- 1Department of Chemistry, Science and Research Branch, Islamic Azad University, Tehran, Iran
- 2Chemistry and Chemical Engineering Research Centre of Iran, Tehran, Iran
- 3Department of Science, Payame Noor University (PNU), Tehran, Iran
Deep eutectic solvent (DES) has been considered as a useful catalyst and reaction medium for various organic transformations. Herein, we report the catalytic application of novel deep eutectic solvent- based surfactant (DES surfactant) for the selective and fast oxidation of alcohols to aldehydes. The readily accessible DES surfactants (FeCl3/BHDC) was prepared using inexpensive ferric chloride (FeCl3) and benzyl hexadecyl dimethyl ammonium chloride in a simple manner. The synthesized FeCl3/BHDC was characterized using various techniques, including, FTIR spectroscopy, thermal gravimetric analysis (TGA), scanning electron microscopy (SEM), and energy- dispersive X-ray spectroscopy (EDS) to determine its structure. The catalytic activity of FeCl3/BHDC in the selective oxidation of various alcohols to corresponding aldehyde derivative was investigated. The results showed the reaction could be completed within very short reaction times ranging from 2 to 15 min, while achieving good to excellent yields. This protocol offers a facile strategy and excellent efficiency in selectively oxidizing various alcohol derivatives to their respective aldehydes and ketones, utilizing hydrogen peroxide in the presence of catalytic DES surfactant.
Introduction
Carbonyl compounds, including aldehydes and ketones, are essential in various chemical production processes in both laboratory and industrial settings (Abad et al., 2005). They serve as versatile intermediates for synthesizing a wide range of compounds with commercial applications (Gröbel and Seebach, 1977). Aldehydes, in particular, are valuable precursors for many organic transformations and find extensive use in the synthesis of agricultural and medicinal compounds (Michael and March 2007). They play a crucial role in the production of consumable goods such as perfumes, beverages, and medicinal intermediates (Patai’s Chemistry of Functional Groups, 1966). Benzaldehyde, an aromatic aldehyde, holds significant importance due to its versatile applications in various industries. Benzaldehyde serves as a primary ingredient in the production of perfumes, where its distinct almond-like fragrance is highly valued. It is also utilized in the flavor industry to provide almond or cherry-like flavors to food and beverages. Additionally, benzaldehyde acts as a precursor in the synthesis of numerous pharmaceuticals and agrochemicals, contributing to the development of agricultural and medicinal compounds (Aljaafari et al., 2022). Historically, benzaldehydes were produced through the hydrolysis of benzyl chloride or the oxidation of toluene, which had drawbacks such as the generation of chlorinated by-products and hazardous acidic compounds, as well as harsh reaction conditions and poor selectivity (Artzi et al., 2009).
In recent years, the industrial synthesis of benzaldehydes by the oxidation of benzyl alcohol has gained popularity due to its advantages of easily controllable conditions and high yield (Corey and Suggs, 1975). However, the oxidation of alcohols typically involves the use of expensive oxidizing agents like dichromate, chromic acid, or permanganate in stoichiometric quantities (Shaabani et al., 2021). These oxidants, such as potassium permanganate (KMnO4) and potassium dichromate (K2Cr2O7), possess strong oxidizing properties but also come with environmental challenges and high costs (Lou and Xu, 2002; Xu et al., 2020). To address these concerns, a potential alternative is the selective oxidation of alcohols using pure oxygen (O2) and hydrogen peroxide (H2O2) as the oxidants (Nagy et al., 2020). This substitution aims to minimize the environmental impact associated with the use of inorganic oxidants like KMnO4 and K2Cr2O7. Pure oxygen is readily available in the atmosphere, and hydrogen peroxide can be produced from sustainable sources or synthesized using greener methods (Nagy et al., 2020). By utilizing pure oxygen and hydrogen peroxide, the oxidation of alcohols can be performed under environmentally friendly reaction conditions, leading to improved selectivity and reduced formation of unwanted by-products (Crombie et al., 2021). Moreover, this substitution has the potential to be more cost-effective in the long run, considering the environmental and health-related costs associated with traditional inorganic oxidants (Su et al., 2010).
In recent years, there has been growing interest in exploring alternative and sustainable approaches to alcohol oxidation, including the use of catalytic system, activated surfaces (Azizi et al., 2014; Zhang et al., 2014; Liu et al., 2018; Zauche and Espenson, 1998; Li et al., 2020) and ionic liquids (Fall et al., 2010; Dai et al., 2017; Liang et al., 2010). One promising avenue is the utilization of functionalized surfaces with catalytic properties, which aligns with the principles of green chemistry (Yang et al., 2013). These surfaces can provide an alternative to traditional catalysts and offer advantages such as improved selectivity, reduced waste generation, and the potential for recyclability. Additionally, green oxidation of alcohols to aldehydes involves the use of environmentally friendly methods that minimize the use of hazardous reagents and generate less waste (Nørskov et al., 2009; Zhang et al., 2018; Tan et al., 2024; Wei et al., 2019; Wang Y. et al., 2020). These methods include aerobic oxidation using molecular oxygen as the oxidizing agent in the presence of catalysts, biocatalysis using enzymes to selectively oxidize alcohols, oxidation with hydrogen peroxide as a non-toxic oxidant, and the use of supported metal catalysts for efficient and recyclable oxidation reactions (Wei et al., 2022; Wang J. et al., 2020; Li et al., 2022; Wang F. et al., 2024).
DES are novel environmentally friendly solvents that have gained noteworthy attention in recent years due to their various applications in chemistry, materials science, and biotechnology (Zhang et al., 2012). DES are formed by the mixing of two or more components, which undergo a eutectic reaction to form a liquid phase at relatively low temperatures (Dai et al., 2013). DES offer several advantageous properties that make them appealing as solvents and reaction media in various applications (Francisco et al., 2013). These include their low volatility, resulting in reduced release of volatile organic compounds and improved safety during handling; low toxicity, making them environmentally friendly alternatives to conventional solvents; high thermal stability, enabling their use at elevated temperatures without decomposition; wide liquid range, providing flexibility in their application across different temperature ranges; and versatility, as DES can be tailored by selecting different combinations of starting materials, allowing for a wide range of solvents with tunable properties (Wagle et al., 2013; Tang and Row, 2013; Mbous et al., 2017; Abo-Hamad et al., 2015; Liu et al., 2015). These characteristics make DES promising candidates for sustainable and efficient solvent systems in fields such as chemistry, materials science, and biotechnology (Li J. et al., 2023; Procopio and Ramón, 2024; Paparella et al., 2024; Li X. et al., 2023; Cicco et al., 2022). Furthermore, the versatility, tunability, and environmentally friendly nature of metal salts based DES make them valuable solvents and catalyst with diverse applications across multiple fields (Latos et al., 2024; Zhang et al., 2019; Rodríguez-Álvarez et al., 2023; Matuszek et al., 2016; Estager et al., 2014).
Surfactants, on the other hand, are amphiphilic molecules that contain both hydrophilic and hydrophobic sites. They are widely used in various industries and applications, including emulsification, detergency, and solubility. The combination of deep eutectic solvents and surfactants has led to the development of deep eutectic solvent surfactants (Basu et al., 2023). DES surfactants are surfactants that incorporate DES as part of their molecular structure or as the solvent medium in which they are dispersed (Lakshmipraba et al., 2022). DES surfactants offer several advantages compared to conventional surfactants. Firstly, the use of DESs as the solvent medium provides enhanced solubility and stability for the surfactant molecules. This leads to improved performance in applications such as emulsification, dispersion, and solubility. Secondly, DES surfactants exhibit unique properties based on the specific combination of the DES and surfactant components (Manasi et al., 2023). For example, the choice of HBA and HBD in the deep eutectic solvent can influence the polarity and hydrophilic-lipophilic balance of the DES surfactants. This allows for the tailoring of surfactant properties to meet specific application requirements (Munive-Olarte et al., 2022).Additionally, they provide a greener alternative to conventional surfactants. The use of DESs as the solvent medium reduces the reliance on volatile organic compounds and toxic solvents, contributing to improved environmental sustainability (Sheikh Asadi et al., 2024).
As part of our ongoing research on developing efficient homogeneous catalytic systems (Seyyed Shahabi et al., 2020; Azizi and Edrisi, 2017; Mirmashhori et al., 2006) and enhancing the properties of catalytic frameworks using DESs for various transformations, we have developed a new DES surfactant that was formed by chemically combining FeCl3 with BHDC (Figure 1). In addition, their catalytic efficiency for selective oxidation of alcohols to their corresponding carbonyl compounds was also investigated.
Experimental section
Materials and methods
All chemicals used in the experiments were commercially available and were obtained from chemical supplier without further purification. Thin-layer chromatography (TLC) was employed to monitor the reactions under UV light, utilizing Merck 60 HF254 silica plates. The melting points were determined using Büchi 535 melting point apparatus. SEM images were captured using a ZEISS scanning electron microscope. TGA was conducted on an STA-1500 instrument at a heating rate of 10°C/min in air atmosphere. The FT-IR spectra was recorded using a Bomem MB-Series FT-IR spectrometer.
Preparation of DES
FeCl3.6H2O (200 mmol) and BHDC (100 mmol) were mixed together in a round-bottom flask. The resulting mixture was then heated at 60 °C for a 20 min. After the heating process, a dark brown liquid was obtained without undergoing any further purification steps (Figure 2).
General procedure
An alcohol (1.00 mmol) and FeCl3/BHDC (10 mg) were mixed in a test tube. Subsequently, H2O2 32% (3.00 mmol) was added dropwise to the mixture. The reaction was observed to be exothermic and completed within 2–15 min at room temperature, as visually monitored. After the reaction was completed, water (2 mL) and ethyl acetate (2 mL) was added, and the ethyl acetate layer was separated and rapidly purified with silica gel flash chromatography to get the desired aldehyde product. The conversion and selectivity of the reaction were determined by GC analysis. All compounds were known and were characterized by melting and boiling points found to be identical with the ones described in the literature.
Results and discussion
The FeCl3/BHDC was prepared using a simple and straightforward method, as depicted in Figure 2. The prepared FeCl3/BHDC was subsequently subjected to characterization using various spectroscopic techniques. The spectroscopic analysis provides valuable information about the composition, bonding, and functional groups present in the FeCl3/BHDC, enabling a comprehensive understanding of its molecular structure and properties.
The FT-IR spectroscopy analysis of FeCl3/BHDC, FeCl3.6H2O and BHDC were depicted in Figure 3 showed characteristic peaks and changes in specific frequency regions. In the FT-IR spectrum of FeCl3/BHDC, a broad peak was observed in the range of 3432–3352 cm−1, which can be attributed to the presence of water in FeCl3.6H2O.The strong bands in the range of 2,852–2,922 cm−1 correspond to the C-H stretching vibrations of the CH2 group. In the lower frequency region, two peaks were observed around 1,473 cm−1, corresponding to stretching vibrations of benzene rings, and a peak at 1,349 cm−1 was observed, which corresponds to the stretching vibrations of the N–CH2 further confirming the presence of alkyl chains in BHDC. In the region of 609 cm−1 and 562 cm−1, peaks were observed that can be attributed to the bending vibrations of Fe-Cl bonds. The main changes were observed in the 350–700 cm−1 region, which is associated with the interaction between FeCl3 and BHDC. These peaks in this region can indicate the formation of coordination complexes in the molecular structure.
Figure 4 showcases the SEM images of the FeCl3/BHDC. The images reveal that the structure of the FeCl3/BHDC exhibits a plate-like morphology. This observation suggests that the FeCl3/BHDC possesses a layered or sheet-like structure, with distinct flat or plate-like particles. The chemical composition of the FeCl3/BHDC was analyzed using EDS, and the corresponding results are presented in Figure 4B. The EDS analysis revealed the presence of carbon, iron, chlorine, and nitrogen in the structure of the FeCl3/BHDC. These findings provide additional confirmation of the successful reaction between FeCl3 and BHDC, as the detected elements correspond to the components used in the synthesis of the FeCl3/BHDC. In addition to the EDS analysis, EDS mapping was also performed to investigate the elemental distribution within the FeCl3/BHDC (Figure 4C). The results of the EDS mapping analysis demonstrated a uniform distribution of elements throughout the DES structure. This means that the elements present in the DESS, such as carbon (C), iron (Fe), chlorine (Cl), and nitrogen (N), were evenly dispersed and distributed across the entire sample.
The thermal stability of FeCl3/BHDC was investigated using thermogravimetric analysis under air conditions. The results of the TGA analysis are depicted in Figure 5, which reveals four distinct mass losses occurring at different temperature ranges. The first mass loss occurs below 120 °C and is attributed to the evaporation of water molecules weakly bound to FeCl3.6H2O through hydration. The second mass loss takes place in the temperature range of 120°C–180°C corresponds to the loss of water molecules that are strongly linked (dehydrated water). The third step, observed between 250°C and 350°C, corresponds to the decomposition of BHDC. Finally, the fourth mass loss occurs between 350°C and 500°C and corresponds to the complete decomposition of anhydrous ferric chloride with the detachment of HCl (g) and Cl2 (g) gases. This transformation leads to the formation of iron oxide. From the TGA analysis presented in Figure 5, it is evident that the synthesized FeCl3/BHDC exhibits higher thermal stability compared to the BHDC surfactant (Müller et al., 2014; Ahmad Wagay et al., 2020).
After preparation and characterization of FeCl3/BHDC, their catalytic activity in the oxidation process of benzyl alcohol was evaluated. The efficiency of the catalyst, as well as the influence of the oxidant, and reaction time, were optimized. Table 1 presents the results of the reaction conducted with varying amounts of FeCl3/BHDC catalyst (5 mg, 7 mg, 10 mg, 15, and 20 mg). Based on the results presented in Table 1, it was determined that the optimal amount of FeCl3/BHDC catalyst for the alcohol oxidation process was 10 mg as it gives higher yield (98%) of the desired product and shorter reaction time compared to other catalyst loadings tested.
Furthermore, as shown in Table 2, the catalytic activity of FeCl3/BHDC was compared with FeCl3 (Entry 7) and BHDC (Entry 8) alone to evaluate the efficiency of the desired FeCl3/BHDC in the oxidation process. It was found that the combined FeCl3/BHDC gives the highest value for yield (98%). Next, different quantities of the oxidant were tested. Based on the results, it was determined that 3 mmol of H2O2 was the optimal quantity of oxidant for the oxidation process. The effect of different solvents on the reaction yields was also investigated (Table 2, entries 10–13). The tested solvents were water, acetonitrile, ethyl acetate, and methanol. After conducting these studies, it was determined that the solvent-free condition was the most favorable condition for the reaction. Based on the overall analysis, it was concluded that the most favorable conditions for the model reaction were achieved by using 10 mg of FeCl3/BHDC catalyst at room temperature, in the presence of 3 mmol of H2O2 as the oxidant, and under solvent-free conditions. Based on entry 9, the reaction without catalyst and solvent was failed.
Table 3 demonstrates the results of the oxidation of various alcohols to their corresponding carbonyl compounds under the optimized conditions. The findings indicate that all the products were obtained in high yields, justifying the effectiveness of the catalytic system. Table 3 displays using a range of benzylic alcohol derivatives, including primary and secondary benzylic alcohols, which were subjected to oxidation. Secondary benzylic alcohols exhibited higher reactivity in this catalytic system, as evidenced by shorter reaction times and higher yields compared to other compounds. The reactivity of primary benzylic alcohols was influenced by the nature of the substituent group; Primary benzylic alcohols containing an electron-withdrawing group demonstrated superior performance, achieving higher yields compared to those containing an electron-donating group.
In comparison with other oxidation systems reported in the literature, our proposed method is relatively more facile and favorable having shorter reaction time and higher yield (Table 4).
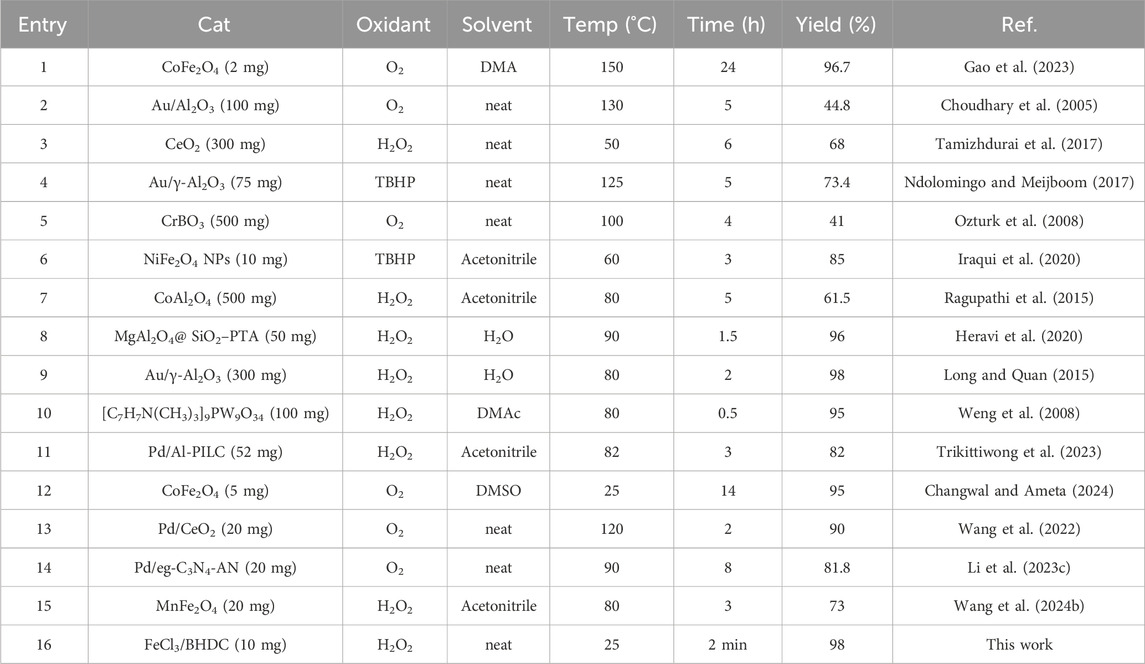
Table 4. Comparison of the performance of various catalyst systems and methods in the synthesis of benzyl alcohol.
To investigate the formation of hydroxyl radicals during the reaction process, a verification experiment was conducted using salicylic acid as a hydroxyl radical trap. The results, shown in Figure 6, revealed a distinct adsorption peak in the UV-vis spectra at approximately 290–590 nm, with a maximum absorption at 470 nm. This peak corresponds to the characteristic absorption of adducts formed between hydroxyl radicals and salicylic acid. These findings strongly indicate the presence of hydroxyl radicals during the oxidation, confirming their involvement in the oxidation of alcohols to aldehydes (Chen et al., 2023; Li W. et al., 2023).
The proposed reaction mechanism for the oxidation of alcohols in the presence of DES is depicted in Figure 7. DES plays two main roles in this system. Firstly, due to the water insolubility of benzyl alcohols and the surface activity of DES, it enables successful biphasic catalysis between the aqueous and alcohol phases for the oxidation reaction. Secondly, iron salts in DES acts as a catalyst on the interphase by coordinating with both the alcohols and H2O2 to form an iron complex. The coordinated intermediate then undergoes a reaction with the alcohol, leading to the oxidation of the alcohol and the formation of the aldehyde product.
The recycling potential of the catalyst is crucial for industrial applications as it can significantly reduce costs and waste generation. The stability and reusability of the DES catalyst were investigated, and the results are presented in Figure 8. The model reaction benzyl alcohols in the presence of FeCl3/BHDC and H2O2 as the catalyst system was employed. After the completion of the reaction, water (2 mL) and ethyl acetate (2 mL) were added, and two phases were separated. The water phase, containing the DES catalyst, was then subjected to evaporation under reduced pressure. The remaining DES was retained and utilized for subsequent consecutive runs. The results indicated that the DES catalyst system exhibited reusability for up to four consecutive runs without any significant decrease in yields.
Conclusion
In our study, we have successfully synthesized and characterized deep eutectic solvent surfactants (FeCl3/BHDC) using easily accessible starting materials. This synthetic method offers simplicity and accessibility, making it practical for large-scale production. The applications of FeCl3/BHDC was investigated as a catalyst for selective oxidation reactions using aqueous hydrogen peroxide. FeCl3/BHDC exhibited excellent catalytic performance, enabling the conversion of various primary and secondary alcohol derivatives into their corresponding aldehydes and ketones with higher yields and shorter reaction time. These approaches contribute to sustainable and greener chemical synthesis by reducing environmental impacts and promoting the use of renewable and non-toxic reagents.
Data availability statement
The original contributions presented in the study are included in the article/Supplementary Material, further inquiries can be directed to the corresponding author.
Author contributions
BC: Methodology, Investigation, Data curation, Writing–original draft. NA: Conceptualization, Methodology, Writing–review and editing. ZM: Resources, Investigation, Supervision, Writing–original draft. HS: Formal analysis, Methodology, Validation, Software, Writing–review and editing.
Funding
The author(s) declare that financial support was received for the research, authorship, and/or publication of this article.
Acknowledgments
This work is based upon research funded by Iran National Science Foundation (INSF) under project No.4013509.
Conflict of interest
The authors declare that the research was conducted in the absence of any commercial or financial relationships that could be construed as a potential conflict of interest.
Publisher’s note
All claims expressed in this article are solely those of the authors and do not necessarily represent those of their affiliated organizations, or those of the publisher, the editors and the reviewers. Any product that may be evaluated in this article, or claim that may be made by its manufacturer, is not guaranteed or endorsed by the publisher.
Supplementary material
The Supplementary Material for this article can be found online at: https://www.frontiersin.org/articles/10.3389/fchem.2024.1416825/full#supplementary-material
References
Abad, A., Concepción, P., Corma, A., and García, H. (2005). A collaborative effect between gold and a support induces the selective oxidation of alcohols. Angew. Chem. Int. Ed. 44 (26), 4066–4069. doi:10.1002/anie.200500382
Abo-Hamad, A., Hayyan, M., AlSaadi, M. A., and Hashim, M. A. (2015). Potential applications of deep eutectic solvents in nanotechnology. J. Chem. Eng. 273, 551–567. doi:10.1016/j.cej.2015.03.091
Ahmad Wagay, T., Askari, H., and Ismail, K. (2020). Synthesis, aggregation and adsorption behavior of benzyldimethylhexadecylammonium based double-chained metallosurfactants. J. Mol. Liq. 299, 112234. doi:10.1016/j.molliq.2019.112234
Aljaafari, N., Abdulraheem Alkhoori, M., Hag-Ali, M., Cheng, W. H., Lim, S. H., Loh, J. Y., et al. (2022). Contribution of aldehydes and their derivatives to antimicrobial and immunomodulatory activities. Molecules 27, 3589–3599. doi:10.3390/molecules27113589
Artzi, N., Shazly, T., Baker, A. B., and Edelman, E. R. (2009). Aldehyde-amine chemistry enables modulated biosealants with tissue-specific adhesion. Adv. Mater. 4 (21), 3399–3403. doi:10.1002/adma.200900340
Azizi, N., and Edrisi, M. (2017). Multicomponent reaction in deep eutectic solvent for synthesis of substituted 1-Aminoalkyl-2-naphthols. Rev. Chem. Intermed. 43 (1), 379–385. doi:10.1007/s11164-016-2628-2
Azizi, N., Khajeh, M., and Alipour, M. (2014). Rapid and selective oxidation of alcohols in deep eutectic solvent. Ind. Eng. Chem. Res. 53 (40), 15561–15565. doi:10.1021/ie502019z
Basu, M., Hassan, P. A., and Shelar, S. B. (2023). Modulation of surfactant self-assembly in deep eutectic solvents and its relevance to drug delivery-A review. J. Mol. Liq. 375, 121301. doi:10.1016/j.molliq.2023.121301
Changwal, R., and Ameta, S. C. (2024). Oxidation of benzyl alcohols by molecular oxygen catalyzed by cobalt ferrite. Res. J. Chem. Environ. 28 (1), 91–97. doi:10.25303/281rjce91097
Chen, Y., Chen, C., Liu, Y., and Yu, L. (2023). Probing the effect of nitrate anion in CAN: an additional opportunity to reduce the catalyst loading for aerobic oxidations. Chin. Chem. Lett. 34, 108489. doi:10.1016/j.cclet.2023.108489
Choudhary, V. R., Dhar, A., Jana, P., Jha, R., and Uphade, B. S. (2005). A green process for chlorine-free benzaldehyde from the solvent-free oxidation of benzyl alcohol with molecular oxygen over a supported nano-size gold catalyst. Green Chem. 7, 768–770. doi:10.1039/b509003b
Cicco, L., Roggio, M., López-Aguilar, M., Vitale, P., Capriati, V., García-Álvarez, J., et al. (2022). Selective aerobic oxidation of alcohols in low melting mixtures and water and use for telescoped one-pot hybrid reactions. Chem. Open 11 (10), e202200160. doi:10.1002/open.202200160
Corey, E. J., and Suggs, J. W. (1975). Pyridinium chlorochromate. An efficient reagent for oxidation of primary and secondary alcohols to carbonyl compounds. Tetrahedron Lett. 16 (31), 2647–2650. doi:10.1016/s0040-4039(00)75204-x
Crombie, C. M., Lewis, R. J., Taylor, R. L., Morgan, D. j., Davies, T. E., Folli, A., et al. (2021). Enhanced selective oxidation of benzyl alcohol via in situ H2O2 production over supported Pd-based catalysts. ACS Catal. 11, 2701–2714. doi:10.1021/acscatal.0c04586
Dai, C., Zhang, J., Huang, C., and Lei, Z. (2017). Ionic liquids in selective oxidation: catalysts and solvents. Chem. Rev. 117, 6929–6983. doi:10.1021/acs.chemrev.7b00030
Dai, Y., van Spronsen, J., Witkamp, G. J., Verpoorte, R., and Choi, Y. H. (2013). Natural deep eutectic solvents as new potential media for green Technology. Anal. Chi. Acta. 766, 61–68. doi:10.1016/j.aca.2012.12.019
Estager, J., Holbrey, J. D., and Swadźba-Kwaśny, M. (2014). Halometallate ionic liquids-revisited. Chem. Soc. Rev. 43, 847–886. doi:10.1039/c3cs60310e
Fall, A., Sene, M., Gaye, M., Gomez, G., and Fall, Y. (2010). Ionic liquid-supported TEMPO as catalyst in the oxidation of alcohols to aldehydes and ketones. Tetrahedron Lett. 51, 4501–4504. doi:10.1016/j.tetlet.2010.06.086
Francisco, M., Van Den Bruinhorst, A., and Kroon, M. C. (2013). Low-transition-temperature mixtures (LTTMs): a new generation of designer solvents. Angew. Chem. Int. Ed. 52 (11), 3074–3085. doi:10.1002/anie.201207548
Gao, G., Rong, R., Zhang, Z., Pan, B., Sun, X., Zhang, Q., et al. (2023). Selective oxidation of benzyl alcohol to benzaldehyde over CoFe2O4 nanocatalyst. Catal. Commun. 183, 106757. doi:10.1016/j.catcom.2023.106757
Gröbel, B.-T., and Seebach, D. (1977). Umpolung of the reactivity of carbonyl compounds through sulfur-containing reagents. Synthesis 1977 (6), 357–402. doi:10.1055/s-1977-24412
Heravi, M., Ghalavand, N., and Hashemi, E. (2020). Hydrogen peroxide as a green oxidant for the selective catalytic oxidation of benzylic and heterocyclic alcohols in different media: an overview. Chemistry 2, 101–178. doi:10.3390/chemistry2010010
Iraqui, S., Kashyap, S., and Rashid, Md. H. (2020). NiFe2O4 nanoparticles: an efficient and reusable catalyst for the selective oxidation of benzyl alcohol to benzaldehyde under mild conditions. Nanoscale Adv. 429, 5790–5802. doi:10.1039/d0na00591f
Lakshmipraba, J., and Prabhu, R. N. (2022). “Deep eutectic solvents, bio-based solvents, and surfactant for green sample pretreatment and determination,” in Green chemical analysis and sample preparations. Editors M. H. El-Maghrabey, V. Sivasankar, and R. N. El-Shaheny (London, United Kingdom: Springer Nature), 353–378.
Latos, P., Gabzdyl, J., Jurczyk, S., and Chrobok, A. (2024). Synergistic effect of deep eutectic solvent and zinc oxide in transesterification of dimethyl terephthalate for the synthesis of alkyl terephthalate esters. Ind. Eng. Chem. Res. 63 (18), 8102–8110. doi:10.1021/acs.iecr.4c00152
Li, C., Feng, M., Cui, L., Wang, F., Xue, B., and Xu, J. (2023c). Solvent-free selective oxidation of benzyl alcohol at atmospheric pressure catalyzed by Pd nanoparticles supported on g-C3N4 materials with tunable nitrogen distributions. ChemCatChem 15 (Issue 20). doi:10.1002/cctc.202300794
Li, J., Wang, X., Bai, R., and Gu, Y. (2023a). Versatile application of strong brønsted acid deep eutectic solvent as recyclable reaction media and catalysts in organic synthesis. J. Mol. Liq. 385, 121876. doi:10.1016/j.molliq.2023.121876
Li, J., Zhang, D., Chi, Y., and Hu, C. (2022). Catalytic application of polyoxovanadates in the selective oxidation of organic molecules. Polyoxometalates 1, 9140012. doi:10.26599/pom.2022.9140012
Li, M., Klunder, K., Blumenthal, E., Prater, M., Lee, J., Matthiesen, J., et al. (2020). Ionic liquid stabilized 2,2,6,6-tetramethylpiperidine 1-oxyl catalysis for alcohol oxidation. ACS Sustain. Chem. Eng. 8 (11), 4489–4498. doi:10.1021/acssuschemeng.9b07650
Li, W., Wang, F., Shi, Y., and Yu, L. (2023d). Polyaniline-supported tungsten-catalyzed oxidative deoximation reaction with high catalyst turnover number. Chin. Chem. Lett. 34, 107505. doi:10.1016/j.cclet.2022.05.019
Li, X., Yan, M., Wu, X., Mota-Morales, J. D., and Lian, H. (2023b). Construction and application of biobased PDES ionic gels with a soft-hard segment. ACS Appl. Polym. Mater. 5 (8), 6265–6277. doi:10.1021/acsapm.3c00913
Liang, Y., Li, Y., Wang, H., Zhou, J., Wang, J., Regier, T., et al. (2010). Co3O4 nanocrystals on graphene as a synergistic catalyst for oxygen reduction reaction. Nat. Mater. 10 (10), 780–786. doi:10.1038/nmat3087
Liu, F., Wang, H., Sapi, A., Tatsumi, H., Zherebetskyy, D., Han, H., et al. (2018). Molecular orientations change reaction kinetics and mechanism: a review on catalytic alcohol oxidation in Gas phase and liquid phase on size-controlled Pt nanoparticles. Catalysts 8, 226–238. doi:10.3390/catal8060226
Liu, P., Hao, J. W., Mo, L. P., and Zhang, Z. H. (2015). Recent advances in the application of deep eutectic solvents as sustainable media as well as catalysts in organic reactions. RSC Adv. 5 (60), 48675–48704. doi:10.1039/c5ra05746a
Long, N. Q., and Quan, N. A. (2015). Highly selective oxidation of benzyl alcohol to benzaldehyde catalyzed by nano Au/γ-Al2O3 under environment-friendly conditions. React. Kinet. Mech. Catal. 114, 147–155. doi:10.1007/s11144-014-0773-4
Lou, J., and Xu, Z. (2002). Selective solvent-free oxidation of alcohols with potassium dichromate. Tetrahedron Lett. 43, 8843–8844. doi:10.1016/s0040-4039(02)02234-7
Manasi, I., Schweins, R., Ma, K., and Edler, k.J. (2023). Nanostructure in amphiphile-based deep eutectic solvents. Langmuir 39 (47), 16776–16784. doi:10.1021/acs.langmuir.3c02105
Matuszek, K., Chrobok, A., Latos, P., Markiton, M., Szymańska, K., Jarzębskibc, A., et al. (2016). Silica-supported chlorometallate(iii) ionic liquids as recyclable catalysts for diels–alder reaction under solventless conditions. Catal. Sci. Technol. 6, 8129–8137. doi:10.1039/c6cy01771a
Mbous, Y. P., Hayyan, M., Hayyan, A., Hashim, M. A., and Looi, C. Y. (2017). Applications of deep eutectic solvents in biotechnology and bioengineering-promises and challenges. Biotechnol. Adv. 35 (2), 105–134. doi:10.1016/j.biotechadv.2016.11.006
Michael, S., and March, B. (2007). Advanced organic chemistry: reactions, mechanisms, and structure. 6th ed. New York: Wiley-Interscience.
Mirmashhori, B., Azizi, N., and Saidi, M. R. (2006). A simple, economical, and highly efficient synthesis of β-hydroxynitriles from epoxide under solvent free conditions. J. Mol. Catal. A Chem. 247 (1-2), 159–161. doi:10.1016/j.molcata.2005.11.042
Müller, M., Carlo Villalba, J., and Anaissi, F. J. (2014). Thermal decomposition (TG-DTA) of iron salts [FeCl3.6H2O] and [Fe(NO3)3.9H2O] with morphologic and chemical analysis of final product. Semin. Ciênc. Exatas Tecnol. 35 (1), 9–14. doi:10.5433/1679-0375.2014v35n1p9
Munive-Olarte, A., Hidalgo-Moyle, J. J., Velasquillo, C., Juarez-Moreno, K., and Mota-Morales, J. D. (2022). Boosting cell proliferation in three-dimensional polyacrylates/nanohydroxyapatite scaffolds synthesized by deep eutectic solvent-based emulsion templating. Colloid Interface Sci. 607, 298–311. doi:10.1016/j.jcis.2021.08.149
Nagy, G., Gal, T., Sranko, D. F., Safran, G., Maroti, B., Sajo, I. E., et al. (2020). Selective aerobic oxidation of benzyl alcohol on alumina supported Au-Ru and Au-Ir catalysts. Mol. Catal. 492, 110917. doi:10.1016/j.mcat.2020.110917
Ndolomingo, M. J., and Meijboom, R. (2017). Selective liquid phase oxidation of benzyl alcohol to benzaldehyde by tert-butyl hydroperoxide over γ-Al2O3 supported copper and gold nanoparticles. Appl. Surf. Sci. 398, 19–32. doi:10.1016/j.apsusc.2016.12.020
Nørskov, J. K., Bligaard, T., Rossmeisl, J., and Christensen, C. H. (2009). Towards the computational design of solid catalysts. Nat. Chem. 1, 37–46. doi:10.1038/nchem.121
Ozturk, O. F., Karan, B., and Karabulut, S. (2008). Solvent-free oxidation of benzyl alcohol over chromium orthoborate. Catal. Commun. 9, 1644–1648. doi:10.1016/j.catcom.2008.01.016
Paparella, A. N., Stallone, M., Pulpito, M., Capriati, V., and Vitale, P. (2024). An enhanced stereoselective synthesis of α,β-unsaturated esters through the Horner–Wadsworth–Emmons reaction in deep eutectic solvents. Org. Biomol. Chem. 22 (9), 1885–1891. doi:10.1039/d3ob02083e
Patai’s Chemistry of Functional Groups (1966). The carbonyl group. PATAI'S chemistry of functional groups, 1. John Wiley & Sons.
Procopio, D., and Ramón, D. J. (2024). Visible-light-mediated amide synthesis in deep eutectic solvents. Adv. Synth. Catal. 20, 870–876. doi:10.1002/adsc.202300725
Ragupathi, C., Vijaya, J. J., Narayanan, S., Jesudoss, S. K., and Kennedy, L. J. (2015). Highly selective oxidation of benzyl alcohol to benzaldehyde with hydrogen peroxide by cobalt aluminate catalysis: a comparison of conventional and microwave methods. Ceram. Int. 41 (2), 2069–2080. doi:10.1016/j.ceramint.2014.10.002
Rodríguez-Álvarez, M. J., García-Garrido, S. E., Perrone, S., García-Álvarez, J., and Capriati, V. (2023). Deep eutectic solvents and heterogeneous catalysis with metallic nanoparticles: a powerful partnership in sustainable synthesis. Curr. Opin. Green Sustain. Chem. 39, 100723. doi:10.1016/j.cogsc.2022.100723
Seyyed Shahabi, S., Azizi, N., and Vatanpour, V. (2020). Tuning thin-film composite reverse osmosis membranes using deep eutectic solvents and ionic liquids toward enhanced water permeation. J. Membr. Sci. 610, 118267. Related documents. doi:10.1016/j.memsci.2020.118267
Shaabani, A., Amini, M., Shadi, M., Bahri, F., Alavijeh, M. K., and Farhid, H. (2021). Chemically functionalized MIL-101-NH2 with cobalt(II) tetrasulfophthalocyanine: an efficient catalyst for the aerobic oxidation of alcohols and one-pot tandem conversion of alcohols to propargylamines. J. Taiwan Inst. Chem. Eng. 126, 211–222. doi:10.1016/j.jtice.2021.06.046
Sheikh Asadi, A. M., Atamaleki, A., Wang, C., Boczkaj, G., Lutze, H., Imran, M., et al. (2024). Catalysts for advanced oxidation processes: deep eutectic solvents-assisted synthesis – a review. Water Resour. Ind. 31, 100251. doi:10.1016/j.wri.2024.100251
Su, F., Mathew, S., Lipner, G., Fu, X., Antonietti, M., Blechert, S., et al. (2010). mpg-C3N4-Catalyzed selective oxidation of alcohols using O2 and visible light. J. Am. Chem. Soc. 132, 16299–16301. doi:10.1021/ja102866p
Tamizhdurai, P., Sakthinathan, S., Chen, S., Shanthi, K., Sivasanker, S., and Sangeetha, P. (2017). Environmentally friendly synthesis of CeO2 nanoparticles for the catalytic oxidation of benzyl alcohol to benzaldehyde and selective detection of nitrite. Sci. Rep. 7, 46372. Article number. doi:10.1038/srep46372
Tan, H., Zhou, X., Gong, T., You, H., Zheng, Q., Zhao, S.-Y., et al. (2024). Anderson-type polyoxometalate-based metal–organic framework as an efficient heterogeneous catalyst for selective oxidation of benzylic C–H bonds. RSC Adv. 14, 364–372. doi:10.1039/d3ra07120k
Tang, B., and Row, K. H. (2013). Recent developments in deep eutectic solvents in chemical sciences. Monatsh. Chem. 144 (10), 1427–1454. doi:10.1007/s00706-013-1050-3
Trikittiwong, P., Surakiet, D., Aksornkovit, N., and Chaiseeda, K. (2023). Efficient oxidation of benzyl alcohol to benzaldehyde mediated by palladium chloride impregnated on aluminium oxide-pillared clay. Appl. Sci. Eng. 16 (2), 6171.
Wagle, D. V., Zhao, H., and Baker, G. A. (2013). Deep eutectic solvents: sustainable media for nanoscale and functional materials. Accounts Chem. Res. 47, 2299–2308. doi:10.1021/ar5000488
Wang, F., Jing, C., Ping, J., He, D., Shang, W., Zeng, M., et al. (2024a). Polyoxometalate-based Cu(II) complexes as the photocatalyst for oxidation of toluene and photodegradation of metronidazole. Polyoxometalates 3, 9140067. doi:10.26599/pom.2024.9140067
Wang, J., Yu, H., Wei, Z., Li, Q., Xuan, W., and Wei, Y. (2020b). Additive-mediated selective oxidation of alcohols to esters via synergistic effect using single cation cobalt catalyst stabilized with inorganic ligand. Research 2020, 3875920. doi:10.34133/2020/3875920
Wang, Y., Wang, X., Dong, Y., Peng, M., Guo, L., Cui, M., et al. (2024b). Magnetic-responsive pickering emulsions based on MFe2O4 (M = Mn, Fe, Co, Ni, Cu, Zn) for green and efficient oxidation of benzyl alcohol. Green Chem. 26, 6131–6138. doi:10.1039/d4gc00554f
Wang, Y., Wu, Z., Yu, H., Han, S., and Wei, Y. (2020a). Highly efficient oxidation of alcohols to carboxylic acids using polyoxometalate-supported chromium(III) catalyst and CO2. Green Chem. 22, 3150–3154. doi:10.1039/d0gc00388c
Wang, Zh., Zhang, B., Yang, S., Yang, X., Meng, F., Zhai, L., et al. (2022). Dual Pd2+ and Pd0 sites on CeO2 for benzyl alcohol selective oxidation. J. Catal. 414, 385–393. doi:10.1016/j.jcat.2022.09.012
Wei, Z., Ru, S., Zhao, Q., Yu, H., Zhang, G., and Wei, Y. (2019). Highly efficient and practical aerobic oxidation of alcohols by inorganic-ligand supported copper catalysis. Green Chem. 21, 4069–4075. doi:10.1039/c9gc01248f
Wei, Z., Wang, J., Yu, H., Han, S., and Wei, Y. (2022). Recent advances of anderson-type polyoxometalates as catalysts largely for oxidative transformations of organic molecules. Molecules 27, 27165212. doi:10.3390/molecules27165212
Weng, Z., Wang, J., Zhang, S., and Jian, X. (2008). Selective oxidation of benzyl alcohol by heteropolytungstate as reaction-controlled phase-transfer catalyst with hydrogen peroxide. Chem. Soc. Jpn. 81 (4), 525–529. doi:10.1246/bcsj.81.525
Xu, S., Wu, J., Huang, P., Lao, C., Lai, H., Wang, Y., et al. (2020). Selective catalytic oxidation of benzyl alcohol to benzaldehyde by nitrates. Front. Chem. 8, 151–161. doi:10.3389/fchem.2020.00151
Yang, X. F., Wang, A., Qiao, B., Liu, J., and Zhang, T. (2013). Single-atom catalysts: a new frontier in heterogeneous catalysis. Acc. Chem. Res. 46, 1740–1748. doi:10.1021/ar300361m
Zauche, T., and Espenson, J. (1998). Oxidation of alcohols by hydrogen peroxide, catalyzed by methyltrioxorhenium (mto): a hydride abstraction. Inorg. Chem. 28, 6827–6831. doi:10.1021/ic9806784
Zhang, C., Zhang, B., Li, Z., and Hao, J. (2019). Deep eutectic solvent-mediated hierarchically structured Fe-based organic–inorganic hybrid catalyst for oxygen evolution reaction. ACS Appl. Energy Mater 2 (5), 3343–3351. doi:10.1021/acsaem.9b00197
Zhang, M., Zhai, Y., Ru, S., Zang, D., Han, S., Yu, H., et al. (2018). Highly practical and efficient preparation of aldehydes and ketones from aerobic oxidation of alcohols with an inorganic-ligand supported iodine catalyst. Chem. Commun. 54, 10164–10167. doi:10.1039/c8cc03722a
Zhang, Q., De Oliveira Vigier, K., Royer, S., and Jérôme, F. (2012). Deep eutectic solvents: syntheses, properties and applications. Chem. Soc. Rev. 41, 7108–7146. doi:10.1039/c2cs35178a
Keywords: deep eutectic solvent-based surfactants, ferric chloride, benzyl hexadecyl dimethyl ammonium chloride, aldehyde, selective oxidation, hydrogen peroxide
Citation: Shokr Chalaki B, Azizi N, Mirjafary Z and Saeidian H (2024) Selective and fast oxidation of alcohol to aldehyde using novel catalytic deep eutectic solvent surfactants. Front. Chem. 12:1416825. doi: 10.3389/fchem.2024.1416825
Received: 13 April 2024; Accepted: 09 October 2024;
Published: 18 October 2024.
Edited by:
Ranjan Dey, BITS Pilani KK Birla Goa Campus, GOA, IndiaReviewed by:
Piotr Latos, Silesian University of Technology, PolandYongge Wei, Tsinghua University, China
Copyright © 2024 Shokr Chalaki, Azizi, Mirjafary and Saeidian. This is an open-access article distributed under the terms of the Creative Commons Attribution License (CC BY). The use, distribution or reproduction in other forums is permitted, provided the original author(s) and the copyright owner(s) are credited and that the original publication in this journal is cited, in accordance with accepted academic practice. No use, distribution or reproduction is permitted which does not comply with these terms.
*Correspondence: Najmedin Azizi, YXppemlAY2NlcmNpLmFjLmly