- 1Stephen Perse Foundation, Cambridge, United Kingdom
- 2Laboratoire de Physique et d’Étude des Matériaux, ESPCI Paris, PSL Research University, Sorbonne Université, Centre national de la recherche scientifique, Paris, France
- 3Key Laboratory of Industrial Equipment Quality Big Data, No.5 Electronics Research Institute of Ministry of Industry and Information Technology (MIIT), Guangzhou, China
Plastic pollution has emerged as a pressing global concern, driven by the extensive production and consumption of plastic, resulting in over 8 billion tons of plastic waste generated to date. Conventional disposal methods have proven inadequate in effectively managing polymer waste, necessitating the exploration of novel techniques. Previous research has demonstrated the successful application of photoreforming (PR) in converting water-soluble oligomer fragments of plastics into valuable chemicals. However, an unresolved challenge remains in dealing with the insoluble oligomer fragments characterized by complex chemical structures and larger molecular sizes. In this study, we propose a facile approach that involves H2PdCl4-induced activation on PET substrate for PR of PET bottles. Remarkably, this method enables the production of C1-C3 compounds without the reliance on sacrificial reagents or photocatalysts. The significant findings of this study offer a practical solution to address the most formidable aspect of plastic PR, specifically targeting the insoluble oligomer fragments. Moreover, this research contributes to the advancement of effective strategies for the sustainable management of plastic waste.
Introduction
Plastics have been vastly utilized in many fields attributing to their irreplaceable properties and low cost, but the excessive use of plastics consequently engenders an ever-growing environmental challenge (MacLeod et al., 2021; Bergmann et al., 2022; Liu et al., 2022; Sheridan et al., 2022). Particularly, 8 million tons of plastic waste are dumped into the oceans per year, and this continues to climb annually; it is predicted that marine plastic waste will outweigh the marine organisms by 2050 (MacArthur, 2017). Although plastic recycling technology has been rapidly developed, the recycling or utilization of plastics is still far from efficient and economical by traditional methods, especially for plastic of small size (microplastics, <5 mm). Plastic degradation is traditionally approached by thermal and stressful degradation methods, these protocols generally involve high temperatures (>500°C), high pressure (>103 atm), low conversion efficiency (70%), and generate a wide product distribution (Songip et al., 1993; Songip et al., 1994). Alternatively, Garcia and Robertson (Garcia and Robertson, 2017) suggested that spent polyethylene terephthalate (PET) bottles (12% of totally produced plastic) were capable of being recast into bottles by shredding, melting, and remolding the polymer. However, the thermoplastic property of PET indicates low recovery and environmentally unfriendliness (Zhang et al., 2023). This PET waste challenge demands an additional approach to recycling polymer waste. The challenge is attributed to the complex chemical structures, extremely low solubility in water, and inefficient biodegradability.
An alternative solution is to employ plastic waste as feedstock for producing high-value chemicals, under mild and environmentally friendly conditions (Zhao et al., 2022; Dong et al., 2023). Previous works have focused on the photoreforming (PR) of plastic waste toward hydrogen generation via light-driven reactions (Uekert et al., 2018; Uheida et al., 2021), typically, under illumination (Uekert et al., 2018; Cao et al., 2022; Jiao et al., 2022; Ahrens et al., 2023), the semiconductive photocatalysts supply electrons and holes to conduct H2 evolution and plastic reformation, respectively. These works provided valuable insights for managing plastic waste at ambient temperature and pressure. To date, as the liquid supernatant containing high-value products attracts the attention of researchers, the study of PR concentrated on photocatalysis of the supernatant intermediates obtained from NaOH or KOH degreasing, mainly ethylene glycol and other soluble oligomer fragments. However, PR of the more challenging precipitants with larger molecular size and lower solubility remains unexplored and neglected. As the precipitant of plastic generally accounts for a high proportion (∼40%) and contains poisonous and environmentally unfriendly products, the research of supernatant illustrates the low transmission, low recovery efficiency, high cost, and low sustainability of PR research (Ikeda et al., 2013). There is an urgent need for PR plastic valorization to put forward a more economical, efficient, and practical idea.
Bond activation using transition metals (e.g., PdO2) on organic compounds is commonly efficient in manipulating chemical reactions and subsequently controlling the reaction rate or products (Li et al., 2021; Yang et al., 2021), suggesting a potential approach for transforming the precipitants of degreased plastic into valuable products (Bai et al., 2020; Dutta et al., 2022). Meanwhile, due to the degreasing reaction with NaOH, the long chains in the PET material are randomly broken, resulting in a large number of short chains of ethylene terephthalate and a series of by-products. In this way, NaOH promotes the interaction between catalytic and PET, thus enhancing the catalytic activity. However, the synergistic effect of NaOH with PdO2 and PET was not observed, leading us to consider the utilization of acidic Pd compounds (H2PdCl4) to facilitate the degradation of PET. Herein, we report a facile H2PdCl4-induced activation on PET substrate, for PR of PET bottles to produce C1-C3 compounds, without using any sacrificial reagent or photocatalysts. Simultaneously, the PR of PET bottles also contributes to hydrogen evolution under visible light illumination. After the reaction, over 99.8% of the Pd simple substance is successfully recycled and 59.1 wt% of the propiolic acid is produced. Propiolic acids have garnered significant research attention due to their diverse mechanisms, including nucleophilic and electrophilic properties, decarboxylative coupling and addition, and their broad applications, rendering them valuable chemical materials.
This work has exceptional performances, including low cost, high sustainability, and high plastic degradation percentage, as well as highly valuable chemical materials production, suggesting a practical and innovative method for plastic degradation and propiolic acid production. Figure 1.
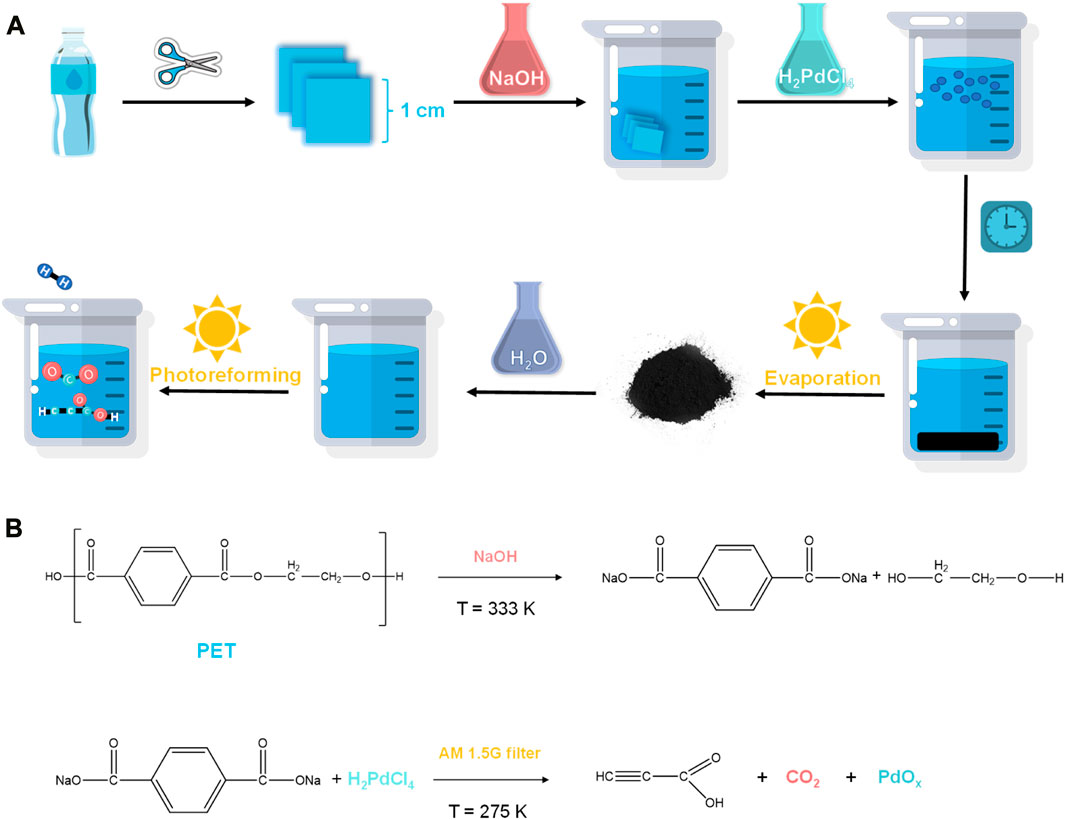
FIGURE 1. Photoreforming process. (A) From PET bottles to propiolic acid through the Pd ion activated photoreforming process. (B) The depolymerization reaction of PET to the monomer, and the photoreforming reaction of monomer.
Results and discussion
In a typical experiment, 2.5 g of randomly collected waste PET bottles were cut into ca. 1 cm2 pieces, and then transferred in 0.5 L NaOH (10 M, aq.) under 60°C for 30 h for degreasing, followed which ca. 1 g of precipitants (Labeled as Sample A, white powder, Figure 3A) was collected. The precipitants obtained from the photoreforming process typically appear as a fine white powder, the texture of the precipitants can range from fine and powdery to slightly granular. It was confirmed to be carbon-hydrogen-oxygen compounds (Figure 3B) with complex chemical structures by combination tests of gas chromatography-mass spectrometry (GC-MS), Fourier transforms infrared spectroscopy (FTIR), and nuclear magnetic resonance (NMR; Figures 2A–D). The white precipitants presented flake morphology with an average size of ca. 3.5 μm as monitored by Zeta-sizer (Figure 3C) and scanning electron microscopy (SEM; Figure 3D). The addition of precipitants into H2PdCl4 aqueous solution (20 mL, 30 mM) triggered a violent release of hydrogen bubbles, accompanied by a rapid exothermic reaction owing to the pH neutralization between H2PdCl4 and the residual NaOH on precipitants, with a reaction time of approximately 20 s. The system turned to a colorless transparent liquid with black precipitants (Labeled as Sample B) with an average size of ca. 2 μm (Figures 3E, G, H) at pH 9.2, from which products were monitored in the liquid phase (Figure 3F). The obtained products exhibited smaller geometrical and molecular sizes relative to that before the treatment, although 1H NMR presented no obvious change in this stage (Figure 2D). As additional proof, powder X-ray diffraction (PXRD) also confirmed the trace of diffraction patterns from PET precipitants (Figure 2E).11 These results prove an efficient reaction between the large-size compounds in precipitants and the Pd-based acid, which drives the micro-plastic to nano-plastic in a very short time (ca. 20 s).
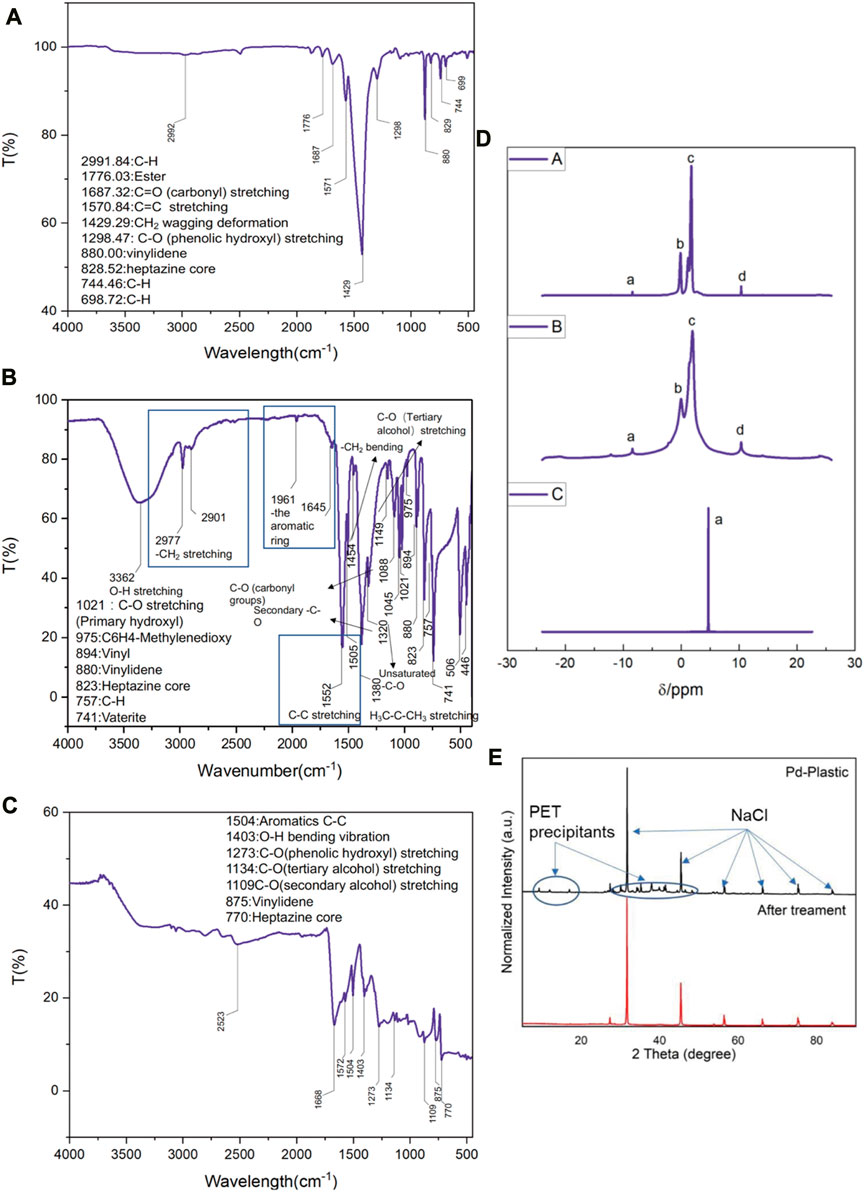
FIGURE 2. (A) FTIR of the sample A obtained from PET bottles after NaOH degreasing. (B) FTIR of the sample B obtained from A after H2PdCl4 treatment. (C) FTIR of the sample C obtained from B after 4 h illumination. (D) 1H NMR spectra of samples A, B, and C. (E) PXRD patterns of sample B before and after illumination treatment, NaCl (JCPDS No = 78-0751).
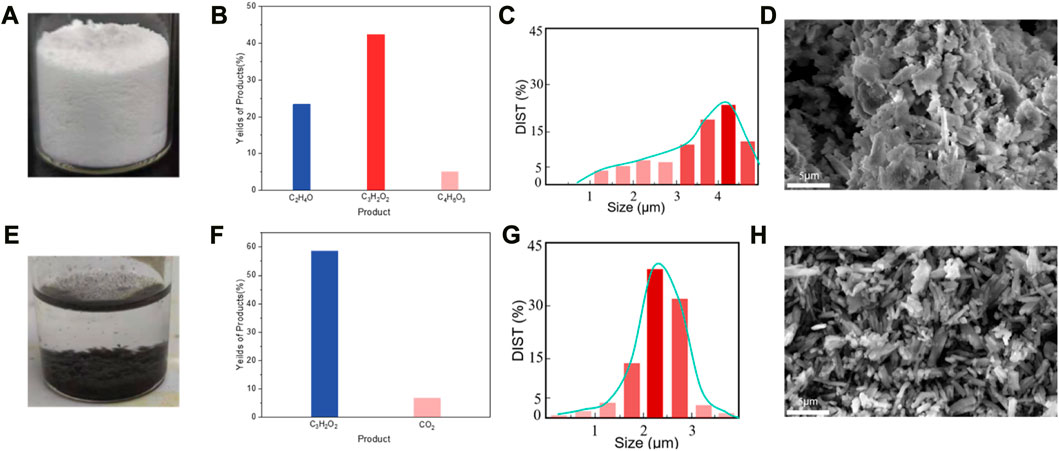
FIGURE 3. Pd activated degradation of the precipitants from degreased PET bottles. (A), Digital photograph of the precipitants obtained from PET bottles after NaOH degreasing. (B), The molar composition of precipitants was averaged from 5 individual tests and (C), corresponding size distribution (D), and SEM image. (E), Digital photograph of precipitants treated by H2PdCl4. (F), The molar composition of the liquid averaged from 5 individual tests and corresponding size distribution (G) and SEM image (H).
After H2PdCl4 treatment, the system was illuminated by simulated solar light (AM 1.5G filter) to drive the PR process. As shown in Figure 4A, the C2 to C4 compounds (Labeled as Sample C) were obtained as the main products after 2 h illumination (Figure 4B). In detail, the substance was degraded to predominant products consisting of 59.1 wt% of propiolic acid, 7.4 wt% carbon dioxide, and 5.1 wt% of hydrogen, relative to the weight of precipitants (1 g) from NaOH degreasing. Besides, after the PR reaction, the remaining weight of the precipitants was measured to be ca. 50 mg, indicating a conversion rate of ca. 95% according to the following calculation:
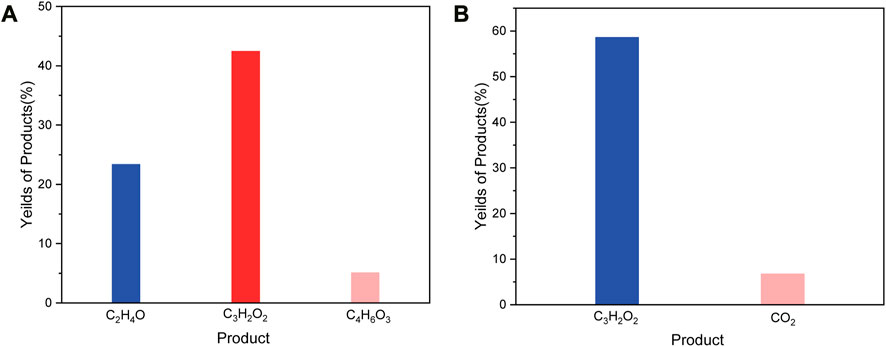
FIGURE 4. (A), Products yield after 2 h Pd activated photoreforming over PET. (B), Products yield after 4 h Pd activated photoreforming over PET.
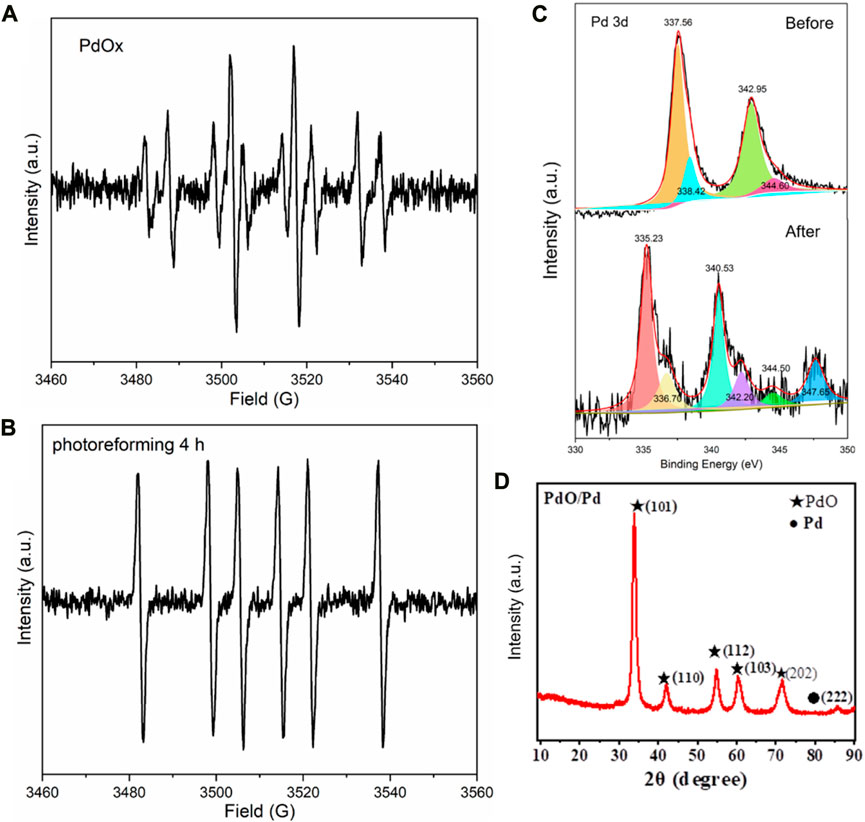
FIGURE 5. (A,B) EPR of sample B before and after illumination treatment. (C), XPS of Pd before and after illumination treatment. (D) XRD of the obtained Pd species, JCPDS of PdO: 46-1043, JCPDS of Pd: 85-0713.
According to the comparison of the XPS patterns of Pd shown in Figure 5C, the evident changes consist of two kinds of peaks: 1) Sharp peaks: from the Pd(Ⅱ) (around 337 eV) and Pd 3d3/2 (around 343 eV) to the Pd(0) (around 335 eV) and Pd 3d5/2 (around 341 eV), the Pd compound participated in the oxidation reaction. After calculation, the quantity of Pd in sharp peaks accounts for 75.9%. 2) Intermediate peak: the Pd around 347 eV illustrates its higher valence than Pd2+, the Pd compound participated in the strong reduction reaction (the main reaction) (Ohtani et al., 1993; Brun et al., 1999). After calculation, the quantity of Pd in sharp peaks accounts for 22.5%. The changes in valence and chemical state suggest the combination of oxidation and reduction. The addition of H2PdCl4 to a photocatalytic reaction mixture of primary alcohols leads to the efficient production of the corresponding ethers and alkanes and the hemiacetal intermediate results in the strong reduction and oxidation of Pd particles (Litter, 1999; Guo et al., 2023).
As the product from PR of PET, the obtained propionic acids serve as not only the basic structural units for medical chemistry but also as the main component of the bioactive compounds and conducting polymers. For decades, research on propiolic acids has attracted a lot of attention according to their triggered mechanisms: 1) acids as nucleophiles; 2) electrophilic alkynes; 3) decarboxylative coupling; 4) decarboxylative addition; 5) electrophilic acids (Chen et al., 2020). The application of propiolic acids is abundant and attractive so it is defined as a valuable chemical material. Besides, after filtration and evaporation, the residual Pd ion in the liquid was monitored to be ca. 0.17 wt% relative to the initially added Pd ion, it thus represents the recovery of 99.8% from the PdOx and Pd precipitates (Figure 5D). In summary, our research can not only provide an economical plastic degradation method but also produce valuable and necessary chemical material (Table 1).
Conclusion
In summary, we have developed an innovative plastic recycling method. By degreasing and PR processing, we establish an innovative concept of plastic degradation and reformation. PR is so robust and environmentally friendly that this method is critically important for plastic degradation and valuable chemical materials production. Remarkably, the high production of propiolic acids (59.1 wt%) and high percentage recovery of Pd (99.8%) illustrates the high value of the product and low cost of reaction. With these sustainable and widely available properties, our method can become a strong competitor for landfilling plastic. Furthermore, given the widely available industrial production equipment, large-scale continuous production of our sustainable PR method for applications as an innovative method for plastic degradation can be expected in the future.
Experimental procedures
In a typical experiment, 2.5 g PET flake (approximately 1 cm × 1 cm) was stirred in 10 M NaOH for 30 h at 60 °C followed by centrifugation to collect precipitants as substrate for subsequent experiments. The precipitate (1 g) was dissolved in 20 mL of deionized water, 20 mL of 30 mM H2PdCl4, and 10 mL of 35 wt% H2O2 was added successively. The reactor was then illuminated by a 300 W Xe lamp with AM 1.5G filter, and the output of the light was adjusted to 100 mW·cm−2 (AM 1.5G filter). After filtration, washing, and evaporation, 99.8% of Pd was recycled as the black precipitate PdOx.
Characterization techniques
Electron Paramagnetic Resonance (EPR) (BRUKER A300) was utilized to identify free radicals and hydroxyl groups. To examine hydroxyl groups and free radicals, simulated Sun irradiation was utilized.
For qualitative detection of molecular alterations in functional groups, FTIR spectroscopy (Thermo Fisher Nicolet IS 50/6700) was utilized. Using the ratio of the hydroxyl group, benzene ring, and ester group, the primary product of the reaction was estimated. Hydroxyl group is the ratio of absorbance peak regions at approximately 3,600 cm−1. The benzene ring is the ratio of absorbance peak regions at around 1600 cm−1. Ester group is the ratio of the regions beneath the absorbance maxima at around 1200 cm−1.
Under 300 °C, gas chromatography-mass spectrometry (GC-MS) (SHIMADZU QP2010ULTRA) was used to measure the spectral peak strength of ions and to separate and identify complicated components.
For analyzing the relative molecular mass of the products, a Gel Permeation Chromatograph (GPC) (WATERS ACQUITY APC) was utilized. And 1H nuclear magnetic resonance (NMR) spectra (BRUKER AVANCE III HD 400 MHz) were utilized to evaluate the interaction between spin nuclei and high magnetic fields, as well as analytical methods for detecting the molecular structure and chemical composition of samples.
The crystal structure of the materials was determined using an X-ray diffractometer (XRD) (Thermo Fisher Nexsa). Photoluminescence (PL) (Ocean Insight SR2) was utilized for the study of physical difficulties including electronic states, electronic transition processes, and electron-lattice interactions in solids. A constant 400 nm light source was used to detect the products under various situations.
Data availability statement
The original contributions presented in the study are included in the article/Supplementary Material, further inquiries can be directed to the corresponding author.
Author contributions
DL: Writing–original draft, Formal Analysis, Investigation, Methodology. HY: Conceptualization, Data curation, Formal Analysis, Funding acquisition, Investigation, Methodology, Project administration, Resources, Supervision, Validation, Visualization, Writing–original draft, Writing–review and editing. YH: Conceptualization, Data curation, Formal Analysis, Methodology, Project administration, Supervision, Writing–review and editing.
Funding
The authors declare financial support was received for the research, authorship, and/or publication of this article. This work is supported by the Key-Area Research and Development Program of Guangdong Province (Grant No. 2021B0101310003), Guangzhou Basic and Applied Basic Research Foundation (Grant Nos. 202201010440 and 2023A03J0024), Guangdong Junior Innovative Talents Project for Ordinary Universities (Grant No. 2021KQNCX018), the National Natural Science Foundation of China (Grant No. 62074060), Guangdong Basic and Applied Basic Research Foundation (Grant No. 2020B1515020032), Guangdong Basic and Applied Basic Research Foundation (Grant No. 2022A1515140064).
Conflict of interest
The authors declare that the research was conducted in the absence of any commercial or financial relationships that could be construed as a potential conflict of interest.
Publisher’s note
All claims expressed in this article are solely those of the authors and do not necessarily represent those of their affiliated organizations, or those of the publisher, the editors and the reviewers. Any product that may be evaluated in this article, or claim that may be made by its manufacturer, is not guaranteed or endorsed by the publisher.
References
Ahrens, A., Bonde, A., Sun, H., Wittig, N. K., Hammershøj, H. C. D., Batista, G. M. F., et al. (2023). Catalytic disconnection of C–O bonds in epoxy resins and composites. Nature 617, 730–737. doi:10.1038/s41586-023-05944-6
Bai, X., Wu, C., Ge, S., and Lu, Y. (2020). Pd/Cu-Catalyzed enantioselective sequential heck/sonogashira coupling: Asymmetric synthesis of oxindoles containing trifluoromethylated quaternary stereogenic centers. Angew. Chem. Int. Ed. 59, 2764–2768. doi:10.1002/anie.201913148
Bergmann, M., Collard, F., Fabres, J., Gabrielsen, G. W., Provencher, J. F., Rochman, C. M., et al. (2022). Plastic pollution in the arctic. Nat. Rev. Earth Environ. 3, 323–337. doi:10.1038/s43017-022-00279-8
Brun, M., Berthet, A., and Bertolini, J. C. (1999). XPS, AES and Auger parameter of Pd and PdO. J. Electron Spectrosc. Relat. Phenom. 104, 55–60. doi:10.1016/S0368-2048(98)00312-0
Cao, B., Wan, S., Wang, Y., Guo, H., Ou, M., and Zhong, Q. (2022). Highly-efficient visible-light-driven photocatalytic H2 evolution integrated with microplastic degradation over MXene/ZnxCd1-xS photocatalyst. J. Colloid Interface Sci. 605, 311–319. doi:10.1016/j.jcis.2021.07.113
Chen, C., Wu, J., Yan, G., and Huang, D. (2020). Recent advances of propiolic acids in organic reactions. Tetrahedron Lett. 61, 151415. doi:10.1016/j.tetlet.2019.151415
Dong, Q., Lele, A. D., Zhao, X., Li, S., Cheng, S., Wang, Y., et al. (2023). Depolymerization of plastics by means of electrified spatiotemporal heating. Nature 616, 488–494. doi:10.1038/s41586-023-05845-8
Dutta, S., Bhattacharya, T., Geffers, F. J., Bürger, M., Maiti, D., and Werz, D. B. (2022). Pd-catalysed C–H functionalisation of free carboxylic acids. Chem. Sci. 13, 2551–2573. doi:10.1039/D1SC05392B
Garcia, J. M., and Robertson, M. L. (2017). The future of plastics recycling. Science 358, 870–872. doi:10.1126/science.aaq0324
Guo, X., Wu, H., Wu, P., He, M., and Guan, Y. (2023). Efficient synthesis of bioetheric fuel additive by combining the reductive and direct etherification of furfural in one-pot over Pd nanoparticles deposited on zeolites. Green Energy & Environ. 8, 519–529. doi:10.1016/j.gee.2021.07.001
Hu, J., Yang, M., Ke, X., Yang, S., Wang, K., Huang, H., et al. (2022). Cubic-cubic perovskite quantum dots/PbS mixed dimensional materials for highly efficient CO2 reduction. J. Power Sources 461, 228838–228844. doi:10.1016/j.jpowsour.2020.228838
Ikeda, T., Xiong, A., Yoshinaga, T., Maeda, K., Domen, K., and Teranishi, T. (2013). Polyol synthesis of size-controlled Rh nanoparticles and their application to photocatalytic overall water splitting under visible light. J. Phys. Chem. C 117, 2467–2473. doi:10.1021/jp305968u
Jiao, X., Hu, Z., Wu, Y., Zheng, K., Li, L., Zhu, S., et al. (2022). Photoconverting polyethylene terephthalate into exclusive carbon dioxide by heterostructured NiO/Fe2O3 nanosheets under mild conditions. Sci. China Mater. 65, 985–991. doi:10.1007/s40843-021-1823-9
Li, X., Wang, T., Zheng, Z., Yang, Q., Li, C., and Li, B. (2021). Pd modified defective HNb3O8 with dual active sites for photocatalytic coproduction of hydrogen fuel and value-added chemicals. Appl. Catal. B Environ. 296, 120381. doi:10.1016/j.apcatb.2021.120381
Litter, M. (1999). Heterogeneous photocatalysis Transition metal ions in photocatalytic systems. Appl. Catal. B Environ. 23, 89–114. doi:10.1016/S0926-3373(99)00069-7
Liu, Y., Zhong, Q., Xu, P., Huang, H., Yang, F., Cao, M., et al. (2022). Solar thermal catalysis for sustainable and efficient polyester upcycling. Matter 5, 1305–1317. doi:10.1016/j.matt.2022.02.002
MacLeod, M., Arp, H. P. H., Tekman, M. B., and Jahnke, A. (2021). The global threat from plastic pollution. Science 373, 61–65. doi:10.1126/science.abg5433
Ohtani, B., Kakimoto, M., Nishimoto, S., and Kagiya, T. (1993). Photocatalytic reaction of neat alcohols by metal-loaded titanium(IV) oxide particles. J. Photochem. Photobiol. A Chem. 70, 265–272. doi:10.1016/1010-6030(93)85052-A
Sheridan, E. A., Fonvielle, J. A., Cottingham, S., Zhang, Y., Dittmar, T., Aldridge, D. C., et al. (2022). Plastic pollution fosters more microbial growth in lakes than natural organic matter. Nat. Commun. 13, 4175. doi:10.1038/s41467-022-31691-9
Songip, A. R., Masuda, T., Kuwahara, H., and Hashimoto, K. (1994). Kinetic studies for catalytic cracking of heavy oil from waste plastics over REY zeolite. Energy fuels. 8, 131–135. doi:10.1021/ef00043a022
Songip, A. R., Masuda, T., Kuwahara, H., and Hashimoto, K. (1993). Test to screen catalysts for reforming heavy oil from waste plastics. Appl. Catal. B Environ. 2, 153–164. doi:10.1016/0926-3373(93)80045-F
Uekert, T., Kuehnel, M. F., Wakerley, D. W., and Reisner, E. (2018). Plastic waste as a feedstock for solar-driven H2 generation. Energy Environ. Sci. 11, 2853–2857. doi:10.1039/C8EE01408F
Uheida, A., Mejía, H. G., Abdel-Rehim, M., Hamd, W., and Dutta, J. (2021). Visible light photocatalytic degradation of polypropylene microplastics in a continuous water flow system. J. Hazard. Mater. 406, 124299. doi:10.1016/j.jhazmat.2020.124299
Wang, W., Huang, H., Ke, X., Liu, X., Yang, S., Wang, K., et al. (2022). Morphology-and size-dependent FAPbBr3/WO3 Z-scheme photocatalysts for the controllable photo-oxidation of benzyl alcohol. Mater. Des. 215, 110502–110511. doi:10.1016/j.matdes.2022.110502
Yang, W.-C., Chen, X.-B., Song, K.-L., Wu, B., Gan, W.-E., Zheng, Z.-J., et al. (2021). Pd-catalyzed enantioselective tandem C–C bond activation/cacchi reaction between cyclobutanones and o-ethynylanilines. Org. Lett. 23, 1309–1314. doi:10.1021/acs.orglett.0c04297
Zhang, S., Hu, Q., Zhang, Y.-X., Guo, H., Wu, Y., Sun, M., et al. (2023). Depolymerization of polyesters by a binuclear catalyst for plastic recycling. Nat. Sustain. 6, 965–973. doi:10.1038/s41893-023-01118-4
Keywords: photoreforming, plastic recycle, PET, energy regeneration, palladium
Citation: Li DT, Yu H and Huang Y (2023) Facile H2PdCl4-induced photoreforming of insoluble PET waste for C1-C3 compound production. Front. Chem. 11:1265556. doi: 10.3389/fchem.2023.1265556
Received: 23 July 2023; Accepted: 28 August 2023;
Published: 19 September 2023.
Edited by:
Hani Nasser Abdelhamid, Assiut University, EgyptReviewed by:
Preeti Gupta, Leibniz Institute for Solid State and Materials Research Dresden (IFW Dresden), GermanyLakshmi Narayanan Mosur Saravana Murthy, Intel, United States
Copyright © 2023 Li, Yu and Huang. This is an open-access article distributed under the terms of the Creative Commons Attribution License (CC BY). The use, distribution or reproduction in other forums is permitted, provided the original author(s) and the copyright owner(s) are credited and that the original publication in this journal is cited, in accordance with accepted academic practice. No use, distribution or reproduction is permitted which does not comply with these terms.
*Correspondence: He Yu, eXVoZXl1MjU3OUAxNjMuY29t
†These authors have contributed equally to this work