- 1Department of Chemistry, Government College University Faisalabad, Faisalabad, Pakistan
- 2Department of Chemistry, COMSATS University Islamabad, Lahore, Pakistan
- 3Department of Radiological Sciences, College of Applied Medical Sciences, King Khalid University, Abha, Saudi Arabia
- 4Department of Biology, College of Science, Princess Nourah bint Abdulrahman University, Riyadh, Saudi Arabia
- 5Department of Clinical Laboratory Sciences, College of Applied Medical Sciences, King Khalid University, Abha, Saudi Arabia
- 6Chemistry Department, Faculty of Science, King Khalid University, Abha, Saudi Arabia
Plant extract-mediated fabrication of metal nanocomposites is used in cell proliferation inhibition and topical wound treatment, demonstrating significant effectiveness. Salvia hispanica L. (chia) seed extract (CE) is used as the reaction medium for the green fabrication of ecofriendly ZnO(CE) nanoparticles (NPs) and Ag/Ag2O(CE) and ZnO/Ag/Ag2O(CE) nanocomposites. The resultant nanoparticles and nanocomposite materials were characterized using UV–visible, Fourier-transform infrared (FTIR) spectroscopy, scanning electron microscopy (SEM), X-ray diffraction (XRD), and energy-dispersive X-ray (EDX) techniques. In the context of antioxidant studies, ZnO/Ag/Ag2O(CE) exhibited 57% reducing power and 86% 2,2, diphenyl-1-picrylhydrazyl (DPPH) free radical scavenging. All three materials showed strong antibacterial activity against Staphylococcus aureus (S. aureus), Escherichia coli (E.coli), and Bacillus subtilis (B. subtilis) bacterial strains. Additionally, ZnO(CE), Ag/Ag2O(CE), and ZnO/Ag/Ag2O(CE) also revealed 64.47%, 42.56%, and 75.27% in vitro Michigan Cancer Foundation-7 (MCF7) cancer cell line inhibition, respectively, at a concentration of 100 μg/mL. Selectively, the most effective composite material, ZnO/Ag/Ag2O(CE), was used to evaluate in vivo wound healing potential in rat models. The study revealed 96% wound closure in 10 days, which was quite rapid healing compared to wound healing using clinically available ointment. Therefore, in conclusion, the ZnO/Ag/Ag2O(CE) nanocomposite material could be considered for further testing and formulation as a good anticancer and wound healing agent.
1 Introduction
The unique attributes of nanoparticles are augmented by their higher surface area/active mass-to-weight ratio, reactivity, and bioavailability. Their integration into nanocomposites and matrixes yielded nanomaterials with a unique set of physical, chemical, and biochemical properties, which resulted in intensive studies for a wide range of applications (Bilal et al., 2022). Over the last couple of decades, nanomaterials in the form of nanopowders, nanocomposites, nanofibers, etc., have been under extensive study to be used in potential applications, such as medical products and devices (Haleem et al., 2023), water treatment systems (Naseem and Durrani, 2021), air filters and air purifiers (Mahmoudi et al., 2023), polishing agents (Amir et al., 2022), catalysts (Patra et al., 2023), biosensors (Malik et al., 2023), food packaging and food protection films (Cushen et al., 2014), paints and varnish (Ganguli and Chaudhuri, 2021), and cosmetics (Włodarczyk and Kwarciak-Kozłowska, 2021). Among these applications, the design of innovative and multifunctional nanocomposites for anticancer, antimicrobial, and wound healing agents is of basic interest due to their life-saving nature. The most life-threatening disease is cancer, the most prevalent and leading cause of mortality in the world. Multiple options are in practice to create a barrier to its proliferation in the human body, but none has been perfected yet. Nanomaterials are engineered for this purpose (Nirmala et al., 2023). For example, the treatment of breast cancer involves loading doxorubicin into liposomes, followed by loading siRNA molecules onto solid lipid and other nanoparticles. These advancements were aimed at targeted therapy to enhance therapeutic efficacy (Senapati et al., 2018). Photothermal therapy (PTT), using gold and silver nanoparticles, is being exercised to destroy cancer cells and stimulate immune responses (Borzęcka et al., 2022). Moreover, as drug delivery systems (DDSs), nanoparticles play a pivotal role in improving the therapeutic efficacy of anticancer treatment and reducing the cytotoxic effect (Sharma et al., 2023). Bacterial infection is a global burden due to its life-threatening outbreaks. A long history of microbial infection treatment is associated with antibiotics, but according to the reported data and experienced clinicians, sooner or later, bacterial resistance becomes a hallmark of the use of antibiotics, which reduces their efficacy significantly. Nanomaterials paralyze and kill bacteria effectively and also eliminate the chance of bacterial resistance. These potential properties intensify attempts to develop antibacterial nanomaterials to treat different infectious ailments, such as deep-seated infections and wound healing (Gupta et al., 2019; Nandhini et al., 2024). The use of antibacterial compounds is not only limited to the in vivo treatment of bacterial infections but is also required for disinfecting healthcare instruments, especially surgical tools. Reported data advocate that gold nanoparticles and quantum dots, using infrared light, play a vital role in disinfecting the instruments from bacteria (Haleem et al., 2023).
Wounds are injuries to the skin tissues caused by external stimuli or trauma, categorized as acute and chronic wounds based on their healing period. Acute wounds take few weeks to heal, while chronic wounds may take several months. Contamination, colonization, and infection are the three main stages in the wound infection continuum (Li et al., 2021). Wound contamination represents the microbes in non-replicating form in an open wound. This stage does not affect the normal inflammatory response or wound healing process. Untreated microbes start replicating into colonies, which further damages the tissue and partially inactivates the inflammatory response. Further migration and replication of microbes deep into the wound bed result in an immune reaction with the characteristics of infection (acute state) (Swanson et al., 2015). The production of the glycocalyx (biofilm) by some microbes at the wound site leads to the development of a protective layer, which makes the wound hard to detect and treat (chronic state) (Malone et al., 2017). Further progression may lead to systemic complications (cellulitis, osteomyelitis, and septicemia) from topical wounds (Li et al., 2021). Chronic wounds affect approximately 20 million people globally, with an economic burden of approximately $31 billion per year for treatment and management (Järbrink et al., 2017; Nussbaum et al., 2018).
The wound healing process is an intricate interaction among wound cells, the components of the extracellular matrix, and biochemical signal pathways. Commonly exercised traditional wound healing treatments are a slow process, which is associated with some typical limitations such as sluggish tissue regeneration, long-term persistence of wound infections, and poor wound closure. This hampers the wound healing process seriously. Nanotechnology has created new research opportunities in the field of wound healing strategies and has made significant advances in cancer therapy and wound healing (Nandhini et al., 2024). Metallic nanoparticles (NPs), such as silver (Ag), gold (Au), and zinc (Zn), have been reported to have promising bactericidal properties and low cytotoxicity, which make them useful for wound healing (Rajendran et al., 2018). Ag NPs aid in the healing of superficial wounds (Solanki et al., 2023) and are often combined with other metals, particularly Au and Zn, to boost their antibacterial potential. Physical and chemical methods of NP synthesis are gradually being replaced by ecofriendly green synthesis methods because of their multiple demerits, such as the release of toxic and harmful chemicals, consumption of a large amount of energy, and use of complex synthesis conditions and equipment (Ying et al., 2022). The green synthesis of ZnO NP/silica gel dressings using Aloe barbadensis leaf extract was found effective in healing mouse wounds (Batool et al., 2020). The blend of Ag and Zn NPs has also been used in wound healing, such as the 1% Ag–ZnO/silver oxide (AgO) nanocomposites currently being investigated for the treatment of topical wounds (de Moura et al., 2022), chitosan dressings loaded with Ag and Zn NPs (chitosan/AgO/ZnO) (Li et al., 2010), and chitosan/polyethylene glycol/ZnO/Ag (chitosan/PEG/ZnO/Ag) nanocomposite dressings (Liu and Kim, 2012). Hydrogels containing Ag@ZnO core–shell nanocomposites synthesized from the leaf extract of Hibiscus sabdariffa were used for wound healing in a mouse model (Malone et al., 2017).
Salvia hispanica L. is a flowering plant belonging to the mint family (Lamiaceae) native to central and southern Mexico. The seeds obtained from this plant are commonly termed chia seeds, which possess a great deal of nutritional and medicinal value. Chia seeds are a potential source for synthesizing nanomaterials as they are readily available, inexpensive, and can easily be processed into different forms (such as gel, powder, and liquid). This easy processability is the main reason for using chia seeds as a source for nanoparticle synthesis. Chia seeds are made up of proteins, lipids, polysaccharides, some phytochemicals, flavonoids, and phenolic compounds. These flavonoids and phenolic acids act as great reducing agents and stabilizing agents for the nanoparticles. These compounds also provide great antioxidant properties to the nanomaterials; hence, they are potential antibacterial and antioxidant materials. Compounds like alpha-linolenic acid reduce inflammation and can inhibit cancer cell growth, and flavonoids and chlorogenic acid help neutralize harmful free radicals in the body, which can protect the body from bacterial attacks, reduce inflammation, and inhibit cancer cell growth. Phytochemicals like quercetin, kaempferol, and caffeic acid have the chemical ability to interfere with cancer cell proliferation, induce apoptosis (programmed cell death), and inhibit angiogenesis (the formation of new blood vessels that supply tumors), hence contributing to anticancer activity. The chemical composition of chia seeds allows easy modification through simple chemical or enzymatic treatments, further expanding their potential applications in nanoparticle synthesis (Reyes-Caudillo et al., 2008). The use of dark chia seed extract (CE) in the synthesis of Ag NPs boosted their antimicrobial activities (Hernández-Morales et al., 2019). The aim of this study is to synthesize ZnO(CE) NPs, Ag/Ag2O(CE), and ZnO/Ag/Ag2O(CE) nanocomposites by using the aqueous extract of chia seeds as a reducing and stabilizing reagent for the investigation of anticancer, antibacterial, and wound healing potential.
2 Materials and methods
2.1 Chemicals
Silver nitrate (AgNO3), zinc nitrate hexahydrate (Zn(NO3)2.6H2O), sodium hydroxide (NaOH), sodium phosphate, potassium ferricyanide, trichloroacetic acid, ferric chloride, ascorbic acid, 2,2, diphenyl-1-picrylhydrazyl (DPPH), methanol, 3-(4,5-dimethylthiazol-2-yl)-2,5-diphenyl-2H-tetrazolium bromide (MTT), dimethyl sulfoxide, and Mueller Hinton agar (MHA) were obtained from Sigma-Aldrich and used without further purification. Chia seeds were obtained from the local market. All the solutions were prepared in double-distilled deionized water.
2.2 Preparation of the chia seed extract
Chia seeds (9.6 g) were cleaned three times using distilled water and dried at room temperature. After drying, the chia seeds were heated in 100 mL of distilled water for 30 min and immediately filtrated using a nylon-fiber sieve. The CE was stored in a refrigerator at 5°C and used for NP synthesis a day after its preparation for the best antimicrobial results (Hernández-Morales et al., 2019).
2.3 Synthesis of ZnO NPs
The CE (30 mL) was heated at 60°C, followed by the addition of 1.33 g of Zn(NO3)2.6H2O (0.15 M) and agitated for at least 4 h. The solution color changed from transparent to light brown, indicating the formation of precipitates. The solution was cooled to room temperature and then centrifuged at 4,000 rpm for 20 min. The prepared nanoparticles were rinsed repeatedly in distilled water to remove any unreacted material. The material was dried at 80°C for 6 h and then calcinated at 500°C for 4 h (Sorbiun et al., 2018).
2.4 Synthesis of the Ag/Ag2O nanocomposite
Ag/Ag2O(CE) was synthesized by adding 0.8491 g AgNO3 to 20 mL deionized water and mixing well to form a uniform solution (0.25 M), followed by the addition of 1.6 mL CE. The mixture was stirred vigorously for 15 min. The precipitates turned the color of the solution from transparent to light yellow. Further overnight stirring at room temperature was carried out to obtain AgO NPs. The resultant mixture was centrifuged at 4,000 rpm for 10 min. The prepared precipitates were separated, dried at 80°C, and then calcinated at 300°C for 2 h. The resulting brown precipitates indicated the successful synthesis of Ag/Ag2O(CE) (Hernández-Morales et al., 2019).
2.5 Synthesis of the ZnO/Ag/Ag2O nanocomposite
The ZnO/Ag/Ag2O nanocomposite was prepared using a co-precipitation method with slight modifications (Hernández-Morales et al., 2019). The nanocomposite was synthesized by the addition of AgNO3 (0.212 g∼0.025 M [0.0012 mol]) and Zn(NO3)2.6H2O (0.376 g∼0.025 M [0.0012 mol]) to 50 mL of distilled water. The components were mixed well to create a homogeneous solution. Then, 1.6 mL of CE was added dropwise under vigorous stirring for 15 min at ambient temperature. The change in the color of the solution from transparent to brown was an indication of precipitate formation. Overnight stirring of the solution resulted in brown precipitates settling at the bottom of the beaker. The mixture was centrifuged at 4,000 rpm for 10 min, followed by the collection of precipitates, drying at 80°C for 6 h, and then calcination at 400°C for 4 h. ZnO/Ag/Ag2O(CE) nanocomposites were consequently obtained in the form of brown powder. The visual analysis of the final nanomaterials synthesized is shown in Supplementary Figure S1.
2.6 Characterizations
A UV–visible spectrophotometer (Shimadzu UV-2550 Spectrophotometer), Fourier-transform infrared (FTIR) spectrophotometer (Nicolet Magna 550 Spectrophotometer), scanning electron microscope, powder X-ray diffractometer, and energy-dispersive X-ray (EDX) spectrophotometer were used to characterize ZnO(CE) NPs and Ag/Ag2O(CE) and ZnO/Ag/Ag2O(CE) composites in order to identify their physical features. The UV–visible spectra in the range of 200–800 nm were recorded. The FTIR spectrophotometer covering a wave number range of 650–4,000 cm-1 was utilized to determine the functional groups found in ZnO(CE) NPs and Ag/Ag2O(CE) and ZnO/Ag/Ag2O(CE) nanocomposites. SEM investigated the appearance and structure of nanoparticles and nanocomposites. XRD patterns were recorded using a PANalytical X’Pert (Naseem and Durrani, 2021) material research diffractometer with CuKα radiation to determine sample phases in the range of 10°–80°.
2.7 Antioxidant study
The antioxidant potential of the synthesized NPs and nanocomposites was determined using the most commonly adopted methods, such as reducing power and DPPH free radical scavenging assays.
2.7.1 Reducing power assay
The antioxidant activity of ZnO(CE), Ag/Ag2O(CE), and ZnO/Ag/Ag2O(CE) was determined according to a method described previously (Soni and Dhiman, 2017). In brief, 2.5 mL of different concentrations (62.5 μg/mL, 125 μg/mL, 250 μg/mL, 500 μg/mL, and 1,000 μg/mL) of the sample (ZnO(CE) or Ag/Ag2O(CE) or ZnO/Ag/Ag2O(CE)) was added separately to 2.5 mL of 200 mmol/L sodium phosphate (pH 6.6), which was then mixed with 2.5 mL of the potassium ferricyanide solution. The mixture was then incubated at 50 C for 20 min, followed by the addition of 2.5 mL of 10% trichloroacetic acid (w/v), and centrifuged at 650 rpm for 10 min. After centrifugation, 5 mL of the supernatant was mixed with 5 mL of distilled water and 1 mL of 0.1% ferric chloride. The absorbance of this solution was measured at 700 nm compared to ascorbic acid used as the standard. Higher absorbance yielded a higher reducing potential.
2.7.2 DPPH free radical scavenging assay
The (DPPH free radical assay was carried out to assess the radical scavenging activity of the antioxidant component using a previously reported protocol (Al-Shmgani et al., 2016). In brief, a ZnO(CE), Ag/Ag2O(CE), or ZnO/Ag/Ag2O(CE) sample methanol solution of different concentrations (125 µg–1,000 μg/mL) was mixed with 4 mL of the 0.2 mM methanol solution of DPPH free radical. After 30 min of incubation at room temperature, the absorbance was recorded at 517 nm. The scavenging activity was measured using Eq 1:
where Ablank is the absorbance of the DPPH solution and Asample is the absorbance of the sample solution. The lower the absorbance, the higher the DPPH radical scavenging activity.
2.8 Antibacterial potential study
The antimicrobial activity of ZnO(CE) NPs and Ag/Ag2O(CE) and ZnO/Ag/Ag2O(CE) nanocomposites was investigated using the well diffusion method with slight modifications, as previously reported (Al-Shmgani et al., 2016). The antibacterial activity was assessed against three bacterial strains: S. aureus, B. subtilis, and E. coli. An agar solution containing 3.8 gMH of agar was prepared and sterilized in an autoclave. The sterilized media were then transferred to Petri dishes, and bacterial cultures were spread on the solid medium surface. A stock solution of nanocomposites was prepared at various concentrations (3 mg/mL, 1.5 mg/mL, 0.750 mg/mL, and 0.375 mg/mL). DMSO and CFR 30 of 30 μg/mL were used as the negative and positive controls, respectively. Six wells were punctured in each Petri plate, 40 ppm solution from each dilution was inoculated into every Petri dish, and a CFR 30 disc was placed in the center. These Petri dishes were incubated at 37°C for 24 h. After 24 h, the nanocomposites inhibited the growth of the bacterial cultures, and the diameter of the zone of inhibition (ZOI, mm) was measured against each organism. Triplicate measurements were taken to ensure the accuracy and reliability of the results.
2.9 Anticancer activity
Using a slightly altered MTT assay developed by Bio Basic Inc. (Canada), the cytotoxicity of ZnO(CE) NPs and Ag/Ag2O(CE) and ZnO/Ag/Ag2O(CE) nanocomposites was evaluated using the breast cancer cell line MCF7 provided by the American Type Culture Collection (ATCC) center (Hashem and El-Sayyad, 2023). The viability and inhibition percentages were determined using the following Eqs 2, 3, respectively:
2.10 Wound healing in vivo studies
The Department of Pharmaceutics, Government College University Faisalabad, Pakistan, approved the experimental methods for analyzing wound healing. The four mice used in the experiment were categorized into two groups, each with a pair of mice. Group I was designated as the control group, and group II was treated with ZnO/Ag/Ag2O(CE). Under anesthesia with chloroform, a full-thickness excision wound measuring 6 mm was made after shaving the dorsal side hair of the mice using a sterile surgical blade. The mice in the treatment group were given 1 mL of synthetic ZnO/Ag/Ag2O(CE) (2 mM) in the form of niosomes, whereas the mice in the control group were given 1 mL of standard saline. These ingredients were mixed into a 2 × 2-cm2 dressing, which was then placed on the wound bed. For 10 days, this application was done once every day. The contraction of the wound tissue was observed at intervals of 0, 2, 4, 6, 8, and 10 days to gauge the degree of wound healing. Additionally, on the specified days, pictures of the wounds in the control and treated mice were taken and compared (Al-Shmgani et al., 2016).
3 Results and discussion
3.1 FTIR studies
FTIR analysis identified the functional groups in the ZnO(CE) NPs and Ag/Ag2O(CE), and ZnO/Ag/Ag2O(CE) nanocomposites, as shown in Figure 1, with spectral ranges from 4,000 to 600 cm−1. Specifically, the band within 3,483.2–3,286.0 cm−1 is due to O-H group stretching vibrations from phytochemicals like polyphenols, alkaloids, and flavonoids in the chia seed extract, confirming the existence of diverse encapsulated and reducing bioactive molecules in the extract (Hernández-Morales et al., 2019). In the case of Ag/Ag2O(CE), the faint band observed in the AgO spectrum at approximately 3,238 cm−1 can be allocated to the stretching of the C–H bonds in the aldehyde molecule. Various absorbent bands at 1,760 cm–1, 1,373 cm–1, 1,293 cm−1, and 1,070–1,000 cm−1 are due to C=C bond vibrations associated with stretching (Laouini et al., 2021), the presence of C-O (Sobhani-Nasab and Behpour, 2015) and C=O stretching vibrations due to carbonyl and carboxylate groups (Jaiswal et al., 2022), and CH2O stretching or bending vibrational bands (Chen et al., 2014). Furthermore, C-H bending was identified as the cause of the band observed at 807 cm–1 (Barwant et al., 2022). The absorption range observed in ZnO(CE) at 3,271 cm−1, 1,638.7 cm−1, 1,376.2 cm–1, 1,050.9 cm−1, and 814 cm–1 is linked to the O-H stretching vibrations of alcoholic and carboxylic groups, C=C bonding in alkene, C-H stretching of the methyl group, C-O bonding in polysaccharides (Zafar et al., 2021), and the O-H functional group (Sorbiun et al., 2018). The FTIR spectrum of ZnO/Ag/Ag2O(CE) indicates the existence of a strong band at approximately 3,296 cm-1 linked to the vibratory stretching of the hydrogen-bonded O-H group, indicating that the water molecules are captured by the samples (Ding et al., 2019). The bands observed at 1,625 cm–1 and 1,524 cm–1 indicated the C=C stretching vibrations (Thatikayala et al., 2020) and the N-O stretching band, respectively. Furthermore, bands between 1,300 and 1,043 cm–1 were detected, attributed to the O-H bending and CO-O-CO stretching of bonds. C = C bending vibrations of unsaturated compounds in the seed extract were observed at 819 cm–1. In the case of ZnO/Ag/Ag2O(CE), a distinct peak was observed within the 1,094–821 cm−1 range, corresponding to the combined absorptions of Zn-O and Ag-O bonds.
3.2 XRD studies
The X-ray diffraction spectrum of Ag/Ag2O(CE) is shown in Supplementary Figure S2. Several diffraction patterns were observed in the face-centered cubic structure at 38.1, 44.3, and 64.6 corresponding to the metallic Ag (111), (200), and (220), respectively (JCPDS card no. 04-0783) (Elyamny et al., 2021). Changes required. Peaks at 32.7°, 38.06°, 54.8°, and 65.6° were assigned to cubic phase Ag2O diffraction planes (111), (200), (220), and (311) (JCPDS card no. 41-1104) (Sophia et al., 2020). The average size of the Ag/Ag2O nanoparticles was calculated using Scherrer’s equation (Eq. 4) and the full breadth at half-maximum (FWHM) of the diffraction patterns.
Here, D is the crystalline size of the nanoparticles, K denotes a constant of 0.94, λ is the wavelength of radiation (1.54056 Å for CuKα radiation), β is the peak breadth at half-maximum intensity, and Ѳ is the peak position. Thus, the average size of the green synthesized Ag/Ag2O(CE) nanoparticles was 46.6 nm. The XRD pattern of the green synthesized ZnO nanoparticles, as shown in Supplementary Figure S2, indicates the crystalline structure of the synthesized nanoparticles. The sharp diffraction bands were observed at 2Ѳ values: 31.68, 34.34, 36.02, 47.51, 56.26, 62.67, 67.1 68.18, 69.73, 72.7, and 77.7 of (100), (002), (101), (102), (110), (103), (200), (112), (201), (004), and (202) planes, respectively, which confirms the hexagonal wurtzite structure (JCPDS no. 75-1526) of the synthesized nanoparticles (Althubiti et al., 2023). Thus, the average dimension of green synthesized ZnO nanoparticles is estimated to be 16.15 nm.
The XRD spectra of the ZnO/Ag/Ag2O(CE) nanocomposite obtained (Figure 2A) were confirmed against the standards of Ag, Ag2O, and ZnO. The diffraction lines located at 38.1 (111), 44.28 (200), and 64.6 (220) verified the presence of Ag in the ZnO/Ag/Ag2O composite (JCPDS card no. 04-0783) (Umukoro et al., 2016). Peaks at 32.1 (111), 38.1 (200), 55.1 (220), and 65.4 (311) can be attributed to the crystalline plane of Ag2O (JCPDS card no. 41-1104). Thus, it is validated that Ag and Ag2O exist together in ZnO/Ag/Ag2O (Umukoro et al., 2016). The remaining characteristic peaks at 31.8°, 34.4°, 36.3°, 47.5°, 56.6°, 62.9°, 67.9°, 68.7°, and 69.1° match (100), (002), (101), (102), (110), (103), (200), (112), and (201) crystalline orientations of ZnO (Ding et al., 2019). The suggested average particle size of the nanocomposite was 22.42 nm. The doping of ZnO into Ag/Ag2O induces alterations in peak intensity and diffraction angle in the XRD patterns depicted in Figure 2B. This doping may introduce new phases into the host material, leading to reduced peak intensity as crystal planes undergo transformation. Additionally, a minor shift toward higher angles occurs, which is attributed to the expansion of host lattice parameters in the final composite ZnO/Ag/Ag2O (Hosseini et al., 2015; Pandi et al., 2023).
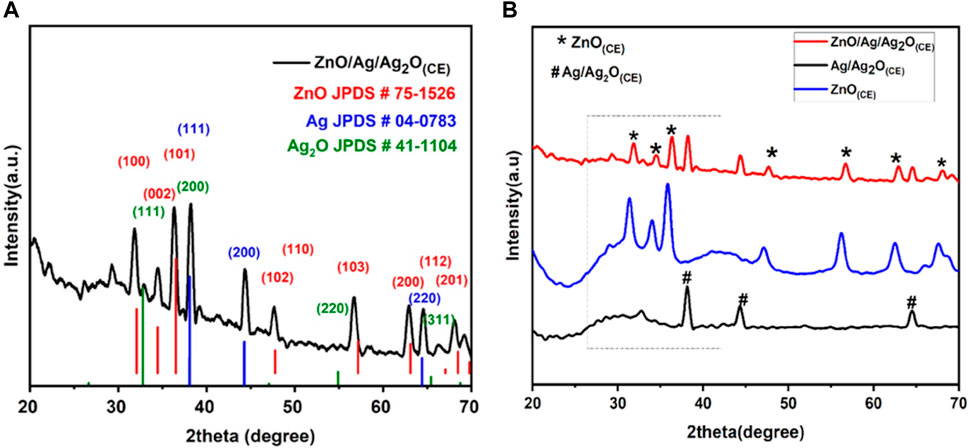
Figure 2. (A) XRD spectra of ZnO/Ag/Ag2O(CE). (B) Stacked XRD graphs of ZnO(CE), Ag/Ag2O(CE), and ZnO/Ag/Ag2O(CE).
3.3 UV–visible studies
The UV–visible spectra of chia seed mucilage, ZnO(CE) NPs, and Ag/Ag2O(CE) and AgO.ZnO(CE) nanocomposites are shown in Figure 3A. The peak observed at 315 nm shows the interaction of phenols, flavonoids, and alkaloids in the chia seed mucilage. The chia seed mucilage peak is used as the standard. Ag/Ag2O(CE) shows a peak at 319 nm, indicating the conjugation of AgO and the chia seed due to the appearance of a redshift, while the ZnO(CE) peak was observed at 297 nm, showing a blue shift compared to the chia seed mucilage. The peak at 285 nm exhibited by ZnO/Ag/Ag2O(CE) showed a higher blue shift of 30 nm compared to the chia seed mucilage. This optical property was also supported by calculating the Tauc plots for the Ag/Ag2O(CE), ZnO(CE), chia seed extract, and final ZnO/Ag/Ag2O(CE) composite (Figure 3B). The band gap energies of Ag/Ag2O(CE), ZnO(CE), chia seed extract, and ZnO/Ag/Ag2O(CE) were calculated as 4.39, 4.38, 4.41, and 4.92 eV, respectively. This increase in the band gap energy of the final composite compared to the Ag/Ag2O(CE), ZnO(CE), and chia seed extract also confirms the predicted blue shift. This increase also justified the infiltration of the dopants in the final composite.
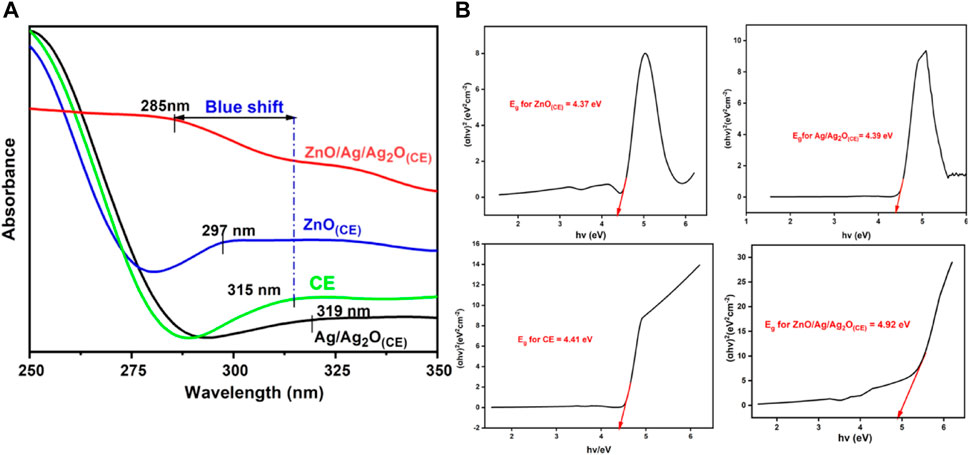
Figure 3. (A) UV–visible spectra of chia seed extract, ZnO(CE), Ag/Ag2O(CE), and ZnO/Ag/Ag2O(CE). (B) Tauc’s plot analysis for band gap energy calculations of the prepared nanocomposites.
3.4 SEM analysis
SEM is used to study the fabrication of the Ag/Ag2O(CE) nanocomposite (Figure 4A) and shows a particle-like heterostructure; there is no obvious difference between the morphology and size of Ag and Ag/Ag2O (Yang et al., 2016). Figure 4B shows the SEM images of ZnO(CE) NPs at a lower resolution; it shows that particles are agglomerated and complete separation has probably not happened, while a higher magnification image shows irregularly shaped nanoparticles (Mohan and Renjanadevi, 2016). Figure 4C shows a spherical-shaped nanocomposite of ZnO/Ag/Ag2O(CE) (Umukoro et al., 2016). EDX analysis also showed the presence of silver oxide, zinc oxide, and the ZnO/Ag/Ag2O composite (Supplementary Figure S3).
3.5 Antibacterial studies
Bio-synthesized ZnO NPs and Ag/Ag2O and ZnO/Ag/Ag2O nanocomposites were evaluated for their antibacterial activity toward S. aureus, B. subtilis, and E. coli using the well diffusion approach, as shown in Supplementary Figure S4. It was observed that microbial growth of 3 mg/mL of NPs showed the highest diameter of the zone of inhibition against each microorganism. The maximum zone of inhibition was shown by Ag/Ag2O(CE) at 3 mg/mL against B. subtilis, at 17 mm and 14 mm toward S. aureus, and 13.5 mm against E. coli, while ZnO(CE) demonstrated the maximum ZOI at 16 mm against B. subtilis, 14 mm against S. aureus, and 12.5 mm against E. coli. The ZOI was shown by ZnO/Ag/Ag2O(CE) at 25.3 mm against B. subtilis, 21 mm against S. aureus, and 17.5 mm against E. coli at 3 mg/mL.
The negative control showed no ZOI, and the positive control showed the highest inhibition against B. subtilis. Among these NPs and nanocomposites, ZnO/Ag/Ag2O(CE) exhibited the maximum zone of inhibition against B. subtilis at 25 mm. ZnO is toxic to bacteria and has antibacterial properties against resilient species (Bilal et al., 2022). Previous studies showed that growth inhibition increased with increasing NP content in wells, and a similar case was observed in this study (Lawrence, 2015). Depending on the size and concentration of the NPs, the inhibitory zone dimension differed against various bacteria. Antimicrobial activity against S. aureus, E. coli, and B. subtilis is shown in Figure 5.
The general antibacterial action of the prepared nanocomposites occurs when ZnO and Ag/Ag2O nanoparticles work synergistically for their antibacterial activity. ZnO nanoparticles generate reactive oxygen species (ROS), such as superoxide radicals (O•2−), hydroxyl radicals (OH•), and singlet oxygen, under light irradiation or in an aerobic environment. The reaction can be represented as
These ROS can induce oxidative stress in bacterial cells, causing damage to lipids, proteins, and DNA, ultimately causing cell death. Similarly, Ag/Ag2O nanoparticles release silver ions (Ag+) in the presence of aqueous media or in contact with bacterial cell membranes. The reaction can be represented as
Silver ions disrupt bacterial cell membrane integrity, interfere with cell division, and inhibit enzymatic activity crucial for bacterial survival. This combined action of ROS generated by ZnO and silver ions released from Ag/Ag2O nanoparticles results in a synergistic antibacterial effect. ROS cause oxidative damage to bacterial cells, making them more susceptible to the antimicrobial action of silver ions. Additionally, silver ions can enhance ROS generation by ZnO through redox reactions, further amplifying the antibacterial activity. The cumulative effects of ROS-induced oxidative stress and silver ion toxicity lead to irreversible damage to bacterial cells. This antibacterial action is shown in Figure 6.
3.6 Antioxidant activities
3.6.1 Reducing power activity
Free radicals which are highly reactive molecules can be neutralized by obtaining electrons from antioxidants. Antioxidants stabilize these free radicals by donating electrons, known as their reducing power. This reducing power is utilized in the chemical reaction where Fe+3/ferrocyanide complex is converted to its ferrous(Fe+2) form through direct electron donation (Karthick et al., 2012). The product was measured at 700 nm after a strong Prussian blue complex was formed. The reducing capacity of Ag/Ag2O(CE) exhibited absorbance values of 0.099, 0.162, 0.216, 0.323, and 0.401, whereas ZnO(CE) displayed absorbance values of 0.0571, 0.1016, 0.198, 0.277, and 0.355. The ZnO/Ag/Ag2O(CE) nanocomposite exhibited absorbance values of 0.197, 0.263, 0.307, 0.433, and 0.573, while the standard ascorbic acid exhibited optical density values of 0.284, 0.397, 0.522, 0.612, and 0.786, as shown in Figure 7. Increased absorbance of the reaction mixture or concentration-dependent reducing power was demonstrated by the nanocomposites. However, their reducing power was not as powerful as that of ascorbic acid (standard), which demonstrated the highest reducing activity. Reactive radicals can be reduced to more stable and non-reactive forms by receiving electrons from antioxidant substances (Gülçın et al., 2003). The outcomes from such investigations indicated that the synthesized ZnO/Ag/Ag2O(CE) possessed higher antioxidant activity than Ag/Ag2O(CE) and ZnO(CE). It was found that absorbance increased with the sample concentration. Higher reaction mixture absorbance suggests higher reduction efficiency.
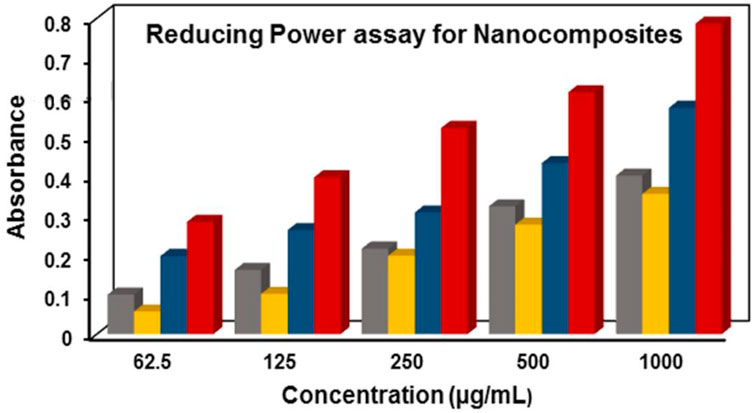
Figure 7. Reducing power assay for ZnO(CE) (yellow), Ag/Ag2O(CE) (gray), ZnO/Ag/Ag2O(CE) (blue) and ascorbic acid(Ref) (red).
3.6.2 DPPH free radical scavenging study
The free radical scavenging activity of the ZnO(CE) NPs and Ag/Ag2O (CE) and ZnO/Ag/Ag2O(CE) nanocomposites was evaluated using the DPPH technique. The analysis was carried out thrice. At the maximum concentration of 1,000 μg/mL, the synthesized Ag/Ag2O (CE) had better antioxidant activity of 69.91%, whereas ZnO (CE) exhibited higher DPPH activity of 52.138% at 1,000 μg/mL. ZnO/Ag/Ag2O (CE) showed the highest radical scavenging activity of 86.554% at 1,000 μg/mL (Aththanayaka et al., 2023). Antioxidant activity increased with the concentration of the tested samples in the DPPH assay (Awan et al., 2023). Thus, the antioxidant activity of ZnO/Ag/Ag2O (CE) was higher than that of Ag/Ag2O (CE) NPs and ZnO(CE), which was significant (Figure 8).
3.7 Anticancer activity
Anticancer analysis results revealed that ZnO(CE) NPs and Ag/Ag2O(CE) and ZnO/Ag/Ag2O(CE) nanocomposites exhibited anticancer activity against MCF7 breast cancer cells, with IC50 values of 100.24, 100.26, and 100.23, respectively (Narayan et al., 2010). The anticancer activity of ZnO(CE), Ag/Ag2O(CE), and Zn/Ag/Ag2O(CE) toward MCF7 at a concentration of 100 μg/mL was 64.47%, 42.56%, and 75.27%, respectively, as shown in Figure 9. Metal oxide nanoparticles (ZnO and Ag/Ag2O) and nanocomposites (ZnO/Ag/Ag2O) generate free radicals, which give rise to ROS. The ROS induce oxidative stress in cancer cells by disrupting the cellular metabolic pathways and damaging DNA, proteins, and lipids, leading to apoptosis. In the ZnO/Ag/Ag2O nanocomposite, zinc and silver free radicals interfere with the mitochondrial protease, resulting in apoptosis through caspase activation, and inhibit the cell cycle to stop further cancer proliferation (Roshani et al., 2023). The proposed anticancer mechanism of the ZnO/Ag/Ag2O nanocomposite is shown in Figure 10.
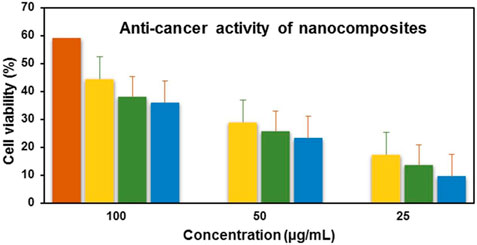
Figure 9. Anticancer activity of doxorubicin(+) (red), ZnO(CE) (green), Ag/Ag2O(CE) (blue), ZnO/Ag/Ag2O (yellow).
3.8 In vivo wound healing studies
An excision wound model was used to illustrate the wound healing ability of the synthesized Ag NPs. During therapy, ZnO/Ag/Ag2O(CE)-treated wounds showed no indication of microbial contamination, hemorrhage, or pus development, whereas the control wounds revealed significant inflammation and bleeding. From day 6 onwards, the ZnO/Ag/Ag2O(CE)-treated group showed distinguished wound closure and decreased wound size, which improved during the remaining days of treatment compared to controls (Supplementary Figure S5).
The ZnO/Ag/Ag2O(CE)-treated wound had approximately 96% closure at the conclusion of the trial, whereas the control wound had approximately 76% closure (Figure 11A). A visual analysis (Figure 11B) showed that ZnO/Ag/Ag2O(CE)-treated rats had superior wound healing activity than control groups. Previous research has demonstrated the possible influence of AgNPs on wound healing in an animal species and demonstrated that quick healing and improved cosmetic quality appear in a dose-dependent manner. Moreover, AgNPs showed advantages due to their antimicrobial properties, mitigation of wound swelling via decreased lymphocyte and mast cell intrusion, and amendment of fibrogenic cytokines (Tian et al., 2006). Similarly, the impact of AgNPs on dermal stiffness and epidermis re-epithelialization during wound recovery indicated that AgNPs may speed up wound closure (Liu et al., 2010). This characteristic is considered to enhance keratinocyte proliferation and movement.
In addition, AgNPs may promote the differentiation of fibroblasts into myofibroblasts, resulting in wound contraction (Gunasekaran et al., 2011). Zinc oxide is valuable in wound dressings because of its capability to protect against bacteria (Shu et al., 2023). The enhanced wound healing efficacy results primarily from the synergistic antimicrobial effects of Ag and Zn, leading to a reduction in inflammation. This not only prevents additional infections in the wound but also accelerates the epithelialization process by creating a favorable healing environment (Kantipudi et al., 2018).
Peng, Y. et al. (2022) demonstrated the preparation of cellulose/chitosan/Ag/Ag2O/ZnO nanocomposites through synthetic methods, evaluating their efficacy against E. coli and S. aureus bacterial strains (Peng et al., 2022). Aththanayaka, S. et al. (2023) outlined a synthetic approach for synthesizing Ag/Ag2O/ZnO nanocomposites utilizing Murusi peel and Kew peel, showcasing promising antioxidant activity through DPPH radical scavenging assays (Aththanayaka et al., 2023). Hezam, A. et al. (2023) attributed the antibacterial activity of Ag, ZnO, and Ag2O to intrinsic properties while also investigating the cytotoxicity of Ag/Ag2O/ZnO on Huh-7 human liver cancer cells, yielding IC50 values of 112.9 and 313 μg/mL for samples prepared using 3 mL (3AgZn) and 7 mL AgNO3 (7AgZn), respectively (Hezam et al., 2023). Ramakrishnegowda, D. H. et al. (2023) reported potent antibacterial activity against E. coli, S. aureus, and B. subtilis, with higher efficacy observed against Salmonella typhi. Additionally, AgO/Ag/ZnO exhibited superior inhibition of Candida albicans compared to the standard, along with potent anticancer activity against HCT-116 cell lines (IC50 value: 16.61 μg/mL) (Ramakrishnegowda et al., 2023). de Moura et al. (2022) conducted wound healing analysis, revealing an 82.7% reduction in neutrophil activity after 21 days in wounds treated with Ag-ZnO/AgO nanoparticles compared to the vehicle group (de Moura et al., 2022). A comparative literature review have also been summarized in Table 1 for the reader’s interest. Building on these findings, our present research on the ZnO/Ag/Ag2O(CE) nanocomposite comprehensively examines its antibacterial activity, showcasing the highest zone of inhibition against B. subtilis, along with their antioxidant, anticancer, and wound healing activities, demonstrating 96% healing within 10 days.
4 Conclusion
In conclusion, an ecofriendly method was used to fabricate stable ZnO(CE) NPs and Ag/Ag2O(CE) and ZnO/Ag/Ag2OCE) nanocomposites using S. hispanica L. (commonly known as chia seeds) extract without any special capping agent. The synthesized nanocomposites were characterized by UV–visible, FTIR, SEM, and XRD techniques. The XRD analysis revealed that the crystallite sizes of ZnO(CE) NPs and Ag/Ag2O(CE) and ZnO/Ag/Ag2O(CE) nanocomposites were 16.15 nm, 46.6 nm, and 22.42 nm, respectively. The SEM analysis showed that Ag/Ag2O(CE) had hetero-structure particles, ZnO(CE) had agglomerated nanoparticles, and ZnO/Ag/Ag2O(CE) exhibited a spherical particle-like morphology of the composite. ZnO(CE) NPs and Ag/Ag2O(CE) and ZnO/Ag/Ag2O(CE) nanocomposites exhibited good antibacterial activity against S. aureus, E. coli, and B. subtilis. The resistance of these nanoparticles and nanocomposites to B. subtilis bacterial strains was 17 mm, 16 mm, and 25.3 mm, respectively. The highest antioxidant activity, including reducing power activity and DPPH radical scavenging activity, was depicted by ZnO/Ag/Ag2O(CE), while the lowest activity was shown by Ag/Ag2O(CE). ZnO/Ag/Ag2O(CE) toward the MCF7 cancer cell line yielded the highest in vitro anticancer activity, i.e., 75.27% inhibition at a sample concentration of 100 μg/mL. Finally, the in vivo treatment of wounds in an animal model with the ZnO/Ag/Ag2O(CE) nanocomposite showed approximately 96% closure in 10 days, while the control showed ∼50% closure in 10 days. These findings emphasize the significant role of ecofriendly fabricated multifunctional ZnO/Ag/Ag2O(CE) nanocomposites in cancer inhibition and wound healing processes.
Data availability statement
The original contributions presented in the study are included in the article/Supplementary Material, further inquiries can be directed to the corresponding authors.
Ethics statement
The animal study was approved by the Animal Ethics Committee, Government College University Faisalabad. The study was conducted in accordance with the local legislation and institutional requirements.
Author contributions
AR: investigation, writing–original draft, funding acquisition, validation, and visualization. FA: data curation, formal analysis, software, and writing–review and editing. MJ: data curation, formal analysis, writing–review and editing, and resources. SN: conceptualization, methodology, project administration, and writing–original draft. SH: conceptualization, methodology, visualization, and writing–original draft. HA: data curation, software, and writing–review and editing. MN: data curation, formal analysis, and writing–review and editing. ZA: formal analysis, resources, and writing–review and editing. AA: data curation, funding acquisition, and writing–review and editing. MM: data curation, software, validation, and writing–review and editing. AM: data curation, validation, and writing–review and editing. MB: data curation, formal analysis, validation, funding acquisition, visualization, software, writing–review and editing. AA: data curation, conceptualization, formal analysis, validation, funding acquisition, visualization, writing–review and editing.
Funding
The author(s) declare that financial support was received for the research, authorship, and/or publication of this article. The Princess Nourah bint Abdulrahman University Researchers Supporting (project number: PNURSP2024R224), Princess Nourah bint Abdulrahman University, Riyadh, Saudi Arabia. Research Project under grant number RGP2/454/44; Deanship of Scientific Research at King Khalid University.
Acknowledgments
The authors acknowledge the Princess Nourah bint Abdulrahman University Researchers Supporting (project number: PNURSP2024R224), Princess Nourah bint Abdulrahman University, Riyadh, Saudi Arabia. The authors acknowledge the Deanship of Scientific Research at King Khalid University for funding this work through the Research Project under grant number RGP2/454/44. The authors acknowledge the Department of Chemistry and Department of Pharmaceutics, Government College University Faisalabad (GCUF), and COMSATS University Islamabad, Lahore Campus, Lahore, Pakistan, for providing the experimental support to undertake this research.
Conflict of interest
The authors declare that the research was conducted in the absence of any commercial or financial relationships that could be construed as a potential conflict of interest.
Publisher’s note
All claims expressed in this article are solely those of the authors and do not necessarily represent those of their affiliated organizations, or those of the publisher, the editors, and the reviewers. Any product that may be evaluated in this article, or claim that may be made by its manufacturer, is not guaranteed or endorsed by the publisher.
Supplementary material
The Supplementary Material for this article can be found online at: https://www.frontiersin.org/articles/10.3389/fchem.2024.1405385/full#supplementary-material
References
Al-Shmgani, H. S., Mohammed, W. H., Sulaiman, G. M., and Saadoon, A. H. (2016). Biosynthesis of silver nanoparticles from catharanthus roseus leaf extract and assessing their antioxidant, antimicrobial, and wound-healing activities. Artif. Cells Nanomedicine Biotechnol. 45 (6), 1234–1240. doi:10.1080/21691401.2016.1220950
Althubiti, N. A., Taha, T. A., Azab, A. A., and Abdelhamid, H. N. (2023). ZnO-based nanocomposites for hydrogen generation via hydrolysis of borohydride. J. Sol-Gel Sci. Technol. 106 (3), 837–846. doi:10.1007/s10971-023-06099-6
Amir, M., Sharma, R., Mishra, V., Wazed Ali, S., and Khan, G. S. (2022). Polishing performance of magnetic nanocomposites based nanoabrasive. Mater. Today Proc. 56, 549–554. doi:10.1016/j.matpr.2022.02.276
Aththanayaka, S., Thiripuranathar, G., and Ekanayake, S. (2023). Microwave-assisted phytogenic Ag/Ag2O/ZnO nanocomposite as a replacement of Ag/Ag2O and ZnO nanoparticles: a comparative antioxidant study. Surfaces Interfaces 43, 103547. doi:10.1016/j.surfin.2023.103547
Awan, S. S., Khan, R. T., Mehmood, A., Hafeez, M., Abass, S. R., Nazir, M., et al. (2023). Ailanthus altissima leaf extract mediated green production of zinc oxide (ZnO) nanoparticles for antibacterial and antioxidant activity. Saudi J. Biol. Sci. 30 (1), 103487. doi:10.1016/j.sjbs.2022.103487
Barwant, M., Ugale, Y., Ghotekar, S., Basnet, P., Nguyen, V., Pansambal, S., et al. (2022). Eco-friendly synthesis and characterizations of Ag/AgO/Ag2O nanoparticles using leaf extracts of solanum elaeagnifolium for antioxidant, anticancer, and DNA cleavage activities. Chem. Pap. 76 (7), 4309–4321. doi:10.1007/s11696-022-02178-0
Batool, M., Khurshid, S., Qureshi, Z., and Daoush, W. M. (2020). Adsorption, antimicrobial and wound healing activities of biosynthesised zinc oxide nanoparticles. Chem. Pap. 75 (3), 893–907. doi:10.1007/s11696-020-01343-7
Bilal, B., Niazi, R., Nadeem, S., Farid, M. A., Nazir, M. S., Akhter, T., et al. (2022). Fabrication of guided tissue regeneration membrane using lignin-mediated ZnO nanoparticles in biopolymer matrix for antimicrobial activity. Front. Chem. 10, 837858. doi:10.3389/fchem.2022.837858
Borzęcka, W., Pereira, P. M. R., Fernandes, R., Trindade, T., Torres, T., and Tomé, J. P. C. (2022). Spherical and rod shaped mesoporous silica nanoparticles for cancer-targeted and photosensitizer delivery in photodynamic therapy. J. Mater Chem. B 10, 3248–3259. doi:10.1039/d1tb02299g
Chen, Q., Jiang, H., Ye, H., Lǐ, J., and Huang, J. (2014). Preparation, antibacterial, and antioxidant activities of silver/chitosan composites. J. Carbohydr. Chem. 33 (6), 298–312. doi:10.1080/07328303.2014.931962
Cushen, M., Kerry, J., Morris, M., Cruz-Romero, M., and Cummins, E. (2014). Evaluation and simulation of silver and copper nanoparticle migration from polyethylene nanocomposites to food and an associated exposure assessment. J. Agric. Food Chem. 62, 1403–1411. doi:10.1021/jf404038y
de Moura, F. B. R., Ferreira, B. A., Muniz, E. H., Justino, A. B., Silva, A. G., De Azambuja Ribeiro, R. I. M., et al. (2022). Antioxidant, anti-inflammatory, and wound healing effects of topical silver-doped zinc oxide and silver oxide nanocomposites. Int. J. Pharm. 617, 121620. doi:10.1016/j.ijpharm.2022.121620
Ding, W., Zhao, L., Yan, H., Wang, X., Liu, X., Zhang, X., et al. (2019). Bovine serum albumin assisted synthesis of Ag/Ag2O/ZnO photocatalyst with enhanced photocatalytic activity under visible light. Colloids Surfaces A Physicochem. Eng. Aspects 568, 131–140. doi:10.1016/j.colsurfa.2019.02.015
Elyamny, S., Eltarahony, M., Abu-Serie, M. M., Nabil, M., and Kashyout, A. E.-H. B. (2021). One-pot fabrication of Ag @Ag2O core–shell nanostructures for biosafe antimicrobial and antibiofilm applications. Sci. Rep. 11 (1), 22543. doi:10.1038/s41598-021-01687-4
Ganguli, P., and Chaudhuri, S. (2021). Nanomaterials in antimicrobial paints and coatings to prevent biodegradation of man-made surfaces: a review. Mater. Today Proc. 45, 3769–3777. doi:10.1016/j.matpr.2021.01.275
Gülçın, İ., Oktay, M., Kıreçcı, E., and Küfrevioğlu, Ö. İ. (2003). Screening of antioxidant and antimicrobial activities of anise (pimpinella anisum L.) seed extracts. Food Chem. 83 (3), 371–382. doi:10.1016/s0308-8146(03)00098-0
Gunasekaran, T., Nigusse, T., and Dhanaraju, M. D. (2011). Silver nanoparticles as real topical bullets for wound healing. J. Am. Coll. Clin. Wound Specialists 3 (4), 82–96. doi:10.1016/j.jcws.2012.05.001
Gupta, A., Mumtaz, S., Li, C. H., Hussain, I., and Rotello, V. M. (2019). Combatting antibiotic-resistant bacteria using nanomaterials. Chem. Soc. Rev. 48, 415–427. doi:10.1039/c7cs00748e
Haleem, A., Javaid, M., Singh, R. P., Rab, S., and Suman, R. (2023). Applications of nanotechnology in medical field: a brief review. Glob. Health J. 7, 70–77. doi:10.1016/j.glohj.2023.02.008
Hashem, A. H., and El-Sayyad, G. S. (2023). Antimicrobial and anticancer activities of biosynthesized bimetallic silver-zinc oxide nanoparticles (Ag-ZnO NPs) using pomegranate peel extract. Biomass Convers. Biorefinery. doi:10.1007/s13399-023-04126-8
Hernández-Morales, L., Espinoza-Gómez, H., Flores-López, L. Z., Sotelo-Barrera, E. L., Núñez-Rivera, A., Cadena-Nava, R. D., et al. (2019). Study of the green synthesis of silver nanoparticles using a natural extract of dark or white Salvia hispanica L. seeds and their antibacterial application. Appl. Surf. Sci. 489, 952–961. doi:10.1016/j.apsusc.2019.06.031
Hezam, A., Abutaha, N., Almekhlafi, F. A., Saeed, A. M. N., Abishad, P., and Wadaan, M. A. (2023). Smart plasmonic Ag/Ag2O/ZnO nanocomposite with promising photothermal and photodynamic antibacterial activity under 600 nm visible light illumination. J. Photochem. Photobiol. A Chem. 435, 114322. doi:10.1016/j.jphotochem.2022.114322
Hosseini, S. M., Sarsari, I. A., Kameli, P., and Salamati, H. (2015). Effect of Ag doping on structural, optical, and photocatalytic properties of ZnO nanoparticles. J. Alloys Compd. 640, 408–415. doi:10.1016/j.jallcom.2015.03.136
Jaiswal, K. K., Banerjee, I., Dutta, S., Verma, R., Gunti, L., Awasthi, S., et al. (2022). Microwave-assisted polycrystalline Ag/AgO/AgCl nanocomposites synthesis using banana corm (rhizome of musa sp.) extract: characterization and antimicrobial studies. J. Industrial Eng. Chem. 107, 145–154. doi:10.1016/j.jiec.2021.11.041
Järbrink, K., Ni, G., Sönnergren, H., Schmidtchen, A., Pang, C., Bajpai, R., et al. (2017). The humanistic and economic burden of chronic wounds: a protocol for a systematic review. Syst. Rev. 6, 15. doi:10.1186/s13643-016-0400-8
Kantipudi, S., Sunkara, J. R., Rallabhandi, M., Thonangi, C. V., Cholla, R. D., Kollu, P., et al. (2018). Enhanced wound healing activity of Ag–ZnO composite NPs in wistar albino rats. Iet Nanobiotechnology 12 (4), 473–478. doi:10.1049/iet-nbt.2017.0087
Karthick, A. S., Nisha, S., and Nallathambi, A. (2012). A review on methods, application and properties of immobilized enzyme chemical science review and letters A review on methods, application and properties of immobilized Enzyme.2012 *correspondence. Che Sci. Rev. Lett. 1 (3).
Laouini, S. E., Bouafia, A., Солдатов, А. В., Algarni, H., Tedjani, M. L., Ali, G. a. M., et al. (2021). Green synthesized of Ag/Ag2O nanoparticles using aqueous leaves extracts of Phoenix dactylifera L. And their azo dye photodegradation. Membranes 11 (7), 468. doi:10.3390/membranes11070468
Lawrence, R. (2015). Green synthesis and Anti-bacterial activity of Silver Oxide nanoparticles prepared from Pinuslongifolia leaves extract. Natural Product Chemistry View project working on essential oil View project.
Li, L., Jing, D., Deng, H., Liu, Z., and Li, X. (2010). Preparation, characterization and antimicrobial activities of chitosan/Ag/ZnO blend films. Chem. Eng. J. 160 (1), 378–382. doi:10.1016/j.cej.2010.03.051
Li, S., Renick, P., Senkowsky, J., Nair, A., and Tang, L. (2021). Diagnostics for wound infections. Adv. Wound Care (New Rochelle) 10, 317–327. doi:10.1089/wound.2019.1103
Liu, X., Lee, P., Ho, C. S., Lui, V. C. H., Chen, Y., Che, C., et al. (2010). Silver nanoparticles mediate differential responses in keratinocytes and fibroblasts during skin wound healing. ChemMedChem 5 (3), 468–475. doi:10.1002/cmdc.200900502
Liu, Y., and Kim, H. I. (2012). Characterization and antibacterial properties of genipin-crosslinked chitosan/poly(ethylene glycol)/ZnO/Ag nanocomposites. Carbohydr. Polym. 89 (1), 111–116. doi:10.1016/j.carbpol.2012.02.058
Mahmoudi, A., Tavakoly Sany, S. B., Ahari Salmasi, M., Bakhshi, A., Bustan, A., Heydari, S., et al. (2023). Application of nanotechnology in air purifiers as a viable approach to protect against Corona virus. IET Nanobiotechnol 17, 289–301. doi:10.1049/nbt2.12132
Malik, S., Singh, J., Goyat, R., Saharan, Y., Chaudhry, V., Umar, A., et al. (2023). Nanomaterials-based biosensor and their applications: a review. Heliyon 9, e19929. doi:10.1016/j.heliyon.2023.e19929
Malone, M., Bjarnsholt, T., McBain, A. J., James, G. A., Stoodley, P., Leaper, D., et al. (2017). The prevalence of biofilms in chronic wounds: a systematic review and meta-analysis of published data. J. Wound Care 26, 20–25. doi:10.12968/jowc.2017.26.1.20
Mohan, A. C., and Renjanadevi, B. (2016). Preparation of zinc oxide nanoparticles and its characterization using scanning electron microscopy (SEM) and X-ray diffraction(XRD). Procedia Technol. 24, 761–766. doi:10.1016/j.protcy.2016.05.078
Nandhini, J., Karthikeyan, E., and Rajeshkumar, S. (2024). Nanomaterials for wound healing: current status and futuristic frontier. Biomed. Technol. 6, 26–45. doi:10.1016/j.bmt.2023.10.001
Narayan, K. M. V., Williams, D., Gregg, E. W., and Cowie, C. C. (2010). Diabetes public health: from data to policy. Oxford University Press.
Naseem, T., and Durrani, T. (2021). The role of some important metal oxide nanoparticles for wastewater and antibacterial applications: a review. Environ. Chem. Ecotoxicol. 3, 59–75. doi:10.1016/j.enceco.2020.12.001
Nirmala, M. J., Kizhuveetil, U., Johnson, A., G, B., Nagarajan, R., and Muthuvijayan, V. (2023). Cancer nanomedicine: a review of nano-therapeutics and challenges ahead. RSC Adv. 13, 8606–8629. doi:10.1039/d2ra07863e
Nussbaum, S. R., Carter, M. J., Fife, C. E., DaVanzo, J., Haught, R., Nusgart, M., et al. (2018). An economic evaluation of the impact, cost, and medicare policy implications of chronic nonhealing wounds. Value Health 21, 27–32. doi:10.1016/j.jval.2017.07.007
Pandi, V. S., Anusuya, M., Dagar, A., and Annu Dagar, (2023). Synthesis and characterization of ZnO, ZnO doped Ag2O nanoparticles and its photocatalytic activity. Sci. Temper 14 (03), 827–833. doi:10.58414/scientifictemper.2023.14.3.41
Patra, S., Mishra, S., Parhi, B., Mishra, H., and Swain, S. K. (2023). Role of transition metal nanocomposites in organic reactions: a state of art as an alternative to conventional catalysts. Results Chem. 6, 101172. doi:10.1016/j.rechem.2023.101172
Peng, Y., Zhou, H., Wu, Y., Ma, Z., Zhang, R., Tu, H., et al. (2022). A new strategy to construct cellulose-chitosan films supporting Ag/Ag2O/ZnO heterostructures for high photocatalytic and antibacterial performance. J. Colloid Interface Sci. 609, 188–199. doi:10.1016/j.jcis.2021.11.155
Rajendran, N. K., Kumar, S. S. D., Houreld, N. N., and Abrahamse, H. (2018). A review on nanoparticle based treatment for wound healing. J. Drug Deliv. Sci. Technol. 44, 421–430. doi:10.1016/j.jddst.2018.01.009
Ramakrishnegowda, D. H., Swamy, C. K., Kumar, B. A., Rangappa, S., Rangappa, K. S., and Shivanna, S. (2023). Ag mediated plasmonic AgO/ZnO composite and its pharmaceutical relevance. Mater. Sci. Eng. B 292, 116437. doi:10.1016/j.mseb.2023.116437
Reyes-Caudillo, E., Tecante, A., and Valdivia-López, M. A. (2008). Dietary fibre content and antioxidant activity of phenolic compounds present in Mexican chia (Salvia hispanica L.) seeds. Food Chem. 107, 656–663. doi:10.1016/j.foodchem.2007.08.062
Roshani, M., Rezaian-Isfahni, A., Lotfalizadeh, M. H., Khassafi, N., Abadi, M. H. J. N., and Nejati, M. J. C. C. I. (2023). Metal nanoparticles as a potential technique for the diagnosis and treatment of gastrointestinal cancer: a comprehensive review. a Compr. Rev. 23 (1), 280. doi:10.1186/s12935-023-03115-1
Senapati, S., Mahanta, A. K., Kumar, S., and Maiti, P. (2018). Controlled drug delivery vehicles for cancer treatment and their performance. Signal Transduct. Target Ther. 3, 7. doi:10.1038/s41392-017-0004-3
Sharma, A., Shambhwani, D., Pandey, S., Singh, J., Lalhlenmawia, H., Kumarasamy, M., et al. (2023). Advances in lung cancer treatment using nanomedicines. ACS Omega 8, 10–41. doi:10.1021/acsomega.2c04078
Shu, G., Xu, D., Xie, S., Chang, L., Liu, X., Jian, Y., et al. (2023). The antioxidant, antibacterial, and infected wound healing effects of ZnO quantum dots-chitosan biocomposite. Appl. Surf. Sci. 611, 155727. doi:10.1016/j.apsusc.2022.155727
Sobhani-Nasab, A., and Behpour, M. (2015). Synthesis and characterization of AgO nanostructures by precipitation method and its photocatalyst application. J. Mater. Sci. Mater. Electron. 27 (2), 1191–1196. doi:10.1007/s10854-015-3873-7
Solanki, P. R., Kumar, A., Singh, R. P., Singh, J., and Singh, K. R. (2023). Nanotechnological aspects for next-generation wound management. Elsevier.
Soni, N., and Dhiman, R. C. (2017). Phytochemical, anti-oxidant, larvicidal, and antimicrobial activities of Castor (ricinus communis) synthesized silver nanoparticles. Chin. Herb. Med. 9 (3), 289–294. doi:10.1016/s1674-6384(17)60106-0
Sophia, P. J., Balaji, D., Peters, T., Chander, D. S., Rishaban, S. V., Shanthi, P. V., et al. (2020). Solar induced photocatalytic degradation of methylene blue by CDS/AG2O nanocomposites. ChemistrySelect 5 (14), 4125–4135. doi:10.1002/slct.202000475
Sorbiun, M., Mehr, E. S., Ramazani, A., and Fardood, S. T. (2018). Green synthesis of zinc oxide and copper oxide nanoparticles using aqueous extract of oak fruit hull (JAFT) and comparing their photocatalytic degradation of basic violet 3. Int. J. Environ. Res. 12 (1), 29–37. doi:10.1007/s41742-018-0064-4
Swanson, T., Keast, D., Cooper, R., Black, J., Angel, D., Schultz, G., et al. (2015). Ten top tips: identification of wound infection in a chronic wound. Wounds Int. 6 (2), 22–27.
Thatikayala, D., Banothu, V., Kim, J.-S., Shin, D. B., Vijayalakshmi, S., and Park, J. (2020). Enhanced photocatalytic and antibacterial activity of ZnO/Ag nanostructure synthesized by Tamarindus indica pulp extract. J. Mater. Sci. Mater. Electron. 31 (7), 5324–5335. doi:10.1007/s10854-020-03093-4
Tian, J., Wong, K. K., Ho, C. M., Lok, C. N., Yu, W., Ming, C., et al. (2006). Topical delivery of silver nanoparticles promotes wound healing. ChemMedChem 2 (1), 129–136. doi:10.1002/cmdc.200600171
Umukoro, E. H., Peleyeju, M. G., Ngila, J. C., and Arotiba, O. A. (2016). Photocatalytic degradation of acid blue 74 in water using Ag–Ag 2 O–zno nanostuctures anchored on graphene oxide. Solid State Sci. 51, 66–73. doi:10.1016/j.solidstatesciences.2015.11.015
Włodarczyk, R., and Kwarciak-Kozłowska, A. (2021). Nanoparticles from the cosmetics and medical industries in legal and environmental aspects. Sustainability 13, 5805. doi:10.3390/su13115805
Yang, H., Tian, J., Li, T., and Cui, H. (2016). Synthesis of novel Ag/Ag2O heterostructures with solar full spectrum (UV, visible and near-infrared) light-driven photocatalytic activity and enhanced photoelectrochemical performance. Catal. Commun. 87, 82–85. doi:10.1016/j.catcom.2016.09.013
Ying, S., Guan, Z., Ofoegbu, P. C., Clubb, P., Rico, C., He, F., et al. (2022). Green synthesis of nanoparticles: current developments and limitations. Environ. Technol. Innovation 26, 102336. doi:10.1016/j.eti.2022.102336
Keywords: metallic nanoparticles and nanocomposite, green synthesis, antimicrobial in vitro, anticancer in vitro, wound healing in vivo
Citation: Rafique A, Amjad F, Janjua MRSA, Naqvi SAR, Hassan SU, Abdullah H, Nazir MS, Ali Z, Alshihri AA, Momenah MA, Mansour AA, Bajaber MA and Alalwiat AA (2024) Chia seed-mediated fabrication of ZnO/Ag/Ag2O nanocomposites: structural, antioxidant, anticancer, and wound healing studies. Front. Chem. 12:1405385. doi: 10.3389/fchem.2024.1405385
Received: 22 March 2024; Accepted: 11 June 2024;
Published: 11 July 2024.
Edited by:
Fadwa Odeh, The University of Jordan, JordanReviewed by:
Kalpana Madgula (Garudadri), RiKarbon, Inc., United StatesBuzuayehu Abebe, Adama Science and Technology University, Ethiopia
Copyright © 2024 Rafique, Amjad, Janjua, Naqvi, Hassan, Abdullah, Nazir, Ali, Alshihri, Momenah, Mansour, Bajaber and Alalwiat. This is an open-access article distributed under the terms of the Creative Commons Attribution License (CC BY). The use, distribution or reproduction in other forums is permitted, provided the original author(s) and the copyright owner(s) are credited and that the original publication in this journal is cited, in accordance with accepted academic practice. No use, distribution or reproduction is permitted which does not comply with these terms.
*Correspondence: Syed Ali Raza Naqvi, ZHJhbGlyYXphQGdjdWYuZWR1LnBr; Sadaf Ul Hassan, c2FkYWZ1bGhhc3NhbkBjdWlsYWhvcmUuZWR1LnBr