- State Key Laboratory Breeding Base of Green Pesticide and Agricultural Bioengineering, Key Laboratory of Green Pesticide and Agricultural Bioengineering Ministry of Education, Guizhou University, Guiyang, China
An acid-catalyzed 2-alkylation of indole molecules is developed. Only catalytic amount of the commercially available, inexpensive and traceless HI is used as the sole reaction promoter. 2,3-Disubstituted indole molecules bearing congested tertiary carbon centers are afforded as the final products in moderate to excellent yields.
Introduction
Indole and its derivatives are versatile molecules and have found significant applications in biological and medicinal research (Ma et al., 2016) (Figure 1A). For example, the indole derivative neoechinulin A has been isolated from P. griseofulvum and Aspergillus sp. and shown significant cytotoxic activity against P388 cells (Ma et al., 2016). Typrostatin A and B can be obtained from A. fumigatus, which is present in the sea sediment and the Ficus carica in both Japan and China. They have exhibited excellent antiphytopathogenic activity and have been used in the investigation of novel anti-tumor reagents (Ma et al., 2016). Therefore, the development of simple and efficient methods for functionalization of indole molecules is attractive and important to both scientific research and drug manufacturing.
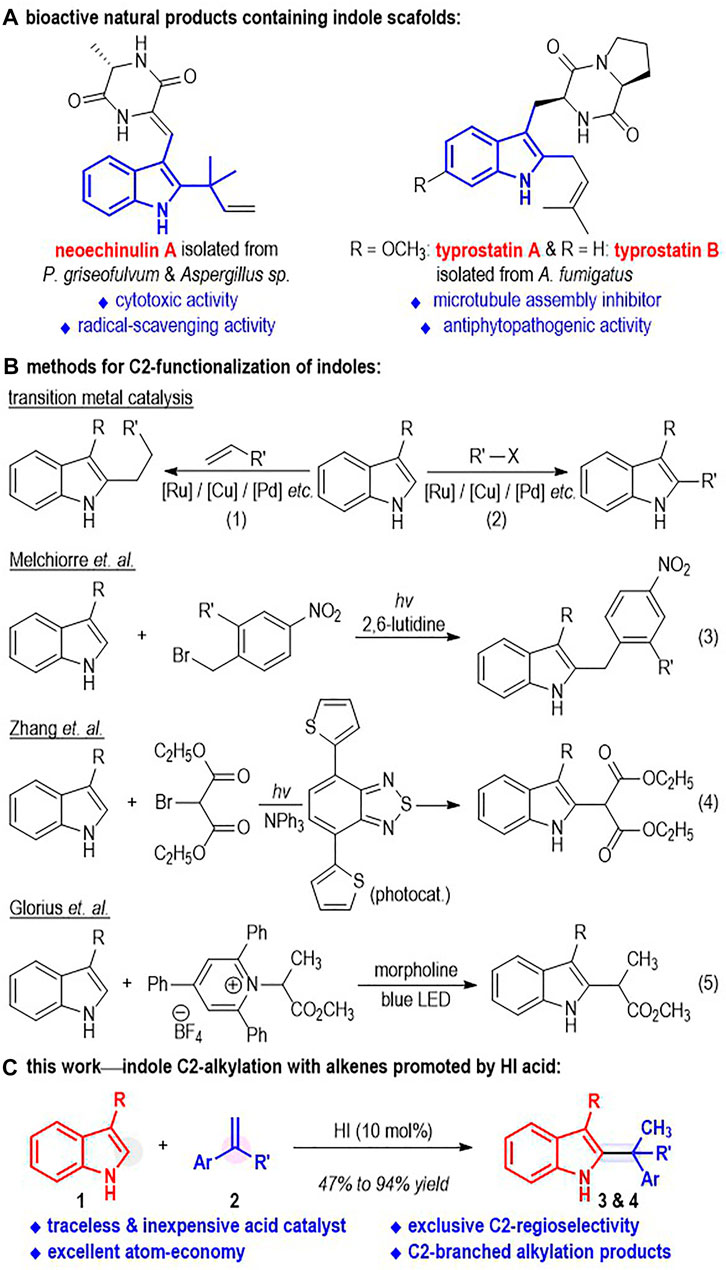
FIGURE 1. Bioactive Indole Derivatives bearing C2-Substituents and C2-Functionalizations of Unprotected Indoles.
Indole can be functionalized at each position around its aromatic structure through various transformations. Traditionally, the C3-positions of indole molecules have been widely used as nucleophiles to react with a variety of electrophiles in both enantioselective (Austin and MacMillan et al., 2002; Zhou and Tang et al., 2002; Evans et al., 2005; Wang et al., 2006; Terada et al., 2007; Bandini et al., 2009; Joucla and Djakovitch et al., 2009; Bartoli et al., 2010) and non-chiral fashion (Bartoli et al., 2005; Kimura et al., 2005; Moran et al., 2006; Kusurkar et al., 2008; Lerch et al., 2014). Transition metals, amines and Lewis acids are frequently used as effective catalysts for these reactions. The C2-positions of the N-protected indoles can undergo transition metal-catalyzed alkylations (Doyle et al., 2010; Johansen and Kerr et al., 2010; Jiao and Bach et al., 2011; Pan et al., 2012; Lin et al., 2013; Yoshino et al., 2013; Su et al., 2014; Soni et al., 2018; Wang and Wang, 2021), arylations (Lane and Sames, 2004; Wang et al., 2005; Deprez et al., 2006; Lebrasseur and Larrosa et al., 2008; Phipps et al., 2008; Yang et al., 2008; Ackermann and Lygin, 2011; Sauermann et al., 2011; Li et al., 2016; Yang and Shi, 2018), alkenylations (Nakao et al., 2006; Maehara et al., 2008; Ding and Yoshikai, 2012; Li et al., 2013a; Li et al., 2013b; Liang et al., 2014; Schramm et al., 2015; Wong et al., 2015; Zhang W et al., 2015; Zhou et al., 2016), alkynylations (Yang et al., 2010; Tolnai et al., 2013; Sauermann et al., 2015; Zhang Z Z et al., 2015; Kong et al., 2016), aminations (Sun et al., 2014; Sun et al., 2015) and thiolation reactions (Gensch et al., 2016). A directing group is generally required to be installed on the N atom of the indole molecule for the C2-functional group introductions in these transformations. In contrast, intermolecular reactions for direct functionalization of the C2-positions of the unprotected indoles have been relatively less developed (Figure 1B). Success within this direction includes the transition metal-catalyzed indole C2-alkylations with alkyl halides (Straathof et al., 2014; Shao et al., 2015; Yang et al., 2015) and alkenes (Weng et al., 2016; Zhou et al., 2017; Bai et al., 2020) (Figure 1B, eq. 1 and eq. 2). Melchiorre (Kandukuri et al., 2015) and co-workers have disclosed the formation of the electron donor-acceptor (EDA) complex between indoles and electron-deficient benzylbromides and reported a metal-free photo-catalyzed direct indole C2-alkylation via formation of this EDA complex (eq. 3). Zhang (Wang et al., 2016) and co-workers reported in 2016 the organic semiconductor-catalyzed, visible light-promoted indole C2-alkylation with 2-bromomalanates (eq. 4). Recently, Glorius (James et al., 2019) and co-workers used the pyridinium salt as the EDA complex acceptor and realized the indole C2-alkylation reaction under basic conditions with white light irradiation (eq. 5). To the best of our knowledge, the direct and metal-free C2-alkylation of indoles with unactivated alkenes has not been disclosed.
Herein, we report an acid-promoted regioselective C2-alkylation reaction of unprotected indoles 1 (Figure 1C). Unactivated alkenes 2 are used as the alkylation reagent, with no sacrificing atoms or functional groups lost during this transformation. The use of EDA acceptors can be avoided in this protocol. A traceless and inexpensive HI is used in a catalytic amount as the sole reaction catalyst. The C2-branched alkylation products 3 or 4 bearing a tertiary carbon center are afforded in excellent regioselective fashion with good to excellent isolated yields. The reaction features excellent atom-economy and C2-regioselectivity.
The reaction was initially carried out by using 3-methylindole 1a and 1,1-diphenylethene 2a as the substrates in dichloromethane under the catalysis of different organic and inorganic acids (Table 1, entries 1–7). To our delight, the indole C2-branched alkylation product 3a can be obtained in promising yields with a variety of strong acids after stirring at 30°C for 12 h (entries 1–6). The target product of 3a could be afforded in 21% yield in the presence of 30 mol% of HCl acid (entry 1). The yield of 3a could be dramatically improved to 87% when switching the HCl into HBr (entry 2). Gratifyingly, the product 3a was obtained in 92% yield when using HI as the acid catalyst (entry 3). Other organic or inorganic acids gave the desired product 3a in lower yields (entries 4–6). Notably, the reaction could not happen when using acetic acid as the reaction catalyst, which is probably due to its weak acidity (entry 7). A diversity of organic solvents could be used as the reaction mediate (entries 8–11). For example, the reaction went on smoothly in the solvents of EtOAc, hexane and toluene, with the desired product 3a afforded in good yields (entries 8–10). However, solvents with high polarities such as H2O, DMF, THF, CH3OH and MTBE could not be used for this transformation (entry 11). The amount of the HI catalyst could be decreased to 0.1 mol% without obvious erosion of the product yield (entries 12–13). Further decreasing the amount of the HI to 0.05 mol% resulted in significant loss of the yield (entry 14). The reaction temperature could also be decreased to 25°C with the desired product of 3a afforded in an even higher yield (entry 15).
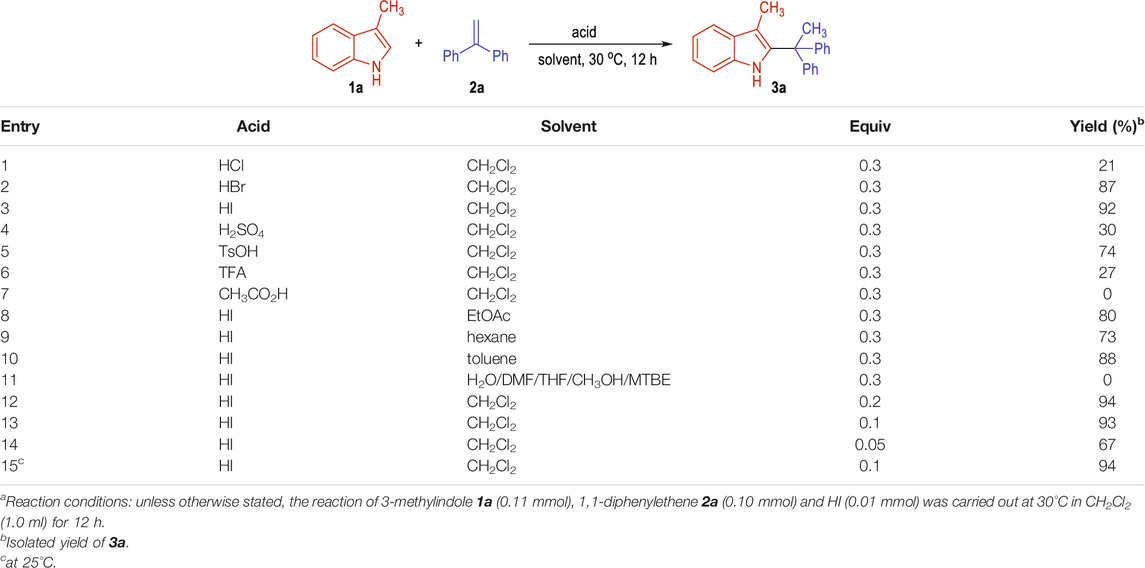
TABLE 1. Optimization of reaction conditions.a
Having identified the optimal reaction condition for the HI-induced indole C2-alkylation, we next evaluated the scope of this transformation using indole substrates 1 bearing different substituents (Scheme 1). Both electron-donating and electron-withdrawing substituents are well tolerated on the benzene rings of the indole structure, with the target C2-alkylated indole products afforded in moderate to excellent yields (Scheme 1, 3a to 3m). We also examined the effect of the substitution position of the C5 with NO2 group, but gave no desired product. The C3-methyl group on the indole molecule can be changed into other alkyl groups, with the desired C2-alkylated indole products afforded in a bit lower yields (e.g., 3n to 3o). However, switching the C3-methyl group on the reaction substrates into a C3-phenyl group resulted in no formation of the target product.
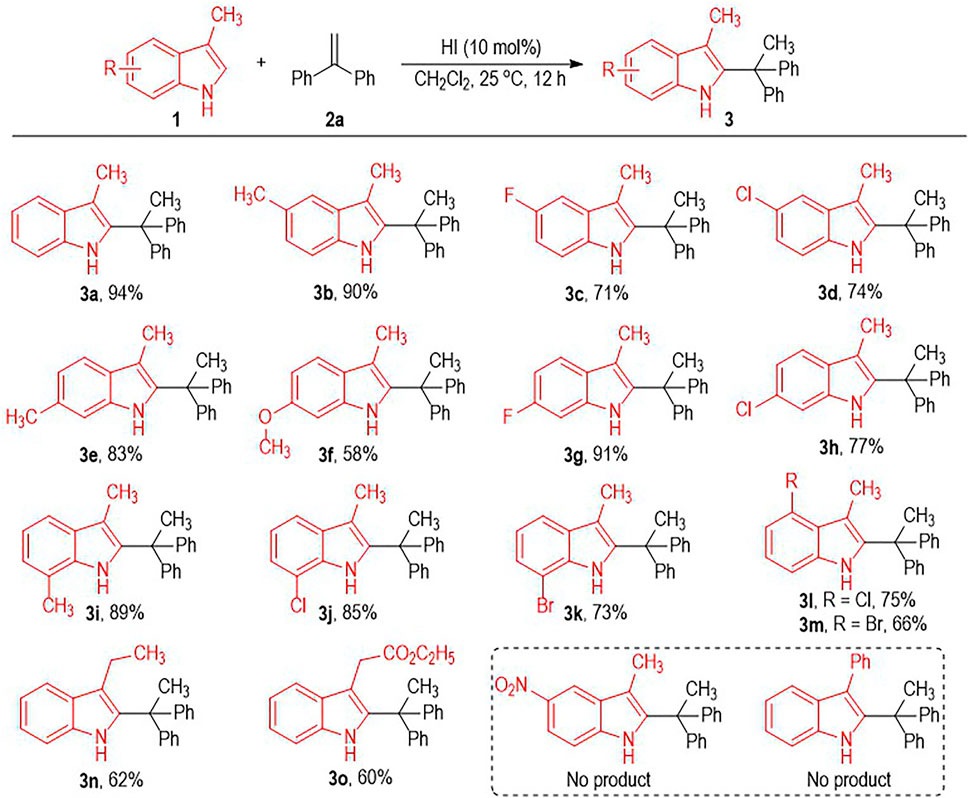
SCHEME 1. Scope of Indoles 1.a. a Reaction conditions as stated in Table 1, entry 15. Yields are isolated yields after purification by column chromatography.
The alkene substrates 2 can also tolerate diverse substituents and substitution patterns (Scheme 2). Various substituents can be introduced to the para-and meta-positions of the phenyl rings on 2a, with the corresponding products afforded in good to excellent yields (Scheme 2, 4a to 4f). However, installing substituents on the ortho-position of the phenyl rings on 2a leads to no formation of the desired products, which is probably due to the substantially increased steric hindrance during the C2-branched product formation process. One of the phenyl rings on 2a can be switched into a naphthyl or a thiophenyl group with retention of the good to excellent product yields (4g to 4h). To our delight, one of the two aryl groups on the alkene substrates can be replaced by a methyl group without much erosion on the product yield (4i to 4j). It is worth noting that the internal alkene of 1,1-diphenylpropene also works well in this photo-induced indole C2-alkylation process, with the target product 4k afforded in 55% yield. It is not surprising that the 1,1-diarylethenes bearing two substituted phenyl groups generally give the desired indole C2-branched alkylation products in excellent yields (4l to 4m).
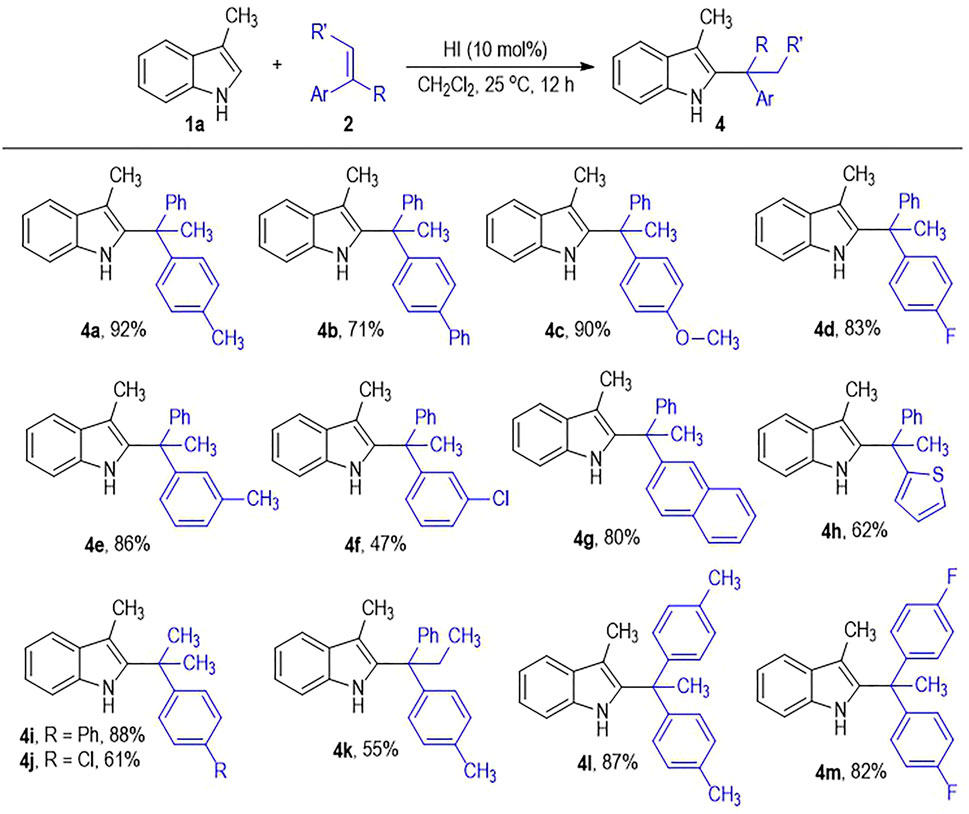
SCHEME 2. Scope of Alkenes 2.a. a Reaction conditions as stated in Table 1, entry 15. Yields are isolated yields after purification by column chromatography.
The HI-induced C2-alkylation reaction of the 3-methylindole 1a with 2a can be carried out at Gram scales to give the functionalized indole product 3a in an excellent yield (Scheme 3). The indole NH group on 3a can be efficiently protected by CH3I and the N-methylindole product 5 can be afforded in almost quantitative yield.
Conclusion
In summary, we have disclosed a metal-free reaction for the synthesis of 2-alkylation of 3-alkylindole derivatives. 1,1-Disubstituted alkenes are used as the alkylation reagent with the C2-branched alkylated indole products afforded in generally good to excellent yields with excellent Markovnikov regioselectivity. A catalytic amount of the commercially available and inexpensive HI is used as the sole reaction catalyst. Further investigations towards the applications of the C2-functionalized indole molecules are in progress in our laboratory.
Data Availability Statement
The original contributions presented in the study are included in the article/Supplementary Material, further inquiries can be directed to the corresponding author.
Author Contributions
XP conducted most of the experiments. QL and YN participated in some of the experiments. XP conceptualized and directed the whole project. XP drafted the manuscript. All of the authors contributed in scientific discussions.
Funding
We acknowledge financial support from the Program of Introducing Talents of Discipline to Universities of China (111 Program, D20023) at Guizhou University, Frontiers Science Center for Asymmetric Synthesis and Medicinal Molecules, Department of Education, Guizhou Province (Qianjiaohe KY (2020)004), and Guizhou University (China).
Conflict of Interest
The authors declare that the research was conducted in the absence of any commercial or financial relationships that could be construed as a potential conflict of interest.
Publisher’s Note
All claims expressed in this article are solely those of the authors and do not necessarily represent those of their affiliated organizations, or those of the publisher, the editors, and the reviewers. Any product that may be evaluated in this article, or claim that may be made by its manufacturer, is not guaranteed or endorsed by the publisher.
Supplementary Material
The Supplementary Material for this article can be found online at: https://www.frontiersin.org/articles/10.3389/fchem.2022.860764/full#supplementary-material
References
Ackermann, L., and Lygin, A. V. (2011). Ruthenium-Catalyzed Direct C-H Bond Arylations of Heteroarenes. Org. Lett. 13, 3332–3335. doi:10.1021/ol2010648
Austin, J. F., and MacMillan, D. W. C. (2002). Enantioselective Organocatalytic Indole Alkylations. Design of a New and Highly Effective Chiral Amine for Iminium Catalysis. J. Am. Chem. Soc. 124, 1172–1173. doi:10.1021/ja017255c
Bai, J.-F., Zhao, L., Wang, F., Yan, F., Kano, T., Maruoka, K., et al. (2020). Organocatalytic Formal (3 + 2) Cycloaddition toward Chiral Pyrrolo[1,2-A]indoles via Dynamic Kinetic Resolution of Allene Intermediates. Org. Lett. 22, 5439–5445. doi:10.1021/acs.orglett.0c01812
Bandini, M., and Eichholzer, A. (2009). Catalytic Functionalization of Indoles in a New Dimension. Angew. Chem. Int. Edition 48, 9608–9644. doi:10.1002/anie.200901843
Bartoli, G., Bencivenni, G., and Dalpozzo, R. (2010). Organocatalytic Strategies for the Asymmetric Functionalization of Indoles. Chem. Soc. Rev. 39, 4449–4465. doi:10.1039/b923063g
Bartoli, G., Bosco, M., Giuli, S., Giuliani, A., Lucarelli, L., Marcantoni, E., et al. (2005). Efficient Preparation of 2-Indolyl-1-Nitroalkane Derivatives Employing Nitroalkenes as Versatile Michael Acceptors: New Practical Linear Approach to Alkyl 9H-β-Carboline-4-Carboxylate. J. Org. Chem. 70, 1941–1944. doi:10.1021/jo048776w
Deprez, N. R., Kalyani, D., Krause, A., and Sanford, M. S. (2006). Room Temperature Palladium-Catalyzed 2-Arylation of Indoles. J. Am. Chem. Soc. 128, 4972–4973. doi:10.1021/ja060809x
Ding, Z., and Yoshikai, N. (2012). Mild and Efficient C2-Alkenylation of Indoles with Alkynes Catalyzed by a Cobalt Complex. Angew. Chem. Int. Ed. 51, 4698–4701. doi:10.1002/anie.201200019
Doyle, M. P., Duffy, R., Ratnikov, M., and Zhou, L. (2010). Catalytic Carbene Insertion into C−H Bonds. Chem. Rev. 110, 704–724. doi:10.1021/cr900239n
Evans, D. A., Fandrick, K. R., and Song, H.-J. (2005). Enantioselective Friedel−Crafts Alkylations of α,β-Unsaturated 2-Acyl Imidazoles Catalyzed by Bis(oxazolinyl)pyridine−Scandium(III) Triflate Complexes. J. Am. Chem. Soc. 127, 8942–8943. doi:10.1021/ja052433d
Gensch, T., Klauck, F. J. R., and Glorius, F. (2016). Cobalt-Catalyzed C−H Thiolation through Dehydrogenative Cross-Coupling. Angew. Chem. Int. Ed. 55, 11287–11291. doi:10.1002/anie.201605193
James, M. J., Strieth‐Kalthoff, F., Sandfort, F., Klauck, F. J. R., Wagener, F., and Glorius, F. (2019). Visible‐Light‐Mediated Charge Transfer Enables C−C Bond Formation with Traceless Acceptor Groups. Chem. Eur. J. 25, 8240–8244. doi:10.1002/chem.201901397
Jiao, L., and Bach, T. (2011). Palladium-Catalyzed Direct 2-Alkylation of Indoles by Norbornene-Mediated Regioselective Cascade C-H Activation. J. Am. Chem. Soc. 133, 12990–12993. doi:10.1021/ja2055066
Johansen, M. B., and Kerr, M. A. (2010). Direct Functionalization of Indoles: Copper-Catalyzed Malonyl Carbenoid Insertions. Org. Lett. 12, 4956–4959. doi:10.1021/ol1020948
Joucla, L., and Djakovitch, L. (2009). Transition Metal-Catalysed, Direct and Site-Selective N1-, C2- or C3-Arylation of the Indole Nucleus: 20 Years of Improvements. Adv. Synth. Catal. 351, 673–714. doi:10.1002/adsc.200900059
Kandukuri, S. R., Bahamonde, A., Chatterjee, I., Jurberg, I. D., Escudero-Adán, E. C., and Melchiorre, P. (2015). X-Ray Characterization of an Electron Donor-Acceptor Complex that Drives the Photochemical Alkylation of Indoles. Angew. Chem. Int. Ed. 54, 1485–1489. doi:10.1002/anie.201409529
Kimura, M., Futamata, M., Mukai, R., and Tamaru, Y. (2005). Pd-Catalyzed C3-Selective Allylation of Indoles with Allyl Alcohols Promoted by Triethylborane. J. Am. Chem. Soc. 127, 4592–4593. doi:10.1021/ja0501161
Kong, L., Yu, S., Tang, G., Wang, H., Zhou, X., and Li, X. (2016). Cobalt(III)-Catalyzed C-C Coupling of Arenes with 7-Oxabenzonorbornadiene and 2-Vinyloxirane via C-H Activation. Org. Lett. 18, 3802–3805. doi:10.1021/acs.orglett.6b01806
Kusurkar, R. S., Alkobati, N. A. H., Gokule, A. S., and Puranik, V. G. (2008). Use of the Pictet-Spengler Reaction for the Synthesis of 1,4-Disubstituted-1,2,3,4-Tetrahydro-β-Carbolines and 1,4-Disubstituted-β-Carbolines: Formation of γ-carbolines. Tetrahedron 64, 1654–1662. doi:10.1016/j.tet.2007.12.008
Lane, B. S., and Sames, D. (2004). Direct C−H Bond Arylation: Selective Palladium-Catalyzed C2-Arylation of N-Substituted Indoles. Org. Lett. 6, 2897–2900. doi:10.1021/ol0490072
Lebrasseur, N., and Larrosa, I. (2008). Room Temperature and Phosphine Free Palladium Catalyzed Direct C-2 Arylation of Indoles. J. Am. Chem. Soc. 130, 2926–2927. doi:10.1021/ja710731a
Lerch, S., Unkel, L. N., and Brasholz, M. (2014). Tandem Organocatalysis and Photocatalysis: An Anthraquinone‐Catalyzed Indole‐C3‐Alkylation/Photooxidation/1,2‐Shift Sequence. Angew. Chem. Int. Ed. 53, 6558–6562. doi:10.1002/anie.201402920
Li, B., Ma, J., Xie, W., Song, H., Xu, S., and Wang, B. (2013b). Regioselective C2 Oxidative Olefination of Indoles and Pyrroles through Cationic Rhodium(III)-Catalyzed CH Bond Activation. Chem. Eur. J. 19, 11863–11868. doi:10.1002/chem.201301987
Li, B., Ma, J., Xie, W., Song, H., Xu, S., and Wang, B. (2013a). Ruthenium-Catalyzed Regioselective C2 Alkenylation of Indoles and Pyrroles via C-H Bond Functionalization. J. Org. Chem. 78, 9345–9353. doi:10.1021/jo401579m
Li, T., Wang, Z., Qin, W.-B., and Wen, T.-B. (2016). Rhodium-Catalyzed/Copper-Mediated Selective C2 Alkynylation of Indoles and C1 Alkynylation of Carbazoles Withγ-Substitutedtert-Propargyl Alcohols. ChemCatChem 8, 2146–2154. doi:10.1002/cctc.201600218
Liang, L., Fu, S., Lin, D., Zhang, X.-Q., Deng, Y., Jiang, H., et al. (2014). Ruthenium(II)-Catalyzed Direct Addition of Indole/Pyrrole C2-H Bonds to Alkynes. J. Org. Chem. 79, 9472–9480. doi:10.1021/jo501460h
Lin, Q., Chu, L., and Qing, F.-L. (2013). Direct Introduction of Ethoxycarbonyldifluoromethyl-Group to Heteroarenes with Ethyl Bromodifluoroacetate via Visible-Light Photocatalysis. Chin. J. Chem. 31, 885–891. doi:10.1002/cjoc.201300411
Ma, Y.-M., Liang, X.-A., Kong, Y., and Jia, B. (2016). Structural Diversity and Biological Activities of Indole Diketopiperazine Alkaloids from Fungi. J. Agric. Food Chem. 64, 6659–6671. doi:10.1021/acs.jafc.6b01772
Maehara, A., Tsurugi, H., Satoh, T., and Miura, M. (2008). Regioselective C−H Functionalization Directed by a Removable Carboxyl Group: Palladium-Catalyzed Vinylation at the Unusual Position of Indole and Related Heteroaromatic Rings. Org. Lett. 10, 1159–1162. doi:10.1021/ol8000602
Moran, J., Suen, T., and Beauchemin, A. M. (2006). Photoinduced 1,4-Additions of Indoles to Enones. J. Org. Chem. 71, 676–679. doi:10.1021/jo0521044
Nakao, Y., Kanyiva, K. S., Oda, S., and Hiyama, T. (2006). Hydroheteroarylation of Alkynes under Mild Nickel Catalysis. J. Am. Chem. Soc. 128, 8146–8147. doi:10.1021/ja0623459
Pan, S., Ryu, N., and Shibata, T. (2012). Ir(I)-Catalyzed C-H Bond Alkylation of C2-Position of Indole with Alkenes: Selective Synthesis of Linear or Branched 2-Alkylindoles. J. Am. Chem. Soc. 134, 17474–17477. doi:10.1021/ja308742x
Phipps, R. J., Grimster, N. P., and Gaunt, M. J. (2008). Cu(II)-Catalyzed Direct and Site-Selective Arylation of Indoles under Mild Conditions. J. Am. Chem. Soc. 130, 8172–8174. doi:10.1021/ja801767s
Sauermann, N., González, M. J., and Ackermann, L. (2015). Cobalt(III)-Catalyzed C-H Alkynylation with Bromoalkynes under Mild Conditions. Org. Lett. 17, 5316–5319. doi:10.1021/acs.orglett.5b02678
Schramm, Y., Takeuchi, M., Semba, K., Nakao, Y., and Hartwig, J. F. (2015). Anti-Markovnikov Hydroheteroarylation of Unactivated Alkenes with Indoles, Pyrroles, Benzofurans, and Furans Catalyzed by a Nickel-N-Heterocyclic Carbene System. J. Am. Chem. Soc. 137, 12215–12218. doi:10.1021/jacs.5b08039
Shao, C., Shi, G., Zhang, Y., Pan, S., and Guan, X. (2015). Palladium-Catalyzed C-H Ethoxycarbonyldifluoromethylation of Electron-Rich Heteroarenes. Org. Lett. 17, 2652–2655. doi:10.1021/acs.orglett.5b01024
Soni, V., Sharma, D. M., and Punji, B. (2018). Nickel-Catalyzed Regioselective C(2)−H Difluoroalkylation of Indoles with Difluoroalkyl Bromides. Chem. Asian J. 13, 2516–2521. doi:10.1002/asia.201800504
Straathof, N. J. W., Gemoets, H. P. L., Wang, X., Schouten, J. C., Hessel, V., and Noël, T. (2014). Rapid Trifluoromethylation and Perfluoroalkylation of Five-Membered Heterocycles by Photoredox Catalysis in Continuous Flow. ChemSusChem 7, 1612–1617. doi:10.1002/cssc.201301282
Su, Y.-M., Hou, Y., Yin, F., Xu, Y.-M., Li, Y., Zheng, X., et al. (2014). Visible Light-Mediated C-H Difluoromethylation of Electron-Rich Heteroarenes. Org. Lett. 16, 2958–2961. doi:10.1021/ol501094z
Sun, B., Yoshino, T., Matsunaga, S., and Kanai, M. (2015). A Cp*CoI2-Dimer as a Precursor for Cationic Co(iii)-Catalysis: Application to C-H Phosphoramidation of Indoles. Chem. Commun. 51, 4659–4661. doi:10.1039/C4CC10284C
Sun, B., Yoshino, T., Matsunaga, S., and Kanai, M. (2014). Air‐Stable Carbonyl(pentamethylcyclopentadienyl)cobalt Diiodide Complex as a Precursor for Cationic (Pentamethylcyclopentadienyl)Cobalt(III) Catalysis: Application for Directed C‐2 Selective CH Amidation of Indoles. Adv. Synth. Catal. 356, 1491–1495. doi:10.1002/adsc.201301110
Terada, M., and Sorimachi, K. (2007). Enantioselective Friedel−Crafts Reaction of Electron-Rich Alkenes Catalyzed by Chiral Brønsted Acid. J. Am. Chem. Soc. 129, 292–293. doi:10.1021/ja0678166
Tolnai, G. L., Ganss, S., Brand, J. P., and Waser, J. (2013). C2-Selective Direct Alkynylation of Indoles. Org. Lett. 15, 112–115. doi:10.1021/ol3031389
Wang, L., Huang, W., Li, R., Gehrig, D., Blom, P. W. M., Landfester, K., et al. (2016). Structural Design Principle of Small-Molecule Organic Semiconductors for Metal-free, Visible-Light-Promoted Photocatalysis. Angew. Chem. Int. Ed. 55, 9783–9787. doi:10.1002/anie.201603789
Wang, X., Lane, B. S., and Sames, D. (2005). Direct C-Arylation of Free (NH)-Indoles and Pyrroles Catalyzed by Ar−Rh(III) Complexes Assembled In Situ. J. Am. Chem. Soc. 127, 4996–4997. doi:10.1021/ja050279p
Wang, Y.-Q., Song, J., Hong, R., Li, H., and Deng, L. (2006). Asymmetric Friedel−Crafts Reaction of Indoles with Imines by an Organic Catalyst. J. Am. Chem. Soc. 128, 8156–8157. doi:10.1021/ja062700v
Wang, Z., and Wang, C. (2021). Manganese/NaOPh Co-catalyzed C2-Selective C-H Conjugate Addition of Indoles to α,β-unsaturated Carbonyls. Green. Synth. Catal. 2, 66–69. doi:10.1016/j.gresc.2021.01.010
Weng, J.-Q., Fan, R.-J., Deng, Q.-M., Liu, R.-R., Gao, J.-R., and Jia, Y.-X. (2016). Enantioselective Friedel-Crafts Alkylation Reactions of 3-Substituted Indoles with Electron-Deficient Alkenes. J. Org. Chem. 81, 3023–3030. doi:10.1021/acs.joc.6b00123
Wong, M. Y., Yamakawa, T., and Yoshikai, N. (2015). Iron-Catalyzed Directed C2-Alkylation and Alkenylation of Indole with Vinylarenes and Alkynes. Org. Lett. 17, 442–445. doi:10.1021/ol503395g
Yang, L., Zhao, L., and Li, C.-J. (2010). Palladium-Catalyzed Direct Oxidative Heck-Cassar-Sonogashira Type Alkynylation of Indoles with Alkynes under Oxygen. Chem. Commun. 46, 4184–4186. doi:10.1039/c0cc00014k
Yang, Q.-Q., Marchini, M., Xiao, W.-J., Ceroni, P., and Bandini, M. (2015). Visible-Light-Induced Direct Photocatalytic Carboxylation of Indoles with CBr4/MeOH. Chem. Eur. J. 21, 18052–18056. doi:10.1002/chem.201503787
Yang, S.-D., Sun, C.-L., Fang, Z., Li, B.-J., Li, Y.-Z., and Shi, Z.-J. (2008). Palladium-Catalyzed Direct Arylation of (Hetero)Arenes with Aryl Boronic Acids. Angew. Chem. Int. Ed. 47, 1473–1476. doi:10.1002/anie.200704619
Yang, Y., and Shi, Z. (2018). Regioselective Direct Arylation of Indoles on the Benzenoid Moiety. Chem. Commun. 54, 1676–1685. doi:10.1039/c7cc08752g
Yoshino, T., Ikemoto, H., Matsunaga, S., and Kanai, M. (2013). Cp*CoIII -Catalyzed C2-Selective Addition of Indoles to Imines. Chem. Eur. J. 19, 9142–9146. doi:10.1002/chem.201301505
Zhang, W., Wei, J., Fu, S., Lin, D., Jiang, H., and Zeng, W. (2015). Highly Stereoselective Ruthenium(II)-Catalyzed Direct C2-Syn-Alkenylation of Indoles with Alkynes. Org. Lett. 17, 1349–1352. doi:10.1021/ol503618m
Zhang, Z.-Z., Liu, B., Wang, C.-Y., and Shi, B.-F. (2015). Cobalt(III)-Catalyzed C2-Selective C-H Alkynylation of Indoles. Org. Lett. 17, 4094–4097. doi:10.1021/acs.orglett.5b02038
Zhou, J., and Tang, Y. (2002). Sidearm Effect: Improvement of the Enantiomeric Excess in the Asymmetric Michael Addition of Indoles to Alkylidene Malonates. J. Am. Chem. Soc. 124, 9030–9031. doi:10.1021/ja026936k
Zhou, X., Fan, Z., Zhang, Z., Lu, P., and Wang, Y. (2016). Construction of Pyrrolo[1,2-a]Indoles via Cobalt(III)-Catalyzed Enaminylation of 1-(Pyrimidin-2-Yl)-1h-Indoles with Ketenimines and Subsequent Base-Promoted Cyclization. Org. Lett. 18, 4706–4709. doi:10.1021/acs.orglett.6b02353
Keywords: metal-free, acid catalysis, atom economy, indole-2-alkylation, alkene
Citation: Pan X, Liu Q and Nong Y (2022) 2-Alkylation of 3-Alkyindoles With Unactivated Alkenes. Front. Chem. 10:860764. doi: 10.3389/fchem.2022.860764
Received: 23 January 2022; Accepted: 28 January 2022;
Published: 24 February 2022.
Edited by:
Yaqiong Su, Xi’an Jiaotong University, ChinaCopyright © 2022 Pan, Liu and Nong. This is an open-access article distributed under the terms of the Creative Commons Attribution License (CC BY). The use, distribution or reproduction in other forums is permitted, provided the original author(s) and the copyright owner(s) are credited and that the original publication in this journal is cited, in accordance with accepted academic practice. No use, distribution or reproduction is permitted which does not comply with these terms.
*Correspondence: Xuling Pan, UGFuWEwxOTk2QDE2My5jb20=