- 1Oak Ridge National Laboratory, Oak Ridge, TN, United States
- 2Ultra Safe Nuclear Corporation, Oak Ridge, TN, United States
The reprocessing used nuclear fuel (UNF) releases volatile fission and activation products, including 129I, into the off-gas of a processing plant. Mitigation of the release of vapor phase radionuclides is necessary for meeting regulatory requirements in the United States and other countries. In an aqueous reprocessing plant, volatile radioiodine could be present in several forms, depending on the chemistry of the process used. Inorganic iodine will be the predominate species in any shearing or voloxidation pretreatment off-gas and dissolver off-gas (DOG). Organic iodides such as CH3I, C4H9I, and C12H25I have been proposed to be generated during solvent extraction; thus, these species must be captured from the vessel off-gas (VOG). The abatement of inorganic and organic iodide species to meet United States regulatory requirements has been demonstrated in laboratory experiments using Ag-based solid sorbents. The data presented in this paper includes the effect of gas composition (e.g., the presence of water vapor and NOx), iodine speciation (I2, CH3I, C4H9I, C12H25I), and sorbent bed parameters (e.g., temperature, sorbent age) on complete iodine capture on Ag-mordenite in an aqueous reprocessing plant.
1 Introduction
1.1 EPA regulatory limits on radioiodine release
The abatement of radioiodine from a nuclear fuel reprocessing facility in the United States would be governed by two regulatory bodies: the United States Environmental Protection Agency (EPA) and the United States Nuclear Regulatory Commission (NRC, 2012). Of these two regulatory bodies, EPA regulations impose stricter limits on the amount of iodine potentially released from any proposed facility. Under 40 CFR Part 190 (EPA-1, 1977), Environmental Radiation Protection Standards for Nuclear Power Operations, the total iodine release to the environment from the entire fuel cycle must be no greater than 0.005 Ci/GWy of 129I of electrical energy generated via the fuel cycle. A framework for meeting the 0.005 Ci/GWy for 129I, stipulated in 40 CFR 190 that also accounts for variations in source terms as a function of fuel burnup, was described in Jubin et al. (2012).
1.2 Iodine partitioning in an aqueous reprocessing facility
The general scheme of aqueous reprocessing plants follows a sequence of steps involving the shearing of fuel pins that have been stored between 3 and 5 years, the dissolution of the fuel in acidic media, separation of constituents using select partially immiscible organic extractants, such as tributyl phosphate (TBP) in an organic diluent such as kerosene, followed by product and waste stream treatment (Figure 1). It is estimated that >0.1% of radioiodine is released in the shearing step with a further >90% being released during the dissolution of the fuel. The remaining 5%–10% is carried into the solvent extraction steps.
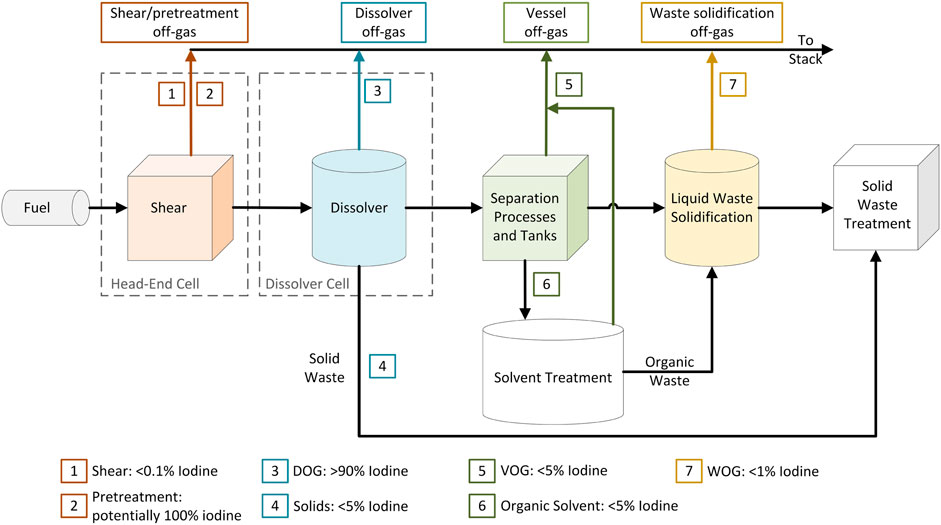
FIGURE 1. Diagram of an aqueous reprocessing facility with estimated iodine partitioning through the plant expressed as a percentage of the total initial used nuclear fuel (UNF) iodine inventory.
For used fuel with a burn up between 30 and 60 GW days per metric ton in heavy metal (GWd/tIHM), Jubin et al. estimate 129I activities of 0.92 and 0.90 Ci/GWy. This necessitates decontamination factors of 184 and 179 respectively. To meet these decontamination factors, a more thorough investigation into the specifics of 129I partitioning in a fuel reprocessing plant is required.
Jubin and Strachan (2015) estimated an initial iodine inventory of 368 g/tIHM based on simulations for fuel burnup of 60 GWd/tIHM after 5 years of cooling. Of this quantity, the bulk is expected to be observed in the shearing and dissolution process off-gas stream (i.e., DOG) (∼347g/tIHM) with the remainder observed in the solvent extraction step (i.e., vessel off-gas [VOG]) (∼10.7 g/tIHM) (Hebel and Cottone, 1982).
The forms and species of iodine -bearing compound relevant to aqueous reprocessing systems were investigated in Bruffey et al. (2015). By using enthalpy, entropy and heat capacity modeling, and free energy minimization, several thermodynamically favorable reaction pathways were identified based on organic and inorganic species found in reprocessing systems.
1.3 Organic speciation of iodine
Organic iodides likely form during solvent extraction, which may partition into the VOG. Degradation of tributyl phosphate and the organic diluent due to acid hydrolysis reactions and radiolysis yields shorter chain organic and radical species. Subsequent attacks by these radical species producing further short chain organics has been identified by Mincher et al. (2009), these species may react with iodide in the system to form organic iodides. Iodides of straight chain alkanes ranging from methyl to dodecyl were found during tests at the Wiederaufarbeitungsanlage Karlsruhe (WAK) (Herrmann et al., 1988). Dodecyl iodide has been reported as the most prevalent organic iodide species under process conditions in the VOG (Heinrich et al., 1981; Herrmann et al., 1988). The recycling of nitric acid streams results in the introduction of organic impurities from solvent extraction stages, into the dissolution stage promoting the formation of short chain organic iodides that end up in the DOG. Further, organic impurities have been shown to be present in commercially acquired nitric acids that are likely to be used in commercial scale activities. Nakamura et al. (1973).
1.4 Industrial methods of iodine capture
Several different methods have been proposed and implemented to varying degrees industrially to facilitate iodine capture from the off-gas. Among them, two major categories (solid adsorbent methods and wet scrubbing methods) exist. Wet scrubbing is done either as a caustic solution of 1–2 M NaOH (McKay, 1982) (Table 1). In cases of significant CO2 presence, KOH may be used to prevent sodium carbonate precipitation (Dean, 1999). Caustic scrubbing yields high efficiency for elemental iodine but poor efficiency for organic forms (Trevorrow et al., 1983). Alternatively, the Mercurex process, consisting of a scrub solution of 0.4 M Hg(NO3)2 and up to 14 M HNO3, yields higher removal efficiency for organic forms (Jubin, 1988). A number of silver based solid adsorbents have been found favorable for industrial use either alone or in conjunction with wet scrubbing techniques (McKay, 1982). For a complete review of iodine sorbents used historically, see Riley et al. (2016).
Reduced silver mordenite, the sorbent used in this study, has been researched as an iodine sorbent since the 1970s and has been demonstrated to effectively capture organic iodide (as CH3I) in addition to inorganic I2 (e.g., Thomas et al., 1978; Scheele et al., 1983; Nenoff et al., 2014).
1.5 Study objective
This paper presents a comprehensive overview of proposed methodologies for iodine capture from aqueous reprocessing off-gas using data collected through several sets of experiments that explored the generation and capture of organic iodides on solid sorbents. In order to meet EPA and NRC regulations, iodine abatement tests were designed to explore the effects of gas stream chemistry (i.e., varying [NO], [NO2], and [H2O]), iodine speciation (i.e., iodoalkanes vs inorganic iodine), and sorbent bed engineering design (i.e., flow rate, sorbent age, and sorbent bed temperature) on iodine capture by Ag mordenite. The results are presented by off-gas stream with a particular focus on the DOG, which will likely contain >90% of the iodine inventory, and the VOG, which could contain ∼5% of the iodine inventory.
2 Iodine speciation experiments
To confirm the potential speciation of organic iodides expected in DOG and VOG streams, simple bench-top experiments simulating dissolver and solvent extraction conditions have been conducted. Processes were simulated in a 500 ml round-bottom flask held in a heating mantle. Air flowed into the flask at ∼0.1 LPM and bubbled through the process liquid. Then, the off-gas generated in the headspace was sampled with a gas-tight syringe. Off-gas samples were analyzed in 200 ml volumes with an Agilent 8890 Ga Chromatograph (GC) coupled to a 5977B mass spectrometer. A 30 m, 0.25 mm inner diameter, DB-UI GC column was used with a temperature gradient of 30°C–200°C and a ramp time of 10°C/min. This method captured organic iodide standards ranging from CH3I to C12H25I. Peaks in the chromatogram were positively identified using their mass spectrum coupled to a NIST database in the MassHunter Software. These experiments provide the relative abundances of organic iodides in the headspace samples. The measurements are by gas phase standards, however these data cannot be used to calculate an extract concentration because of peak interferences from other volatile organics present in the headspace.
In the DOG simulations, the process liquid comprised 100 ml of 3M HNO3 that was previously contacted and separated from 100 ml of 30 vol% TBP/70 vol% n-dodecane mixture to represent recycled HNO₃ in a dissolver. The acid was heated to 100°C, and 1 ml of 10% KI solution was added to the flask (∼1,000 ppm I−). This iodine concentration is elevated above expected dissolver conditions but was chosen such that iodine speciation in the off-gas would be easily detectable. In VOG simulations, the process liquid comprised 50 ml of 30 vol% TBP/70 vol% n-dodecane mixture and 50 ml of the residual HNO₃ solution left over from the dissolver experiment. This aqueous-organic mixture was maintained at 40°C and mixed vigorously throughout the experiment. Off-gas samples were collected over 6 h during both experiments.
The DOG benchtop experiments primarily detected I2, followed by roughly equivalent concentrations of CH3I and C4H9I (∼1 ppm each). The presence of minor C4H9I was unexpected given prior focus on I2 and CH3I in the DOG literature. This alkyl iodide likely formed only in the experimental DOG because recycled acid containing TBP was used instead of fresh acid. Butyl iodide is not expected to form in conditions where fresh acid is used during dissolution, but CH3I has been proposed to form in fresh-acid experiments because of impurities in the acid (Nakamura et al., 1973). The VOG experiments resulted only in the detection of CH3I and C4H9I in the off-gas. No I2 or C12H25I was detected in the VOG. Although C12H25I may have formed in solution, its low vapor pressure likely precluded it from forming in any considerable quantity within the headspace.
3 Iodine abatement experiments
3.1 Sorbent selection
Silver-functionalized sorbents are considered the industry standard given the thermodynamically favorable reaction of Ag and iodine to form AgI or AgIO3 under most modeled off-gas capture conditions. These sorbents include Ag-mordenite (AgZ), Ag-faujasite (AgX), Ag-alumina (AgA), and Ag-aerogel (Routamo, 1996). Of these sorbents, AgZ is physically and chemically robust to NOx gasses in the DOG, given its high Si to Al ratio, while still maintaining a relatively high capacity for iodine. Thus, all experiments presented here reflect iodine adsorption onto AgZ.
Silver mordenite is procured from IONEX and contains 9.5–11.9 wt% Ag. The AgZ is reduced to Ag0Z in-house under a 4% H2/Ar gas stream, which significantly improves its iodine loading potential. The sorbent pellets are approximately 1.6 x 3 mm, with a bulk density of 1.87 g/cm3, surface area of 179 m2/g, and chemical formula of Ag4.09H4.12(AlO2)8.21(SiO2)43.26 ·xH2O (Nan, 2017). The chemical and physical effects of sorbent aging in off-gas streams have been well characterized (Wren et al., 1986).
The silver in the mordenite chemically reacts with iodine to form AgI. This compound is stable under most industrial conditions, therefore AgZ likely not suitable for regeneration. Jubin et al. (2019) attempted regeneration of iodine-loaded AgZ and AgNO3-impregnated alumina at 200°C and found that less than 1% of the adsorbed iodine was released over 3 h.
3.2 Experimental methods
A thermogravimetric analyzer has been used to determine iodine mass gain on AgZ. A custom-manifold upstream of the TGA allows various components to be valved-in to the simulated off-gas stream (Figure 2). This includes NOx gasses (NO + NO2), humid air, and iodine species (i.e., I2, CH3I, C4H9I, or C12H25I). All flow rates are regulated with Sierra Mass Flow controllers that display the active flow rate for monitoring. Nominal tests run at a total superficial velocity of 10 m/min at 150°C. Apparent mass gain measured with the TGA is confirmed with neutron activation analysis (NAA) at the High Flux Isotope Reactor at Oak Ridge National Laboratory. Detailed methods can be found in other works (Greaney et al., 2020; Greaney et al., 2021).
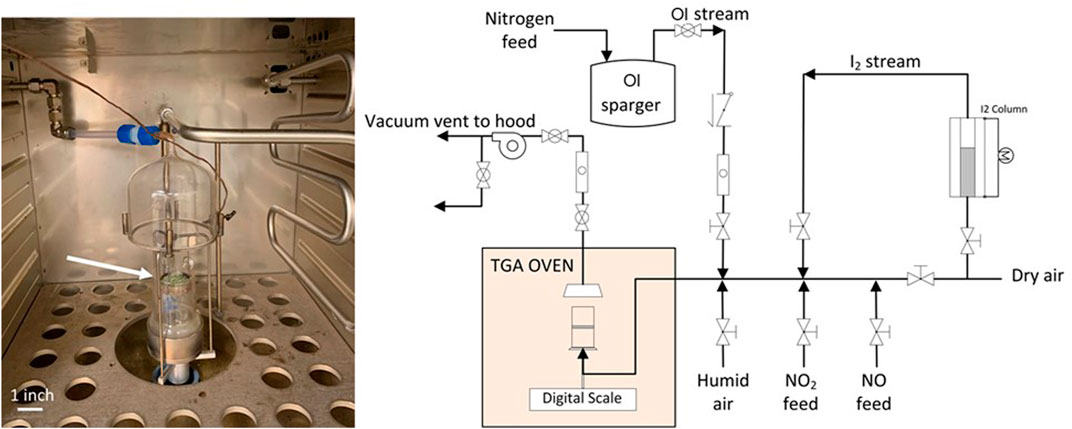
FIGURE 2. Photograph of the TGA thin bed that contains AgZ pellets that reacted with iodine to form AgI or AgIO3 (green pellets at end of arrow) (left); schematic of the manifold used for TGA testing at ORNL (right). OI (right figure) represents organic iodide spargers.
Gaseous iodine is generated by flowing a known flow rate of air through a packed bed of crystalline I2 held at 18°C, then diluting the I2-saturated gas stream into a larger gas flow to a set concentration. Vapor phase organic iodides are generated with the same concept but with liquid CH3I, C4H9I, and C12H25I bubblers held constant at ‒30°C, 20°C, and 75°C, respectively. Nitric oxide is generated with a compressed cylinder, and NO2 is generated by heating a cylinder of liquid N2O4 to generate NO2 at a set flow rate. Water vapor is added to the gas streams with a water bubbler.
4 DOG iodine capture
4.1 DOG experimental design
In a nominal aqueous reprocessing flow sheet, the DOG is expected to contain >90% of the iodine present in the used nuclear fuel (Sakurai et al., 1989). This iodine is predominately in inorganic forms, such as I2 (Sakurai et al., 1989; Sakurai et al., 1997), but minor CH3I and C4H9I may form, as shown thermodynamically (Bruffey et al., 2015) and experimentally in this study. The DOG contains other chemical components that could affect iodine sorption onto Ag-based sorbents, mainly water and NOx gasses. The DOG is a humid gas stream with a dew point potentially >20°C, depending on the configuration of condensers upstream of the iodine sorbent bed. Additionally, heating HNO₃ will form NO2 and NO gas, and concentrations will likely range between 0.5% and 1.5% total NOx (Birdwell 1990).
To test the effects of water and NOx on iodine sorption onto AgZ, a test matrix of eight experiments was designed using a fractional factorial analysis scheme for both I2 and CH3I following the study performed in Jubin (1981). Four variables were explored: sorbent bed temperature of 135°C or 165°C, dew point of ‒70°C or 0°C, NO2 concentration of 0% or 1%, and NO concentration of 0% or 1%. These temperatures were chosen to bracket the optimized operating temperature of 150°C to determine if slightly adjusting the temperature could increase iodine sorption. The water concentrations were chosen to compare an extremely dry system to the humid stream expected in the DOG. The NO and NO2 gas concentrations were selected from the experimental results of Birdwell (1990) that show the NO + NO2 concentration in the off-gas typically fluctuate between 0% and 1.5%, after the gas is scrubbed with two condensers.
Vapor phase I2 (25 ppm-mol) was flowed over a thin bed of AgZ. Mass gain was measured in real time using a TGA, and iodine loading was confirmed with NAA. Tests were run until loading was complete (i.e., when the TGA loading curve did not show any mass gain for at least 24 h). This typically occurred in 1–2 weeks. The results of these tests are presented as box and whisker plots in Figure 3 in which iodine loading is measured in milligrams of iodide per Gram of sorbent (y-axis), and the two set points for each variable are plotted on the x-axis. Nominal iodine loading on AgZ (9.5–11.9 wt% Ag) under dry conditions results in ∼100 mg I per g of sorbent (stylized as mg I/g sorbent). Replicate tests suggest that errors on iodine loading experiments vary within 10 mg I/g sorbent.
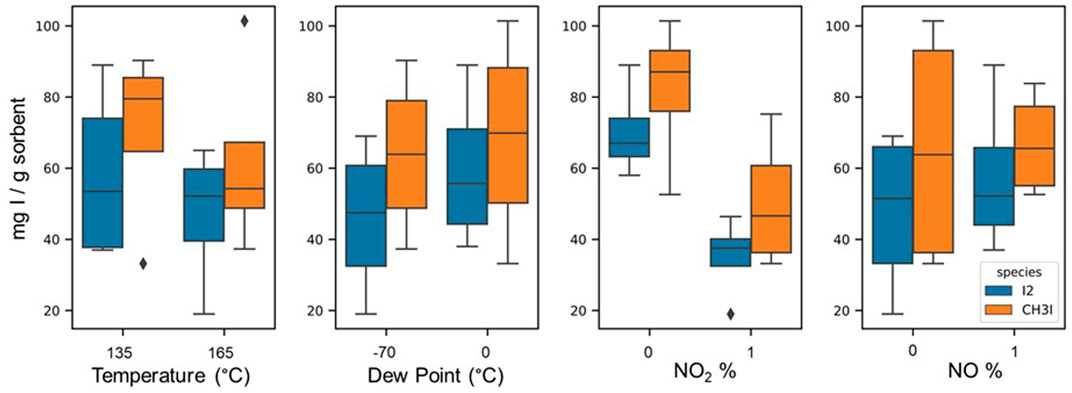
FIGURE 3. Effects of each temperature, dew point, NO2 vol%, and NO vol% on I2 and CH3I sorption (mg I/g sorbent) on AgZ. Each box and whisker plot represents iodine loading results of four tests. Iodine data are in blue (left-hand boxes), and CH3I data are in orange (right-hand boxes).
4.2 DOG results
These results indicate that the presence of NO and the dew point of the gas stream do not affect iodine sorption on AgZ in a statistically significant way over ∼2 weeks test durations. Increasing the temperature of the sorbent bed from 135°C to 165°C has a slightly negative effect on both I2 and CH3I sorption, dropping average loadings to ∼55 mg I/g sorbent. Thus, sorbent beds are recommended to be maintained below 165°C. Nitrogen dioxide has the most detrimental effect, decreasing sorbent capacity by up to 62% with typical loadings between 30 and 50 mg I/g of sorbent. This is likely due to aging effects on the sorbent as the NO2 oxidizes the Ag0 to Ag1+, forming Ag2O and decreasing the overall reactive potential for AgI or AgIO3 to form. When an equivalent volume percent of NO is added to the system in addition to NO2, iodine sorption increased relative to tests with solely NO2. This implies that adding NO decreases the effect of sorbent oxidation by NO2. Thus, the ratio of NO to NO2 in the DOG should be monitored to assess sorbent efficiency potential.
The detrimental effect of NO2 on AgZ capacity must be considered when designing flow sheets for sorbent beds in an aqueous reprocessing facility. Although AgZ has a maximum sorbent efficiency of ∼100 mg I/g sorbent, the effect of NOx aging will likely lower actual sorbent efficiency to 40–50 mg I/g sorbent. Nevertheless, deep-bed tests completed at Idaho National Laboratory in the presence of NO2 show that AgZ sorbent beds can maintain decontamination factors of >10,000 for I2 and >1,000 for CH3I, even with sorbent capacities decreased to ∼40 mg I/g sorbent (Bruffey et al., 2019). The tests conducted in this paper and at INL suggest that DOG iodine sorbent systems should be conservatively modeled to accommodate 40–50 mg I/g sorbent to meet required decontamination factors.
5 VOG iodine capture
5.1 VOG experimental design
In a nominal aqueous reprocessing flow sheet, the VOG is expected to contain an estimated 5% of the total iodine inventory. Residual iodine in the dissolver can remain in solution or as AgI or PdI colloids that precipitate out (Figure 1). Any iodine that remains in the aqueous phase will be transferred to the separation stage where it will either partition into the organic phase or be volatilized into the VOG. Here, organic iodides such as CH3I, C4H9I, and C12H25I have been suggested to form in the off-gas in parts per billion quantities (Jubin et al., 2013; Bruffey et al., 2015). Although the VOG simulation experiments in this study did not detect C12H25I in the off-gas, it was still included in the AgZ sorption experiments. If present in the VOG, then all these iodine species must be abated to meet United States regulatory standards.
A series of experiments were completed to test the effects of varying organic iodide speciation and concentration in the off-gas, superficial velocity of the off-gas, and effects of aging on AgZ sorbent capacity. For these experiments, ∼2 g of reduced AgZ were loaded into the thin-bed TGA and exposed to gas streams containing 5, 10, or 50 ppm of CH3I, C4H9I, and C12H25I until the sorbent reached saturation. The time to saturation and total saturation capacity were recorded. Because the VOG stream has a lower number of iodine moles passing through the sorbent beds, beds may stay online in a facility longer than DOG beds. Thus, these experiments were repeated with AgZ that had been aged for 9 months in a humid gas stream to determine how long-term sorbent aging may affect sorbent capacity.
5.2 VOG results
These experiments found that the sorption rate of organic iodides by AgZ depends on the hydrocarbon chain length and the concentration in the off-gas. At 50 ppm in the off-gas, the sorption rate on to fresh AgZ is 8% slower for CH3I, 20% slower for C4H9I, and 40% slower for C12H25I relative to I2, which averages 0.70 mg I per g sorbent per hour (Figure 4). The slower loading rate of the longer-chain organics implies that they may be more penetrative into the sorbent bed than I2. When 9-month aged sorbent was tested in lieu of “fresh” sorbent, sorption rate on to AgZ decreased by 40%–60% for the organic iodides in concentrated (50 ppm) gas streams. At more realistic VOG concentrations (5 ppm), the sorption rate on to fresh AgZ of the three organic iodides is nearly identical: 0.14 mg I/g sorbent/hour ( ± 0.03 mg I/g sorbent/hour). When a 50% reduction in sorption rate due to aging is factored in, this iodine sorption rate may be nearer to 0.07 mg I/g sorbent/hour.
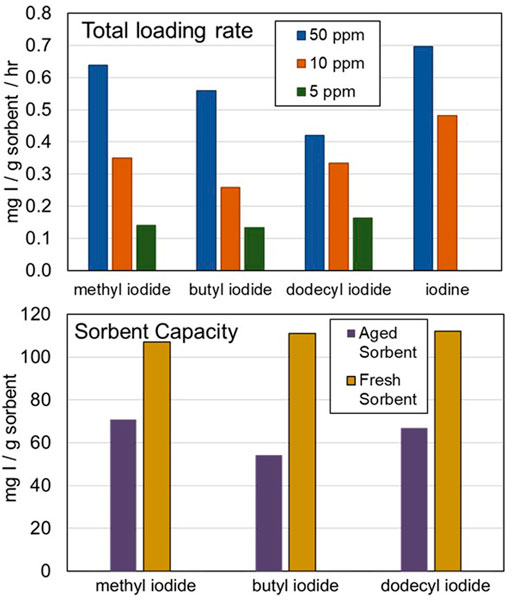
FIGURE 4. Summary of organic iodide experimental results. (Top) Effects of organic iodide chain length and concentration on total loading rate. (Bottom) Effects of sorbent aging on total sorbent capacity for organic iodide adsorption.
Although the sorption rate varies as a function of hydrocarbon chain length, the saturation concentration does not. The capacity of fresh AgZ ranges from 106 to 112 mg I/g sorbent for the three organic iodides and I. Aging AgZ in a humid air stream for 9 months drops the overall sorbent capacity by ∼35% for CH3I, ∼50% for C4H9I, and ∼40% for C12H25I for an overall iodine capacity of 35–70 mg I/g sorbent. These data mirror the results of the DOG testing, which shows that sorbent that is more quickly aged in a highly oxidizing environment has an iodine capacity between 40 and 50 mg I/g sorbent. Because the VOG stream does not contain the strong oxidant NO2, the sorbent likely ages slower relative to DOG sorbent. The reduction in sorbent capacity from ∼100 mg I/g sorbent to ∼50 mg I/g sorbent may occur over longer timescales in the VOG than the day-long timescales observed in the DOG testing. However, these bench-scale tests did not include additional chemical components (e.g., volatilized organics) that could be present in the VOG that could reduce sorbent capacity for iodine due to physiosorption. The effect of these components should be further investigated.
Throughout the long-chain organic iodide testing, C12H25I was difficult to maintain in the gas phase in the simulated VOG. The vapor pressure of C12H25I is exceedingly low: 0.7 mm Hg compared with 314 mm Hg for C4H9I at 100°C (Li and Rossini 1961). Thus, if any point of the VOG is not heat-traced or insulated, there is a high likelihood that C12H25I will condense out of the gas phase. Herrmann et al. (1988) previously suggested that C12H25I was the predominant phase in the VOG; however, this phase was measured in a cold trap and not directly in the gas phase. The experiments presented here suggest that C4H9I and CH3I are more prominent in the VOG.
6 Conclusion
Multiple experiments were conducted to comprehensively assess the abatement of radioiodine across the DOG and VOG in an aqueous reprocessing facility. These experiments assessed the effects of gaseous components (e.g., NOx and humidity in the DOG) and iodine speciation on abatement behavior. The DOG will primarily contain I2 and minor CH3I and C4H9I. Although AgZ may have a full capacity of ∼100 mg I/g sorbent, the effect on the sorbent exposed to the chemistry of the DOG and longevity of the VOG iodine sorbent systems should be conservatively modeled to accommodate a capacity of 40–50 mg I/g sorbent on AgZ to meet required decontamination factors.
Data availability statement
The raw data supporting the conclusion of this article will be made available by the authors, without undue reservation.
Author contributions
AG conducted laboratory experiments and co-authored the manuscript. SB planned and conducted laboratory experiments. RN contributed a literature review and co-authored the manuscript. LM co-authored the manuscript.
Funding
This work was completed under funding by the Material Recovery and Wasteform Development program under the United States Department of Energy.
Acknowledgments
The authors acknowledge David Glasgow for conducting NAA at Oak Ridge National Laboratory and Amy Welty and Nick Soelberg at Idaho National Laboratory for their thoughtful discussions and contributions to this work.
Conflict of interest
SB is employed by Ultra Safe Nuclear Corporation.
The remaining authors declare that the research was conducted in the absence of any commercial or financial relationships that could be construed as a potential conflict of interest.
Publisher’s note
All claims expressed in this article are solely those of the authors and do not necessarily represent those of their affiliated organizations, or those of the publisher, the editors and the reviewers. Any product that may be evaluated in this article, or claim that may be made by its manufacturer, is not guaranteed or endorsed by the publisher.
References
Birdwell, J. F. (1990). “Iodine and NOx behavior in the dissolver off-gas and IODOX systems in the Oak Ridge National laboratory integrated equipment test facility,” in Proceedings of the 21st DOE/NRC Nuclear Air Cleaning Conference, San Diego, California, 13-16 August 1990 (Oak Ridge National Laboratory).
Bruffey, S. H., Greaney, A. T., Jubin, R. T., Soelberg, N. R., and Welty, A. (2019). Kinetics of aging process on reduced Ag exchanged mordenite in dry air and humid air. AIChE J. 67, e17182. doi:10.1002/aic.17182
Bruffey, S., Spencer, B. B., Strachan, D. M., Jubin, R. T., Soelberg, N. R., and Riley, B. J. (2015). “A literature survey to identify potentially problematic volatile iodine-bearing species present in off-gas streams,”. ORNL-SPR-2015/290.
Dean, J. A (Editor) (1999). Lange’s handbook of chemistry. 15th edition (New York, United States: McGraw-Hill).
EPA-1 (1977). Protection of environment: Chapter I–environmental protection agency (continued), environmental radiation protection standards for nuclear power Operations 40CFR190.10. Washington, DC: US Environmental Protection Agency.
Greaney, A. T., Bruffey, S. H., and Jubin, R. T. (2020). Effect of NOx and water variations on iodine loading of AgZ. Oak Ridge: ORNL-TM.
Greaney, A. T., Bruffey, S. H., Welty, A. K., and Soelberg, N. R. (2021). “Organic iodide sorption from dilute gas streams,”. ORNL-TM-2021-2198.
Hebel, W., and Cottone, G. (1982). Management modes for iodine-129 (No. CONF-810971-). New York: Harwood Academic Pub.
Heinrich, E., Schmieder, H., Roesch, W., and Weirich, F. (1981). “Improved iodine and tritium control in reprocessing plants,” in Proceedings of the 16th DOE Nuclear Air Cleaning Conference, Cambridge, MA, 14 July 1981 (The Harvard Air Cleaning Laboratory), 612–627.
Herrmann, B., Motoi, V., Fies, H., Stojanik, B., Furrer, J., and Kaempffer, R. (1988). “Testing an iodine filter for the vessel off gas of the German industrial-scale reprocessing plant (NUREG/CP-0098, CONF-880822),” in Proceedings of the 20th DOE/NRC Nuclear Air Cleaning Conference, Cambridge, MA, 16 January 1988 (The Harvard Air Cleaning Laboratory), 234–246.
Jubin, R. T. (1988). Airborne waste management technology applicable for use in reprocessing plants for control of iodine and other off-gas constituents. United States: Oak Ridge National Laboratory, 68. doi:10.2172/5169490
Jubin, R. T., Greaney, A. T., Spencer, B. B., and Bruffey, S. H. (2019). Testing of an iodine and tritium capture system for an NO-based tritium pretreatment process. United States: Oak Ridge National Laboratory. doi:10.2172/1617811
Jubin, R. T. (1981). “Organic iodine removal from simulated dissolver off-gas streams using partially exchanged silver mordenite,” in 17th DOE Nuclear Air Cleaning Conference, San Diego, California, 13-16 August 1990 (Oak Ridge National Laboratory).
Jubin, R. T., Soelberg, N. R., Strachan, D. M., and Ilas, G. (2012). Fuel age impacts on gaseous fission product capture during separations (No. PNNL-22550). Richland, WA, United States: Pacific Northwest National Lab.
Jubin, R. T., and Strachan, D. (2015). Assessments and options for removal and immobilization of volatile radionuclides from the processing of used nuclear fuel (No. ORNL/SPR-2015/115). Oak Ridge, TN: Oak Ridge National Lab.
Jubin, R. T., Strachan, D. M., and Soelberg, N. R. (2013). Iodine pathways and off-gas stream characteristics for aqueous reprocessing plants – a literature survey and assessment. United States: Idaho National Laboratory.
Li, J. C. M., and Rossini, F. D. (1961). Vapor Pressures and Boiling Points of the l-Fluoroalkanes, l-Chloroalkanes, l-Bromoalkanes, and l-Iodoalkanes, C1to C20. J. Chem. Eng. Data 6, 268–270. doi:10.1021/je60010a025
McKay, H. A. C. (1982). “Management modes for iodine-129,” in Proceedings of a meeting organized by the Commission of the European Communities, Brussels, Belgium, 25 Feburary 1982 (United States: Oak Ridge National Laboratory).
Mincher, B. J., Modolo, G., and Mezyk, S. P. (2009). Review article: The effects of radiation chemistry on solvent extraction: 1. Conditions in acidic solution and a review of TBP radiolysis. Solvent Extr. Ion. Exch. 27 (1), 1–25. doi:10.1080/07366290802544767
Nakamura, K., Saeki, M., and Tachikawa, E. (1973). formation of organic iodides upon heating nitric acid solutions containing carrier-free lodine-131. J. Nucl. Sci. Technol. 10 (6), 367–373. doi:10.1080/18811248.1973.9735435
Nan, Y. (2017). Adsorption of Iodine and Water on Silver-Exchanged Mordenite. Doctor of Philosophy (PhD) Dissertations. (United States: Sryacuse University).
Nenoff, T. M., Rodriguez, M. A., Soelberg, N. R., and Chapman, K. W. (2014). Silver-mordenite for radiologic gas capture from complex streams: Dual catalytic CH3I decomposition and I confinement. Microporous Mesoporous Mater. 200, 297–303. doi:10.1016/j.micromeso.2014.04.041
NRC (2012). Energy: Part 20 - standards for protection against radiation. Washington, DC: US Nuclear Regulatory Commission.
Riley, B. J., Vienna, J. D., Strachan, D. M., McCloy, J. S., and Jerden, J. L. (2016). Materials and processes for the effective capture and immobilization of radioiodine: A review. J. Nucl. Mater. 470, 307–326. doi:10.1016/j.jnucmat.2015.11.038
Routamo, T. (1996). “Effect of hypoiodous acid volatility on the iodine source term in reactor accidents,” in Proceedings of the Fourth CSNI Workshop on the Chemistry of Iodine in Reactor Safety, 10-12 June 1996 (Würenlingen, Switzerland: Paul Scherrer Institute), 635–653.
Sakurai, T., Takahashi, A., Ishikawa, N., and Komaki, Y. (1989). The behavior of iodine in a simulated spent-fuel solution. Nucl. Technol. 85 (2), 206–212. doi:10.13182/nt89-a34241
Sakurai, T., Takahashi, A., Ye, M., Kihara, T., and Fujine, S. (1997). Trapping and measuring radioiodine (Iodine-129) in cartridge filters. J. Nucl. Sci. Technol. 34 (2), 211–216. doi:10.1080/18811248.1997.9733648
Scheele, R. D., Burger, L. L., and Matsuzaki, C. L. (1983). Methyl iodide sorption by reduced silver mordenite, PNNL-4489, UC-70. Richland, Washington: Pacific Northwest National Laboratory.
Thomas, T. R., Staples, B. A., and Murphy, L. P. (1978). “The development of Ag°Z for bulk 129I removal from nuclear fuel reprocessing plants and PbX for 129I storage,” in Proceedings of the 15th DOE Nuclear Air Cleaning Conference (CONF-780819-15), Cambridge, MA, 21 August 1978 (The Harvard Air Cleaning Laboratory), 394.
Trevorrow, L. E., Vandegrift, G. F., Kolba, V. M., and Steindler, M. J. (1983). Compatibility of technologies with regulations in the waste management of H-3, I-129, C-14, and Kr-85. Part I Initial information base. United States: Oak Ridge National Laboratory. doi:10.2172/5795448
Wilhelm, J. G., and Furrer, J. (1977). CEC seminar on radioactive effluents from nuclear fuel reprocessing plants. Karlsruhe: European Commission.
Keywords: aqueous reprocessing, radioiodine, silver mordenite, off-gas, organic iodide
Citation: Greaney AT, Ngelale RO, Bruffey SH and Martin LR (2023) Abatement of radioiodine in aqueous reprocessing off-gas. Front. Chem. 10:1078668. doi: 10.3389/fchem.2022.1078668
Received: 24 October 2022; Accepted: 28 December 2022;
Published: 11 January 2023.
Edited by:
Dan Gregg, Australian Nuclear Science and Technology Organisation, AustraliaReviewed by:
Phuoc Hoang Ho, Chalmers University of Technology, SwedenBarbara Tora, AGH University of Science and Technology, Poland
Copyright © 2023 Greaney, Ngelale, Bruffey and Martin. This is an open-access article distributed under the terms of the Creative Commons Attribution License (CC BY). The use, distribution or reproduction in other forums is permitted, provided the original author(s) and the copyright owner(s) are credited and that the original publication in this journal is cited, in accordance with accepted academic practice. No use, distribution or reproduction is permitted which does not comply with these terms.
*Correspondence: Allison T. Greaney, Z3JlYW5leWF0QG9ybmwuZ292