- 1School of Biotechnology and Health Sciences, Wuyi University, Jiangmen, China
- 2International Healthcare Innovation Institute (Jiangmen), Jiangmen, China
- 3School of Chemical Engineering and Light Industry, Guangdong University of Technology, Guangzhou, China
- 4Key Laboratory of Drug Research, Shanghai Institute of Materia Medica, Chinese Academy of Sciences, Shanghai, China
Two new patchoulene sesquiterpenoid glycosides (1–2), a natural patchoulane-type sesquiterpenoid (3) and a natural cadinene-type sesquiterpenoid (4), were isolated from the aerial parts of Pogostemon cablin (Blanco) Benth., together with eleven known sesquiterpenoids (5–15) and eleven known flavonoids (16–26). Their chemical structures were elucidated on the basis of spectroscopic methods, including NMR, HRESIMS, IR, and CD spectroscopic data analysis, as well as chemical hydrolysis. The isolated compounds 1–13 and 15–26 were tested for inhibitory effects on the proliferation of HepG2 cancer cells. Among them, compounds 17 and 19 displayed anti-proliferative effects against HepG2 cells with IC50 values of 25.59 and 2.30 μM, respectively. Furthermore, the flow cytometry analysis and Western blotting assays revealed that compound 19 significantly induced apoptosis of HepG2 cells by downregulating the ratio of Bcl-2/Bax and upregulating the expression of cleaved caspase-3 and cleaved caspase-9. Therefore, the potential pharmaceutical applications of P. cablin would be applied according to our study findings.
Introduction
Pogostemon cablin (Blanco) Benth. is an annual herb known as patchouli, a form of traditional Chinese medicine for the treatment of upset stomach, vomiting, diarrhea, headache, and fever. The plant, a member of the genus Pogostemon, Lamiaceae family, was native to South and Southeast Asia, such as Indonesia, Malaysia, Philippines, and India, and was introduced to China in the 9th century as a spice. It was fostered in Guangdong, Guangxi, Fujian, and Taiwan provinces in China (Feng et al., 1994). In recent years, a large number of phytochemical studies on P. cablin had been carried out to concentrate on the constituents of this plant, which showed the presence of various monoterpenes and sesquiterpenoids (Hikino et al., 1968; Terhune et al., 1973), triterpenoids (Huang et al., 2009), steroids (Kongkathip et al., 2009), flavonoids (Ding et al., 2009), alkaloids (Büchi et al., 1966), and glycosides (Wang et al., 2010). The reported constituents from P. cablin possessed marked activities such as antibacterial activity, anti-influenza virus, anti-inflammation, cytotoxicity, antimutagenic activity, antiplatelet aggregation, and insecticidal activity (Li et al., 2013). In our continuous phytochemical studies on the constituents of this medicinal herb, two new patchoulene sesquiterpenoid glycosides (1–2), a natural patchoulane-type sesquiterpenoid (3) and a natural cadinene-type sesquiterpenoid (4), as well as eleven known sesquiterpenoids (5–15) and eleven known flavonoids (16–26), were isolated and characterized. In addition, the anti-proliferative activities of the isolated compounds (1–13 and 15–26) against HepG2 cancer cells were assessed in this article. Furthermore, the apoptosis-inducing effects of compound 19 in HepG2 cells were also investigated in the current study. To the best of our knowledge, the apoptosis-induced activity of compound 19 is reported for the first time.
Experiment
General Experimental Procedures
Column chromatographies (CC) were carried out with silica gel (200–300 mesh, Qingdao Marine Chemical Factory), silica gel for chromatography C18 SMB 100–20/45 (Fuji Silysia Chemical Ltd), and Sephadex LH-20 (Pharmacia Biotech AB). TLC was performed using precoated silica gel GF254 plates (Yantai Chemical Industry Research Institute). MPLC (medium pressure liquid chromatography) was carried out on a Buchi Pure C-815 apparatus. High-performance liquid chromatography (HPLC) was carried out on a Waters 1500-Series system. The semi-preparative C18 column used was the SunFire® C18 OBD (250 × 10 mm) apparatus. UPLC was carried out on a Waters Acquity UPLC-Class instrument. IR data were obtained with a KBr pellet on a Thermo Scientific Fourier Transform NICOLET iS5 Infrared Spectrometer (Waltham, USA). High-resolution electrospray ion mass (HRESIMS) was performed on a Thermo Scientific Q-Exactive mass spectrometer (Waltham, USA). 1D and 2D NMR data were recorded on a Bruker AVANCE NEO 500 spectrometer (Bremen, Germany) with chloroform-d and methanol-d4 as solvents. Chemical shift values were expressed in δ (ppm) relative to tetramethylsilane (TMS) as the internal standard. An Anton Paar MCP 200 automatic polarimeter (Graz, Austria) was used to determine optical rotations in ACN at 25°C. CD spectra were obtained on a Chirascan spectrometer (England, United Kingdom) at room temperature using a 0.2-cm standard cell. All solvents used in column chromatography and HPLC were of analytical grade (Guangzhou Chemical Reagents Company Ltd., Guangzhou, China) and chromatographic grade (Thermo Fisher), respectively.
Plant Material
The aerial parts of P. cablin were collected in September 2019, GuangXi Province, China. The plant material was identified by Professor Xiaoji Zheng, and a voucher specimen (201909PC) was deposited at Wuyi University, Jiangmen, China.
Extraction and Isolation
The air-dried aerial parts of P. cablin (13.0 kg) were powdered and extracted with 95% ethanol (3 × 30 L) at room temperature for 6 days. The extract was evaporated under reduced pressure (45°C) to afford a brown residue (2.0 kg), which was suspended in water (5 L) and extracted sequentially with petroleum ether, EtOAc (ethyl acetate), and n-BuOH at room temperature. The EtOAc fraction (178 g) was subjected to a silica gel (200–300 mesh) column using a gradient petroleum ether (PE)-EtOAc system (100:1, 50:1, 30:1, 10:1, 5:1, 1:1, and 0:1, each 15 L) as eluents to afford 19 fractions (Fr. 1–Fr. 19). Fr. 4 (2.8 g) was subjected to Sephadex LH-20 and yielded two subfractions, namely, (Fr. 4.1- Fr. 4.2) and Fr. 4.2 (1.2 g), which were further purified by using a reversed phase (C18 SMB 100–20/45, 30 g, H2O-MeOH: 40:1 to 0:100) column to obtain compound 10 (432 mg). Fr. 7 (4.5 g) was submitted to a silica gel (200–300 mesh) column using PE and EtOAc as eluents (50:1 to 0:1) to afford five subfractions (Fr. 7.1–Fr. 7.5); Fr. 7.4 (950 mg) was submitted to Sephadex LH-20 and eluted with the CHCl2–CH3OH (1:1) system to obtain compound 12 (7 mg), Fr. 7.3 (530 mg) was submitted to Sephadex LH-20 and eluted with the CHCl2–CH3OH (1:1) system to afford compounds 18 (77 mg) and 24 (44 mg). Fr. 8 (5.5 g) was subjected to Sephadex LH-20 and eluted with the CHCl2–CH3OH (1:1) system to afford two subfractions (Fr. 8.1 and Fr. 8.2), and Fr. 8.2 (1.6 g) was further purified by using a silica gel (200–300 mesh) column using PE and EtOAc as eluents (100:1 to 0:1) to obtain compound 23 (373 mg). Fr. 9 (15.5 g) was subjected to a reversed phase (C18 SMB 100–20/45, 30 g, H2O-MeOH: 30:1 to 0:100) column, and four subfractions (Fr. 9.1–Fr. 9.4) were yielded, and Fr. 9.1 (200 mg) was further purified by semi-preparative HPLC (0–60 min: isocratic 80% CH3CN in water) to yield compounds 4 (25 mg) and 5 (15 mg); Fr. 9.2 (330 mg) was purified by semi-preparative HPLC (0–60 min: isocratic 80% CH3CN in water) to obtain 6 (27 mg) and 9 (20 mg); Fr. 9.4 (500 mg) was subjected to semi-preparative HPLC (0–60 min: isocratic 80% CH3CN in water) to afford compounds 16 (4 mg), 20 (14 mg), and 25 (48 mg). Fr. 10 (4.1 g) was submitted to a silica gel (200–300 mesh) column using PE and EtOAc as eluents (30:1 to 0:1) to obtain Fr. 10.1–Fr. 10.3, and then, Fr. 10.3 (2.0 g) was further purified by Sephadex LH-20 to obtain compound 19 (75 mg). Fr. 12 (5.4 g) was subjected to Sephadex LH-20 to afford two subfractions (Fr. 12.1 and Fr. 12.2). Fr. 12.1 (1.3 g) was further purified using a reversed phase (C18 SMB 100–20/45, 30 g, H2O-MeOH: 30:1 to 0:100) column to obtain compounds 3 (20 mg) and 22 (5 mg). Fr. 12.2 (150 mg) was subjected to semi-preparative HPLC (0–60 min: isocratic 60% CH3CN in water) to afford compounds 7 (1 mg), 13 (1 mg), 14 (1 mg), and 17 (41 mg). Fr. 13 (1.3 g) was submitted to a silica gel (200–300 mesh) column using PE and EtOAc as eluents (50:1 to 0:1) to yield two subfractions (Fr. 13.1 and Fr. 13.2), and Fr. 13.1 (300 mg) was subjected to Sephadex LH-20 and a reversed phase (C18 SMB 100–20/45, 30 g, H2O-MeOH: 30:1 to 0:100) column to obtain compounds 11 (5 mg), 21 (8 mg), 8 (26 mg), and 26 (12 mg). Fr. 15 (6.3 g) was submitted to a silica gel (200–300 mesh) column using PE and EtOAc as eluents (30:1 to 0:1) and further purified by using a reversed phase (C18 SMB 100–20/45, 30 g, H2O-MeOH: 40:1 to 0:100) column to obtain compound 15 (5 mg). Fr. 19 (11.5 g) was subjected to a silica gel column (200–300 mesh, 5 × 40 cm, 120 g) using a gradient CH2Cl2-MeOH system (10:1, 5:1, 1:1, 0:1) to obtain two subfractions (Fr. 19.1 and Fr. 19.2). Fr. 19.1 (2.0 g) was submitted to a reversed phase (C18 SMB 100–20/45, 30 g, H2O-MeOH: 50:1 to 0:100) column and semi-preparative HPLC (0–60 min: isocratic 40% CH3CN in water) to yield compounds 1 (6 mg) and 2 (6 mg).
Compound Characterization
Compound 1: an amorphous white powder; [α]25 D = −35.5 (c 0.1, MeOH); IR (KBr) νmax 3,449, 1,638, 1,075, and 536 cm−1; 1H and 13C NMR data, see Table 1 and Table 2; HRESIMS m/z 427.2318 [M + HCOO]- (calcd for C22H35O8, 427.2326).
Compound 2: an amorphous white powder; [α]25 D = −25.3 (c 0.1, MeOH); IR (KBr) νmax 3,449, 2,947, 1,638, 1,384, 1,077, and 536 cm−1; 1H and 13C NMR data, see Table 1 and Table 2; HRESIMS m/z 427.2320 [M + HCOO]- (calcd for C22H35O8, 427.2326).
Compound 3: colorless oil; [α]25 D = −37.0 (c 0.1, MeOH); IR (KBr) νmax 3,449, 2,964, 2,956, 2,922, 2,851, 1,685, 1,603, 1,261, 1,166, 1,105 and 1,026 cm−1; 1H and 13C NMR data, see Table 1 and Table 2; HRESIMS m/z 281.2109 [M + H]+ (calcd for C17H29O3, 281.2111).
Compound 4: an amorphous white powder; [α]25 D = −38.0 (c 0.1, MeOH); IR (KBr) νmax 3,474, 2,962, 2,923, 2,856, 1707, 1,644, 1,451, and 1,394 cm−1; 1H and 13C NMR data, see Table 1 and Table 2; HRESIMS m/z 237.1849 [M + H]+ (calcd for C15H25O2, 237.1849).
Quantum Chemical ECD Calculation
Electronic circular dichroism (ECD) was applied to establish the absolute configurations of 1′ and 2′, according to the reported method (Stephens and Harada, 2010). According to the key correlations observed in the NOESY spectrum, CHEM3D software with the MM2 force field was applied to search the preliminary conformational distribution. Geometric optimization of compounds 1′ and 2′ was calculated with the density functional theory (DFT) method and time-dependent DFT (TDDFT) via the Gaussian 09 program (Gaussian, Inc., Wallingford CT, USA). The optimized conformers obtained were submitted to CD calculation by the TDDFT [B3LYP/6-31G(d)] method. The computational data were fitted in the Origin 2021 (OriginLab Corporation, Northampton, MA, USA).
Acid Hydrolysis
Each compound (1–2 mg) was hydrolyzed with 1 M HCl (4 mL) for 4 h at 80°C. The reaction mixture was extracted with ethyl acetate 3 times (Zhang et al., 2020). The water layer was evaporated repeatedly under reduced pressure with MeOH until dryness, giving a monosaccharide residue. It was dissolved in anhydrous pyridine (1 mL), and L-cysteine methyl ester hydrochloride (3 mg) was added. The mixture was kept at 60°C for 1 h. Then, 5 μL (0.5 mg) O-tolylisothiocyanate was added and stirred at 60°C for another 1 h. The reaction mixture was directly analyzed by reversed-phase HPLC. The absolute configurations of sugars of compounds 1 and 2 were determined by comparing the retention times with derivatives of standard sugars prepared in a similar manner. Retention times for derivatives were 12.2 min (L-glucose) and 12.9 min (D-glucose), respectively. Meanwhile, compounds 1 and 2 were hydrolyzed to obtain compounds 1′ and 2′, respectively.
Anti-Proliferative Activity Assay (MTT Assay)
The antiproliferative activity of the isolated compounds was evaluated against the growth of hepatocellular carcinoma cells HepG2 (cells were grown in Dulbecco’s modified Eagle medium containing 10% FBS at 37°C) using an MTT assay. HepG2 cells were seeded in 96-well plates at a density of 5,000 cells per well and cultured in a 37°C incubator for 24 h (Tang et al., 2020). After 24 h, the medium was removed, and a fresh medium containing different compounds with a series of concentration gradients was added to each well and cultured in a 37°C incubator for 72 h. A measure of 20 μL (5 mg/mL) of the MTT solution was added to each well and cultured in a 37°C incubator for 4 h. Then, the supernatant was removed, and DMSO (100 μL) was added to each well. The optical density was measured at a wavelength of 490 nm after 15 min of shaking. The IC50 values of each compound were calculated with GraphPad Prism 8.0 software (GraphPad Software Inc., San Diego, CA, USA).
Flow Cytometry
HepG2 cells were seeded in 6-well plates (1 × 106 cells/well) for 24 h. Then, the medium was removed, and a fresh medium containing different concentrations of compound 19 (1, 2.5, and 5 μM) was added and cultured again for 48 h. Gemcitabine (GEM) was used as a positive control. After culture, the cells were collected and washed twice with cold PBS; binding buffer solution was added to the collected cell precipitate to make the cell concentration reach 1 × 106/mL. Then, 100 μL of the cell suspension was reabsorbed into the new centrifuge tube, and 5 μL Annexin V-FITC and 5 μL PI were added. After incubation at room temperature for 15 min (in a dark place), 400 μL binding buffer was added to each well. Fluorescence of cells was immediately detected with a flow cytometer and used for quantitative analysis.
Western Blotting
HepG2 cells were seeded in 6-well plates (1 × 106 cells/well) for 24 h. Then, the medium was removed, and a fresh medium containing different concentrations of compound 19 (1, 2.5, and 5 μM) was cultured for another 48 h. The medium was removed and washed with cold PBS; cells were collected and lysed with RIPA buffer, left to stand in an ice bath for 30 min, and centrifuged at 4°C for 20 min at the highest speed. The protein concentration was detected by using the BCA protein assay kit. Proteins were separated by SDS-PAGE gels and transferred to the PVDF membrane. The membrane was blocked with 5% non-fat milk for 2 h. Then, specific primary antibodies were used to bind to the corresponding proteins and left for incubation at 4°C overnight, after washing thrice with TBST (5 min), followed by incubation with a second antibody at room temperature for 50 min. The protein bands were detected using the ECL detection kit, and the gray intensities of the bands were measured by ImageJ software.
Statistical Analysis
All data are presented as the mean ± SD of at least three independent experiments. The means were compared by one-way ANOVA, followed by Dunnett’s test by GraphPad Prism 8.0 software. When the p-value was less than 0.05, the difference between groups was considered statistically significant.
Results and Discussion
Structure Elucidation of New Compounds
Compound 1 was afforded as an amorphous white powder. The molecular formula of compound 1 was determined to be C21H34O6 based on its HRESIMS data (m/z 427.2318 [M + HCOO]-, calcd for C22H35O8 427.2326), indicating the existence of five degrees of unsaturation. The IR spectrum showed characteristic absorptions attributable to a cyclic olefinic bond (1,638 cm−1) and a hydroxyl (3,449 cm−1) bond. The 1H NMR spectrum (Table 1) of compound 1 revealed the presence of an olefinic proton [δH 5.02 (1H, m)] and three methyl groups [δH 0.80 (3H, s), 0.90 (3H, s), and 1.02 (3H, s)]. The 13C NMR and DEPT spectra (Table 2) showed 21 carbon signals due to three methyls, six methylenes, nine methines, and three quaternary carbons. Comprehensive interpretation of the 1D and 2D NMR spectral data allowed full assignment of the 1H and 13C NMR signals of compound 1 (Table 1 and Table 2). The aforementioned data indicated the presence of a β-D-glucopyranosyl moiety (Xiao et al., 2011) [δC 104.8, 78.2, 77.9, 75.2, 71.7, and 62.8]. Acid hydrolysis of compound 1 with 1 mol/L HCl afforded compound 1′ and β-D-glucose that was identified by direct comparison with an authentic sample.
Analysis of 1H–1H COSY in combination with HSQC showed correlations from H-2 to H-9, as shown by bold lines in Figure 1. In the HMBC spectrum, as shown in Figure 1, the correlations were observed from H-15 to C-1/C-9, H-2 to C-10/C-5/C-4, H-12 to C-10/C-7, H-13 to C-10/C-7, and H-14 to C-5/C-3/C-1′. Comprehensive interpretation of 1D and 2D NMR spectra of compound 1 revealed the presence of a patchoulene-type sesquiterpene unit in 1, which was similar to δ-patchoulene (Faraldos et al., 2010). However, its carbon spectrum NMR data have not been reported in the literature. The observed key HMBC correlations between H-14 and C-1′, as well as between H-1′ and C-14, indicated that the two units were linked via the C-14–O–C-1′ bond to form a patchoulene-type sesquiterpenoid glycoside. Thus, the planar structure of compound 1 was established (Figure 1).
The relative configuration of compound 1 was established by the NOESY interactions (Figure 2). The correlations between H-14a and H-6a/H-6b and between H-14a/H-14b and H-13 indicated the β-orientation of H-4, H-5, H-7, and CH3-15. Thus, the relative configuration of the sesquiterpenoid unit in compound 1 was determined.
Finally, according to the quantum chemical electronic circular dichroism (ECD) calculation, as shown in Figure 3, the absolute configuration of compound 1 was deduced to be 4R, 5R, 7S, and 10S by a comparison of the experimental ECD spectrum of compound 1′ with the calculated one. Therefore, compound 1 was determined to be (4R, 5R, 7S, 10S)-14-hydroxypatchoulene-14-O-β-D-glucopyranoside, and named Pogopatchoulene A.
Compound 2 was afforded as an amorphous white powder. The molecular formula of compound 2 was deduced to be C21H34O6 by its HRESIMS [M + HCOO]- ion at m/z 427.2320 (calcd for C22H35O8 427.2326), corresponding to five degrees of unsaturation. The UV and IR spectra of compound 2 displayed similar signals to those of 1. The 13C NMR spectrum revealed the presence of 21 carbon signals including three methyls, seven methylenes, seven methines, and four quaternary carbons. The 1D NMR signals of compound 2 closely resembled those of 1, indicating that they possessed a similar structure except for the presence of a tetrasubstituted double-bond group in compound 2 instead of a trisubstituted double-bond group in compound 1. A comprehensive comparison of their 2D NMR data revealed that the tetrasubstituted double-bond group in compound 2 was in positions 1 and 5 instead of positions 1 and 2 in compound 1. Furthermore, compound 2 was hydrolyzed with 1 mol/L HCl to obtain compound 2′ and β-D-glucose. Hence, the planar structure of compound 2 was established.
The relative configuration of compound 2 could be suggested by the interpretation of the NOESY data, as shown in Figure 2; the cross peaks between H-14a/H-14b and H-13 and between H-14a and H-6a indicated that H-4, H-7, and CH3-15 were in β-orientation. Finally, the absolute configuration of compound 2 was determined to be 4S, 7, and 10R, according to the quantum chemical ECD calculation presented in Figure 3. In conclusion, compound 2 was determined as (4S, 7R, 10R)-14-hydroxypatchoulene-14-O-β-D-glucopyranoside, and named Pogopatchoulene B.
Compound 3 was shown to have the molecular formula C17H28O3 by its HRESIMS data (m/z 281.2109 [M + H]+, calcd for C17H29O3: 281.2111). The UV spectrum showed maximal absorption at 286 nm. The IR spectra revealed the characteristic absorptions for hydroxyl (3,449 cm−1) and carbonyl (1,685 cm−1) bonds. The 1H NMR spectrum displayed the signals for four methyl protons [δH 0.84 (3H, s, H-14), 1.07 (6H, overlapped, H-13 and H-12), and 2.04 (3H, s, H-17)]. The 13C NMR and DEPT spectra displayed 17 carbon signals including four methyls, six methylenes, three methines, and four quaternary carbons. Comparison of the NMR data of compound 3 with the known compound patchoulan-1, 15-diol (8) suggested that their NMR signals were similar except for extra acetyl [δH 2.04 (3H, s), δC 21.1, 171.4] in compound 3. With the aid of 1H-1H COSY, HSQC, and HMBC experiments, all the 1H and 13C NMR signals were assigned, as shown in Table 1 and the relative configuration is shown in Figure 4, which was in accordance with a reaction product named patchouli alcohol acetate (Niwa et al., 1987). However, no relevant nuclear magnetic data on patchouli alcohol acetate had been reported in the literature. Therefore, we reported its carbon spectrum for the first time.
The molecular formula of compound 4 was established as C15H24O2 by a quasi-molecular ion peak at m/z 237.1849 [M + H]+ (calcd for C15H25O2: 237.1849) in its HRESIMS. IR spectra showed the characteristic absorptions for hydroxyl (3,474 cm−1), carbonyl (1707 cm−1), and olefinic bonds (1,644 cm−1). The 1H NMR spectrum displayed two olefinic protons [δH 4.65 (1H, m) and 4.50 (1H, s)] and three methyl groups [δH 1.10 (3H, d), 1.20 (3H, d), and 1.80 (3H, s)]. We compared the NMR data on compound 4 with those of the synthetic compound (+)-[2R-(2α, 4aβ, 5α, 8β, 8aβ)]-octahydro-4a-hydroxy-2, 5-dimethyl-8-(1-methylethenyl)-1(2H)-naphthalenone (Gijsen et al., 1994), which were exactly the same. Hence, compound 4 was determined.
In a summary, two new patchoulene sesquiterpenoid glycosides (1–2), a natural patchoulane-type sesquiterpenoid (3) and a natural cadinene-type sesquiterpenoid (4) were isolated and determined from P. cablin. Other known compounds, as rel-(1S, 4R, 5R, 7R, 10R)-10-desmethyl-10-hydroxy-1-methyl-3-oxo-11-eudesmene (5) (Chavez et al., 1995), corymbolone (6) (Garbarino et al., 1985), 1β, 4β-dihydroxyeudesman-11-ene (7) (Li et al., 2005), patchoulan-1, 15-diol (8) (Ding et al., 2011), 2, 3, 4, 4α, 5, 6, 8, 8α-octayhydro-1-hydroxy-4, 8α, 9, 9-tetramethyl-1, 6-methanonaphthalen-7(1H)-one (9) (Barton et al., 1987), patchouli alcohol (10) (Barton et al., 1987), 3R-3-hydroxypatchoulol (11) (Aleu et al., 2001), pogostol (12) (Stierle et al., 2003), 1S, 4R, 5S, 6R, 7S, 10S-1 (5), 6 (7)-diepoxy-4-guaiol (13) (Chen et al., 2020), (5R, 8S)-3-hydroxy-3, 8-dimethyl-5-(prop-1-en-2-yl)-3, 4, 5, 6, 7, 8-hexahydroazulen-1(2H)-one (14) (Huang et al., 2015), 8-keto-9 (10)-α-patchoulene-4α-ol (15) (Li et al., 2013), 3,7-dihydroxy-5, 3′, 4′-trimethoxyflavone (16) (Dong et al., 1999), velutin (17) (Zahir et al., 1996), 3, 5-dihydroxy-7, 4' -dimethoxy flavone (18) (Zhang et al., 2001), 5, 4′-dihydroxy-7-methoxyflavone (19) (Zhang et al., 2001), 5-hydroxy-3, 7, 3′, 4′-tetramethoxyflavone (20) (Wang et al., 2020), (2R, 3R)-(+)-4′, 7-di-O-methyldihydroquercetin (21) (Yoon et al., 2019), 7, 3′, 4′-tri-O-methyldhq (22) (Kiehlmann and Slade, 2003), 5-hydroxy-7, 3′, 4′-trimethoxyflavanone (23) (Zhang et al., 2001), 7, 4′-dimethylapigenin (24) (Chen et al., 2017), ereiodictyol-7, 3′-dimethyl ether (5, 4′-dihydroxy-7, 3′-dimethoxyflavanone) (25) (Vasconcelos et al., 1998), and (2S)-5, 3′, 4′ trihydroxy-7-methoxy-flavanone (26) (Vasconcelos et al., 1998) were also obtained and established by comparing with reported spectroscopic data in the literature reports. Compounds 5–7, 9, 13, 16, 21, 22, and 26 were isolated from this plant for the first time.
Anti-Proliferative Effects of Isolates
An ethanol crude extract of P. cablin displayed in vitro anti-tumor potential in our preliminary experiments. Herein, most isolates (1–13 and 15–26) were evaluated for antiproliferative activities in HepG2 cells. Among the tested samples, compounds 17 and 19 displayed anti-proliferative effects against HepG2 cells with IC50 values of 25.59 and 2.30 μM, respectively. This observation was in agreement with reported data (Huong et al., 2005), in which the IC50 value of compound 19 against HepG2 cells was 1.60 μM. Previous studies have revealed that flavonoids with 2 and 3 double bonds have a higher ability to induce cell apoptosis than flavonols (Wang et al., 1999; Hui et al., 2014; Isoda et al., 2014). In addition, the presence of hydroxyl groups at the 4′ positions of the B ring is important for granulocyte differentiation (Takahashi et al., 1998; Isoda et al., 2014). According to our data, flavone compound 19 showed the best antiproliferative effects against HepG2 cells than other flavonoids; it can be concluded that 3-methoxy and 4′- hydroxyl might be important for its activity. The other compounds including sesquiterpenoids and dihydroflavones were inactive, as shown in Table 3. Our data suggest that the anti-tumor effects of P. cablin might be attributed, at least partially, to its bioactive flavonoids.
Compound 19 Induce Apoptosis in HepG2 Cells
Apoptosis plays an important role in maintaining tissue homeostasis (Ma, et al., 2018). Induction of apoptosis in tumor cells is an important survival strategy against cancer pathologic progression (Rejhová, et al., 2018), and many natural products have been shown to have anticancer effects through the mitochondrial-mediated apoptosis pathway (Friedman, 2015). The cytotoxic effects of compound 19 on HepG2 have been reported, but the mechanism of action has not been studied. Therefore, the apoptosis-inducing effects of compound 19 in HepG2 cells were also investigated in the current study. As shown in Figure 5, after treatment with compound 19 (1, 2.5, and 5 μM) for 48 h, the flow cytometric apoptosis experiment showed a dose-dependent increase in the percentage of early apoptotic cells when compared with the control group, which showed significant difference (p < 0.001).
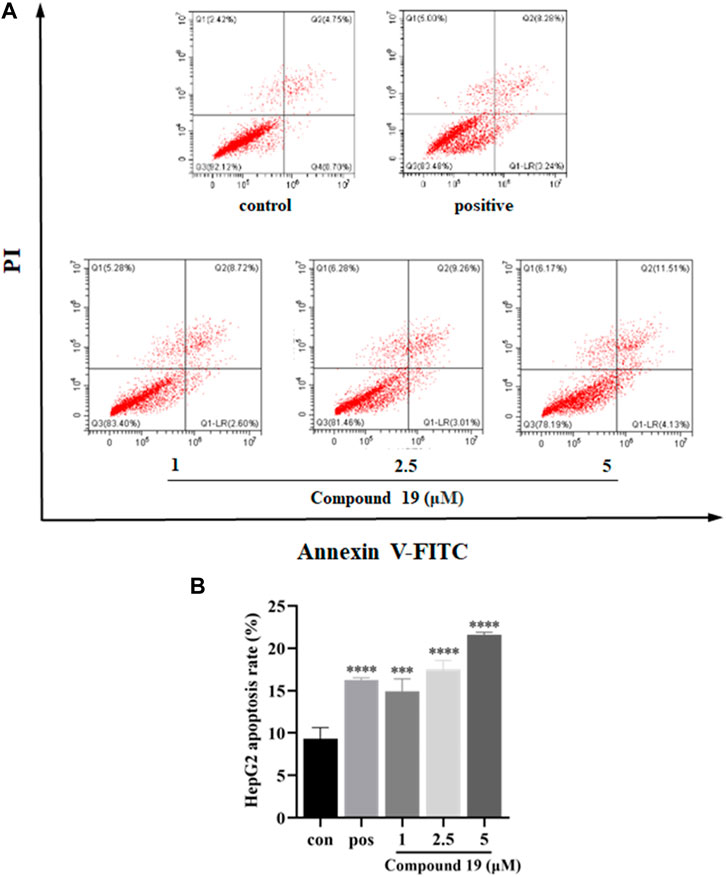
FIGURE 5. Impact of compound 19 on apoptosis of HepG2 cells. Cells were seeded in 6-well plates (1 × 106 cells/well) and treated with compound 19 (1, 2.5, and 5 μM) for 48 h. Gemcitabine (GEM) was used as a positive control. Annexin V-FITC/PI staining flow cytometry was used to analyze the apoptosis of HepG2 cells after treatment with compound 19; analysis is shown in (A). The experiments were run in triplicate. Data were presented as mean ± SD. The explicit analysis is shown in (B). ∗p < 0.05, ∗∗p < 0.01, and ∗∗∗p < 0.001 vs. the control group.
Additionally, caspase-9 is an important initiator caspase within the cascade of apoptosis transduction in the mitochondrial-mediated apoptosis pathway. Upon proapoptotic factor release into the cytosol, procaspase-9 is recruited at apoptosome and self-cleaved to caspase-9 and then stimulates downstream executioner caspases, such as caspase-3, which subsequently cause apoptotic cell death (Brentnall et al., 2013; Sadeghi et al., 2019). Our results showed that compared with the control group, compound 19 significantly upregulated the expressions of cleaved caspase-9 and cleaved caspase-3 and significantly downregulated the expressions of caspase-3 and caspase-9. Moreover, both Bcl-2 (an anti-apoptotic protein) and Bax (a proapoptotic protein) belong to the B-cell lymphoma (Bcl-2) family and are critical in the mitochondrial-mediated apoptotic pathway. Western blotting results are shown in Figure 6; compared with the control group, compound 19 significantly upregulated the expressions of Bax and downregulated the expressions of Bcl-2. Thus, compound 19 may induce apoptosis in HepG2 cells and activate the mitochondrial-mediated apoptotic pathway. Our results showed for the first time that compound 19 exerted anti-proliferative effects by inducing apoptotic cell death.
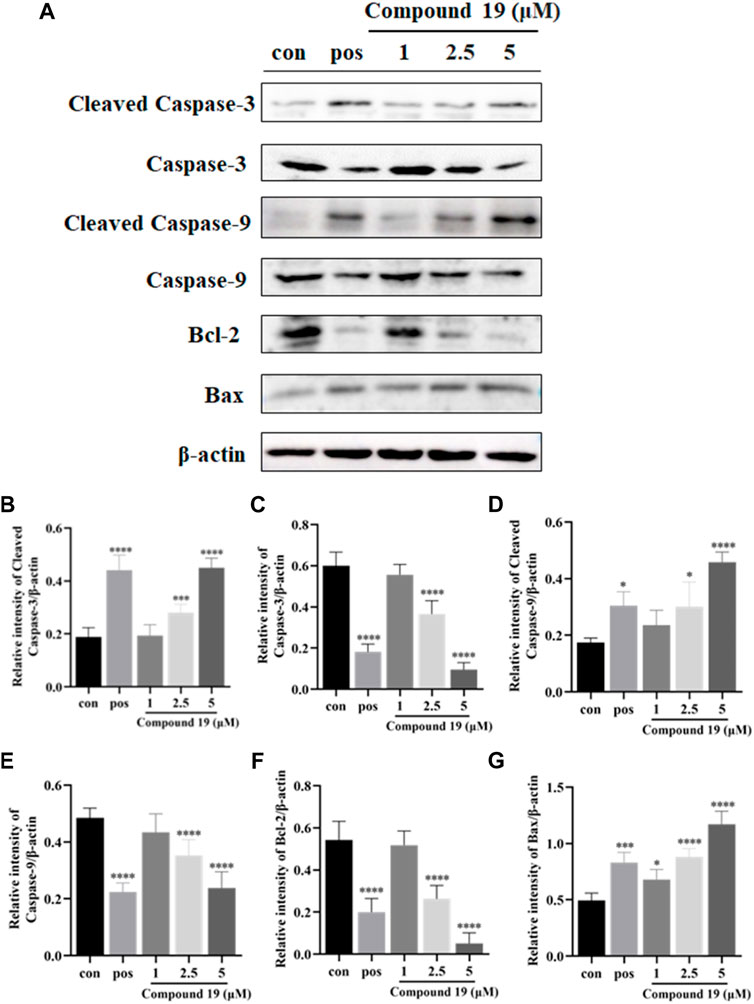
FIGURE 6. Impact of compound 19 on the protein expressions of cleaved caspase-3, cleaved caspase-9, caspase-3, caspase-9, Bcl-2, and Bax in HepG2 cells. Cells were seeded in 6-well plates (1 × 106 cells/well) and treated with different concentrations of compound 19 (1, 2.5, and 5 μM) for 48 h. Western blotting was used to detect the protein expression levels after compound 19 was applied to HepG2 cells (A). The ratio of the corresponding protein to β-actin is calculated in (B–G). The experiments were run in triplicate. Data were presented as mean ± SD. ∗p < 0.05, ∗∗p < 0.01, and ∗∗∗p < 0.001 vs. the control group.
Conclusion
In conclusion, this study was a phytochemical investigation that explored the chemical profiles and pharmacological properties of active constituents from P. cablin. Twenty-six compounds including two new patchoulene sesquiterpenoid glycosides and two natural sesquiterpenoids were isolated from the aerial parts of P. cablin, and the absolute configurations of new compounds (1–2) were established. The antiproliferative activities against the human tumor cell line (HepG2) of the isolated compounds (1–13 and 15–26) were examined by the MTT assay. Compounds 17 and 19 displayed anti-proliferative effects with IC50 values of 25.59 and 2.30 μM against HepG2 cells, respectively. In this article, the related mechanism of the anti-proliferative activity of compound 19 has been further studied, and it was found to induce the apoptosis of HepG2 cells by downregulating the ratio of Bcl-2/Bax and upregulating the expressions of cleaved caspase-3 and cleaved caspase-9. Therefore, findings from the current study supported that the chemical constituents from P. cablin were multitudinous, which were beneficial for the further fundamental and development research of P. cablin.
Data Availability Statement
The original contributions presented in the study are included in the article/Supplementary Material; further inquiries can be directed to the corresponding authors.
Author Contributions
XP: data curation, validation, and writing—original draft preparation. SA: software and writing—original draft preparation. YZ: data curation and software. FF: data curation and software. MW: data curation. PL: data curation. YW: software. LG: software and methodology. KZ: supervision and writing—reviewing and editing. DL: supervision, conceptualization, methodology, writing—reviewing and editing. JY: supervision and writing—reviewing and editing.
Funding
This work was supported by grants from the Department of Education of Guangdong Province (Nos. 2017KSYS010 and 2019KZDZX2003) and the Jiangmen Program for Innovative Research Team (No. 2018630100180019806).
Conflict of Interest
The authors declare that the research was conducted in the absence of any commercial or financial relationships that could be construed as a potential conflict of interest.
The handling editor SW declared a past co-authorship with the author KZ.
Publisher’s Note
All claims expressed in this article are solely those of the authors and do not necessarily represent those of their affiliated organizations, or those of the publisher, the editors, and the reviewers. Any product that may be evaluated in this article, or claim that may be made by its manufacturer, is not guaranteed or endorsed by the publisher.
Supplementary Material
The Supplementary Material for this article can be found online at: https://www.frontiersin.org/articles/10.3389/fchem.2022.938851/full#supplementary-material
References
Aleu, J., Hanson, J. R., Hernández Galán, R., and Collado, I. G. (2001). Biotransformation of the Fungistatic Sesquiterpenoids Patchoulol, Ginsenol, Cedrol and Globulol by Botrytis Cinerea. J. Mol. Catal. B Enzym. 11, 329–334. doi:10.1016/S1381-1177(00)00014-X
Barton, D. H. R., Bel?il, J.-C., Billion, A., Boivion, J., Lallemand, J.-Y., and Mergui, S. (1987). Functionalisation of Saturated Hydrocarbons. Part 9. Oxidation of Patchouli Alcohol by the ‘gif System’: Isolation and Organoleptic Properties of Three New Ketonic Derivatives. Helv. Chim. Acta 70, 273–280. doi:10.1002/hlca.19870700202
Brentnall, M., Rodriguez-Menocal, L., De Guevara, R. L., Cepero, E., and Boise, L. H. (2013). Caspase-9, Caspase-3 and Caspase-7 Have Distinct Roles during Intrinsic Apoptosis. BMC Cell. Biol. 14 (1), 1–9. doi:10.1186/1471-2121-14-32
Büchi, G., Goldman, I. M., and Mayo, D. W. (1966). The Structures of Two Alkaloids from Patchouli Oil. J. Am. Chem. Soc. 88, 3109–3113. doi:10.1021/ja00965a040
Chavez, J. P., Gottlieb, O. R., and Yoshida, M. (1995). 10-Desmethyl-1-methyl-eudesmanes from Ocotea Corymbosa. Phytochemistry 39, 849–852. doi:10.1016/0031-9422(94)00977-2
Chen, Y. M., Cao, N. K., Tu, P. F., and Jiang, Y. (2017). Chemical Constituents from Murraya Euchrestifolia. Zhongguo Zhong Yao Za Zhi 42, 1916–1921. doi:10.19540/j.cnki.cjcmm.20170228.002j
Chen, Y. W., Dong, F. W., Qin, T. L., Zhang, F., Wu, S. L., Yang, M. Y., et al. (2020). Terpenoids from Stems and Leaves of Aphanamixis Grandifolia. Chin. J. Exp. Traditional Med. Formulae 26, 168–173. doi:10.13422/j.cnki.syfjx.20201311
Dahai, W., Yin, Z., Zhang, Q., Ye, W., Zhang, X., and Zhang, J. (2010). Nonvolatile Chemical Constituents from Pogostemon Cablin. Cjcmm 35, 2704–2707. doi:10.4268/cjcmm20102014
Ding, W.-B., Lin, L.-D., Liu, M.-F., and Wei, X.-Y. (2011). Two New Sesquiterpene Glycosides fromPogostemon Cablin. J. Asian Nat. Prod. Res. 13, 599–603. doi:10.1080/10286020.2011.577424
Ding, W., Liu, M., Wei, X., and Lin, L. (2009). Strong Polarity Components of Pogostemon Cablin (Blance) Benth. J. Trop. Subtropical Bot. 17, 610–616. doi:10.3969/j.issn.1005-3395.2009.06.017
Dong, H., Gou, Y.-L., Cao, S.-G., Chen, S.-X., Sim, K.-Y., Goh, S.-H., et al. (1999). Eicosenones and Methylated Flavonols from Amomumkoenigii. Phytochemistry 50, 899–902. doi:10.1016/S0031-9422(98)00622-0
Faraldos, J. A., Wu, S., Chappell, J., and Coates, R. M. (2010). Doubly Deuterium-Labeled Patchouli Alcohol from Cyclization of Singly Labeled [2-2H1]Farnesyl Diphosphate Catalyzed by Recombinant Patchoulol Synthase. J. Am. Chem. Soc. 132, 2998–3008. doi:10.1021/ja909251r
Feng, C., Sui, C., and Wu, H. (1994). Synthesizing Site and Storing Position of Essential Oil in Pogostemon Cablin. Chin. Traditional Herb. Drugs 38, 116–119.
Friedman, M. (2015). Chemistry and Anticarcinogenic Mechanisms of Glycoalkaloids Produced by Eggplants, Potatoes, and Tomatoes. J. Agric. Food Chem. 63 (13), 3323–3337. doi:10.1021/acs.jafc.5b00818
Garbarino, J. A., Gambaro, V., and Chamy, M. C. (1985). The Structure of Corymbolone, an Eudesmane Sesquiterpenoid Keto-Alcohol from Cyperus Corymbosus. J. Nat. Prod. 48, 323–325. doi:10.1021/np50038a023
Gijsen, H. J. M., Wijnberg, J. B. P. A., and de Groot, A. (1994). Thermal Rearrangement of Bicyclogermacrane-1,8-Dione. Synthesis of Humulenedione and (−)-cubenol, Starting from Natural (+)-Aromadendrene-V. Tetrahedron 50, 4745–4754. doi:10.1002/chin.19943323510.1016/s0040-4020(01)85013-4
Hikino, H., Ito, K., and Takemoto, T. (1968). Structure of Pogostol. Chem. Pharm. Bull. 16, 1608–1610. doi:10.1248/cpb.16.1608
Huang, A.-C., Sefton, M. A., Sumby, C. J., Tiekink, E. R. T., and Taylor, D. K. (2015). Mechanistic Studies on the Autoxidation of α-Guaiene: Structural Diversity of the Sesquiterpenoid Downstream Products. J. Nat. Prod. 78, 131–145. doi:10.1021/np500819f
Huang, L., Mu, S., Zhang, J., Deng, B., Song, Z., and Hao, X. (2009). Chemical Constituents from Involatile Moiety of Pogostemon Cablin. Zhongguo Zhong Yao Za Zhi 34, 410–413. doi:10.3321/j.issn:1001-5302.2009.04.010
Hui, H., Chen, Y., Yang, H., Zhao, K., Wang, Q., Zhao, L., et al. (2014). Oroxylin A Has Therapeutic Potential in Acute Myelogenous Leukemia by Dual Effects Targeting PPARγ and RXRα. Int. J. Cancer 134 (5), 1195–1206. doi:10.1002/ijc.28435
Huong, D. T., Luong, D. V., Thao, T. T., and Sung, T. V. (2005). A New Flavone and Cytotoxic Activity of Flavonoid Constituents Isolated from Miliusa Balansae (Annonaceae). Pharmazie 60, 627–629. doi:10.1002/chin.200550192
Isoda, H., Motojima, H., Onaga, S., Samet, I., Villareal, M. O., and Han, J. (2014). Analysis of the Erythroid Differentiation Effect of Flavonoid Apigenin on K562 Human Chronic Leukemia Cells. Chemico-Biological Interact. 220, 269–277. doi:10.1016/j.cbi.2014.07.006
Kiehlmann, E., and Slade, P. W. (2003). Methylation of Dihydroquercetin Acetates: Synthesis of 5-O-Methyldihydroquercetin. J. Nat. Prod. 66, 1562–1566. doi:10.1021/np034005w
Kongkathip, N., Sam-ang, P., Kongkathip, B., Pankaew, Y., Tanasombat, M., and Udomkusonsri, P. (2009). Development of Patchouli Extraction with Quality Control and Isolation of Active Compounds with Antibacterial Activity. Agric. Nat. Resour. 43, 519–525.
Li, F., Li, C.-J., Ma, J., Yang, J.-Z., Chen, H., Liu, X.-M., et al. (2013). Four New Sesquiterpenes from the Stems of Pogostemon Cablin. Fitoterapia 86, 183–187. doi:10.1016/j.fitote.2013.03.010
Li, X., Yang, M., Han, Y.-F., and Gao, K. (2005). New Sesquiterpenes fromErigeron Annus. Planta Med. 71, 268–272. doi:10.1055/s-2005-837827
Ma, Z.-J., Lu, L., Yang, J.-J., Wang, X.-X., Su, G., Wang, Z.-l., et al. (2018). Lariciresinol Induces Apoptosis in HepG2 Cells via Mitochondrial-Mediated Apoptosis Pathway. Eur. J. Pharmacol. 821, 1–10. doi:10.1016/j.ejphar.2017.12.027
Niwa, H., Hasegawa, T., Ban, N., and Yamada, K. (1987). Stereocontrolled Total Synthesis of (±)-norpatchoulenol and Two Metabolites of Patchouli Alcohol, (±)-hydroxy Patchouli Alcohol and the Corresponding (±)-carboxylic Acid. Tetrahedron 43, 825–834. doi:10.1016/S0040-4020(01)90019-5
Rejhová, A., Opattová, A., Čumová, A., Slíva, D., and Vodička, P. (2018). Natural Compounds and Combination Therapy in Colorectal Cancer Treatment. Eur. J. Med. Chem. 144, 582–594. doi:10.1016/j.ejmech.2017.12.039
Sadeghi, S., Davoodvandi, A., Pourhanifeh, M. H., Sharifi, N., ArefNezhad, R., Sahebnasagh, R., et al. (2019). Anti-cancer Effects of Cinnamon: Insights into its Apoptosis Effects. Eur. J. Med. Chem. 178, 131–140. doi:10.1016/j.ejmech.2019.05.067
Stephens, P. J., and Harada, N. (2009). ECD Cotton Effect Approximated by the Gaussian Curve and Other Methods. Chirality 22, 229–233. doi:10.1002/chir.20733
Stierle, A. A., Stierle, D. B., Goldstein, E., Parker, K., Bugni, T., Baarson, C., et al. (2003). A Novel 5-HT Receptor Ligand and Related Cytotoxic Compounds from an Acid Mine Waste Extremophile. J. Nat. Prod. 66, 1097–1100. doi:10.1021/np030044w
Takahashi, T., Kobori, M., Shinmoto, H., and Tsushida, T. (1998). Structure-activity Relationships of Flavonoids and the Induction of Granulocytic- or Monocytic-Differentiation in HL60 Human Myeloid Leukemia Cells. Biosci. Biotechnol. Biochem. 62 (11), 2199–2204. doi:10.1271/bbb.62.2199
Tang, S., Zhang, X.-T., Ma, Y.-B., Huang, X.-Y., Geng, C.-A., Li, T.-Z., et al. (2020). Artemyrianolides A-S, Cytotoxic Sesquiterpenoids from Artemisia Myriantha. J. Nat. Prod. 83, 2618–2630. doi:10.1021/acs.jnatprod.0c00396
Terhune, S. J., Hogg, J. W., and Lawrence, B. M. (1973). Cycloseychellene, a New Tetracyclic Sesquiterpene from. Tetrahedron Lett. 14, 4705–4706. doi:10.1016/S0040-4039(01)87315-9
Vasconcelos, J. M. J., Silva, A. M. S., and Cavaleiro, J. A. S. (1998). Chromones and Flavanones from Artemisia Campestris Subsp. Maritima. Phytochemistry 49, 1421–1424. doi:10.1002/chin.19991127010.1016/s0031-9422(98)00180-0
Wang, I.-K., Lin-Shiau, S.-Y., and Lin, J.-K. (1999). Induction of Apoptosis by Apigenin and Related Flavonoids through Cytochrome C Release and Activation of Caspase-9 and Caspase-3 in Leukaemia HL-60 Cells. Eur. J. Cancer 35 (10), 1517–1525. doi:10.1016/S0959-8049(99)00168-9
Wang, Z.-H., Li, Q., Huang, M., Xu, P.-F., Yang, L.-P., Zhai, Y.-Y., et al. (2020). Chemical Constituents of Callicarpa Macrophylla. Chem. Nat. Compd. 56, 1125–1127. doi:10.1007/s10600-020-03243-4
Xiao, H. M., Zu, L. B., Li, S. P., Wang, K. J., and Li, N. (2011). Chemical Constituents from Dried Fruits of Rubus Chingii. Chin. J. Med. Chem. 21, 220–226. doi:10.1007/s10570-010-9464-0
Yoon, K. D., Lee, J.-Y., Kim, T. Y., Kang, H., Ha, K.-S., Ham, T.-H., et al. (2019). In Vitro and In Vivo Anti-hyperglycemic Activities of Taxifolin and its Derivatives Isolated from Pigmented Rice (Oryzae Sativa L. Cv. Superhongmi). J. Agric. Food Chem. 68, 742–750. doi:10.1021/np034005w10.1021/acs.jafc.9b04962
Zahir, A., Jossang, A., Bodo, B., Provost, J., Cosson, J.-P., and Sévenet, T. (1996). DNA Topoisomerase I Inhibitors: Cytotoxic Flavones from Lethedon Tannaensis. J. Nat. Prod. 59 (7), 701–703. doi:10.1021/np960336f
Zhang, G. W., Ma, X. Q., and Su, J. Y. (2001). Flavonoids Isolated from Pogostemon Cablin (In English). Chin. Traditional Herb. Drugs 32, 870–873. doi:10.7501/j.issn.0253-2670.2001.10.2001010478
Keywords: Pogostemon cablin, sesquiterpenoid, flavonoid, antiproliferative activity, apoptosis
Citation: Peng X, Ang S, Zhang Y, Fan F, Wu M, Liang P, Wen Y, Gan L, Zhang K, Li D and Yue J (2022) Chemical Constituents With Antiproliferative Activity From Pogostemon cablin (Blanco) Benth.. Front. Chem. 10:938851. doi: 10.3389/fchem.2022.938851
Received: 08 May 2022; Accepted: 13 June 2022;
Published: 15 July 2022.
Edited by:
Shao-Hua Wang, Lanzhou University, ChinaReviewed by:
Yi Zhang, Guangdong Ocean University, ChinaNai-Yun Ji, Yantai Institute of Coastal Zone Research (CAS), China
Copyright © 2022 Peng, Ang, Zhang, Fan, Wu, Liang, Wen, Gan, Zhang, Li and Yue. This is an open-access article distributed under the terms of the Creative Commons Attribution License (CC BY). The use, distribution or reproduction in other forums is permitted, provided the original author(s) and the copyright owner(s) are credited and that the original publication in this journal is cited, in accordance with accepted academic practice. No use, distribution or reproduction is permitted which does not comply with these terms.
*Correspondence: Kun Zhang, a3poYW5nQGdkdXQuZWR1LmNu; Dongli Li, d3l1Y2hlbWxkbEAxMjYuY29t
†These authors have contributed equally to this work and share first authorship