- 1Collaborative Innovation Centre of Henan Province for Green Manufacturing of Fine Chemicals, Key Laboratory of Green Chemical Media and Reactions, Ministry of Education, Henan Engineering Research Centre of Chiral Hydroxyl Pharmaceutical, Henan Engineering Laboratory of Chemical Pharmaceutical and Biomedical Materials, School of Chemistry and Chemical Engineering, Henan Normal University, Xinxiang, China
- 2National Engineering Research Center of Low-Carbon Processing and Utilization of Forest Biomass, Nanjing Forestry University, Nanjing, China
- 3Green Catalysis Center, College of Chemistry, Zhengzhou University, Zhengzhou, China
We reported a general transition metal-free transformation to access C3-carbamoylated 2H-indazoles via visible light-induced oxidative decarboxylation coupling, in the presence of oxamic acids as the coupling sources, 4CzIPN as the photocatalyst, and Cs2CO3 as the base. The great application potential of this mild condition is highlighted by the late-stage modification of drugs, N-terminal modification of peptides, and the good antitumor activity of the novel desired product.
Introduction
Nitrogen heterocycles are the essential structural elements widely ubiquitous in pharmaceutical chemistry, (Vitaku et al., 2014; Bhutani et al., 2021; Ma et al., 2021a), organic chemistry, (Chen et al., 2021; Darroudi et al., 2021; Jiang et al., 2021; Meng et al., 2021; Qu et al., 2021; Wang and Wang, 2021; Chen and Xuan, 2022; Gao et al., 2022; Wu et al., 2022; Zhang et al., 2022), and material chemistry (Huang and Yu, 2021). Among these, 2H-indazole is one of the most important heterocycles, existing in various drugs and bioactive molecules (Figure 1). The drug Niraparib with this scaffold is approved to treat various tumors including advanced epithelial ovarian carcinoma and primary peritoneal carcinoma. (Jones et al., 2009). The derivative Pazopanib has become the first-line anti-advanced renal cell carcinoma via inhibiting the activity of vascular endothelial growth factor receptor VEGFR. (Harris et al., 2008). The 3C-like protease inhibitor S-217622 has entered into clinical trials and exhibits antiviral activity against the coronavirus disease 2019 (COVID-19). (Unoh et al., 2022). Therefore, direct and site-selective incorporation of diverse functional groups into 2H-indazole is of broad interest in organic synthesis and the pharmaceutical industry.
Recent decades have witnessed the impressive achievement of direct C-H functionalization of 2H-indazoles via radical reactions. (Ghosh et al., 2020; Wang et al., 2022a; Ghosh et al., 2022). The C3-phosphonylation, (Singsardar et al., 2018), oxyalkylation, (Singsardar et al., 2019), trifluoromethylation, (Murugan et al., 2019; Wei et al., 2021), amination, (Neogi et al., 2020; Sun et al., 2021a), alkoxylation, (Sun et al., 2021b), arylation, (Aganda et al., 2019; Vidyacharan et al., 2019; Saritha et al., 2021), alkylation, (Liu et al., 2020; Ma et al., 2021b; Ma et al., 2022a), sulfonylation, (Kim et al., 2020; Mahanty et al., 2020), and selenylation (Lin et al., 2022) of 2H-indazole were reported. However, the development of sustainable strategies to introduce other pharmacophores into 2H-indazole is still highly desirable. Amide groups represent a fundamental class of functional groups widely spread in most drugs, bioactive compounds, and peptides. Compared with the traditional condensation method, the C-H carbamoylation protocol provides the desired product without prefunctionalization of the 2H-indazole and wasteful coupling reagents. Nevertheless, the direct carbamoylation of 2H-indazole is rarely reported. Only recently, Lee’s group reports an elegant carbamoylation reaction of 2H-indazole using oxamic acid as a carbamoylating source under an elevated temperature in the presence of the strong oxidant (NH4)S2O8. (Bhat and Lee, 2021). However, the heating process which is essential for the radical generation results in the consumption of fossil fuels and the potential safety hazard. Meanwhile, a great quantity of strong oxidant might be detrimental to the sensitive functional groups. Photocatalysis has emerged as a strong strategy to the functionalization of the nitrogen heterocycles. (Liu et al., 2017; Bagdi et al., 2020; Yuan et al., 2020; He et al., 2021; Qi et al., 2021; Yi and He, 2021; Ma et al., 2022b; Wang et al., 2022b; Ma et al., 2022c; Ma et al., 2022d; Ma et al., 2022e; Shi et al., 2022; Xiang et al., 2022; Yan et al., 2022; Yang et al., 2022; Zhu et al., 2022). The mild reaction condition and the visible light-induced neutral redox cycle may solve the above problems. Herein, we reported a visible light-mediated strong oxidant-free protocol to access the carbamoylated 2H-indazoles under mild conditions and the late-stage modification of drugs and peptides (Scheme 1).
Results and discussion
We chose 2-phenyl-2H-indazole (1a) and 2-(hexylamino)-2-oxoacetic acid (2a) as model substrates to investigate the decarboxylative C (sp2)-C (sp2) coupling reaction under 405 nm purple LED irradiation at room temperature. Consistent with the expected, when 4CzIPN was used as the photocatalyst and Cs2CO3 as the base, 1a and 2a could be converted into the carbamoylated 2H-indazole 3a in 56% yield under O2 atmosphere (Table 1, entry 1). Other transition metal-free photocatalysts including Rhodamine B, Rhodamine 6G, Fluorescein, Na2-Eosin Y, and Rose bengal were catalytically inactive, with no product detected (Table 1, entries 2–6). Then, a systematic survey of bases were conducted. The results indicated that replacing Cs2CO3 with other inorganic bases (Na2CO3, K2CO3, LiOH, KOH, CsOH) or organic bases (Et3N, DIPEA, TMEDA, DABCO) decreases the formation of the desired product (Table 1, entries 7–15). A range of solvents, such as, DCM, MeCN, DMF, DMAC, NMP, THF, DMC, EG, and H2O were screened (Table 1, entries 16–24). We found that DMSO is superior in this process. Increasing the amount of 2a to 2.5 equiv. improved the yield to 74% (Table 1, entry 25). Because the insoluble residue existed in the reaction system, the volume of DMSO was increased to 3 ml, along with the generation of products in 91% yield (Table 1, entry 26). In the absence of visible light or photocatalyst, no product was detected, which confirms the photochemical nature of this method (Table 1, entries 27–28). The reaction was completely inhibited in the absence of Cs2CO3, indicating the essential role of the base in the transformation (Table 1, entry 29). Taken together, the optimal reaction conditions were established as follows: 1a (0.2 mmol), 2a (2.5 equiv), 4CzIPN (5 mol%) as a catalyst, Cs2CO3 (2 equiv) as a base, DMSO (3 ml) as a solvent, at 35°C under O2 atmosphere and the irradiation of purple LED (λmax = 405 nm) for 12 h.
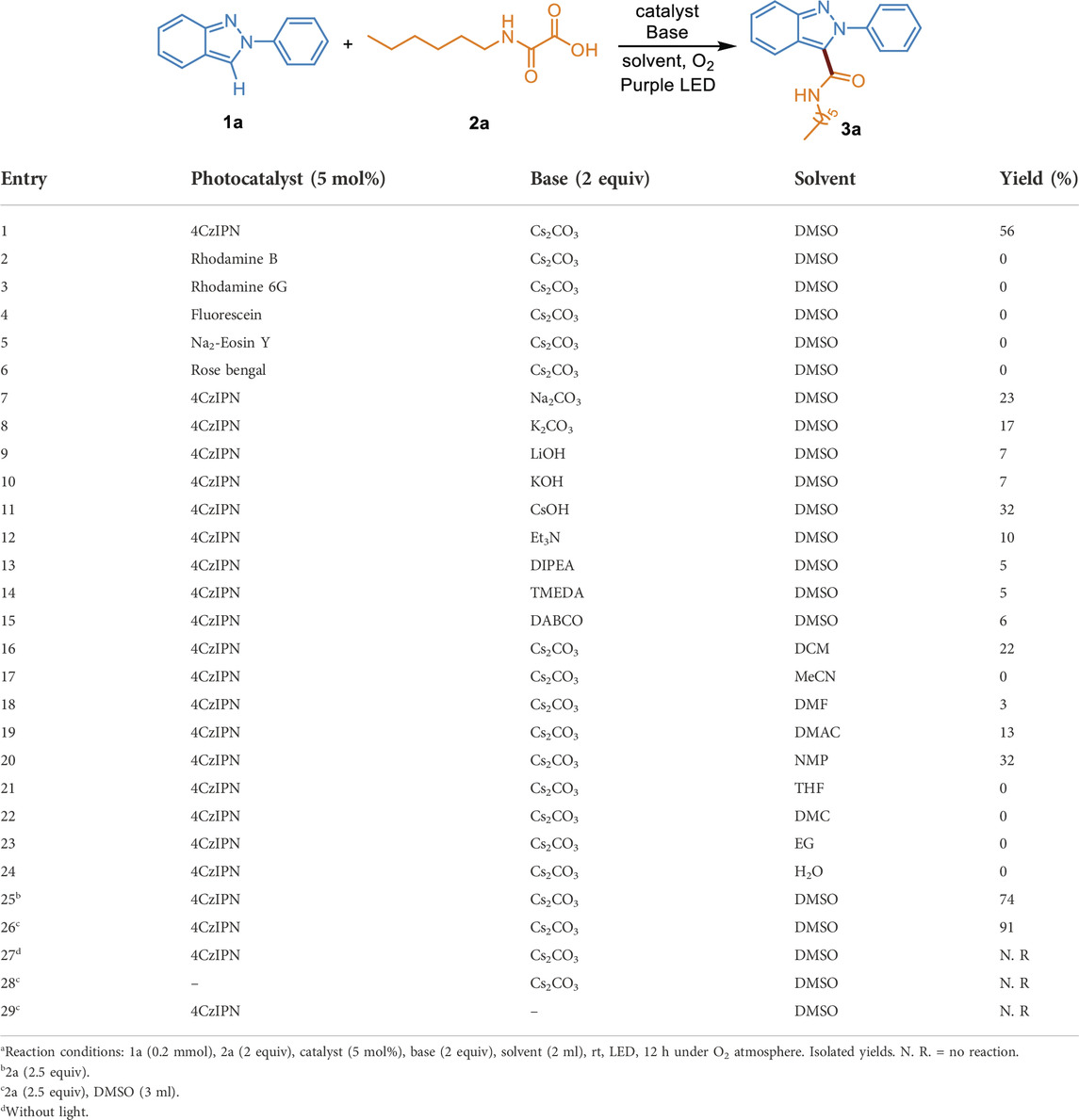
TABLE 1. Optimization of reaction conditionsa.
With the optimal conditions for the construction of carbamoylated 2H-indazoles in hand, we further explored the scope and generality of this reaction. Firstly, the scope of aryl-2H-indazoles was examined. As shown in Scheme 2, the substitutions on the phenyl group exhibited good tolerance. The electron-donating groups (p-Me and m-Me) could give the desired products 3b-3c in 72% and 64% yields, respectively. The derivatives with electron-withdrawing groups (p-Cl, m-Cl, p-Br, m-Br, and p-CF3) were also effective substrates for this transformation, affording the corresponding products 3d-3h in moderate to good yields. Moreover, both the electron-donating substitution (5-OMe) and the electron-withdrawing groups (5-F, 5-Cl, and 5-Br) on the heteronucleus were well tolerant to the standard conditions (3i-3l). The 2H-indazoles with disubstitution were also evaluated to react with 2a under the optimal condition, delivering the corresponding products 3m-3o in 49–59% yields.
Subsequently, we investigated the reactivity profile of a variety of oxamic acids 2. As depicted in Scheme 3, oxamic acids with different length alkyl chains reacted well with 1a, affording the desired products 3p-3s in 58%–94% yields. The benzyl group was also compatible with the method, giving the product 3t in 42% yield. Both the secondary carbon (cyclopentyl and cyclohexyl group) and tertiary carbon (2-phenylpropyl group) substituted oxamic acids were successful in providing the corresponding products 3u-3w in 80%–93% yields. Meanwhile, the substrates containing primary aromatic amines reacted well with 1a and produced the desired products 3x-3aa in 54%–93% yields. The oxamic acids bearing secondary amine also showed good reactiveness and could be smoothly converted into the carbamoylated products 3ab and 3ac. Moreover, the oxamic acid without N-substitution was tolerated to generate the desired product 3ad in 55% yield.
To evaluate the synthetic utility of this decarboxylative carbamoylation transformation in the pharmaceutical industry, the late-stage modification of drugs and natural products was conducted. Delightfully, the non-sulfonylureas antibiabetic drug Nateglinide, the lipid regulator Gemfibrozil, and the antiviral drug amantadine could be successfully connected with 2H-indazole, affording the desired products 4a-4c in 35%–76% yield (Scheme 4). The natural product dehydroabietylamine was also suitable and gave the products 4d in 40% yield. The N-terminal modificated of peptides play an important role in drug development and biochemical research. Inspired by the good functional group tolerance of this sustainable system, we then applied the photocatalytic method in the modification of natural amino acids and peptides. As shown in Scheme 4, the important amino acid in humans, l-leucine, could be converted into the corresponding products 4e in 63% yield. What’s more, both the dipeptide (l-phenylalanine-l-leucine) and the tripeptides (l-glycine-l-proline-l-phenylalanine and l-glycine-l-phenylalanine-l-leucine) reacted well with 2H-indazole, delivering the coupling products 4f-4h in 40%–73% yields. The above results indicate that this method could be used in the development of peptidomimetic drugs and probe molecules.
To investigate the mechanism of this carbamoylation reaction, a radical scavenge experiment was conducted (Scheme 5). When 2,2,6,6-tetramethylpiperidinyl-1-oxyl (TEMPO) was added to the standard conditions, the reaction completely shuttled down. Moreover, the carbamoyl radical trapped adduct 5 was detected by HRMS. It indicates that this photocatalytic transformation occurred via a radical pathway. Next, it was found that the yields of 3a were decreased to 9% and 23% under N2 atmosphere or air atmosphere, revealing that O2 is important in the photocatalytic system.
We performed the Stern–Volmer luminescence-quenching experiments by mixing the photocatalyst 4CzIPN with different concentrations of 2H-indazole 1a, 2-(hexylamino)-2-oxoacetic acid 2a, or the Cs salt of 2a (6). As depicted in Scheme 6A, the fluorescence of photoredox catalyst 4CzIPN was quenched by the addition of 1a and 6, and the linear relationships were observed between I0/I and the concentration of 1a and 6 (see the Supplementary Figure S2). The oxidative potential of 6 was E1/2ox = +0.9 V vs. SCE (Scheme 6B), indicating that the excited state 4CzIPN (E1/2(P*/P−) = +1.35 V vs. SCE) (Shang et al., 2019) could be reductively quenched by 6 rather than 1a (E1/2ox = +1.4 V vs. SCE) (Ma et al., 2021b).
A plausible mechanism for this sustainable reaction was proposed according to the above experimental results and the previous reports (Scheme 7). Initially, 4CzIPN was activated into the excited state 4CzIPN* under visible light irradiation. The oxamic acid 2 was in situ converted into the Cs salt 6 in the presence of the base Cs2CO3. 6 underwent the oxidization of 4CzIPN* via single electron transfer (SET) and fragmentation to generate the key carbamoyl radical 7, along with the production of the radical anion 4CzIPN•-. 4CzIPN•- was oxidated by O2 to regenerate the ground state 4CzIPN and close the photoredox cycle. On the other hand, radical 7 attacked the C3-position of 1a to deliver intermediate 8. It underwent the 4CzIPN* mediated oxidation and base mediated dehydrogenate to afford the desired product 3.
To highlight this greener protocol in the pharmaceutical industry, we evaluated the in vitro antitumor activity of these carbamoylated 2H-indazole derivatives. As depicted in Scheme 8, compound 4d possessed better antitumor activity against Ramos cell than that of the FDA-approved drug 5-fluorouracil (5-FU, IC50 = 36.0 × 10−6 mol/L), suggesting that this method could provide novel chemical entries for anti-human B cell lymphoma treatment.
Conclusion
In summary, we have developed a visible-light-promoted, transition metal-free, strong oxidant-free method to achieve the direct decarboxylation/carbamylation of 2-aryl-2H-indazoles. This mild and general protocol is tolerant of sensitive functional groups and sterically hindered groups. It is highlighted by the successful application in the late-stage modification of drugs, natural products, amino acids, and peptides. Moreover, the good antitumor activity of compound 4d indicates that this strategy could be used in antitumor drug development. Further activity studies and structural modification are ongoing in our laboratory.
Data availability statement
The original contributions presented in the study are included in the article/Supplementary Material, further inquiries can be directed to the corresponding authors.
Author contributions
All authors listed have made a substantial, direct, and intellectual contribution to the work, and approved it for publication.
Funding
We acknowledge the financial support from the National Natural Science Foundation of China (82003585), the Technical innovation Team of Henan Normal University (2022TD03), the Postgraduate Education Reform and Quality Improvement Project of Henan Province (YJS2021AL079), the Scientific Research and Practice Innovation Program of Henan Normal University (YL202103), and the National College Students’ innovation and entrepreneurship training program of China (202210476076).
Acknowledgments
We would like to thank the Large Instrument Sharing System for the support of structure confirmation.
Conflict of interest
The authors declare that the research was conducted in the absence of any commercial or financial relationships that could be construed as a potential conflict of interest.
The handling editor declared a past co-authorship with the author QL.
Publisher’s note
All claims expressed in this article are solely those of the authors and do not necessarily represent those of their affiliated organizations, or those of the publisher, the editors and the reviewers. Any product that may be evaluated in this article, or claim that may be made by its manufacturer, is not guaranteed or endorsed by the publisher.
Supplementary material
The Supplementary Material for this article can be found online at: https://www.frontiersin.org/articles/10.3389/fchem.2022.1087834/full#supplementary-material
References
Aganda, K. C. C., Kim, J., and Lee, A. (2019). Visible-light-mediated direct C3-arylation of 2H-indazoles enabled by an electron-donor–acceptor complex. Org. Biomol. Chem. 17, 9698–9702. doi:10.1039/c9ob02074h
Bagdi, A. K., Rahman, M., Bhattacherjee, D., Zyryanov, G. V., Ghosh, S., Chupakhin, O. N., et al. (2020). Visible light promoted cross-dehydrogenative coupling: A decade update. Green Chem. 22, 6632–6681. doi:10.1039/d0gc02437f
Bhat, V. S., and Lee, A. (2021). Direct C3 carbamoylation of 2H-indazoles. Eur. J. Org. Chem. 2021, 3382–3385. doi:10.1002/ejoc.202100461
Bhutani, P., Joshi, G., Raja, N., Bachhav, N., Rajanna, P. K., Bhutani, H., et al. (2021). U.S. FDA approved drugs from 2015–june 2020: A perspective. J. Med. Chem. 64, 2339–2381. doi:10.1021/acs.jmedchem.0c01786
Chen, J.-Y., Wu, H.-Y., Gui, Q.-W., Yan, S.-S., Deng, J., Lin, Y.-W., et al. (2021). Sustainable electrochemical cross-dehydrogenative coupling of 4-quinolones and diorganyl diselenides. Chin. J. Catal. 42, 1445–1450. doi:10.1016/s1872-2067(20)63750-0
Chen, Z., and Xuan, J. (2022). Photochemical synthesis of aroylated heterocycles under catalyst and additive free conditions. Chin. J. Org. Chem. 42, 923–924. doi:10.6023/cjoc202200018
Darroudi, M., Mohammadi Ziarani, G., Bahar, S., Ghasemi, J. B., and Badiei, A. (2021). Lansoprazole-based colorimetric chemosensor for efficient binding and sensing of carbonate ion: Spectroscopy and DFT studies. Front. Chem. 8, 626472. doi:10.3389/fchem.2020.626472
Gao, F., Zhang, S., Lv, Q., and Yu, B. (2022). Recent advances in graphene oxide catalyzed organic transformations. Chin. Chem. Lett. 33, 2354–2362. doi:10.1016/j.cclet.2021.10.081
Ghosh, D., Ghosh, S., Ghosh, A., Pyne, P., Majumder, S., and Hajra, A. (2022). Visible light-induced functionalization of indazole and pyrazole: A recent update. Chem. Commun. 58, 4435–4455. doi:10.1039/d2cc00002d
Ghosh, S., Mondal, S., and Hajra, A. (2020). Direct catalytic functionalization of indazole derivatives. Adv. Synth. Catal. 362, 3768–3794. doi:10.1002/adsc.202000423
Harris, P. A., Boloor, A., Cheung, M., Kumar, R., Crosby, R. M., Davis-Ward, R. G., et al. (2008). Discovery of 5-[[4-[(2, 3-dimethyl-2H-indazol-6-yl)methylamino]-2-pyrimidinyl]amino]-2-methyl-benzenesulfonamide (Pazopanib), a novel and potent vascular endothelial growth factor receptor inhibitor. J. Med. Chem. 51, 4632–4640. doi:10.1021/jm800566m
He, S., Li, H., Chen, X., Krylov, I. B., Terent'ev, A. O., Qu, L., et al. (2021). Advances of N-hydroxyphthalimide esters in photocatalytic alkylation reactions. Chin. J. Org. Chem. 41, 4661–4689. doi:10.6023/cjoc202105041
Huang, J., and Yu, G. (2021). Structural engineering in polymer semiconductors with aromatic N-heterocycles. Chem. Mat. 33, 1513–1539. doi:10.1021/acs.chemmater.0c03975
Jiang, J., Xiao, F., He, W.-M., and Wang, L. (2021). The application of clean production in organic synthesis. Chin. Chem. Lett. 32, 1637–1644. doi:10.1016/j.cclet.2021.02.057
Jones, P., Altamura, S., Boueres, J., Ferrigno, F., Fonsi, M., Giomini, C., et al. (2009). Discovery of 2-{4-[(3S)-Piperidin-3-yl]phenyl}-2H-indazole-7-carboxamide (MK-4827): A novel oral poly(ADP-ribose)polymerase (parp) inhibitor efficacious in BRCA-1 and -2 mutant tumors. J. Med. Chem. 52, 7170–7185. doi:10.1021/jm901188v
Kim, W., Kim, H. Y., and Oh, K. (2020). Electrochemical radical–radical cross-coupling approach between sodium sulfinates and 2H-indazoles to 3-sulfonylated 2H-indazoles. Org. Lett. 22, 6319–6323. doi:10.1021/acs.orglett.0c02144
Lin, S., Cheng, X., Hasimujiang, B., Xu, Z., Li, F., and Ruan, Z. (2022). Electrochemical regioselective C–H selenylation of 2H-indazole derivatives. Org. Biomol. Chem. 20, 117–121. doi:10.1039/d1ob02108g
Liu, B., Lim, C.-H., and Miyake, G. M. (2017). Visible-light-promoted C–S cross-coupling via intermolecular charge transfer. J. Am. Chem. Soc. 139, 13616–13619. doi:10.1021/jacs.7b07390
Liu, L., Jiang, P., Liu, Y., Du, H., and Tan, J. (2020). Direct radical alkylation and acylation of 2H-indazoles using substituted Hantzsch esters as radical reservoirs. Org. Chem. Front. 7, 2278–2283. doi:10.1039/d0qo00507j
Ma, C.-H., Ji, Y., Zhao, J., He, X., Zhang, S.-T., Jiang, Y.-Q., et al. (2022). Transition-metal-free three-component acetalation-pyridylation of alkenes via photoredox catalysis. Chin. J. Catal. 43, 571–583. doi:10.1016/s1872-2067(21)63917-7
Ma, C.-H., Zhao, L., He, X., Jiang, Y.-Q., and Yu, B. (2022). Visible-light-induced direct 3-ethoxycarbonylmethylation of 2-aryl-2H-indazoles in water. Org. Chem. Front. 9, 1445–1450. doi:10.1039/d1qo01870a
Ma, C., Feng, Z., Li, J., Zhang, D., Li, W., Jiang, Y., et al. (2021). Photocatalytic transition-metal-free direct 3-alkylation of 2-aryl-2H-indazoles in dimethyl carbonate. Org. Chem. Front. 8, 3286–3291. doi:10.1039/d1qo00064k
Ma, C., Li, Q., Zhao, M., Fan, G., Zhao, J., Zhang, D., et al. (2021). Discovery of 1-amino-1H-imidazole-5-carboxamide derivatives as highly selective, covalent bruton’s tyrosine kinase (BTK) inhibitors. J. Med. Chem. 64, 16242–16270. doi:10.1021/acs.jmedchem.1c01559
Ma, C., Meng, H., He, X., Jiang, Y., and Yu, B. (2022). Visible-light-promoted transition-metal-free construction of 3-perfluoroalkylated thioflavones. Front. Chem. 10, 953978. doi:10.3389/fchem.2022.953978
Ma, C., Meng, H., Li, J., Yang, X., Jiang, Y., and Yu, B. (2022). Photocatalytic transition-metal-free direct 3-acetalation of quinoxaline-2( 1 H )-ones. Chin. J. Chem. 40, 2655–2662. doi:10.1002/cjoc.202200386
Ma, C., Tian, Y., Wang, J., He, X., Jiang, Y., and Yu, B. (2022). Visible-light-Driven transition-metal-free site-selective access to isonicotinamides. Org. Lett. 24, 8265–8270. doi:10.1021/acs.orglett.2c02949
Mahanty, K., Maiti, D., and De Sarkar, S. (2020). Regioselective C–H sulfonylation of 2H-indazoles by electrosynthesis. J. Org. Chem. 85, 3699–3708. doi:10.1021/acs.joc.9b03330
Meng, N., Lv, Y., Liu, Q., Liu, R., Zhao, X., and Wei, W. (2021). Visible-light-induced three-component reaction of quinoxalin-2(1H)-ones, alkenes and CF3SO2Na leading to 3-trifluoroalkylated quinoxalin-2(1H)-ones. Chin. Chem. Lett. 32, 258–262. doi:10.1016/j.cclet.2020.11.034
Murugan, A., Babu, V. N., Polu, A., Sabarinathan, N., Bakthadoss, M., and Sharada, D. S. (2019). Regioselective C3–H trifluoromethylation of 2H-indazole under transition-metal-free photoredox catalysis. J. Org. Chem. 84, 7796–7803. doi:10.1021/acs.joc.9b00676
Neogi, S., Ghosh, A. K., Majhi, K., Samanta, S., Kibriya, G., and Hajra, A. (2020). Organophotoredox-Catalyzed direct C–H amination of 2H-indazoles with amines. Org. Lett. 22, 5605–5609. doi:10.1021/acs.orglett.0c01973
Qi, Y., Gu, X., Huang, X., Shen, G., Yang, B., He, Q., et al. (2021). Microwave-assisted controllable synthesis of 2-acylbenzothiazoles and bibenzo[b] [1, 4]thiazines from aryl methyl ketones and disulfanediyldianilines. Chin. Chem. Lett. 32, 3544–3547. doi:10.1016/j.cclet.2021.05.069
Qu, Z., Wang, P., Chen, X., Deng, G.-J., and Huang, H. (2021). Visible-light-driven cadogan reaction. Chin. Chem. Lett. 32, 2582–2586. doi:10.1016/j.cclet.2021.02.047
Saritha, R., Annes, S. B., and Ramesh, S. (2021). Metal-free, regioselective, visible light activation of 4CzIPN for the arylation of 2H-indazole derivatives. RSC Adv. 11, 14079–14084. doi:10.1039/d1ra02372a
Shang, T.-Y., Lu, L.-H., Cao, Z., Liu, Y., He, W.-M., and Yu, B. (2019). Recent advances of 1, 2, 3, 5-tetrakis(carbazol-9-yl)-4, 6-dicyanobenzene (4CzIPN) in photocatalytic transformations. Chem. Commun. 55, 5408–5419. doi:10.1039/c9cc01047e
Shi, T., Liu, Y.-T., Wang, S.-S., Lv, Q.-Y., and Yu, B. (2022). Recyclable carbon nitride nanosheet-photocatalyzed aminomethylation of imidazo[1, 2-a]pyridines in green solvent. Chin. J. Chem. 40, 97–103. doi:10.1002/cjoc.202100444
Singsardar, M., Dey, A., Sarkar, R., and Hajra, A. (2018). Visible-light-induced organophotoredox-catalyzed phosphonylation of 2H-indazoles with diphenylphosphine oxide. J. Org. Chem. 83, 12694–12701. doi:10.1021/acs.joc.8b02019
Singsardar, M., Laru, S., Mondal, S., and Hajra, A. (2019). Visible-light-induced regioselective cross-dehydrogenative coupling of 2H-indazoles with ethers. J. Org. Chem. 84, 4543–4550. doi:10.1021/acs.joc.9b00318
Sun, M., Li, L., Wang, L., Huo, J., Sun, M., and Li, P. (2021). Controllable chemoselectivity in the reaction of 2H-indazoles with alcohols under visible-light irradiation: Synthesis of C3-alkoxylated 2H-indazoles and ortho-alkoxycarbonylated azobenzenes. Org. Chem. Front. 8, 4230–4236. doi:10.1039/d1qo00592h
Sun, M., Zhou, Y., Li, L., Wang, L., Ma, Y., and Li, P. (2021). Electrochemically promoted C-3 amination of 2H-indazoles. Org. Chem. Front. 8, 754–759. doi:10.1039/d0qo01088j
Unoh, Y., Uehara, S., Nakahara, K., Nobori, H., Yamatsu, Y., Yamamoto, S., et al. (2022). Discovery of S-217622, a noncovalent oral SARS-CoV-2 3CL protease inhibitor clinical candidate for treating COVID-19. J. Med. Chem. 65, 6499–6512. doi:10.1021/acs.jmedchem.2c00117
Vidyacharan, S., Ramanjaneyulu, B. T., Jang, S., and Kim, D.-P. (2019). Continuous-flow visible light organophotocatalysis for direct arylation of 2H-indazoles: Fast access to drug molecules. ChemSusChem 12, 2581–2586. doi:10.1002/cssc.201900736
Vitaku, E., Smith, D. T., and Njardarson, J. T. (2014). Analysis of the structural diversity, substitution patterns, and frequency of nitrogen heterocycles among U.S. FDA approved pharmaceuticals. J. Med. Chem. 57, 10257–10274. doi:10.1021/jm501100b
Wang, D., Wang, J., Ma, C., Jiang, Y., and Yu, B. (2022). C-3 functionalization of 2-aryl-2H-indazoles under photo/electrocatalysis. Chin. J. Org. Chem. 42. doi:10.6023/cjoc202208039
Wang, F., and Wang, S.-Y. (2021). Visible-light-promoted cross-coupling reaction of hypervalent bis-catecholato silicon compounds with selenosulfonates or thiosulfonates. Org. Chem. Front. 8, 1976–1982. doi:10.1039/d1qo00085c
Wang, Z., Liu, Q., Liu, R., Ji, Z., Li, Y., Zhao, X., et al. (2022). Visible-light-initiated 4CzIPN catalyzed multi-component tandem reactions to assemble sulfonated quinoxalin-2(1H)-ones. Chin. Chem. Lett. 33, 1479–1482. doi:10.1016/j.cclet.2021.08.036
Wei, T., Wang, K., Yu, Z., Hou, J., and Xie, Y. (2021). Electrochemically mediated trifluoromethylation of 2H-indazole derivatives using CF3SO2Na. Tetrahedron Lett. 86, 153313. doi:10.1016/j.tetlet.2021.153313
Wu, Z.-L., Chen, J.-Y., Tian, X.-Z., Ouyang, W.-T., Zhang, Z.-T., and He, W.-M. (2022). Electrochemical regioselective synthesis of N-substituted/unsubstituted 4-selanylisoquinolin-1(2H)-ones. Chin. Chem. Lett. 33, 1501–1504. doi:10.1016/j.cclet.2021.08.071
Xiang, P., Sun, K., Wang, S., Chen, X., Qu, L., and Yu, B. (2022). Direct benzylation reactions from benzyl halides enabled by transition-metal-free photocatalysis. Chin. Chem. Lett. 33, 5074–5079. doi:10.1016/j.cclet.2022.03.096
Yan, Q., Cui, W., Li, J., Xu, G., Song, X., Lv, J., et al. (2022). C–H benzylation of quinoxalin-2(1H)-ones via visible-light riboflavin photocatalysis. Org. Chem. Front. 9, 2653–2658. doi:10.1039/d1qo01910d
Yang, D., Yan, Q., Zhu, E., Lv, J., and He, W.-M. (2022). Carbon–sulfur bond formation via photochemical strategies: An efficient method for the synthesis of sulfur-containing compounds. Chin. Chem. Lett. 33, 1798–1816. doi:10.1016/j.cclet.2021.09.068
Yi, R., and He, W. (2021). Proton-coupled electron transfer for photosynthesis of phosphorylated N-heteroaromatics. Chin. J. Org. Chem. 41, 1267–1268. doi:10.6023/cjoc202100022
Yuan, X., Yang, G., and Yu, B. (2020). Photoinduced decatungstate-catalyzed C-H functionalization. Chin. J. Org. Chem. 40, 3620–3632. doi:10.6023/cjoc202006068
Zhang, H., Xu, J., Ouyang, Y., Yue, X., Zhou, C., Ni, Z., et al. (2022). Molecular oxygen-mediated selective hydroxyalkylation and alkylation of quinoxalin-2(1H)-ones with alkylboronic acids. Chin. Chem. Lett. 33, 2036–2040. doi:10.1016/j.cclet.2021.09.069
Keywords: photocatalysis, 2H-indazole, carbamoylation, green oxidant, antitumor
Citation: Ma C, Shang L, Zhao H, He X, Lv Q, Zhang D and Jiang Y (2022) Visible light-promoted transition metal-free direct C3-carbamoylation of 2H-Indazoles. Front. Chem. 10:1087834. doi: 10.3389/fchem.2022.1087834
Received: 02 November 2022; Accepted: 14 November 2022;
Published: 29 November 2022.
Edited by:
Wei-Min He, University of South China, ChinaReviewed by:
Ge Wu, Wenzhou Medical University, ChinaDaoshan Yang, Qingdao University of Science and Technology, China
Copyright © 2022 Ma, Shang, Zhao, He, Lv, Zhang and Jiang. This is an open-access article distributed under the terms of the Creative Commons Attribution License (CC BY). The use, distribution or reproduction in other forums is permitted, provided the original author(s) and the copyright owner(s) are credited and that the original publication in this journal is cited, in accordance with accepted academic practice. No use, distribution or reproduction is permitted which does not comply with these terms.
*Correspondence: Dandan Zhang, ddz1110@126.com; Yuqin Jiang, jiangyuqin@htu.edu.cn