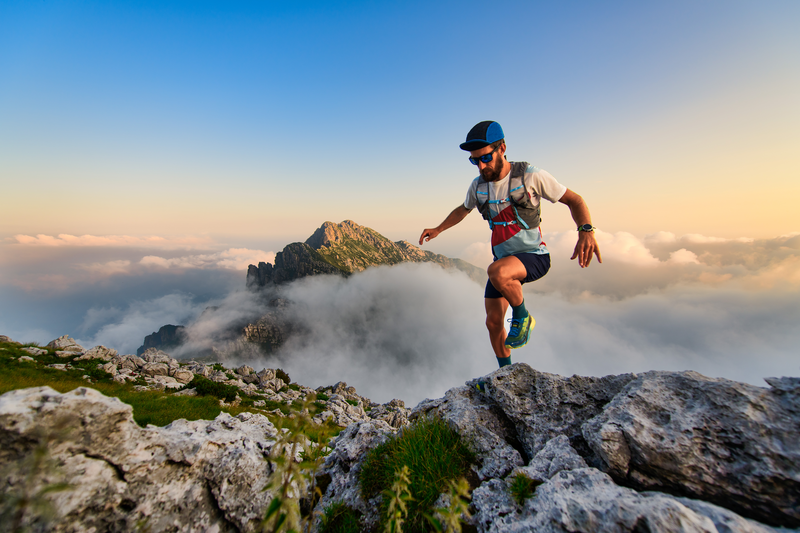
94% of researchers rate our articles as excellent or good
Learn more about the work of our research integrity team to safeguard the quality of each article we publish.
Find out more
ORIGINAL RESEARCH article
Front. Chem. , 19 July 2022
Sec. Organic Chemistry
Volume 10 - 2022 | https://doi.org/10.3389/fchem.2022.939644
This article is part of the Research Topic Advances in Novel Natural Product Pesticides View all 24 articles
Crop disease caused by fungi seriously affected food security and economic development. Inspired by the utilization of fungicide containing 1,2,4-triazole and trifluoromethylpyrimidine, a novel series of 1,2,4-triazolo[4,3-c]trifluoromethylpyrimidine derivatives bearing the thioether moiety were synthesized. Meanwhile, the antifungal activities of the title compounds were evaluated and most compounds exhibited obvious antifungal activities against cucumber Botrytis cinerea, strawberry Botrytis cinerea, tobacco Botrytis cinerea, blueberry Botrytis cinerea, Phytophthora infestans, and Pyricularia oryzae Cav. Among the compounds, 4, 5h, 5o, and 5r showed significant antifungal activities against three of the four Botrytis cinerea, which indicated the potential to become the leading structures or candidates for resistance to Botrytis cinerea.
Crop disease caused by fungi seriously affected food security and economic development (O’Brien, 2017; Yang et al., 2021). The application of existing antifungal agents was limited, due to the high resistance and security caused by fungicide abuse (Wei et al., 2021). It is increasingly urgent to develop new antimicrobial agents with high antimicrobial performances and good environmental friendliness.
Triazole fungicides are vital five-membered nitrogen-containing heterocycles, which are one of the largest categories of fungicides in the world, and they are widely used in agriculture field. The listed triazole fungicides include Cyproconazole, Epoxiconazole, and Prothioconazole (Figure 1). And there are also triazolopyrimidine fungicides ametoctradin. 1,2,4-Triazole derivatives have been extensively applied in antifungal (Sun et al., 2021), antitumor (Abdelrehim, 2021), antibacterial (Pathak et al., 2021), antiviral (Shao et al., 2021), herbicidal (Yang et al., 2022), and insecticidal (Fan et al., 2019) agents.
Pyrimidine is an essential part of DNA and RNA in living system (Kumar and Narasimhan, 2018). Pyrimidine derivatives exhibited diverse biological properties, such as antineoplastic (Kesari et al., 2021), anti-inflammatory (Abdel-Aziz et al., 2021), antiviral (Abu-Zaied et al., 2021), and antimicrobial (El-mahdy and Farouk, 2021) activities. In addition, fused pyrimidine systems, such as pyrrolo [3,2-d]pyrimidine and 1,2,4-triazolo[1,5-a]pyrimidine, also possess extensive biological activities (Cawrse et al., 2018; Wang et al., 2019; Baillache and Unciti-Broceta, 2020; Basyouni et al., 2021; Wang R.-X. et al., 2021; Fayed et al., 2022). For example, Abulkhair (El-Shershaby et al., 2021) developed a set of triazoloquinazoline derivatives and screened the most potential compound against four human cancer cell lines. Since the invention of ethirimol, a series of pyrimidine fungicides were commercialized including ethirimol, dimethirimol, azoxystrobin, and fenarimol (Figure 1).
Moreover thioether is generally recognized as a linking structure which is able to reduce the lipophilicity. Meanwhile, the linker is beneficial to enhance the drug likeness of bioactive molecules (Ding et al., 2021).
Based on the aforementioned consideration, we constructed a fused pyrimidine structure similar to ametoctradin. Furthermore, referring our previous works (Wu W. et al., 2019; Yu et al., 2021), trifluoromethyl was introduced into the pyrimidine scaffold and thioether linker was promoted. Finally we designed and synthesized a novel series of thio-1,2,4-triazolo[4,3-c]pyrimidine derivatives on account of the molecular hybridization strategy (Figure 2).
All solvents were dried by standard methods in advance and distilled before use. The melting points of the products were determined on a XT-4 binocular microscope (Beijing Tech Instrument Co., China). 1H NMR and 13C NMR (solvent DMSO-d6) spectral analyses were performed on a Bruker Avance NEO 600 NMR spectrometer (1H, 600 MHz; 13C, 150 MHz) at room temperature. TMS was used as an internal standard. Mass spectrometry (MS) data were obtained on a Thermo Scientific Q Exactive Focus instrument. The following abbreviations were used to label chemical shift multiplicities: s = singlet, d = doublet, t = triplet, and m = multiplet. Analytical TLC was performed on silica gel GF-254.
Intermediates 1 and 2 were synthesized by the methods of our previous work (Wu W.-N. et al., 2019).
2-Methyl-4-chloro-6-trifluoromethylpyrimidine (20 mmol) and absolute ethanol (50 ml) were weighed, added into a three-necked flask, and stirred under ice bath conditions, and hydrazine hydrate (30 mmol) was added dropwise slowly to the reaction system; after the addition was completed, the reaction was carried out in an ice bath, and the reaction was followed by TLC until the raw material was completed. Then water was added to obtain a yellow solid. The solid was purified by column chromatography, eluting with petroleum ether: ethyl acetate 20:1, which gave a pale yellow solid.
4-Hydrazinyl-2-methyl-6-(trifluoromethyl)pyrimidine (3): Pale yellow solid; yield 40.6%; m.p.113.4–114.8°C; 1H NMR (600 MHz, DMSO-d6) δ 8.45(s, 1H, pyrimidine-H), 7.14(s, 1H, pyrimidine-NH-), 4.60(s, 1H, -NH2), 2.86(s, 3H, -CH3).
Intermediate 3 (50 mmol) and triethylamine (75 mmol) were placed in a three-necked flask of 250 ml, anhydrous ethanol was added to dissolve. CS2 (75 mmol) was slowly added dropwise, stirred for 30 min, and then heated to reflux for 3 h; the reaction was detected by TLC. After the reaction was completed, the solvent was rotary-evaporated and water was added to suction filtration to remove insoluble matter. The filtrate was adjusted to pH 3 with 10% hydrochloric acid, placed in a refrigerator, and left to stand overnight, suction-filtered, and dried to obtain intermediate 4.
5-Methyl-7-(trifluoromethyl)-[1,2,4]triazolo [4,3-c]pyrimidine-3-thiol (4) White solid; yield 53.4%; m.p.143.7–144.2°C; 1H NMR (600 MHz, DMSO-d6) δ 8.42(s, 1H, pyrimidine-H), 2.91 (s, 3H, CH3-); 13C NMR (150 MHz, DMSO-d6) δ 165.31, 152.96, 152.82, 142.23(q, J = 35.1 Hz), 142.23(q, J = 272.25 Hz), 108.16, 20.11; MS (ESI) m/z: 233.1 ([M-H]-).
Intermediate 4 (10 mmol) was added into a triethylamine (15 mmol) aqueous solution, and after stirring at room temperature for 0.5 h, benzyl chloride (11 mmol) with different substituent was added, the reaction was carried out at room temperature, and the reaction was detected by TLC. After completion, the system was filtered to obtain the solid mixture. The mixture was purified by column chromatography to obtain the target compounds 5a–5s.
3-[(2,4-dichlorobenzyl)thio]-5-methyl-7-(trifluoromethyl)-[1,2,4]triazolo[4,3-c]pyrimidine (5q): White solid; yield 69.49%; m.p.72.1–74.6°C; 1H NMR (600 MHz, DMSO-d6) δ 8.32(s, 1H, Pyrimidine), 7.69(d, 1H, J = 7.8 Hz), 7.67(d, 1H, J = 1.8 Hz), 7.34(dd, 1H, J1 = 1.8 H, J2 = 6.6 Hz), 4.64(s, 2H, SCH2), 2.93(s, 3H, CH3); 13C NMR (150 MHz, DMSO-d6) δ 167.00, 152.58, 152.23, 141.98 (q, J = 35.5 Hz), 134.81, 134.32, 133.62, 133.27, 129.44, 127.95, 122.44 (q, J = 271.5Hz), 109.41, 32.75, 20.11; MS (ESI) m/z: 393.0([M + H]+), 415.0 ([M + Na]+).
The mycelial growth rates method (Du et al., 2021; Wang S. et al., 2021) was selected to evaluate the antifungal activities of the compounds 5a–5r against six phytopathogenic fungi, including cucumber Botrytis cinerea, tobacco Botrytis cinerea, blueberry Botrytis cinerea, Phytophthora infestans, strawberry Botrytis cinerea, and Pyricularia oryzae Cav. The tested compounds were dissolved in 0.5 ml dimethyl formamide (DMF) and then added 9.5 ml sterile water to prepare the test liquids. The test liquids were poured into 90 ml potato dextrose agar (PDA) to maintain the concentrations of the compounds, 50 μg/ml. Each treatment was replicated three times. The inoculated plates were fostered at 25 ± 1°C for 3–4 days. Tebuconazole and pyraclostrobin were acted as positive controls. The cross method was utilized to measure the diameter of the mycelium. The inhibition rate I (%) was calculated through the following formula, where C (cm) represents the average diameter of fungi growth on untreated PDA and T (cm) represents the average diameter of fungi on treated PDA.
The general synthetic route for the target compounds 5a–5s is depicted in Scheme 1. Acetamidine hydrochloride and ethyl trifluoroacetoacetate were used as raw materials. The target compounds 5a–5s were synthesized in five steps, including cyclization, chlorination, hydrazinolysis, cyclization, and thioetherification. The synthesized compounds were confirmed by 1H NMR, 13C NMR, and mass spectrometry.
In the 1H NMR data of compound 5q, a singlet appeared at 8.32 ppm indicated the presence of CH proton of the 8-trifluoromethylpyrimidine. A dd peak presented at 7.34 ppm signified the presence of CH proton in 3-phenyl. A singlet appeared at 4.64 ppm indicated the presence of CH of -SCH2- group. The singlet at 2.93 ppm indicated the CH3 proton in pyrimidine ring. Meanwhile, in the 13C NMR data of compound 5q, two quartets at 141.98 and 122.44 ppm indicated the presence of -CF3 in the pyrimidine fragment. Signals at 32.75 and 20.11 ppm indicated the presence of carbon in the -SCH2- and CH3. In addition, compound 5q was further confirmed by MS data with the ([M + H]+) and [M + Na]+peaks.
The antifungal activity of key intermediate 4 and target compounds 5a–5s was evaluated through the mycelial growth rates method. Most compounds exhibited some activity towards Botrytis cinerea (Table 1). As cucumber Botrytis cinerea, the inhibition rates of compounds 4, 5b, 5f, 5h, 5m, 5n, 5o, and 5r were 75.86, 77.78, 71.97, 72.31, 75.63, 76.58, 80.38, and 73.57%, respectively. Moreover, compounds 4, 5g, 5h, 5i, 5j, 5k, 5l, 5o, 5p, 5q, 5r, and 5s showed good antifungal activities against strawberry Botrytis cinerea and, the inhibition rates were 82.68, 72.89, 74.37, 76.35, 77.85, 77.32, 76.66, 75.31, 70.60, 71.52, 79.85, and 73.75%, respectively. In particular, four compounds 4, 5h, 5o, and 5r presented obvious activity to three of the four Botrytis cinerea. This also indicated the potential of these four compounds as leading structures or candidates against Botrytis cinerea.
TABLE 1. The antifungal activities of the compounds 4 and 5a–5s against plant pathogens of cucumber Botrytis cinerea, strawberry Botrytis cinerea, tobacco Botrytis cinerea, blueberry Botrytis cinerea, Phytophthora infestans, and Pyricularia oryzae Cav. in vitro at 50 μg/ml.
Further structure–activity relationship analysis signified that the introduction of halogen atom could improve the antifungal activities against Botrytis cinerea. Especially for strawberry Botrytis cinerea, it was more obvious. Moreover, the introduction of electron withdrawing group, such as -CN, -CF3, and -NO2, could increase the activity of compounds to a certain extent. For example, compounds 5i, 5j, 5k, and 5l appeared a good activity against strawberry Botrytis cinerea. In addition, most compounds represented ordinary activities towards Phytophthora infestans and Pyricularia oryzae Cav., which indicated these compounds were not favor to the inhibition of these two fungi.
In summary, a novel series of 1,2,4-triazolo[4,3-c]trifluoromethylpyrimidine derivatives bearing the thioether moiety were designed, synthesized, and characterized. The antifungal activities of the target compounds were also evaluated. Most compounds exhibited obvious antifungal activities against cucumber Botrytis cinerea, strawberry Botrytis cinerea, tobacco Botrytis cinerea, blueberry Botrytis cinerea, Phytophthora infestans and Pyricularia oryzae Cav. Notably, four compounds 4, 5h, 5o, and 5r were selected and showed significant antifungal activities against three of the four Botrytis cinerea. This indicated that these compounds are expected to become the leading structures or candidates for resistance to Botrytis cinerea.
The datasets presented in this study can be found in online repositories. The names of the repository/repositories and accession number(s) can be found below: Cambridge Crystallographic Data Centre, 2189490.
CL and NP contributed to the synthesis, purification, and characterization of all compounds and the activity research and prepared the original manuscript. QF and WW designed and supervised the research and revised the manuscript. All authors have read and agreed to the published version of the manuscript.
This research was financially supported by Science and Technology Fund Project of Guizhou [NO. (2020)1Z023; NO. QKHJC (2019)1015], Guizhou Provincial Department of Education Youth Science and Technology Talents Growth Project [NO. QJHKYZ (2018)291], the special funding of Guiyang science and technology bureau and Guiyang University [GYU-KY-(2021)].
The authors declare that the research was conducted in the absence of any commercial or financial relationships that could be construed as a potential conflict of interest.
All claims expressed in this article are solely those of the authors and do not necessarily represent those of their affiliated organizations, or those of the publisher, the editors and the reviewers. Any product that may be evaluated in this article, or claim that may be made by its manufacturer, is not guaranteed or endorsed by the publisher.
The Supplementary Material for this article can be found online at: https://www.frontiersin.org/articles/10.3389/fchem.2022.939644/full#supplementary-material
Abdel-Aziz, S. A., Taher, E. S., Lan, P., Asaad, G. F., Gomaa, H. A. M., El-Koussi, N. A., et al. (2021). Design, Synthesis, and Biological Evaluation of New Pyrimidine-5-Carbonitrile Derivatives Bearing 1,3-thiazole Moiety as Novel Anti-inflammatory EGFR Inhibitors with Cardiac Safety Profile. Bioorg. Chem. 111, 104890. doi:10.1016/j.bioorg.2021.104890
Abdelrehim, E.-s. M. (2021). Synthesis and Screening of New [1,3,4]Oxadiazole, [1,2,4]Triazole, and [1,2,4]Triazolo[4,3-B][1,2,4]triazole Derivatives as Potential Antitumor Agents on the Colon Carcinoma Cell Line (HCT-116). ACS Omega 6 (2), 1687–1696. doi:10.1021/acsomega.0c05718
Abu-Zaied, M. A., Elgemeie, G. H., and Mahmoud, N. M. (2021). Anti-covid-19 Drug Analogues: Synthesis of Novel Pyrimidine Thioglycosides as Antiviral Agents against SARS-COV-2 and Avian Influenza H5N1 Viruses. ACS Omega 6 (26), 16890–16904. doi:10.1021/acsomega.1c01501
Baillache, D. J., and Unciti-Broceta, A. (2020). Recent Developments in Anticancer Kinase Inhibitors Based on the Pyrazolo[3,4-D]pyrimidine Scaffold. RSC Med. Chem. 11 (10), 1112–1135. doi:10.1039/D0MD00227E
Basyouni, W. M., Abbas, S. Y., El‐Bayouki, K. A. M., Dawood, R. M., El Awady, M. K., and Abdelhafez, T. H. (2021). Synthesis and Antiviral Screening of 2‐(propylthio)‐7‐substituted‐thiazolo[5,4‐d]pyrimidines as Anti‐bovine Viral Diarrhea Virus Agents. J. Heterocycl. Chem. 58 (9), 1766–1774. doi:10.1002/jhet.4307
Cawrse, B. M., Lapidus, R. S., Cooper, B., Choi, E. Y., and Seley-Radtke, K. L. (2018). Anticancer Properties of Halogenated Pyrrolo[3,2-D ]pyrimidines with Decreased Toxicity via N5 Substitution. ChemMedChem 13 (2), 178–185. doi:10.1002/cmdc.201700641
Ding, M., Wan, S., Wu, N., Yan, Y., Li, J., and Bao, X. (2021). Synthesis, Structural Characterization, and Antibacterial and Antifungal Activities of Novel 1,2,4-Triazole Thioether and Thiazolo[3,2-B]-1,2,4-Triazole Derivatives Bearing the 6-Fluoroquinazolinyl Moiety. J. Agric. Food Chem. 69 (50), 15084–15096. doi:10.1021/acs.jafc.1c02144
Du, S., Yuan, Q., Hu, X., Fu, W., Xu, Q., Wei, Z., et al. (2021). Synthesis and Biological Activity of Novel Antifungal Leads: 3, 5-Dichlorobenzyl Ester Derivatives. J. Agric. Food Chem. 69 (51), 15521–15529. doi:10.1021/acs.jafc.1c04022
El-mahdy, K. M., and Farouk, O. (2021). Efficient Access to Some New Pyrimidine Derivatives and Their Antimicrobial Evaluation. J. Heterocycl. Chem. 58 (12), 2261–2269. doi:10.1002/jhet.4350
El-Shershaby, M. H., Ghiaty, A., Bayoumi, A. H., Ahmed, H. E. A., El-Zoghbi, M. S., El-Adl, K., et al. (2021). 1,2,4-Triazolo [4,3-c] Quinazolines: a Bioisosterism-Guided Approach towards the Development of Novel PCAF Inhibitors with Potential Anticancer Activity. New J. Chem. 45 (25), 11136–11152. doi:10.1039/D1NJ00710F
Fan, C. C., Jiao, S. L., Qin, M., and Zou, Z. H. (2019). 3, 5-Bis (2-Hydroxyphenyl)-1 H-1, 2, 4-Triazole Derivatives: Synthesis, Crystal Structure and Insecticidal Activity. ChemistrySelect 4 (29), 8593–8597. doi:10.1002/slct.201901706
Fayed, E. A., Nosseir, E. S., Atef, A., and El-Kalyoubi, S. A. (2022). In Vitro antimicrobial Evaluation and In Silico Studies of Coumarin Derivatives Tagged with Pyrano-Pyridine and Pyrano-Pyrimidine Moieties as DNA Gyrase Inhibitors. Mol. Divers 26 (1), 341–363. doi:10.1007/s11030-021-10224-4
Kesari, C., Rama, K. R., Sedighi, K., Stenvang, J., Björkling, F., Kankala, S., et al. (2021). Synthesis of Thiazole Linked Chalcones and Their Pyrimidine Analogues as Anticancer Agents. Synth. Commun. 51 (9), 1406–1416. doi:10.1080/00397911.2021.1884262
Kumar, S., and Narasimhan, B. (2018). Therapeutic Potential of Heterocyclic Pyrimidine Scaffolds. Chem. Central J. 12, 38. doi:10.1186/s13065-018-0406-5
O’Brien, P. A. (2017). Biological Control of Plant Diseases. Australas. Plant Pathol. 46, 293–304. doi:10.1007/s13313-017-0481-4
Pathak, P., Novak, J., Shukla, P. K., Grishina, M., Potemkin, V., and Verma, A. (2021). Design, Synthesis, Antibacterial Evaluation, and Computational Studies of Hybrid Oxothiazolidin-1,2,4‐triazole Scaffolds. Arch. Pharm. 354 (6), 2000473. doi:10.1002/ardp.202000473
Shao, W.-B., Wang, P.-Y., Fang, Z.-M., Wang, J.-J., Guo, D.-X., Ji, J., et al. (2021). Synthesis and Biological Evaluation of 1,2,4-Triazole Thioethers as Both Potential Virulence Factor Inhibitors against Plant Bacterial Diseases and Agricultural Antiviral Agents against Tobacco Mosaic Virus Infections. J. Agric. Food Chem. 69 (50), 15108–15122. doi:10.1021/acs.jafc.1c05202
Sun, S.-X., Yan, J.-H., Zuo, J.-T., Wang, X.-B., Chen, M., Lu, A.-M., et al. (2021). Design, Synthesis, Antifungal Evaluation, and Molecular Docking of Novel 1,2,4-triazole Derivatives Containing Oxime Ether and Cyclopropyl Moieties as Potential Sterol Demethylase Inhibitors. New J. Chem. 45 (40), 18898–18907. doi:10.1039/D1NJ03578A
Wang, R.-X., Du, S.-S., Wang, J.-R., Chu, Q.-R., Tang, C., Zhang, Z.-J., et al. (2021). Design, Synthesis, and Antifungal Evaluation of Luotonin A Derivatives against Phytopathogenic Fungi. J. Agric. Food Chem. 69 (48), 14467–14477. doi:10.1021/acs.jafc.1c04242
Wang, S., Li, Z.-R., Suo, F.-Z., Yuan, X.-H., Yu, B., and Liu, H.-M. (2019). Synthesis, Structure-Activity Relationship Studies and Biological Characterization of New [1,2,4]triazolo[1,5-A]pyrimidine-Based LSD1/KDM1A Inhibitors. Eur. J. Med. Chem. 167, 388–401. doi:10.1016/j.ejmech.2019.02.039
Wang, S., Yuan, X.-H., Wang, S.-Q., Zhao, W., Chen, X.-B., and Yu, B. (2021). FDA-approved Pyrimidine-Fused Bicyclic Heterocycles for Cancer Therapy: Synthesis and Clinical Application. Eur. J. Med. Chem. 214, 113218. doi:10.1016/j.ejmech.2021.113218
Wei, L., Zhang, J., Tan, W., Wang, G., Li, Q., Dong, F., et al. (2021). Antifungal Activity of Double Schiff Bases of Chitosan Derivatives Bearing Active Halogeno-Benzenes. Int. J. Biol. Macromol. 179, 292–298. doi:10.1016/j.ijbiomac.2021.02.184
Wu, W.-N., Jiang, Y.-M., Fei, Q., and Du, H.-T. (2019). Synthesis and Fungicidal Activity of Novel 1,2,4-triazole Derivatives Containing a Pyrimidine Moiety. Phosphorus, Sulfur, Silicon Relat. Elem. 194 (12), 1171–1175. doi:10.1080/10426507.2019.1633321
Wu, W., Chen, M., Wang, R., Tu, H., Yang, M., and Ouyang, G. (2019). Novel Pyrimidine Derivatives Containing an Amide Moiety: Design, Synthesis, and Antifungal Activity. Chem. Pap. 73, 719–729. doi:10.1007/s11696-018-0583-7
Yang, L., Sun, Y., Lu, Z., Liang, J., Wang, T., and Luo, J. (2022). Synthesis and Herbicidal Activity of Pyrimidyl‐1,2,4‐triazole Derivatives Containing Aryl Sulfonyl Moiety. J. Heterocycl. Chem 59 (4), 704–719. doi:10.1002/jhet.4410
Yang, Z., Sun, Y., Liu, Q., Li, A., Wang, W., and Gu, W. (2021). Design, Synthesis, and Antifungal Activity of Novel Thiophene/Furan-1,3,4-Oxadiazole Carboxamides as Potent Succinate Dehydrogenase Inhibitors. J. Agric. Food Chem. 69 (45), 13373–13385. doi:10.1021/acs.jafc.1c03857
Keywords: pyrimidine, synthesis, fungicidal activity, 1, 2, 4-triazol, Botrytis cinerea
Citation: Liu C, Fei Q, Pan N and Wu W (2022) Design, Synthesis, and Antifungal Activity of Novel 1,2,4-Triazolo[4,3-c]trifluoromethylpyrimidine Derivatives Bearing the Thioether Moiety. Front. Chem. 10:939644. doi: 10.3389/fchem.2022.939644
Received: 09 May 2022; Accepted: 20 May 2022;
Published: 19 July 2022.
Edited by:
Pei Li, Kaili University, ChinaReviewed by:
Dandan Xie, Guizhou University, ChinaCopyright © 2022 Liu, Fei, Pan and Wu. This is an open-access article distributed under the terms of the Creative Commons Attribution License (CC BY). The use, distribution or reproduction in other forums is permitted, provided the original author(s) and the copyright owner(s) are credited and that the original publication in this journal is cited, in accordance with accepted academic practice. No use, distribution or reproduction is permitted which does not comply with these terms.
*Correspondence: Qiang Fei, ZnFvcmdhbmljQDE2My5jb20=
†These authors have contributed equally to this work
Disclaimer: All claims expressed in this article are solely those of the authors and do not necessarily represent those of their affiliated organizations, or those of the publisher, the editors and the reviewers. Any product that may be evaluated in this article or claim that may be made by its manufacturer is not guaranteed or endorsed by the publisher.
Research integrity at Frontiers
Learn more about the work of our research integrity team to safeguard the quality of each article we publish.