. Front. Microbiol. 6:951. doi: 10.3389/fmicb.2015.00951
Pompilio, A., Crocetta, V., Verginelli, F., and Di Bonaventura, G. (2016). In vitro activity of levofloxacin against planktonic and biofilm Stenotrophomonas maltophilia lifestyles under conditions relevant to pulmonary infection in cystic fibrosis, and relationship with SmeDEF multidrug efflux pump expression. FEMS Microbiol. Lett. 363:fnw145. doi: 10.1093/femsle/fnw145
Pompilio, A., Savini, V., Fiscarelli, E., Gherardi, G., and Di Bonaventura, G. (2020). Clonal diversity, biofilm formation, and antimicrobial resistance among Stenotrophomonas maltophilia strains from cystic fibrosis and non-cystic fibrosis patients. Antibiotics 9:15. doi: 10.3390/antibiotics9010015
Ramage, G., Rajendran, R., Gutierrez-Correa, M., Jones, B., and Williams, C. (2011). Aspergillus biofilms: clinical and industrial significance. FEMS Microbiol. Lett. 324, 89–97. doi: 10.1111/j.1574-6968.2011.02381.x
Rodríguez-Sevilla, G., Crabbé, A., García-Coca, M., Aguilera-Correa, J. J., Esteban, J., and Pérez-Jorge, C. (2019). Antimicrobial treatment provides a competitive advantage to Mycobacterium abscessus in a dual-species biofilm with Pseudomonas aeruginosa. Antimicrob. Agents Chemother. 63, e01547–19. doi: 10.1128/AAC.01547-19
Rogiers, O., Holtappels, M., Siala, W., Lamkanfi, M., Van Bambeke, F., Lagrou, K., et al. (2018). Anidulafungin increases the antibacterial activity of tigecycline in polymicrobial Candida albicans/Staphylococcus aureus biofilms on intraperitoneally implanted foreign bodies. J. Antimicrob. Chemother. 73, 2806–2814. doi: 10.1093/jac/dky246
Sass, G., Nazik, H., Penner, J., Shah, H., Ansari, S. R., Clemons, K. V., et al. (2018). Studies of Pseudomonas aeruginosa mutants indicate pyoverdine as the central factor in inhibition of Aspergillus fumigatus biofilm. J. Bacteriol. 200, e00345–17. doi: 10.1128/JB.00345-17
Savini, V., Catavitello, C., D'Aloisio, M., Balbinot, A., Astolfi, D., Masciarelli, G., et al. (2010). Chloramphenicol and rifampin may be the only options against Stenotrophomonas maltophilia. A tale of a colonized bladder device in a patient with myelofibrosis. Infez. Med. 18, 193–197.
Seidler, M. J., Salvenmoser, S., and Müller, F.-M. C. (2008). Aspergillus fumigatus forms biofilms with reduced antifungal drug susceptibility on bronchial epithelial cells. Antimicrob. Agents Chemother. 52, 4130–4136. doi: 10.1128/AAC.00234-08
Stewart, P. S., Franklin, M. J., Williamson, K. S., Folsom, J. P., Boegli, L., and James, G. A. (2015). Contribution of stress responses to antibiotic tolerance in Pseudomonas aeruginosa biofilms. Antimicrob. Agents Chemother. 59, 3838–3847. doi: 10.1128/AAC.00433-15
Tang, H.-J., Chen, C.-C., Cheng, K.-C., Wu, K.-Y., Lin, Y.-C., Zhang, C.-C., et al. (2013). In vitro efficacies and resistance profiles of rifampin-based combination regimens for biofilm-embedded methicillin-resistant Staphylococcus aureus. Antimicrob. Agents Chemother. 57, 5717–5720. doi: 10.1128/AAC.01236-13
Vinuesa, P., Ochoa-Sánchez, L. E., and Contreras-Moreira, B. (2018). GET_PHYLOMARKERS, a software package to select optimal orthologous clusters for phylogenomics and inferring pan-genome phylogenies, used for a critical geno-taxonomic revision of the genus stenotrophomonas. Front. Microbiol. 9:771. doi: 10.3389/fmicb.2018.00771
Wang, A., Wang, Q., Kudinha, T., Xiao, S., and Zhuo, C. (2016). Effects of fluoroquinolones and azithromycin on biofilm formation of Stenotrophomonas maltophilia. Sci. Rep. 6:29701. doi: 10.1038/srep29701
Wang, C. H., Yu, C.-M., Hsu, S.-T., and Wu, R.-X. (2020). Levofloxacin-resistant Stenotrophomonas maltophilia: risk factors and antibiotic susceptibility patterns in hospitalized patients. J. Hosp. Infect. 104, 46–52. doi: 10.1016/j.jhin.2019.09.001
Wasylnka, J. A., and Moore, M. M. (2002). Uptake of Aspergillus fumigatus conidia by phagocytic and nonphagocytic cells in vitro: quantitation using strains expressing green fluorescent protein. Infect. Immuni. 70, 3156–3163. doi: 10.1128/IAI.70.6.3156-3163.2002
Wu, K., Yau, Y. C. W., Matukas, L., and Waters, V. (2013). Biofilm compared to conventional antimicrobial susceptibility of Stenotrophomonas maltophilia isolates from cystic fibrosis patients. Antimicrob. Agents Chemother. 57, 1546–1548. doi: 10.1128/AAC.02215-12
Yan, Q., Karau, M. J., Raval, Y. S., and Patel, R. (2018). Evaluation of oritavancin combinations with rifampin, gentamicin, or linezolid against prosthetic joint infection-associated methicillin-resistant Staphylococcus aureus biofilms by time-kill assays. Antimicrob. Agents Chemother. 62, e00943–18. doi: 10.1016/j.ijantimicag.2018.07.012
Keywords: Aspergillus fumigatus, Stenotrophomonas maltophilia, polymicrobial biofilm, antimicrobial susceptibility, antifungal agent, antibacterial agent
Citation: Roisin L, Melloul E, Woerther P-L, Royer G, Decousser J-W, Guillot J, Dannaoui E and Botterel F (2020) Modulated Response of Aspergillus fumigatus and Stenotrophomonas maltophilia to Antimicrobial Agents in Polymicrobial Biofilm. Front. Cell. Infect. Microbiol. 10:574028. doi: 10.3389/fcimb.2020.574028
Received: 18 June 2020; Accepted: 07 September 2020;
Published: 06 October 2020.
Edited by:
Jean-Philippe Bouchara, Université d'Angers, FranceReviewed by:
Vishukumar Aimanianda, Institut Pasteur, FranceAlexandre Alanio, Université Paris Diderot, France
Copyright © 2020 Roisin, Melloul, Woerther, Royer, Decousser, Guillot, Dannaoui and Botterel. This is an open-access article distributed under the terms of the Creative Commons Attribution License (CC BY). The use, distribution or reproduction in other forums is permitted, provided the original author(s) and the copyright owner(s) are credited and that the original publication in this journal is cited, in accordance with accepted academic practice. No use, distribution or reproduction is permitted which does not comply with these terms.
*Correspondence: Françoise Botterel, francoise.botterel@aphp.fr