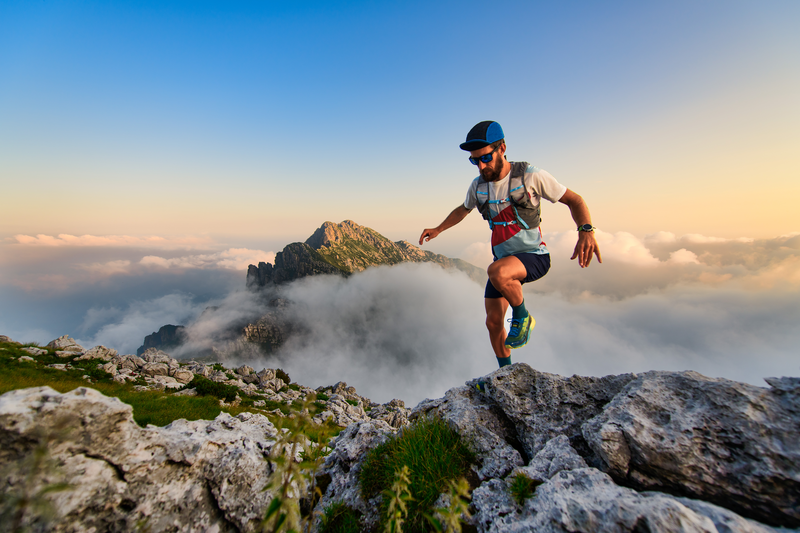
95% of researchers rate our articles as excellent or good
Learn more about the work of our research integrity team to safeguard the quality of each article we publish.
Find out more
REVIEW article
Front. Chem. , 08 August 2022
Sec. Nanoscience
Volume 10 - 2022 | https://doi.org/10.3389/fchem.2022.963004
This article is part of the Research Topic Nanotechnology for Natural Products View all 7 articles
Although medicinal natural products and their derivatives have shown promising effects in disease therapies, they usually suffer the drawbacks in low solubility and stability in the physiological environment, low delivery efficiency, side effects due to multi-targeting, and low site-specific distribution in the lesion. In this review, targeted delivery was well-guided by liposomal formulation in the aspects of preparation of functional liposomes, liposomal medicinal natural products, combined therapies, and image-guided therapy. This review is believed to provide useful guidance to enhance the targeted therapy of medicinal natural products and their derivatives.
Traditional Chinese medicine (TCM) and other herbal medicines have been used in various therapies for thousands of years (Cook et al., 2013). Especially, TCM has been accepted widely in East Asian countries (China, Korea, and Japan) and Southeast Asia countries because of many promising effects against diseases including cancer, infections, murrain, and so on. The key is a wide spectrum of bioactive natural products in the fruit, leaf, flower, root, stem, rhizome, and bark parts of medicinal plants, bacteria, and even special parts of animals, such as alkaloids, polysaccharides and terpenoids, exhibiting anti-inflammation, anti-tumor, anti-hepatic fibrosis, immunosuppression and other effects (Atanasov et al., 2021; Shao et al., 2021). Therefore, natural product has become one of the most important sources of drug discovery. However, they often suffered drawbacks that restricted their applications in disease treatment. First of all, the low solubility and stability in physicochemical environments (blood, body fluid, and low pH in digestion) and rapid blood clearance whereas it is compulsory to retain efficient drug concentration in the therapy. For those hydrophilic natural products, although the solubility is good, they were probably secreted out by the quick renal clearance (Varma et al., 2009). Second, the side effects, i.e., the potential toxicity to unwanted sites, and low bio-availability, occur when the delivery and treatment lack targeting due to multiple biological barriers, such as blood–brain barrier or blood–tumor barrier (Blanco et al., 2015). Thirdly, for the treatments, such as anti-cancer and anti-microorganism, drug-resistance to a single therapy often besets pharmacists. Finally, the modification of the molecule of natural product to solve the problems is a relatively more difficult, cost- and time-consuming task. Therefore, in clinical, suitable drug formulation emerges as an alternative solution.
As one of the first drug delivery systems, liposome formulation has been used universally in clinical trials and clinics (Gregoriadis, 1976). The nanoparticles consisting of natural/synthetic lipid, drug, additives, and surface modification moiety, have shown excellent advantages in drug delivery: 1) The physical compartment of the lipid bilayer protects the natural product and other cargos from erosion under physiological environments. It also reduces the risk of drug exposure to non-lesion sites. 2) The nano-size, good stability, and multifunctions enhance the targeted delivery and controllable drug release (Jain and Jain, 2018). The enhanced permeability and retention (EPR) effect enhances the tumor-targeting delivery of nanoliposome. Furthermore, the lipid membrane promotes cell uptake by endocytosis. 3) The big capacity for hydrophobic and hydrophilic cargoes (e.g. drug and contrast agents) is suitable for combined therapies and image-guided therapy to enhance the therapeutic effects, reduce side effects, and drug resistance. 4) The natural lipid has good biocompatibility.
In past decades, liposome has exhibited an excellent nano-platform for natural products in drug delivery. A comprehensive review is compulsory to summarize the achievements and reflect on the problems as useful guidance for future development.
In liposome, an aqueous core is entrapped by a lipid bilayer (Figure 1). Therefore, the liposome becomes an ideal carrier for hydrophobic (aqueous core) and hydrophilic (lipid bilayer) payloads, respectively. To resist the self-aggregation and body clearance mechanism, PEG modification on the surface has become a routine strategy. To realize the targeted delivery and therapy, functional modifications have been introduced to the liposome surface or embedded in the bilayer for active-targeted delivery and triggerable release.
The liposome is constructed by several strategies such as thin-film hydration, ethanol injection method (Jaafar-Maalej et al., 2010), active drug encapsulation (Gubernator, 2011). Besides typical liposome with bilayer lipid, multilayered liposomes sometimes have better stability and performance in the sustained release (Jeon et al., 2015).
Cholesterol normally plays a compulsory component to stabilize the liposome (Nakhaei et al., 2021); however, it might induce pulmonary hypertension (Szebeni et al., 2000). Ginsenoside Rh2 conjugated to liposome (Rh2-lipo), not only replaces the cholesterol and PEG to stabilize the liposome and prolong its half-life in the blood circulation, but also acts as an active-targeting ligand, and chemotherapy adjuvant (Hong et al., 2020a).
Although lipids are essential for almost all liposome construction, lipid-free liposome has also been reported, depending on the amphiphilic nature of the drug with both hydrophilic and hydrophobic groups. For example, hydrophilic resveratrol was conjugated to hydrophobic norcantharidin (a derivative of cantharidin). The resveratrol-norcantharidin liposomes were prepared by ethanol solution injection and sonication method, and then spontaneously transformed to a tadpole-like structure after 24 h due to the small hydrophobic moiety and bulky NCTD moiety (Yan et al., 2016). This formulation not only improved the drug encapsulation, but also increased the toxicity in zebrafish embryos, compared with free monomer. This strategy helps to deliver natural products by combining hydrophilic and lipophilic molecules.
The payloads of liposomes included hydrophilic or hydrophobic natural product molecules, nanoparticles, and macromolecules (Rahman et al., 2012) as sub-container of natural products.
Traditional liposomes often have limitations in the low stability in physiological environments against self-aggregation and fast body clearance, low efficiency in targeted delivery, and controllable release. Hence, besides normal lipid as the major components regulating their size, stability and rigidity, additional functional lipids are essential for various purposes, such as PEG-lipid, ligand-lipids, antibody-lipid, unsaturated lipid, and thermo-sensitive lipid.
As previously mentioned, PEGylated liposomes containing PEG-lipid are commonly used in clinical and other trials due to the increased water-solubility and the resistance to body clearance (Allen et al., 1995; Sercombe et al., 2015; Deodhar and Dash, 2018). The hydrophilic side of PEG-lipid on the surface of liposomes significantly increased the stability in water by hydration, compared with conventional liposomes which tend to aggregate in the aqueous phase. The sterically-stabilized liposomes by PEGylated modification also reduced the macrophages of the reticuloendothelial system (RES).
The activatable liposomes are capable of releasing cargoes in the lesion upon the external stimulus, such as temperature, sonic radiation, light, pH, ROS, and enzyme, which are normally responsible for site-selectively triggering the drug release.
For thermo-sensitive liposomes, the melting phase transition temperature (Tm) of the lipids is the key to inducing the collapse of liposome and drug release. An ideal Tm is above the physiological temperature (37°C) of human body, which maintains the liposome stability to avoid unwanted leakage. However, it should not be too high to avoid the liposomes to be broken. For example, a K237-modified thermos-sensitive liposomes (K237-PTX-TSL) with DPPC of Tm (41.4°C) released 72.45% of paclitaxel in 20 min (Fu et al., 2019a).
For pH-sensitive liposomes, the liposomes were destabilized and released drugs (doxorubicin or paclitaxel) upon the pH-sensitive lipid DOPE of liposomes changed under low pH (Monteiro et al., 2018; Li et al., 2019). The free amine groups on DOPE molecules would obviously change their supramolecular organization when amine groups were acidified.
For light-/ROS-sensitive liposomes, upon light irradiation, the liposomal photosensitizer Ce6 induced the generation of ROS and oxidized the unsaturated lipid (egg yolk lecithin, PC-98T) to trigger the carrier collapse and release the co-encapsulated drug triptolide (Yu et al., 2021).
For sono-sensitive liposomes, it was believed that ultrasonic induced thermal effect and mechanic stimulation to result in lipid membrane pore and therefore release the drugs (doxorubicin and others) (Patrucco and Terreno, 2020).
Over-expressing receptors are usually validated as a biomarker of many diseases, which have played very important roles in the drug delivery. The liposomes could target the lesion (e.g., tumor) specifically with the aid of antibody-antigen or ligand-receptor interactions.
Vascular endothelial growth factor (VEGF) monoclonal antibody (mAb) was conjugated to liposomes encapsulating paclitaxel, which selectively guided the delivery and accumulation in the VEGF-overexpressing tumor and release the chemotherapeutic agent within the tumor (Shi et al., 2015).
Ligand-conjugation on the surface of liposomes is another strategy for active targeting to enhance therapeutic effects by receptor-mediated endocytosis. Due to the diversities of biological activities, many natural products have shown strong affinities and specificity to the corresponding receptors, and therefore endow the liposomes with excellent recognition, such as glycyrrhetinic acid (Glycyrrhizin) (Mao et al., 2003; Mao et al., 2007; Li et al., 2012a; Tian et al., 2014; Cai et al., 2016), glycyrrhetinic acid derivative ligand 18-GA-Gly (Jin et al., 2017), glycyrrhetinic acid plus galactosylate (Chen et al., 2017), galactosylated-stearate (Li et al., 2018a), galactose (Guo et al., 2011), and folate (Chen et al., 2016; Guo et al., 2022). For example, glycyrrhetinic acid isolated from Glycyrrhiza glabra was used to modify liposome for liver targeting (Tsuji et al., 1991; Tian et al., 2014). Glycyrrhetinic acid-modified liposomes encapsulating wogonin (GA-WG-Lip) have better inhibition efficiency (IC50 2.292 μM) to HepG2, compared with unmodified liposomes WG-Lip (IC50 3.344 μM). GA-WG-Lip also improved cellular uptake and tumor recognition. The other ligands also showed good targeting and were modified on the liposome to deliver natural products, such as tLyp-1 (sequence CGNKRTR) (Jin et al., 2018), transferrin (Leto et al., 2016), and monosaccharide GalNAc with α configuration (Tn antigen) (Li et al., 2021a).
The combination of active-targeting elements is a popular approach to further enhance the delivery, e.g., antibody and tumor lineage-homing cell-penetrating peptide (Lin et al., 2018).
Liposomes are vulnerable to body clearance, while exosomes have promising advantages including low immunogenicity, high bioavailability and targeted delivery, and so on, because of the similar structure of bilayer lipid, proper nanosize, and abundant proteins/receptors on the surface (Zhao et al., 2020; Chen et al., 2022). Exosome-liposome hybrid nanoparticles delivered triptolide to cisplatin-resistant ovarian cancer (Li et al., 2022). The CD47 and signal regulatory protein alpha (SIRPα) which overexpressed on exosomes derived from tumor cells, helped the nanoparticles to escape from the clearance of the mononuclear phagocyte system (MPS). Triptolide could be directly encapsulated into SKOV3-exosomes (SK-Exos) (Liu et al., 2019).
We only picked up some typical examples from lots of liposomal natural products for the demo (Table 1).
Artemisinin, isolated from Artemisia annua L., was discovered in 1972 to treat malaria by Youyou Tu who was awarded the Nobel Prize in physiology or medicine (Figure 2) (Isacchi et al., 2011a; Memvanga and Nkanga, 2021). It also showed activities in chemotherapeutic drug for tumors and lupus erythematosus (Mu and Wang, 2018). The sensitive endoperoxide bridge is believed to be essential for its antimalarial activity. However, this drug always suffers problems in low stability, poor water-solubility, short-duration effect, short half-life and high first-pass metabolism, and so on. Compared with free drugs, both liposomal artemisinin (conventional (A-L) and PEGylated (A-PEGL) liposomes), obviously increased the pharmacokinetic parameters, such as the peak concentration (Cmax), the area under the plasma concentration versus the time curve (AUC0–24h), and half-life (t1/2β) (Isacchi et al., 2011a). Two pH-responsive artemisinin derivatives, ADP109 and AMPm109 were conjugated to piperazine moiety (Zhang et al., 2013a). Due to the modification, their water-solubility was enhanced at acidic pH, which is responsible for the site-specific drug release from liposomes. Liposomal artemisinin also improved its anti-leishmanial potential, compared to its free form (Y. Want et al., 2017).
Polyphenolic compounds are very common secondary metabolites in plant against ultraviolet radiation and pathogens (Figure 3) (Pandey and Rizvi, 2009). For example, resveratrol liposomes showed protective effects on mitochondria in substantia nigra cells of parkinsonized rats (Chen et al., 2021). Liposomal honokiol has shown various biological activities (Niu and Gao, 2009; Guillermo-Lagae et al., 2017; Ong et al., 2020; Li et al., 2021b; Li et al., 2021c). Liposomal honokiol significantly suppressed Lewis lung carcinoma overexpressing VEGF-D by inhibiting the tumor-associated lymphangiogenesis and metastasis (Wen et al., 2009). In 2017, Zhao et al. comprehensively reviewed a variety of liposomal formations of curcumin and its bio-application, which demonstrated its limitation in the poor water-solubility, low bioavailability and rapid body clearance and how liposome formulation significantly improved the inhibition of cancer cells (Rahman et al., 2012; Feng et al., 2017). The vitamin E TPGS-conjugated liposome formulation of poor soluble bisdemethoxycurcumin (DBMC) has significantly enhanced its stability, solubility, and bioavailability (Wang et al., 2021).
Lactones are cyclic carboxylic esters, typically with 5- or 6-membrane-ring, making them very active to be hydrolyzed or attacked by nucleophiles (Figure 4). This poor stability makes it difficult to tolerate physiological environments. Camptothecin (CPT) isolated from Camptotheca acuminata (Camptotheca, Happy tree) (Wall et al., 1966), which used in TCM or Chonemorpha fragrans, commonly used in Ayurveda (an Indian root medicine) (Govindachari and Viswanathan, 1972), has been used to treat tumor as a topoisomerase inhibitor. Its lactone form is active while the hydrolyzed/ring-opening form is inactive. To improve its poor aqueous solubility, and avoid hydrolysis or protein interactions, CPT was encapsulated into liposomes, as well as its other analogs, such as topotecan, irinotecan, lurtotecan, and belotecan (Emerson, 2000; Flaten et al., 2013). For example, liposomal topotecan was used to treat advanced malignancies and neuroblastoma (Zamboni et al., 2009; Chernov et al., 2017). n-Butylidenephthalide (BP) isolated from Angelica sinensis was encapsulated into a polycationic liposome for the treatment of B16/F10 melanoma cells (Gao et al., 2018). 3,5-Dipentadecyloxybenzamidine hydrochloride (TRX-20)-modified liposomes encapsulating triptolide significantly improved the anti-inflammatory effects in the membranous nephropathic model (Yuan et al., 2017). Due to the scaffold of α-methylene-γ-lactone, parthenolide has high electrophilicity to alkylate proteins and thus processes various bioactivities (Jin et al., 2018; An et al., 2022). pH-sensitive liposomes PTL-Lips encapsulating parthenolide have enhanced its antitumor efficacy (Gao et al., 2020a).
Terpenoids are derived from a number of isoprenes and possess functional groups (at least -OH), classified as monoterpene, diterpenoids, sesquiterpenoid, triterpenoids, and so on (Figure 5) (Ludwiczuk et al., 2017). Generally, they are hydrophobic, which means low water solubility. Borneol, a bicyclic monoterpene, isolated from Dryobalanops aromatica Gaertn f. and Blumea balsamifera DC, has been used as a messenger drug encapsulated in liposomes to help other drugs penetrate physiological barriers, such as blood-brain barrier (Zhang et al., 2020; Li et al., 2021d). Galactosylated-stearate (Gal-s) modified liposome enhanced the targeted delivery of curcumol and the treatment of liver cancer cells (Li et al., 2018a). Liposomal ganoderic acid has shown better antitumor effects by inhibiting various signaling pathways (Rahman et al., 2019). Liposomal formulation of betulinic acid enhanced the antitumor effects and reduced systemic toxicity (Mullauer et al., 2011; Zhang et al., 2016). Taxanes with a tetracyclic diterpene, including paclitaxel (Taxol®) and its synthetic analog docetaxel (Taxotere®) have shown antitumor activity by inhibition of microtubules and cell division. The temperature-sensitive liposomes (PTX-TSL) modified with K237 peptide enhanced the cellular uptake and cytotoxicity of paclitaxel against cancer cells and endothelial cells (Fu et al., 2019a; Li et al., 2021a). N-acetylgalactosamine modified liposome (Tn-Lipo-PTX) improved the targeted cytotoxicity to HepG2 cells. Docetaxel (Taxotere) was specifically delivered to hepatocytes by glycyrrhetinic acid-modified liposomes via receptor-mediated endocytosis (Li et al., 2012a). Glycoside normally contained a sugar bound to another functional group via a glycosidic bond (Li et al., 2018b). For example, ginsenoside (triterpenoid glycoside), isolated from genus Panax (ginseng), a class of triterpene glycosides (Hong et al., 2019). The liposomes Rh2-lipo derived from ginsenoside Rh2 have shown multifunction in drug delivery, compared with conventional liposomes (Hong et al., 2020b).
Natural alkaloids normally are basic, contain at least a nitrogen atom, and exhibit diversified biological activity as the secondary metabolites (Figure 6). Their free base form normally has poor water solubility, while their salts are soluble in water (Kukula-Koch et al., 2017). Morphine, isolated from Papaver somniferum, has been universally used to ease the pain. However, it is highly addictive because the high concentration accesses the central nervous system, which normally occurs when the free form is administrated. Liposome formulations prolonged the analgesic effect and reduce addiction (Gómez-Murcia et al., 2019). The formulation also significantly enhanced antitransit effects of morphine (Pol et al., 1996). Matrine, isolated from Sophora flavescens, used to treat hepatic diseases, has shown anti-tumor activity with fewer side effects, compared with other chemotherapeutic drugs. However, its low efficiency of tumor-targeting delivery and moderate anti-cancer activity hindered its further application. Liposomal matrine inhibited the growth of brain glioma (Han et al., 2014). The RGD-modified liposomes loading matrine (RGD-M-LCL) significantly improved the tumor-specificity and suppressed the proliferation of Bcap-37, HT-29 and A375 cells, compared with the free matrine (Liu et al., 2010). Vincristine, an antitumor natural alkaloid isolated from Catharanthus roseus, was encapsulated in liposomes (Marqibo®) (Pathak et al., 2014). A ganglioside GM1 incorporated liposome dramatically prolonged the retention time of vincristine and therefore improved the therapeutic effects on the mice bearing P388 tumor (Boman et al., 1994).
Flavonoids share the backbone of 2-phenyl-1,4-benzopyrone, which normally exhibit fluorescence under UV irradiation and poor solubility in water (Figure 7) (Panche et al., 2016). Liposomal quercetin significantly improved the accumulations in tumor and circulation time, and inhibited tumor growth (Yuan et al., 2006). The antioxidant quercetin encapsulated in liposomes also showed the potential to reduce the oxidative damage to hepatic tissue caused by CCl4 (Mandal and Das, 2005). Breviscapine (Scutellarin as the main component), isolated from TCM Erigeron breviscapus, was extensively used to treat ischemic cerebrovascular and cardiovascular diseases in China, however, it suffered short circulation time (Zhong et al., 2005; Zhou et al., 2014). The drug durations both in vitro and in vivo were significantly prolonged by multivesicular liposome formulation (Zhong et al., 2005). Compared with traditional liposomes releasing 80% of payload within only 4 h in vitro, multivesicular liposomes have shown a better sustained-delivery system for breviscapine which extended the period to 5–6 days. Baicalin (Chen et al., 2016; Xiang et al., 2020) has poor solubility (91 μg/ml) in water at room temperature and oral bioavailability (2.2%). The folate-conjugated liposome-encapsulated baicalin has improved its cytotoxicity and cell uptake. Wogonin (WG), isolated from Scutellaria baicalensis Georgi as a TCM treating inflammation, has been explored with wide-spectrum therapeutic effects, such as anti-tumor, anti-oxidant, anti-hepatitis B virus, anticonvulsant and neuroprotective effects (Tian et al., 2014). The wogonin liposome (WG-Lip) significantly improved the bio-distributions, compared with wogonin solution. The glycyrrhetinic acid-modified liposomes encapsulated wogonin (GA-WG-Lip) further improved targeted delivery. The cytotoxicity test against HepG2 cells and in vivo anti-tumor efficacy also reflected the tendency.
Anthracyclines have a tetracyclic core with an anthraquinone backbone connected to a sugar moiety by a glycosidic linkage (Figure 8). They generally exhibit various anti-cancer effects by inhibiting topoisomerase II and the synthesis of DNA and RNA. For example, daunorubicin, and doxorubicin, isolated from bacteria of the Streptomyces type, were encapsulated in liposomes (DaunoXome™ and Doxil®) for cancer chemotherapy (Schmidt et al., 1998; Piccaluga et al., 2002; Abraham et al., 2005; Waterhouse et al., 2001; O'Byrne et al., 2002). Liposomal formulation enhanced the circulation longevity of idarubicin to improve antitumor activity (Dos Santos et al., 2005). Temperature-sensitive liposomes t-L encapsulating juglone guided the targeted therapy of HepG2 cancer cells when they were exposed to hyperthermia and released juglone (Zhao et al., 2016). Salvianolic acid B (Sal B) from Salvia miltiorrhiza Bge exhibits a strong antioxidant and free radical-scavenging activity to relieve oxidative stress (Isacchi et al., 2011b; Lin et al., 2014). The water-soluble compound, sensitive to UV irradiation and aqueous solutions, also suffers the limitations in poor instability and oral bio-availability. Compared with free and conventional liposomal Sal B, the PEGylated liposomes improved the anti-hyperalgesic effect for a prolonged time.
Polysaccharides are polymeric carbohydrates composed of repeating monosaccharide units linked by glycosidic bond, from linear to highly branched polymeric. Liposomal ophiopogon polysaccharide (OP) from Radix Ophiopogonis Japonici (a TCM), significantly improved the immune-enhancing activity of OP on Kupffer cells (Fan et al., 2014) and non-specific/specific immune response in chickens (Fan et al., 2015). The liposomes also activated mouse peritoneal macrophages (Sun et al., 2016). Liposomal OP improved anti-oxidative and immunological activities, compared with free form (Fan et al., 2016). Liposome formulation also improved lycium barbarum polysaccharides (LBP) (Bo et al., 2017) and rehmannia glutinosa polysaccharide (RGP) (Huang et al., 2016) as antigens for vaccine development. The liposomal heparin spraygel demonstrated comparable efficacy in the treatment of superficial venous thrombosis (Pleban et al., 2008). The liposomal epimedium polysaccharide (EPS) assisted in significantly improving the immune response to the Newcastle disease vaccine (Gao et al., 2012).
Bioactive natural peptides play vital roles in many cellular bioactivities and therefore are used to treat many diseases (Lau and Dunn, 2018). However, it also has limitations in low oral bioavailability and short half-life in plasma. Liposomal formulation was developed for oral administration of insulin (Değim et al., 2006). Hirudin, isolated from Hirudo spp., is a naturally occurring peptide and is therefore used as an anticoagulant to treat many diseases, e.g., diabetic nephropathy (DN). However, the lack of lesion targeting may result in severe side effects, such as hemorrhaging. The liposome significantly enhanced the renal targeting delivery and accumulations, compared with free hirudin, which demonstrated the relief of the renal injury in the diabetic nephropathy rat model (Wang et al., 2019).
Porphyrinoids represent a group of macrocyclic tetrapyrrolic compounds with good photosensitivity, near-infrared (NIR) absorption and high fluorescent quantum yield, which grants the applications in photodynamic therapy (PDT), fluorescent imaging, and photothermal therapy (PTT) (Figure 9) (Liu et al., 2014). To solve their problems in poor water solubility and low stability in physiological environments, liposomal formulation significantly improved the delivery and uptake (Cheng et al., 2021). The lipid medium also disperses them to reduce their aggregation and self-quenching for better photo-sensitivity. Pyropheophorbide acid (PPa), a degraded product of chlorophyll, has been encapsulated into liposome for tumor PDT (Zhou et al., 2019). Under 690 nm-laser irradiation, liposomal PPa significantly suppressed tumor growth. The formulation of protoporphyrin IX (PpIX) improved its internalization and bio-distribution in HeLa cells (Przybylo et al., 2016). The liposomes have significantly improved the water solubility of lipophilic chlorophyll and its NIR fluorescence in sentinel lymph node mapping (Chu et al., 2012).
Betainylated cholesterol (BC), Egg phosphatidylcholine (PC), Phospholipon 90G (P90G), Polyethylene glycol (PEG2000), L-a-Phosphatidylcholine extracted from eggs (EPC), phosphatidylglycerol (PG), Polyethylene glycol complex (LPPC), 1,2-Dipalmitoyl-sn-glycero-3-phosphocholine (MPPC), 1,2-Distearoyl-sn-glycero-3-phospho-(10-rac-glycerol) (DSPG), 1-Myristoyl-2-palmitoyl-sn-glycero-3-phosphatidylcholine (MSPC), 1,2-distearoyl -sn-glycero-3-phosphoethanolamine-N-[PEG(2000)] (DSPE-mPEG2000), Malei-(ethylene glycol)]-1,2-dioleoyl-sn-glycero-3-phosphoethanolamine (DSPE-PEG-MAL), Dipalmitoylphosphatidylcholine (DPPC), Cholesterol (CHOL), 3-Succinyl-30-stearyl glycyrrhetinic acid (18-GA-Suc), Cholesteryl hemisuccinate (CHEMS), Hydrogenated soybean phosphatidylcholine (HSPC), Egg yolk lecithin (EYPC), Distearoyl phosphatidylcholine (DSPC), Monomethoxy polyethyleneglycol 2000-distearoyl phosphatidylethanolamine (mPEG-DSPE), Hydrogenated soy phosphatidylcholine (HSPC), Methoxy polyethylene glycol (MPEG), Distearoyl phosphatidylethanolamine (DSPE)
Owing to the multi-components of natural products, TCM is believed to treat disease by multi-targets interactions, which is especially suitable for drug-resistant therapy. However, it is difficult to clearly and comprehensively demonstrate the key concerns of drug discovery, such as pharmacokinetics and side effects, although many signs of progress have been made to analyze their components, biological targets, metabolites, and signal transduction by applications of modern analytic methods for enzyme, gene and the interactions between them (Su et al., 2007). Although a single drug molecule with clear structure and pharmacokinetics is the mainstream of modern drug discovery, TCM or other herbal medicines containing a complicated mixture of natural products with unclear components and mechanism, have obtained many surprising achievements in various therapies for a long time, which are really hard to refuse.
Thus, to minimize the possible risks and maximize the therapeutic effects, liposomal formulation of the extracts or ingredients has been applied (Bilia et al., 2018). The extract of schisandra chinensis fructus (SCF, a TCM) from Schisandra chinensis (Turcz.) Baill was encapsulated in β-cyclodextrin (β-CD) and then liposome. By analyzing three major bioactive lignans (Schisandra lignans: Schisandrin (SD), schisantherin (ST), and c-schizandrin (SZ)), this formulation enhanced liver uptake (Ding et al., 2019). The liposomal formulation of Aphanamixis polystachya leaf extract showed better performance in the behavior of mice dementia model than the extract (Shariare et al., 2020). The liposomes have high encapsulation efficiency of poor water-soluble pollen extract and therefore improved the bioaccessibility (Hızır-Kadı et al., 2020). Using unpurified soybean phospholipids, the nano-liposomes encapsulating Orthosiphon stamineus ethanolic extract significantly improved the intestinal absorption and anti-oxidation effects (Aisha et al., 2014).
Due to the flexibility in the surface modifications and big capacity for hydrophobic and hydrophilic cargoes, liposome has proved to be an excellent multifunctional platform for combined therapies and image-guided therapy. It may further improve the systematic therapeutic effects, compared with monotherapy by the medicinal natural products alone.
Single molecular treatment usually has a maximally tolerated dose, which probably limits the therapeutic effects. Furthermore, to survive against the treatment, cancer or pathogen has high probability to develop resistance as an “acquired” ability by the fast drug-clearance response and self-evolution, which usually occurs in single therapy, such as chemotherapy (Vasan et al., 2019), radiotherapy (Willers et al., 2013), immunotherapy (Bai et al., 2020), PDT (Casas et al., 2011), and so on. It is worthy to mention that resistant cancer or pathogen will become more aggressive and refractory. To solve the problems, combined therapy has shown promising therapeutic effects. The different drugs in the co-delivery liposomal system could simultaneously and synergistically block the distinct pathways involved in the survival of the targets, which makes the targeting cancer or pathogen more sensitive to the treatments. Therefore, the dose and side-toxicity of individual drugs might be reduced, as well as the possibility of drug resistance due to less exposure to the drugs.
The chemotherapeutic drug suppresses cell proliferation and induces cell death by inhibiting cell survival activities. Combined chemotherapies obviously enhance the therapeutic effects and defeat drug resistance.
To treat drug-resistant malaria infection, artemisinin was co-encapsulated with curcumin into a liposomal system (Isacchi et al., 2012). In another case, stearylamine liposomal monensin was combined with free artemisinin to afford enhanced antimalarial effects which were significantly affected by stearylamine and length of PEG-lipids (Rajendran et al., 2016).
When ginsenosides (Rh2, Rg3, and Rg5) was combined with paclitaxel (PTX), ginsenoside functioned as not only a chemotherapy adjuvant but also a functional membrane material to stabilize liposome and assist active-targeting (Hong et al., 2019). This multifunctional additive helped liposomal system to offer a novel platform for drug delivery.
Paclitaxel was encapsulated in thermosensitive liposomes, and modified with a therapeutic peptide K237 (sequence: HTMYYHHYQHHL) which inhibited the bonding between VEGF and KDR, and the proliferation of human endothelial cells (Fu et al., 2019a). The combined therapy of paclitaxel and k237 enhanced cell uptake and cytotoxicity against cancer cells and endothelial cells.
tLyp-1-conjugated liposomes encapsulating parthenolide and ginsenoside CK enhanced the antitumor activity and reduced the side effects, compared with individual drugs (Jin et al., 2018).
Cisplatin is a first-line chemotherapeutic drug for cancer therapy. However, it is highly debatable because of the severe side effects/toxicity and drug resistance. In the combined therapy of EMT-6 and B16F10 tumor models, although less dose of free cisplatin, liposomal curcumin has improved the antitumor effects and reduced toxicity (Hamano et al., 2019). The combination of liposomal honokiol with cisplatin improved antitumor activity by enhanced induction of apoptosis and inhibition of angiogenesis in A549 lung cancer xenograft model (Jiang et al., 2008). The combination has also been applied to cisplatin-sensitive (A2780s) and -resistant (A2780cp) human ovarian cancer models, which showed significant inhibition (84–88%) and prolonged the survival life (Luo et al., 2008).
A synergistic therapy of lung cancer treatment was achieved by a cocktail of betulinic acid, parthenolide, honokiol, and ginsenoside Rh2 in a liposomal delivery system (Jin et al., 2020). Additionally, the combined therapies were relatively safer, compared with the treatment by cisplatin which exhibited obvious kidney damage.
Although the combination of daunorubicin and cytarabine was the standard for acute myeloid leukemia (AML) in the past decades, a liposomal formulation (CPX-351, Vyxeos™) of the combination was newly approved by the US Food and Drug Administration (FDA) for therapy-related acute myeloid leukemia (t-AML) or AML with myelodysplasia-related changes (AML-MRC), which improved the effects to the subgroups with the reason unclear (Chen et al., 2018).
Liposome co-delivered not only the cytotoxic natural products with other drugs for anti-proliferation (e.g., anti-tumor, anti-malaria, and so on), but also natural products for promoting proliferation. For example, due to the roles in promoting endothelial cell repair and capillary blood circulation, liposomal heparin was further combined with ibuprofen in “nano-spray gel” which exhibited significant effects in wound healing for frostbite (Vaghasiya et al., 2019).
Liposomes improved the targeted delivery and protected the unstable miRNA from the degradation induced by physiological environments and RNA enzymes (Rupaimoole and Slack, 2017; Fu et al., 2019b).
Bioinspired nanoparticles hybridizing CD47-expressing exosomes and cRGD-modified liposomes, encapsulated triptolide and miR497 to treat cisplatin-resistant ovarian cancer (Figure 10) (Li et al., 2022). After the NPs were delivered and accumulated in tumor cells by cRGD guidance and EPR effects, the acid-sensitive carriers encapsulating calcium phosphate (CaP), released the cargoes in the low pH tumor microenvironments. Meanwhile, CD47 helped the carrier to escape from the immune attack. The released triptolide and miR497 initiated the cancer cell apoptosis by multiple pathways. Firstly, triptolide efficiently promoted the polarization of M2 macrophages to M1 form. Secondly, triptolide upregulated cellular GSH and down-regulated ROS. Thirdly, it was believed that triptolide and miR497 synergistically inhibited PI3K/AKT/mTOR signaling pathway. In general, the combined therapies improved the antitumor effects and defeated the drug resistance.
FIGURE 10. The preparation of miR497/TP-HENPs and the mechanism of combined therapies: chemotherapy with miRNA therapy. Triptolide (TP).
In presence of light, oxygen and photosensitizer, PDT damages the targets with reactive oxygen species (ROS), exhibiting distinct advantages in almost non-invasion, low dark toxicity and low drug-resistance. Liposome has shown the excellent ability to combine PDT with chemotherapy and other therapies (Cheng et al., 2021).
A photosensitive liposomal system TP/Ce6-LP, encapsulating natural product triptolide as a chemotherapeutic agent and Ce6 as a photosensitizer, was applied for hepatocellular carcinoma therapy (Figure 11) (Yu et al., 2021). Firstly, upon NIR irradiation (650 nm laser), Ce6 converted oxygen to ROS (1O2) to photo-oxidize the unsaturated lipid egg yolk lecithin (PC-98T) and then induced the structure collapse of liposome, which was responsible for the photo-activatable release of triptolide. Second, ROS also executed PDT to cooperate with chemotherapy. Third, the formulation increased the water solubility of triptolide, and avoided leakage in the delivery, and enhanced the tumor accumulation by EPR effect and laser focusing. Therefore, the system TP/Ce6-LP greatly improved the antitumor efficacy and notably reduced its system toxicity.
FIGURE 11. The preparation of TP/Ce6-LP and the mechanism of combined therapies: chemotherapy with PDT.
By converting light energy to heat energy, PTT increases the target’s temperature to induce cell death, with non-invasion and non-resistance. This therapy relies on the distributions, conversion efficacy, and absorbed wavelength of the photothermal agent. However, hyperthermia therapy replaces the photothermal agent and light irradiation with an external heating instrument. Thermosensitive liposomal system was often fabricated as a drug-release platform by incorporation of special lipid with a suitable phase transition temperature (Tc) above the physiological temperature 37°C.
Nanomagnetic liposomal system ICG-PTL-Lips@MNPs encapsulated parthenolide as a chemotherapeutic agent, indocyanine green (ICG) as a photothermal agent and magnetic Fe3O4 nanoparticles for the targeted and chemo-PTT (Figure 12) (Gao et al., 2020a). First, the magnetic liposomes were directed to tumor sites by an external magnetic field. Second, laser irradiation (808 nm) promoted the system to release the cargoes by photothermal effect. Third, the synergistic combination of chemotherapy and PTT enhanced the antitumor efficacy and reduced the side effects.
FIGURE 12. The preparation of ICG-PTL-Lips@MNPs and the mechanism of combined therapies: chemotherapy with PTT.
Thermosensitive liposomes (F7-TPT-TSL) co-loading two therapeutic agents topotecan (TPT) and F7 were fabricated with DPPC (Tc 41°C) and other lipids for the combined therapies (Figure 13) (Du et al., 2020). After administration of the liposomes and delivery to tumors, the tumor was heated for 30 min with a 42.5°C copper column to induce the drug release. The system significantly improved the antitumor effects and reduced systemic toxicity.
FIGURE 13. The preparation of F7-TPT-TSL and the mechanism of combined therapies: dual chemotherapies with hyperthermia therapy.
As one of the traditional antitumor therapies in the clinic, radiotherapy adopts a high dose of radiation to damage the DNA of cancer cells, leading to killing or shrinking tumors. However, it is hard to treat metastatic foci which is normally too tiny to be detected. The damage to surrounding normal tissue is unavoidable.
In the combined therapies, liposomal honokiol as a chemotherapeutic agent sensitized the tumor cells to radiotherapy (Hu et al., 2008). The combined therapies delayed the tumor growth (8.7 days) and significantly improved the survival time, compared with single treatments.
Multifunctional liposomes GOD-PTL-Lips@NMPs encapsulating parthenolide and glucose oxidase, were modified with Fe3O4 magnetic nanoparticles and chitosan (a linear polysaccharide) for combined therapies (Figure 14) (Gao et al., 2020b). First, the nanoparticles were delivered to tumor tissue by EPR effects and magnetic targeting. Second, after cellular uptake, due to the protonation of -NH2 group, chitosan induced the liposomes to collapse and release the cargoes. Third, Fe3O4 magnetic nanoparticles not only guided the delivery by the magnetic field, but also catalyzed the Fenton reaction for CDT. Fourthly, glucose oxidase (GOD) consumed glucose to starve the targeted cells and generated H2O2 to enhance CDT. Fifthly, parthenolide as a chemotherapeutic agent not only induced cell apoptosis, but also consumed GSH to enhance CDT.
FIGURE 14. The preparation of GOD-PTL-Lips@MNPs and the mechanism of combined therapies: chemotherapy with starvation and CDT.
Personalized medicine required that the treatment was performed according to the personalities of the patients. By co-delivery of therapeutic and imaging contrast agents, multifunctional liposome as a theranostic platform allowed various diagnostic tests to guide therapy and evaluate the therapeutic outcome. As a review recently summarized, liposome-based imaging approaches have visualized the fate of liposomes in vivo, such as computed tomography (CT), magnetic resonance imaging (MRI), positron emission tomography (PET) imaging, photoacoustic imaging (PAI), and FLI (Xia et al., 2019).
FLI has high sensitivity and resolution. Long wavelengths of dye’s absorption and emission, such NIR region, could significantly improve tissue penetration. Before encapsulated in liposomes, a fluorescent probe derived from paclitaxel (PTX) by chemically conjugation to a NIR fluorescent dye DiR (Figure 15A) (Shi et al., 2015). DiR-labeled liposomes modified with ginsenosides, clearly visualized their in vivo distributions at different time points in BGC-823 tumor-bearing mice (Figure 15A) (Hong et al., 2019). The images showed that ginsenosides had guided the liposomes encapsulating paclitaxel (PTX) to tumor site, compared C-lipo group with Rh2-, Rg3-, and Rg5-lipo groups (Figures 15A,B).
FIGURE 15. (A) Imaging contrast agents. (B) FLI of BGC-823 tumor-bearing mice after i.v. injection of DiR-labeled C-lipo and ginsenoside liposomes (C-lipo, Rh2-lipo, Rg3-lipo, and Rg5-lipo). Reproduced (Adapted) under the terms of the Creative Commons Attribution (CC BY-NC 4.0) (Hong et al., 2019). Copyright 2019, Theranostics. (C) SPECT imaging of SCCHN tumor-bearing nude rats after i.v. injection of 99mTc-Gd-liposomes. Reprinted (adapted) with permission (Li et al., 2012b). Copyright 2012, American Chemical Society. (D) MR images of after i.v. injection of sonosensitive gadoteridol- and doxorubicin-loaded liposomes. Reproduced (Adapted) under Creative Commons Attribution License (CC BY) (Shi et al., 2015). Copyright 2020, Patrucco and Terreno; (E) PA images after i.v. injection of free ICG and DOX@GdMSNs-ICG-TSLs. Reprinted (adapted) with permission (Sun et al., 2018). Copyright 2018, American Chemical Society. (F) PET Images of 4T1 tumor-bearing mice with low 89Zr-NRep uptake (mouse A, left) and high 89Zr-NRep uptake (mouse B, right) after i.v. injection. Reproduced (Adapted) under Creative Commons Attribution License (CC BY) (Pérez-Medina et al., 2016). Copyright 2016, Mulder and Reiner et al. (G), PET-CT imaging of a B6CBAF1 mouse after i.v. injection of [52Mn]Mn-DOXIL. Reproduced (Adapted) with permission (Gawne et al., 2018). Copyright 2018, Royal Society of Chemistry. The yellow arrow indicated tumors. Red arrowhead indicated the outside reference standard.
Single-photon emission computed tomography (SPECT) reconstructs the 3D information of the target by detecting gamma rays from radionuclide. 111In-labeled liposomes encapsulating 111In and CPT were delivered to an HT-29 tumor-bearing mouse (Figure 15A) (Flaten et al., 2013). The combination of SPECT images showed that the liposomes mainly accumulated in the liver at 2 h postinjection and the intestine slowly developed to be the second-highest site during 20 h. In another case, SPECT image showed a high accumulation of 99mTc-Gd-liposomes in the tumor at 44 h post-injection (Figures 15A,C) (Li et al., 2012b).
MRI is a popular technique for medical checking imaging in the clinic by detecting protons in water and fat molecules in the body. To improve the sensitivity, contrast agents are often administrated. A temperature-sensitive liposome-encapsulated doxorubicin and gadoteridol [Gd(HPDO3A)(H2O)] as a paramagnetic T1 contrast agent of MRI (Figure 15A) (de Smet et al., 2010). MRI also monitored the release process of doxorubicin and gadoteridol in tumor from thermal- and sono-sensitive liposomes, and the therapeutic outcome (Figures 15A,D) (Patrucco and Terreno, 2020). In an earlier study, MRI was applied to the therapy by liposomes encapsulating doxorubicin and an old contrast agent MnSO4 (Viglianti et al., 2004). In another case, Fe3O4 nanoparticles served as T2 contrast agents of MRI and were encapsulated in liposomes to visualize the combined therapies of parthenolide and other therapeutic agents (Gao et al., 2020b).
PA imaging is a non-toxic, non-invasive technique with excellent penetration and high spatial resolution, by converting light into heat and acoustic signal. PA imaging visualized the bio-distributions of liposomes encapsulating ICG as photothermal agent and doxorubicin, and the synergistic therapeutic effects of chemotherapy and PDT (Figures 15A,E) (Sun et al., 2018).
Currently, PET is the only functional imaging technique to afford metabolic information which is extremely suitable for tumor and metastases diagnosis, while others only offer anatomic information. It relies on the tracer containing the positron-emitting radioisotopes, such as 18F, 52Mn, 64Cu, 89Zr and so on. 89Zr-labeled liposomes facilitated the quantification of the doxorubicin accumulation in tumors (Figures 15A,F) (Pérez-Medina et al., 2016).
Compared with single imaging, dual-modal imaging has extra advantages. For example, due to the limitation in only metabolic information, PET is normally combined with MRI and CT for anatomic information in clinic. PET-MRI clearly visualized the location, morphology, and activity of the tumor before and after treatment with liposomal doxorubicin (Figure 15A) (Zimmermann et al., 2017). 52Mn-labeled Doxil® revealed the long circulation and distributions in organs by PET-CT (Figures 15A,G) (Gawne et al., 2018) as well as 64Cu-liposome (Lee et al., 2018). Similarly, SPECT-CT also offered the information on distributions of liposomal doxorubicin and liposomal vinorelbine, and validated the absence of competing effect (Wu et al., 2017). 188Re-labeled nanoliposomes visualized the treatment of doxorubicin in human colorectal adenocarcinoma-bearing mice by SPECT-CT (Chen et al., 2010).
The tunable size, biocompatible nature, triggerable cargo release, surface modifications for targeted delivery, and physical compartment make the functional liposome as an ideal carrier for natural products and their analogs with higher efficiency and less toxicity.
More and more natural products are isolated and identified with abundant biological activities, due to biological evolutions for survival advantage. Many of them have the potential to be therapeutic agents, which can be encapsulated into multifunctional liposomes for better therapeutic effects.
TCM has many positive results in therapy, although the mechanism of most TCM still remained unclear, such as upregulation of tumor-related T cells (Hoffman et al., 2020). To reduce unexpected side effects, liposomal formulation is a good option.
Liposome not only delivers nature products but also biomacromolecules, such as DNA (Guo et al., 2018), RNA (Hou et al., 2021), protein (Swaminathan and Ehrhardt, 2012), and bacteriophages (Nieth et al., 2015) for therapies. Therefore, it is the potential to improve the therapeutic effects by combined therapy in cooperation with various drugs based on different mechanisms.
Overall, the liposomal system still suffered limited efficiency in targeted delivery to the lesion. For example, developing new techniques to help the liposomal delivery to overcome biological barriers, such as blood-brain barrier and blood-tumor barrier, are also challenges in drug delivery. On one hand, new materials such as cancer membrane, synthetic lipid, blood cell membrane, have exhibited promising advantages in better biocompatibility and targeted delivery. Furthermore, engineering strategy was applied to construct an intelligent platform, such as a liposome-based robot (Inaba et al., 2018; Shoji and Kawano, 2020), bacterial motors (Zhang et al., 2013b; Dogra et al., 2016) and so on. On the other hand, physical tool, such as focused ultrasound, has improved the delivery efficiency of liposomal paclitaxel to mice brain and tumor (Shen et al., 2017).
Although liposome-based molecular imaging has experienced explosive development (Xia et al., 2019), such as FLI, MRI, CT, PET, ultrasound imaging and PA imaging, developing new imaging agents suitable for liposome-labeling, will further promote medicinal nature products-involved image-guided therapy.
In general, liposomal formulation has experienced fast developments in targeted therapy, biocompatibility, safety, and so on. Undoubtedly, as a carrier of medicinal natural products and their derivatives, it plays a very important role in the drug formulation and will significantly promote drug discovery.
XC conceived and wrote the manuscript. HY, SP, MY, and FQ drew the figures. PQ, CZ, and YL revised and reviewed the article. All authors contributed to the manuscript and approved the submitted version.
This work was supported by Standardized Construction of Chinese Medicine Rehabilitation Service Capacity (Grant No. 2019GJZGJ01-01), the Zhejiang Provincial Administration of Traditional Chinese Medicine (Grant No. 2020ZB131), and Nanjing Tech University (Start-up Grant Nos. 38274017101 and 3827401742).
The authors declare that the research was conducted in the absence of any commercial or financial relationships that could be construed as a potential conflict of interest.
All claims expressed in this article are solely those of the authors and do not necessarily represent those of their affiliated organizations, or those of the publisher, the editors, and the reviewers. Any product that may be evaluated in this article, or claim that may be made by its manufacturer, is not guaranteed or endorsed by the publisher.
Abraham, S. A., Waterhouse, D. N., Mayer, L. D., Cullis, P. R., Madden, T. D., and Bally, M. B. (2005). The Liposomal Formulation of Doxorubicin. Methods Enzymol. 391, 71–97. doi:10.1016/s0076-6879(05)91004-5
Aisha, A. F., Majid, A. M. S. A., and Ismail, Z. (2014). Preparation and Characterization of Nano Liposomes of Orthosiphon Stamineusethanolic Extract in Soybean Phospholipids. BMC Biotechnol. 14, 23. doi:10.1186/1472-6750-14-23
Allen, T. M., Hansen, C. B., and de Menezes, D. E. L. (1995). Pharmacokinetics of Long-Circulating Liposomes. Adv. Drug Deliv. Rev. 16, 267–284. doi:10.1016/0169-409X(95)00029-7
An, T., Yin, H., Lu, Y., and Liu, F. (2022). The Emerging Potential of Parthenolide Nanoformulations in Tumor Therapy. Dddt Vol. 16, 1255–1272. doi:10.2147/dddt.s355059
Atanasov, A. G., Zotchev, S. B., Zotchev, S. B., Dirsch, V. M., Supuran, C. T., Rollinger, J. M., et al. (2021). Natural Products in Drug Discovery: Advances and Opportunities. Nat. Rev. Drug Discov. 20, 200–216. doi:10.1038/s41573-020-00114-z
Bai, R., Chen, N., Li, L., Du, N., Bai, L., Lv, Z., et al. (2020). Mechanisms of Cancer Resistance to Immunotherapy. Front. Oncol. 10, 1290. doi:10.3389/fonc.2020.01290
Bilia, A. R., Piazzini, V., Asprea, M., Risaliti, L., Vanti, G., and Bergonzi, M. C. (2018). Plants Extracts Loaded in Nanocarriers: An Emergent Formulating Approach. Nat. Product. Commun. 13, 1934578X1801300–1234. doi:10.1177/1934578X1801300914
Blanco, E., Shen, H., and Ferrari, M. (2015). Principles of Nanoparticle Design for Overcoming Biological Barriers to Drug Delivery. Nat. Biotechnol. 33, 941–951. doi:10.1038/nbt.3330
Bo, R., Sun, Y., Zhou, S., Ou, N., Gu, P., Liu, Z., et al. (2017). Simple Nanoliposomes Encapsulating Lycium Barbarum Polysaccharides as Adjuvants Improve Humoral and Cellular Immunity in Mice. Ijn Vol. 12, 6289–6301. doi:10.2147/ijn.s136820
Boman, N. L., Masin, D., Mayer, L. D., Cullis, P. R., and Bally, M. B. (1994). Liposomal Vincristine Which Exhibits Increased Drug Retention and Increased Circulation Longevity Cures Mice Bearing P388 Tumors. Cancer Res. 54, 2830–2833.
Cai, Y., Xu, Y., Chan, H. F., Fang, X., He, C., and Chen, M. (2016). Glycyrrhetinic Acid Mediated Drug Delivery Carriers for Hepatocellular Carcinoma Therapy. Mol. Pharm. 13, 699–709. doi:10.1021/acs.molpharmaceut.5b00677
Casas, A., Di Venosa, G., Hasan, T., and Batlle, A. (2011). Mechanisms of Resistance to Photodynamic Therapy. Cmc 18, 2486–2515. doi:10.2174/092986711795843272
Chen, E. C., Fathi, A. T., and Brunner, A. M. (2018). Reformulating Acute Myeloid Leukemia: Liposomal Cytarabine and Daunorubicin (CPX-351) as an Emerging Therapy for Secondary AML. Ott Vol. 11, 3425–3434. doi:10.2147/ott.s141212
Chen, J., Chen, Y., Cheng, Y., Gao, Y., Zheng, P., Li, C., et al. (2017). Modifying Glycyrrhetinic Acid Liposomes with Liver-Targeting Ligand of Galactosylated Derivative: Preparation and Evaluations. Oncotarget 8, 102046–102066. doi:10.18632/oncotarget.22143
Chen, J., Liu, Q., Wang, Y., Guo, Y., Xu, X., Huang, P., et al. (2021). Protective Effects of Resveratrol Liposomes on Mitochondria in Substantia Nigra Cells of Parkinsonized Rats. Ann. Palliat. Med. 10, 2458–2468. doi:10.21037/apm-19-426
Chen, L., Wang, L., Zhu, L., Xu, Z., Liu, Y., Li, Z., et al. (2022). Exosomes as Drug Carriers in Anti-cancer Therapy. Front. Cell. Dev. Biol. 10, 728616. doi:10.3389/fcell.2022.728616
Chen, M. H., Chang, C. H., Chang, Y. J., Chen, L. C., Yu, C. Y., Wu, Y. H., et al. (2010). MicroSPECT/CT Imaging and Pharmacokinetics of 188Re-(DXR)-liposome in Human Colorectal Adenocarcinoma-Bearing Mice. Anticancer Res. 30, 65–72.
Chen, Y., Minh, L. V., Liu, J., Angelov, B., Drechsler, M., Garamus, V. M., et al. (2016). Baicalin Loaded in Folate-PEG Modified Liposomes for Enhanced Stability and Tumor Targeting. Colloids Surfaces B Biointerfaces 140, 74–82. doi:10.1016/j.colsurfb.2015.11.018
Cheng, X., Gao, J., Ding, Y., Lu, Y., Wei, Q., Cui, D., et al. (2021). Multi‐Functional Liposome: A Powerful Theranostic Nano‐Platform Enhancing Photodynamic Therapy. Adv. Sci. 8, 2100876. doi:10.1002/Advs.202100876
Chernov, L., Deyell, R. J., Anantha, M., Dos Santos, N., Gilabert-Oriol, R., and Bally, M. B. (2017). Optimization of Liposomal Topotecan for Use in Treating Neuroblastoma. Cancer Med. 6, 1240–1254. doi:10.1002/cam4.1083
Chu, M., Fan, Q., and Wu, M. (2012). Near Infrared Fluorescent Chlorophyll Nanoscale Liposomes for Sentinel Lymph Node Mapping. Ijn 7, 3071–3080. doi:10.2147/IJN.S27546
Cook, C. A. (2013). “The Pre-han Period,” in Chinese Medicine and Healing. Editors T. J. Hinrichs, and L. L. Barnes (Harvard University Press), 5–30. doi:10.2307/j.ctv15pjz2g.4
de Smet, M., Langereis, S., den Bosch, S. v., and Grüll, H. (2010). Temperature-sensitive Liposomes for Doxorubicin Delivery under MRI Guidance. J. Control. Release 143, 120–127. doi:10.1016/j.jconrel.2009.12.002
Değim, I. T., Gümüşel, B., Değim, Z., Özçelikay, T., Tay, A., and Güner, Ş. (2006). Oral Administration of Liposomal Insulin. J. Nanosci. Nanotechnol. 6, 2945–2949. doi:10.1166/jnn.2006.416
Deodhar, S., and Dash, A. K. (2018). Long Circulating Liposomes: Challenges and Opportunities. Ther. Deliv. 9, 857–872. doi:10.4155/tde-2018-0035
Ding, Z., Xiao, J., Zhang, Y., Jiang, Y., Chen, W., Hu, J., et al. (2019). Pharmacokinetics and Liver Uptake of Three Schisandra Lignans in Rats after Oral Administration of Liposome Encapsulating β-cyclodextrin Inclusion Compound of Schisandra Extract. J. Liposome Res. 29, 121–132. doi:10.1080/08982104.2018.1430830
Dogra, N., Izadi, H., and Vanderlick, T. K. (2016). Micro-motors: A Motile Bacteria Based System for Liposome Cargo Transport. Sci. Rep. 6, 29369. doi:10.1038/srep29369
Dos Santos, N., Waterhouse, D., Masin, D., Tardi, P. G., Karlsson, G., Edwards, K., et al. (2005). Substantial Increases in Idarubicin Plasma Concentration by Liposome Encapsulation Mediates Improved Antitumor Activity. J. Control. Release 105, 89–105. doi:10.1016/j.jconrel.2005.03.007
Du, C., Li, S., Li, Y., Galons, H., Guo, N., Teng, Y., et al. (2020). F7 and Topotecan Co-loaded Thermosensitive Liposome as a Nano-Drug Delivery System for Tumor Hyperthermia. Drug Deliv. 27, 836–847. doi:10.1080/10717544.2020.1772409
Emerson, D. L. (2000). Liposomal Delivery of Camptothecins. Pharm. Sci. Technol. Today 3, 205–209. doi:10.1016/S1461-5347(00)00268-6
Fan, Y., Ma, X., Ma, L., Zhang, J., Zhang, W., and Song, X. (2016). Antioxidative and Immunological Activities of Ophiopogon Polysaccharide Liposome from the Root of Ophiopogon Japonicus. Carbohydr. Polym. 135, 110–120. doi:10.1016/j.carbpol.2015.08.089
Fan, Y., Ma, X., Zhang, J., Ma, L., Gao, Y., Zhang, W., et al. (2015). Ophiopogon Polysaccharide Liposome Can Enhance the Non-specific and Specific Immune Response in Chickens. Carbohydr. Polym. 119, 219–227. doi:10.1016/j.carbpol.2014.11.048
Fan, Y., Song, X., Gao, Y., Chen, Y., Ma, L., Zhang, W., et al. (2014). Preparation and Optimization of Ophiopogon Polysaccharide Liposome and its Activity on Kupffer Cells. Int. J. Pharm. 477, 421–430. doi:10.1016/j.ijpharm.2014.10.053
Feng, T., Wei, Y., Lee, R., and Zhao, L. (2017). Liposomal Curcumin and its Application in Cancer. Ijn Vol. 12, 6027–6044. doi:10.2147/IJN.S132434
Flaten, G. E., Chang, T.-T., Phillips, W. T., Brandl, M., Bao, A., and Goins, B. (2013). Liposomal Formulations of Poorly Soluble Camptothecin: Drug Retention and Biodistribution. J. Liposome Res. 23, 70–81. doi:10.3109/08982104.2012.742537
Fu, X., Lu, Y., Guo, J., Liu, H., Deng, A., Kuang, C., et al. (2019). K237-modified Thermosensitive Liposome Enhanced the Delivery Efficiency and Cytotoxicity of Paclitaxel In Vitro. J. Liposome Res. 29, 86–93. doi:10.1080/08982104.2018.1458863
Fu, Y., Chen, J., and Huang, Z. (2019). Recent Progress in microRNA-Based Delivery Systems for the Treatment of Human Disease. ExRNA 1, 24. doi:10.1186/s41544-019-0024-y
Gao, H.-W., Chang, K.-F., Huang, X.-F., Lin, Y.-L., Weng, J.-C., Liao, K.-W., et al. (2018). Antitumor Effect of N-Butylidenephthalide Encapsulated on B16/F10 Melanoma Cells In Vitro with a Polycationic Liposome Containing PEI and Polyethylene Glycol Complex. Molecules 23, 3224. doi:10.3390/molecules23123224
Gao, H., Fan, Y., Wang, D., Hu, Y., Liu, J., Zhao, X., et al. (2012). Optimization on Preparation Condition of Epimedium Polysaccharide Liposome and Evaluation of its Adjuvant Activity. Int. J. Biol. Macromol. 50, 207–213. doi:10.1016/j.ijbiomac.2011.10.021
Gao, W., Li, L., Zhang, X., Luo, L., He, Y., Cong, C., et al. (2020). Nanomagnetic Liposome-Encapsulated Parthenolide and Indocyanine Green for Targeting and Chemo-Photothermal Antitumor Therapy. Nanomedicine 15, 871–890. doi:10.2217/nnm-2019-0038
Gao, W., Wei, S., Li, Z., Li, L., Zhang, X., Li, C., et al. (2020). Nano Magnetic Liposomes-Encapsulated Parthenolide and Glucose Oxidase for Ultra-efficient Synergistic Antitumor Therapy. Nanotechnology 31, 355104. doi:10.1088/1361-6528/ab92c8
Gawne, P., Man, F., Fonslet, J., Radia, R., Bordoloi, J., Cleveland, M., et al. (2018). Manganese-52: Applications in Cell Radiolabelling and Liposomal Nanomedicine PET Imaging Using Oxine (8-hydroxyquinoline) as an Ionophore. Dalton Trans. 47, 9283–9293. doi:10.1039/C8DT00100F
Gómez-Murcia, V., Ribeiro Do Couto, B., Gómez-Fernández, J. C., Milanés, M. V., Laorden, M. L., and Almela, P. (2019). Liposome-Encapsulated Morphine Affords a Prolonged Analgesia while Facilitating Extinction of Reward and Aversive Memories. Front. Pharmacol. 10, 1082. doi:10.3389/fphar.2019.01082
Govindachari, T. R., and Viswanathan, N. (1972). Alkaloids of Mappia Foetida. Phytochemistry 11, 3529–3531. doi:10.1016/S0031-9422(00)89852-0
Gregoriadis, G. (1976). The Carrier Potential of Liposomes in Biology and Medicine. N. Engl. J. Med. 295, 765–770. doi:10.1056/nejm197609302951406
Gubernator, J. (2011). Active Methods of Drug Loading into Liposomes: Recent Strategies for Stable Drug Entrapment and Increasedin Vivoactivity. Expert Opin. Drug Deliv. 8, 565–580. doi:10.1517/17425247.2011.566552
Guillermo-Lagae, R., Santha, S., Thomas, M., Zoelle, E., Stevens, J., Kaushik, R. S., et al. (2017). Antineoplastic Effects of Honokiol on Melanoma. BioMed Res. Int. 2017, 1–10. doi:10.1155/2017/5496398
Guo, B.-h., Cheng, Y., Lin, L.-p., Lin, D.-h., and Wu, W. (2011). Preparation and Characterization of Galactose-Modified Liposomes by a Nonaqueous Enzymatic Reaction. J. Liposome Res. 21, 255–260. doi:10.3109/08982104.2011.573795
Guo, P., Yu, C., Wang, Q., Zhang, R., Meng, X., and Feng, Y. (2018). Liposome Lipid-Based Formulation Has the Least Influence on rAAV Transduction Compared to Other Transfection Agents. Mol. Ther. - Methods & Clin. Dev. 9, 367–375. doi:10.1016/j.omtm.2018.04.004
Guo, R.-b., Zhang, X.-y., Yan, D.-k., Yu, Y.-j., Wang, Y.-j., Geng, H.-x., et al. (2022). Folate-modified Triptolide Liposomes Target Activated Macrophages for Safe Rheumatoid Arthritis Therapy. Biomater. Sci. 10, 499–513. doi:10.1039/D1BM01520F
Hamano, N., Böttger, R., Lee, S. E., Yang, Y., Kulkarni, J. A., Ip, S., et al. (2019). Robust Microfluidic Technology and New Lipid Composition for Fabrication of Curcumin-Loaded Liposomes: Effect on the Anticancer Activity and Safety of Cisplatin. Mol. Pharm. 16, 3957–3967. doi:10.1021/acs.molpharmaceut.9b00583
Han, S.-R., Gong, H., Wang, Y.-M., Lv, X.-Y., Zhang, C., Tong, A.-N., et al. (2014). The Preparation of Matrine Liposome and its Antiglioma Activity Study. J. Chem. 2014, 1–5. doi:10.1155/2014/317320
Hızır-Kadı, İ., Gültekin-Özgüven, M., Altin, G., Demircan, E., and Özçelik, B. (2020). Liposomal Nanodelivery Systems Generated from Proliposomes for Pollen Extract with Improved Solubility and In Vitro Bioaccessibility. Heliyon 6, e05030. doi:10.1016/j.heliyon.2020.e05030
Hoffman, R. D., Li, C.-Y., He, K., Wu, X., He, B.-C., He, T.-C., et al. (2020). Chinese Herbal Medicine and its Regulatory Effects on Tumor Related T Cells. Front. Pharmacol. 11, 492. doi:10.3389/fphar.2020.00492
Hong, C., Liang, J., Xia, J., Zhu, Y., Guo, Y., Wang, A., et al. (2020). One Stone Four Birds: A Novel Liposomal Delivery System Multi-Functionalized with Ginsenoside Rh2 for Tumor Targeting Therapy. Nano-Micro Lett. 12, 129. doi:10.1007/s40820-020-00472-8
Hong, C., Liang, J., Xia, J., Zhu, Y., Guo, Y., Wang, A., et al. (2020). One Stone Four Birds: A Novel Liposomal Delivery System Multi-Functionalized with Ginsenoside Rh2 for Tumor Targeting Therapy. Nano-Micro Lett. 12, 129. doi:10.1007/s40820-020-00472-8
Hong, C., Wang, D., Liang, J., Guo, Y., Zhu, Y., Xia, J., et al. (2019). Novel Ginsenoside-Based Multifunctional Liposomal Delivery System for Combination Therapy of Gastric Cancer. Theranostics 9, 4437–4449. doi:10.7150/thno.34953
Hou, X., Zaks, T., Langer, R., and Dong, Y. (2021). Lipid Nanoparticles for mRNA Delivery. Nat. Rev. Mat. 6, 1078–1094. doi:10.1038/s41578-021-00358-0
Hu, J., Chen, L.-j., Liu, L., Chen, X., Chen, P., Yang, G.-l., et al. (2008). Liposomal Honokiol, a Potent Anti-angiogenesis Agent, in Combination with Radiotherapy Produces a Synergistic Antitumor Efficacy without Increasing Toxicity. Exp. Mol. Med. 40, 617–628. doi:10.3858/emm.2008.40.6.617
Huang, Y., Qin, T., Huang, Y., Liu, Z., Bo, R., Hu, Y., et al. (2016). Rehmannia Glutinosa Polysaccharide Liposome as a Novel Strategy for Stimulating an Efficient Immune Response and Their Effects on Dendritic Cells. Ijn Vol. 11, 6795–6808. doi:10.2147/ijn.s119108
Inaba, H., Uemura, A., Morishita, K., Kohiki, T., Shigenaga, A., Otaka, A., et al. (2018). Light-induced Propulsion of a Giant Liposome Driven by Peptide Nanofibre Growth. Sci. Rep. 8, 6243. doi:10.1038/s41598-018-24675-7
Isacchi, B., Arrigucci, S., Marca, G. l., Bergonzi, M. C., Vannucchi, M. G., Novelli, A., et al. (2011). Conventional and Long-Circulating Liposomes of Artemisinin: Preparation, Characterization, and Pharmacokinetic Profile in Mice. J. Liposome Res. 21, 237–244. doi:10.3109/08982104.2010.539185
Isacchi, B., Bergonzi, M. C., Grazioso, M., Righeschi, C., Pietretti, A., Severini, C., et al. (2012). Artemisinin and Artemisinin Plus Curcumin Liposomal Formulations: Enhanced Antimalarial Efficacy against Plasmodium Berghei-Infected Mice. Eur. J. Pharm. Biopharm. 80, 528–534. doi:10.1016/j.ejpb.2011.11.015
Isacchi, B., Fabbri, V., Galeotti, N., Bergonzi, M. C., Karioti, A., Ghelardini, C., et al. (2011). Salvianolic Acid B and its Liposomal Formulations: Anti-hyperalgesic Activity in the Treatment of Neuropathic Pain. Eur. J. Pharm. Sci. 44, 552–558. doi:10.1016/j.ejps.2011.09.019
Jaafar-Maalej, C., Diab, R., Andrieu, V., Elaissari, A., and Fessi, H. (2010). Ethanol Injection Method for Hydrophilic and Lipophilic Drug-Loaded Liposome Preparation. J. Liposome Res. 20, 228–243. doi:10.3109/08982100903347923
Jain, A., and Jain, S. K. (2018). Stimuli-responsive Smart Liposomes in Cancer Targeting. Cdt 19, 259–270. doi:10.2174/1389450117666160208144143
Jeon, S., Yoo, C. Y., and Park, S. N. (2015). Improved Stability and Skin Permeability of Sodium Hyaluronate-Chitosan Multilayered Liposomes by Layer-By-Layer Electrostatic Deposition for Quercetin Delivery. Colloids Surfaces B Biointerfaces 129, 7–14. doi:10.1016/j.colsurfb.2015.03.018
Jiang, Q.-q., Fan, L.-y., Yang, G.-l., Guo, W.-H., Hou, W.-l., Chen, L.-j., et al. (2008). Improved Therapeutic Effectiveness by Combining Liposomal Honokiol with Cisplatin in Lung Cancer Model. BMC Cancer 8, 242. doi:10.1186/1471-2407-8-242
Jin, S., Wang, X. L., and Li, G. (2017). Preparation and In Vivo Study of Liposomes Mediated by Glycyrrhetinic Acid Derivative Ligand 18-GA-Gly. Zhongguo Zhong Yao Za Zhi 42, 4120–4126. doi:10.19540/j.cnki.cjcmm.20170928.024
Jin, X., Yang, Q., Cai, N., and Zhang, Z. (2020). A Cocktail of Betulinic Acid, Parthenolide, Honokiol and Ginsenoside Rh2 in Liposome Systems for Lung Cancer Treatment. Nanomedicine 15, 41–54. doi:10.2217/nnm-2018-0479
Jin, X., Zhou, J., Zhang, Z., and Lv, H. (2018). The Combined Administration of Parthenolide and Ginsenoside CK in Long Circulation Liposomes with Targeted tLyp-1 Ligand Induce Mitochondria-Mediated Lung Cancer Apoptosis. Artif. Cells, Nanomedicine, Biotechnol. 46, S931–S942. doi:10.1080/21691401.2018.1518913
Kukula-Koch, W. A., and Widelski, J. (2017). “Alkaloids,” in Pharmacognosy. Editors S. Badal, and R. Delgoda (Boston: Academic Press), 163–198. doi:10.1016/b978-0-12-802104-0.00009-3
Lau, J. L., and Dunn, M. K. (2018). Therapeutic Peptides: Historical Perspectives, Current Development Trends, and Future Directions. Bioorg. Med. Chem. 26, 2700–2707. doi:10.1016/j.bmc.2017.06.052
Lee, H., Gaddy, D., Ventura, M., Bernards, N., de Souza, R., Kirpotin, D., et al. (2018). Companion Diagnostic 64Cu-Liposome Positron Emission Tomography Enables Characterization of Drug Delivery to Tumors and Predicts Response to Cancer Nanomedicines. Theranostics 8, 2300–2312. doi:10.7150/thno.21670
Leto, I., Coronnello, M., Righeschi, C., Bergonzi, M. C., Mini, E., and Bilia, A. R. (2016). Enhanced Efficacy of Artemisinin Loaded in Transferrin-Conjugated Liposomes versus Stealth Liposomes against HCT-8 Colon Cancer Cells. ChemMedChem 11, 1745–1751. doi:10.1002/cmdc.201500586
Li, J., Xie, Q., Ma, R., Li, Y., Yuan, J., Ren, M., et al. (2021). Recent Progress on the Synergistic Antitumor Effect of a Borneol-Modified Nanocarrier Drug Delivery System. Front. Med. 8, 750170. doi:10.3389/fmed.2021.750170
Li, J., Xu, H., Ke, X., and Tian, J. (2012). The Anti-tumor Performance of Docetaxel Liposomes Surface-Modified with Glycyrrhetinic Acid. J. Drug Target. 20, 467–473. doi:10.3109/1061186x.2012.685475
Li, L., Feng, Y., Hong, Y., Lin, X., and Shen, L. (2018). Recent Advances in Drug Delivery System for Bioactive Glycosides from Traditional Chinese Medicine. Am. J. Chin. Med. 46, 1791–1824. doi:10.1142/s0192415x18500908
Li, L., He, D., Guo, Q., Zhang, Z., Ru, D., Wang, L., et al. (2022). Exosome-liposome Hybrid Nanoparticle Codelivery of TP and miR497 Conspicuously Overcomes Chemoresistant Ovarian Cancer. J. Nanobiotechnol. 20, 50. doi:10.1186/s12951-022-01264-5
Li, S., Chen, J., Chen, F., Wang, C., Guo, X., Wang, C., et al. (2021). Liposomal Honokiol Promotes Hair Growth via Activating Wnt3a/β-Catenin Signaling Pathway and Down Regulating TGF-Β1 in C57BL/6N Mice. Biomed. Pharmacother. 141, 111793. doi:10.1016/j.biopha.2021.111793
Li, S., Goins, B., Zhang, L., and Bao, A. (2012). Novel Multifunctional Theranostic Liposome Drug Delivery System: Construction, Characterization, and Multimodality MR, Near-Infrared Fluorescent, and Nuclear Imaging. Bioconjugate Chem. 23, 1322–1332. doi:10.1021/bc300175d
Li, S., Li, L., Chen, J., Fan, Y., Wang, C., Du, Y., et al. (2021). Liposomal Honokiol Inhibits Glioblastoma Growth through Regulating Macrophage Polarization. Ann. Transl. Med. 9, 1644. doi:10.21037/atm-21-1836
Li, T., Yu, P., Chen, Y., Sun, B., Dong, P., Zhu, T., et al. (2021). N-acetylgalactosamine-decorated Nanoliposomes for Targeted Delivery of Paclitaxel to Hepatocellular Carcinoma. Eur. J. Med. Chem. 222, 113605. doi:10.1016/j.ejmech.2021.113605
Li, W. J., Lian, Y. W., Guan, Q. S., Li, N., Liang, W. J., Liu, W. X., et al. (2018). Liver‑targeted Delivery of Liposome‑encapsulated Curcumol Using Galactosylated‑stearate. Exp. Ther. Med. 16, 925–930. doi:10.3892/etm.2018.6210
Li, Y., Zhai, Y., Liu, W., Zhang, K., Liu, J., Shi, J., et al. (2019). Ultrasmall Nanostructured Drug Based pH-Sensitive Liposome for Effective Treatment of Drug-Resistant Tumor. J. Nanobiotechnol. 17, 117. doi:10.1186/s12951-019-0550-7
Lin, C., Zhang, X., Chen, H., Bian, Z., Zhang, G., Riaz, M. K., et al. (2018). Dual-ligand Modified Liposomes Provide Effective Local Targeted Delivery of Lung-Cancer Drug by Antibody and Tumor Lineage-Homing Cell-Penetrating Peptide. Drug Deliv. 25, 256–266. doi:10.1080/10717544.2018.1425777
Lin, J., Wang, X., Wu, Q., Dai, J., Guan, H., Cao, W., et al. (2014). Development of Salvianolic Acid B-Tanshinone II A-Glycyrrhetinic Acid Compound Liposomes: Formulation Optimization and its Effects on Proliferation of Hepatic Stellate Cells. Int. J. Pharm. 462, 11–18. doi:10.1016/j.ijpharm.2013.12.040
Liu, H., Shen, M., Zhao, D., Ru, D., Duan, Y., Ding, C., et al. (2019). The Effect of Triptolide-Loaded Exosomes on the Proliferation and Apoptosis of Human Ovarian Cancer SKOV3 Cells. BioMed Res. Int. 2019, 1–14. doi:10.1155/2019/2595801
Liu, T. W., Huynh, E., MacDonald, T. D., and Zheng, G. (2014). “Porphyrins for Imaging, Photodynamic Therapy, and Photothermal Therapy,” in Cancer Theranostics. Editors X. Chen, and S. Wong (Oxford: Academic Press), 229–254. doi:10.1016/b978-0-12-407722-5.00014-1
Liu, X.-y., Ruan, L.-m., Mao, W.-w., Wang, J.-Q., Shen, Y.-q., and Sui, M.-h. (2010). Preparation of RGD-Modified Long Circulating Liposome Loading Matrine, and its In Vitro Anti-cancer Effects. Int. J. Med. Sci. 7, 197–208. doi:10.7150/ijms.7.197
Ludwiczuk, A., Skalicka-Woźniak, K., and Georgiev, M. I. (2017). “Terpenoids,” in Pharmacognosy. Editors S. Badal, and R. Delgoda (Boston: Academic Press), 233–266. doi:10.1016/b978-0-12-802104-0.00011-1
Luo, H., Zhong, Q., Chen, L.-j., Qi, X.-r., Fu, A.-f., Yang, H.-s., et al. (2008). Liposomal Honokiol, a Promising Agent for Treatment of Cisplatin-Resistant Human Ovarian Cancer. J. Cancer Res. Clin. Oncol. 134, 937–945. doi:10.1007/s00432-008-0375-5
Mandal, A. K., and Das, N. (2005). Sugar Coated Liposomal Flavonoid: A Unique Formulation in Combating Carbontetrachloride Induced Hepatic Oxidative Damage. J. Drug Target. 13, 305–315. doi:10.1080/10611860500230278
Mao, S. J., Bi, Y. Q., Jin, H., Wei, D. P., He, R., and Hou, S. X. (2007). Preparation, Characterization and Uptake by Primary Cultured Rat Hepatocytes of Liposomes Surface-Modified with Glycyrrhetinic Acid. Pharmazie 62, 614–619.
Mao, S. J., Hou, S. X., Jin, H., Zhang, L. K., and Jiang-Bin, B. (2003). Preparation of Liposomes Surface-Modified with Glycyrrhetinic Acid Targeting to Hepatocytes. Zhongguo Zhong Yao Za Zhi 28, 328–331.
Memvanga, P. B., and Nkanga, C. I. (2021). Liposomes for Malaria Management: the Evolution from 1980 to 2020. Malar. J. 20, 327. doi:10.1186/s12936-021-03858-0
Monteiro, L. O. F., Malachias, Â., Pound-Lana, G., Magalhães-Paniago, R., Mosqueira, V. C. F., Oliveira, M. C., et al. (2018). Paclitaxel-Loaded pH-Sensitive Liposome: New Insights on Structural and Physicochemical Characterization. Langmuir 34, 5728–5737. doi:10.1021/acs.langmuir.8b00411
Mu, X., and Wang, C. (2018). Artemisinins-a Promising New Treatment for Systemic Lupus Erythematosus: a Descriptive Review. Curr. Rheumatol. Rep. 20, 55. doi:10.1007/s11926-018-0764-y
Mullauer, F. B., van Bloois, L., Daalhuisen, J. B., Ten Brink, M. S., Storm, G., Medema, J. P., et al. (2011). Betulinic Acid Delivered in Liposomes Reduces Growth of Human Lung and Colon Cancers in Mice without Causing Systemic Toxicity. Anticancer Drugs 22, 223–233. doi:10.1097/CAD.0b013e3283421035
Nakhaei, P., Margiana, R., Bokov, D. O., Abdelbasset, W. K., Jadidi Kouhbanani, M. A., Varma, R. S., et al. (2021). Liposomes: Structure, Biomedical Applications, and Stability Parameters with Emphasis on Cholesterol. Front. Bioeng. Biotechnol. 9, 705886. doi:10.3389/fbioe.2021.705886
Nieth, A., Verseux, C., Barnert, S., Süss, R., and Römer, W. (2015). A First Step toward Liposome-Mediated Intracellular Bacteriophage Therapy. Expert Opin. Drug Deliv. 12, 1411–1424. doi:10.1517/17425247.2015.1043125
Niu, T., and Gao, H. (2009). Inhibitory Effects of Liposomal Honokiol on the Lymphoma. Blood 114, 4788. doi:10.1182/blood.V114.22.4788.4788
O'Byrne, K. J., Thomas, A. L., Sharma, R. A., DeCatris, M., Shields, F., Beare, S., et al. (2002). A Phase I Dose-Escalating Study of DaunoXome, Liposomal Daunorubicin, in Metastatic Breast Cancer. Br. J. Cancer 87, 15–20. doi:10.1038/sj.bjc.6600344
Ong, C. P., Lee, W. L., Tang, Y. Q., and Yap, W. H. (2020). Honokiol: A Review of its Anticancer Potential and Mechanisms. Cancers 12, 48. doi:10.3390/cancers12010048
Panche, A. N., Diwan, A. D., and Chandra, S. R. (2016). Flavonoids: an Overview. J. Nutr. Sci. 5, e47. doi:10.1017/jns.2016.41
Pandey, K. B., and Rizvi, S. I. (2009). Plant Polyphenols as Dietary Antioxidants in Human Health and Disease. Oxidative Med. Cell. Longev. 2, 270–278. doi:10.4161/oxim.2.5.9498
Pathak, P., Hess, R., and Weiss, M. A. (2014). Liposomal Vincristine for Relapsed or Refractory Ph-Negative Acute Lymphoblastic Leukemia: a Review of Literature. Ther. Adv. Hematol. 5, 18–24. doi:10.1177/2040620713519016
Patrucco, D., and Terreno, E. (2020). MR-guided Drug Release from Liposomes Triggered by Thermal and Mechanical Ultrasound-Induced Effects. Front. Phys. 8, 325. doi:10.3389/fphy.2020.00325
Pérez-Medina, C., Abdel-Atti, D., Tang, J., Zhao, Y., Fayad, Z. A., Lewis, J. S., et al. (2016). Nanoreporter PET Predicts the Efficacy of Anti-cancer Nanotherapy. Nat. Commun. 7, 11838. doi:10.1038/ncomms11838
Piccaluga, P., Visani, G., Martinelli, G., Isidori, A., Malagola, M., Rondoni, M., et al. (2002). Liposomal Daunorubicin (DaunoXome) for Treatment of Relapsed Meningeal Acute Myeloid Leukemia. Leukemia 16, 1880–1881. doi:10.1038/sj.leu.2402617
Pleban, E., Szopiński, P., Górski, G., Michalak, J., Noszczyk, B., and Ciostek, P. (2008). The Use of Liposomal Heparin Spray-Gel in the Treatment of Superficial Thrombophlebitis: A Multicenter Clinical Investigation Analysis. Pol. J. Surg. 80, 81–55. doi:10.2478/v10035-008-0006-5
Pol, O., Planas, E., and Puig, M. M. (1996). Effects of Morphine and Liposomal Morphine in a Model of Intestinal Inflammation in Mice. Pharmacology 53, 180–189. doi:10.1159/000139429
Przybylo, M., Glogocka, D., Dobrucki, J. W., Fraczkowska, K., Podbielska, H., Kopaczynska, M., et al. (2016). The Cellular Internalization of Liposome Encapsulated Protoporphyrin IX by HeLa Cells. Eur. J. Pharm. Sci. 85, 39–46. doi:10.1016/j.ejps.2016.01.028
Rahman, M., Al-Ghamdi, S. A., Alharbi, K. S., Beg, S., Sharma, K., Anwar, F., et al. (2019). Ganoderic Acid Loaded Nano-Lipidic Carriers Improvise Treatment of Hepatocellular Carcinoma. Drug Deliv. 26, 782–793. doi:10.1080/10717544.2019.1606865
Rahman, S., Cao, S., Steadman, K. J., Wei, M., and Parekh, H. S. (2012). Native and β-cyclodextrin-enclosed Curcumin: Entrapment within Liposomes and Theirin Vitrocytotoxicity in Lung and Colon Cancer. Drug Deliv. 19, 346–353. doi:10.3109/10717544.2012.721143
Rajendran, V., Rohra, S., Raza, M., Hasan, G. M., Dutt, S., and Ghosh, P. C. (2016). Stearylamine Liposomal Delivery of Monensin in Combination with Free Artemisinin Eliminates Blood Stages of Plasmodium Falciparum in Culture and P. Berghei Infection in Murine Malaria. Antimicrob. Agents Chemother. 60, 1304–1318. doi:10.1128/aac.01796-15
Rupaimoole, R., and Slack, F. J. (2017). MicroRNA Therapeutics: towards a New Era for the Management of Cancer and Other Diseases. Nat. Rev. Drug Discov. 16, 203–222. doi:10.1038/nrd.2016.246
Schmidt, P. G., Adler-Moore, J. P., Forssen, E. A., and Proffitt, R. T. (1998). “Unilamellar Liposomes for Anticancer and Antifungal Therapy,” in Medical Applications of Liposomes. Editors D. D. Lasic, and D. Papahadjopoulos (Amsterdam: Elsevier Science B.V.), 703–731. doi:10.1016/b978-044482917-7/50037-5
Sercombe, L., Veerati, T., Moheimani, F., Wu, S. Y., Sood, A. K., and Hua, S. (2015). Advances and Challenges of Liposome Assisted Drug Delivery. Front. Pharmacol. 6, 286. doi:10.3389/fphar.2015.00286
Shao, X.-X., Chen, C., Liang, M.-M., Yu, Z.-y., Zhang, F.-C., Zhou, M.-j., et al. (2021). "Efficacy-Nature-Structure" Relationship of Traditional Chinese Medicine Based on Chemical Structural Data and Bioinformatics Analysis. ACS Omega 6, 33583–33598. doi:10.1021/acsomega.1c04440
Shariare, M. H., Rahman, M., Lubna, S. R., Roy, R. S., Abedin, J., Marzan, A. L., et al. (2020). Liposomal Drug Delivery of Aphanamixis Polystachya Leaf Extracts and its Neurobehavioral Activity in Mice Model. Sci. Rep. 10, 6938. doi:10.1038/s41598-020-63894-9
Shen, Y., Pi, Z., Yan, F., Yeh, C.-K., Zeng, X., Diao, X., et al. (2017). Enhanced Delivery of Paclitaxel Liposomes Using Focused Ultrasound with Microbubbles for Treating Nude Mice Bearing Intracranial Glioblastoma Xenografts. Ijn Vol. 12, 5613–5629. doi:10.2147/IJN.S136401
Shi, C., Cao, H., He, W., Gao, F., Liu, Y., and Yin, L. (2015). Novel Drug Delivery Liposomes Targeted with a Fully Human Anti-vegf165 Monoclonal Antibody Show Superior Antitumor Efficacy In Vivo. Biomed. Pharmacother. 73, 48–57. doi:10.1016/j.biopha.2015.05.008
Shoji, K., and Kawano, R. (2020). Recent Advances in Liposome-Based Molecular Robots. Micromachines 11, 788. doi:10.3390/mi11090788
Su, X., Kong, L., Lei, X., Hu, L., Ye, M., and Zou, H. (2007). Biological Fingerprinting Analysis of Traditional Chinese Medicines with Targeting ADME/Tox Property for Screening of Bioactive Compounds by Chromatographic and MS Methods. Mrmc 7, 87–98. doi:10.2174/138955707779317830
Sun, Q., You, Q., Wang, J., Liu, L., Wang, Y., Song, Y., et al. (2018). Theranostic Nanoplatform: Triple-Modal Imaging-Guided Synergistic Cancer Therapy Based on Liposome-Conjugated Mesoporous Silica Nanoparticles. ACS Appl. Mat. Interfaces 10, 1963–1975. doi:10.1021/acsami.7b13651
Sun, W., Hu, W., Meng, K., Yang, L., Zhang, W., Song, X., et al. (2016). Activation of Macrophages by the Ophiopogon Polysaccharide Liposome from the Root Tuber of Ophiopogon Japonicus. Int. J. Biol. Macromol. 91, 918–925. doi:10.1016/j.ijbiomac.2016.06.037
Swaminathan, J., and Ehrhardt, C. (2012). Liposomal Delivery of Proteins and Peptides. Expert Opin. Drug Deliv. 9, 1489–1503. doi:10.1517/17425247.2012.735658
Szebeni, J., Baranyi, L., Savay, S., Bodo, M., Morse, D. S., Basta, M., et al. (2000). Liposome-induced Pulmonary Hypertension: Properties and Mechanism of a Complement-Mediated Pseudoallergic Reaction. Am. J. Physiology-Heart Circulatory Physiology 279, H1319–H1328. doi:10.1152/ajpheart.2000.279.3.H1319
Tian, J., Wang, L., Wang, L., and Ke, X. (2014). A Wogonin-Loaded Glycyrrhetinic Acid-Modified Liposome for Hepatic Targeting with Anti-tumor Effects. Drug Deliv. 21, 553–559. doi:10.3109/10717544.2013.853850
Tsuji, H., Osaka, S., and Kiwada, H. (1991). Targeting of Liposomes Surface-Modified with Glycyrrhizin to the Liver. I. Preparation and Biological Disoposition. Chem. Pharm. Bull. 39, 1004–1008. doi:10.1248/cpb.39.1004
Vaghasiya, K., Sharma, A., Kumar, K., Ray, E., Adlakha, S., Katare, O. P., et al. (2019). Heparin-Encapsulated Metered-Dose Topical "Nano-Spray Gel" Liposomal Formulation Ensures Rapid On-Site Management of Frostbite Injury by Inflammatory Cytokines Scavenging. ACS Biomater. Sci. Eng. 5, 6617–6631. doi:10.1021/acsbiomaterials.9b01486
Varma, M. V. S., Feng, B., Obach, R. S., Troutman, M. D., Chupka, J., Miller, H. R., et al. (2009). Physicochemical Determinants of Human Renal Clearance. J. Med. Chem. 52, 4844–4852. doi:10.1021/jm900403j
Vasan, N., Baselga, J., and Hyman, D. M. (2019). A View on Drug Resistance in Cancer. Nature 575, 299–309. doi:10.1038/s41586-019-1730-1
Viglianti, B. L., Abraham, S. A., Michelich, C. R., Yarmolenko, P. S., MacFall, J. R., Bally, M. B., et al. (2004). In Vivo monitoring of Tissue Pharmacokinetics of Liposome/drug Using MRI: Illustration of Targeted Delivery. Magn. Reson. Med. 51, 1153–1162. doi:10.1002/mrm.20074
Wall, M. E., Wani, M. C., Cook, C. E., Palmer, K. H., McPhail, A. T., and Sim, G. A. (1966). Plant Antitumor Agents. I. The Isolation and Structure of Camptothecin, a Novel Alkaloidal Leukemia and Tumor Inhibitor from Camptotheca Acuminata1,2. J. Am. Chem. Soc. 88, 3888–3890. doi:10.1021/ja00968a057
Wang, H., Cui, H., Lin, L., Ji, Y., Ni, Q., Li, J., et al. (2019). The Effects of a Hirudin/liposome Complex on a Diabetic Nephropathy Rat Model. BMC Complement. Altern. Med. 19, 118. doi:10.1186/s12906-019-2531-7
Wang, Q., Liu, J., Liu, J., Thant, Y., Weng, W., Wei, C., et al. (2021). Bisdemethoxycurcumin-conjugated Vitamin E TPGS Liposomes Ameliorate Poor Bioavailability of Free Form and Evaluation of its Analgesic and Hypouricemic Activity in Oxonate-Treated Rats. J. Nanopart. Res. 23, 122. doi:10.1007/s11051-021-05222-4
Waterhouse, D. N., Tardi, P. G., Mayer, L. D., and Bally, M. B. (2001). A Comparison of Liposomal Formulations of Doxorubicin with Drug Administered in Free Form. Drug Saf. 24, 903–920. doi:10.2165/00002018-200124120-00004
Wen, J., Fu, A.-f., Chen, L.-J., Xie, X.-J., Yang, G.-L., Chen, X.-C., et al. (2009). Liposomal Honokiol Inhibits VEGF-D-Induced Lymphangiogenesis and Metastasis in Xenograft Tumor Model. Int. J. Cancer 124, 2709–2718. doi:10.1002/ijc.24244
Willers, H., Azzoli, C. G., Santivasi, W. L., and Xia, F. (2013). Basic Mechanisms of Therapeutic Resistance to Radiation and Chemotherapy in Lung Cancer. Cancer J. 19, 200–207. doi:10.1097/PPO.0b013e318292e4e3
Wu, C.-Y., Tang, J.-H., Chan, P.-C., Li, J.-J., Lin, M.-H., Shen, C.-C., et al. (2017). Monitoring Tumor Response after Liposomal Doxorubicin in Combination with Liposomal Vinorelbine Treatment Using 3′-Deoxy-3′-[18F]Fluorothymidine PET. Mol. Imaging Biol. 19, 408–420. doi:10.1007/s11307-016-1005-2
Xia, Y., Xu, C., Zhang, X., Ning, P., Wang, Z., Tian, J., et al. (2019). Liposome-based Probes for Molecular Imaging: from Basic Research to the Bedside. Nanoscale 11, 5822–5838. doi:10.1039/C9NR00207C
Xiang, Y., Long, Y., Yang, Q., Zheng, C., Cui, M., Ci, Z., et al. (2020). Pharmacokinetics, Pharmacodynamics and Toxicity of Baicalin Liposome on Cerebral Ischemia Reperfusion Injury Rats via Intranasal Administration. Brain Res. 1726, 146503. doi:10.1016/j.brainres.2019.146503
Yan, D., Ni, L.-K., Chen, H.-L., Chen, L.-C., Chen, Y.-H., and Cheng, C.-C. (2016). Amphiphilic Nanoparticles of Resveratrol-Norcantharidin to Enhance the Toxicity in Zebrafish Embryo. Bioorg. Med. Chem. Lett. 26, 774–777. doi:10.1016/j.bmcl.2015.12.099
Yu, L., Wang, Z., Mo, Z., Zou, B., Yang, Y., Sun, R., et al. (2021). Synergetic Delivery of Triptolide and Ce6 with Light-Activatable Liposomes for Efficient Hepatocellular Carcinoma Therapy. Acta Pharm. Sin. B 11, 2004–2015. doi:10.1016/j.apsb.2021.02.001
Yuan, Z.-p., Chen, L.-j., Fan, L.-y., Tang, M.-h., Yang, G.-l., Yang, H.-s., et al. (2006). Liposomal Quercetin Efficiently Suppresses Growth of Solid Tumors in Murine Models. Clin. Cancer Res. 12, 3193–3199. doi:10.1158/1078-0432.ccr-05-2365
Yuan, Z.-x., Jia, L., Lim, L. Y., Lin, J.-c., Shu, G., Zhao, L., et al. (2017). Renal-targeted Delivery of Triptolide by Entrapment in Pegylated TRX-20-Modified Liposomes. Ijn Vol. 12, 5673–5686. doi:10.2147/ijn.s141095
Y. Want, M., Islamuddin, M., Chouhan, G., A. Ozbak, H., A. Hemeg, H., P. Chattopadhyay, A., et al. (2017). Nanoliposomal Artemisinin for the Treatment of Murine Visceral Leishmaniasis. Ijn Vol. 12, 2189–2204. doi:10.2147/ijn.s106548
Zamboni, W. C., Strychor, S., Maruca, L., Ramalingam, S., Zamboni, B. A., Wu, H., et al. (2009). Pharmacokinetic Study of Pegylated Liposomal CKD-602 (S-Ckd602) in Patients with Advanced Malignancies. Clin. Pharmacol. Ther. 86, 519–526. doi:10.1038/clpt.2009.141
Zhang, X., Hu, J., and Chen, Y. (2016). Betulinic Acid and the Pharmacological Effects of Tumor Suppression. Mol. Med. Rep. 14, 4489–4495. doi:10.3892/mmr.2016.5792
Zhang, Y. J., Gallis, B., Taya, M., Wang, S., Ho, R. J. Y., and Sasaki, T. (2013). pH-Responsive Artemisinin Derivatives and Lipid Nanoparticle Formulations Inhibit Growth of Breast Cancer Cells In Vitro and Induce Down-Regulation of HER Family Members. PLoS One 8, e59086. doi:10.1371/journal.pone.0059086
Zhang, Y., Liu, S., Wan, J., Yang, Q., Xiang, Y., Ni, L., et al. (2020). Preparation, Characterization and In Vivo Study of Borneol-Baicalin-Liposomes for Treatment of Cerebral Ischemia-Reperfusion Injury. Ijn Vol. 15, 5977–5989. doi:10.2147/ijn.s259938
Zhang, Z., Li, Z., Yu, W., Li, K., Xie, Z., and Shi, Z. (2013). Propulsion of Liposomes Using Bacterial Motors. Nanotechnology 24, 185103. doi:10.1088/0957-4484/24/18/185103
Zhao, X., Luo, G., Bai, C., Peng, H., and Xiong, H. (2016). Juglone Thermosensitive Liposomes: Preparation, Characterization, In Vitro Release and Hyperthermia Cell Evaluation. Int. J. Food Eng. 12, 429–438. doi:10.1515/ijfe-2015-0285
Zhao, X., Wu, D., Ma, X., Wang, J., Hou, W., and Zhang, W. (2020). Exosomes as Drug Carriers for Cancer Therapy and Challenges Regarding Exosome Uptake. Biomed. Pharmacother. 128, 110237. doi:10.1016/j.biopha.2020.110237
Zhong, H., Deng, Y., Wang, X., and Yang, B. (2005). Multivesicular Liposome Formulation for the Sustained Delivery of Breviscapine. Int. J. Pharm. 301, 15–24. doi:10.1016/j.ijpharm.2005.04.001
Zhou, H., Xia, L., Zhong, J., Xiong, S., Yi, X., Chen, L., et al. (2019). Plant-derived Chlorophyll Derivative Loaded Liposomes for Tri-model Imaging Guided Photodynamic Therapy. Nanoscale 11, 19823–19831. doi:10.1039/C9NR06941K
Zhou, Y., Ning, Q., Yu, D.-n., Li, W.-g., and Deng, J. (2014). Improved Oral Bioavailability of Breviscapine via a Pluronic P85-Modified Liposomal Delivery System. J. Pharm. Pharmacol. 66, 903–911. doi:10.1111/jphp.12215
Zimmermann, K., Hossann, M., Hirschberger, J., Troedson, K., Peller, M., Schneider, M., et al. (2017). A Pilot Trial of Doxorubicin Containing Phosphatidyldiglycerol Based Thermosensitive Liposomes in Spontaneous Feline Soft Tissue Sarcoma. Int. J. Hyperth. 33, 178–190. doi:10.1080/02656736.2016.1230233
Keywords: liposome, nano-carrier, targeted-delivery, natural product, traditional Chinese medicine
Citation: Cheng X, Yan H, Pang S, Ya M, Qiu F, Qin P, Zeng C and Lu Y (2022) Liposomes as Multifunctional Nano-Carriers for Medicinal Natural Products. Front. Chem. 10:963004. doi: 10.3389/fchem.2022.963004
Received: 07 June 2022; Accepted: 24 June 2022;
Published: 08 August 2022.
Edited by:
Tongkai Chen, Guangzhou University of Chinese Medicine, ChinaCopyright © 2022 Cheng, Yan, Pang, Ya, Qiu, Qin, Zeng and Lu. This is an open-access article distributed under the terms of the Creative Commons Attribution License (CC BY). The use, distribution or reproduction in other forums is permitted, provided the original author(s) and the copyright owner(s) are credited and that the original publication in this journal is cited, in accordance with accepted academic practice. No use, distribution or reproduction is permitted which does not comply with these terms.
*Correspondence: Xiamin Cheng, eGlhbWluQHUubnVzLmVkdQ==; Chao Zeng, emVuZ2NoYW95dWUxMTBAMTYzLmNvbQ==; Yongna Lu, aWFzX3lubHVAbmp0ZWNoLmVkdS5jbg==
Disclaimer: All claims expressed in this article are solely those of the authors and do not necessarily represent those of their affiliated organizations, or those of the publisher, the editors and the reviewers. Any product that may be evaluated in this article or claim that may be made by its manufacturer is not guaranteed or endorsed by the publisher.
Research integrity at Frontiers
Learn more about the work of our research integrity team to safeguard the quality of each article we publish.