- Flavors and Fragrance Engineering and Technology Research Center of Henan Province, College of Tobacco Science, Henan Agricultural University, Zhengzhou, China
A practical method to synthesize N-heteroaryl esters from N-heteroaryl methanols with acyl cyanides via C–C bond cleavage without using any transition metal is demonstrated here. The use of Na2CO3/15-crown-5 couple enables access to a series of N-heteroaryl esters in high efficiency. This protocol is operationally simple and highly environmentally benign producing only cyanides as byproducts.
Introduction
Heteroaryl esters and their derivatives could serve as interesting building blocks for the preparation of various functionalized products including bioactive natural products, pharmaceuticals, dyes, and flavors (Otera, 2010; Trotier Faurion et al., 2013; Armani et al., 2014; Liu B. et al., 2015; Xu et al., 2018; Bayout et al., 2020; Xu et al., 2022). Therefore, methodologies for the synthesis of these molecular architectures have experienced huge developments in recent years. The conventional syntheses include interesterification and oxidative carbonylation of ethers (Zhao et al., 2014; Lu et al., 2015), and the reactions of alcohols with methanol (Zhang and Wang, 2019), carboxylic acids (Teruaki et al., 2003; Saeed et al., 2008), aldehydes (Tang et al., 2014; Huang et al., 2016; Chun and Chung, 2017), ketones (Huang et al., 2014; Rammurthy et al., 2021), aliphatic amides (Hie et al., 2016; Bourne-Branchu et al., 2017), carbonates (Chen et al., 2014), and acid halides (Tamaddon et al., 2005; Akhlaghinia et al., 2010), respectively. In the last decade, direct activation and functionalization of the C–H bond has emerged as a powerful method in the field of organic synthesis and witnessed significant progress (McMurray et al., 2011; Huang et al., 2012; Wencel-Delord and Glorius, 2013; Chen et al., 2015; Liu C. et al., 2015; Dong et al., 2017). For example, successful benzylic C (sp3)-H acyloxylations of alkyl N-heteroarenes were achieved using simple aldehydes and acids via a copper or palladium catalysis (Scheme 1A) (Jiang et al., 2010; Chen et al., 2018; Cheng et al., 2019). Moreover, Soulé and coworkers achieved oxidative esterification of aldehydes with prefunctionalized 2-alkylheterocycle N-oxides via copper catalysis (Scheme 1B) (Wang et al., 2017).
In recent years, the process of carbon–carbon (C–C) bond cleavage of ketones under transition metal (Fe, Cu, or Ru) catalysis has provided various ester compounds (Yan et al., 2014; Huang et al., 2016; Arzumanyan, 2017).
Recently, a simple and direct aerobic oxidative esterification reaction of arylacetonitriles with alcohols/phenols is achieved in the presence of a copper salt and molecular oxygen (Dong et al., 2021). On the other hand, Song and Plietker groups reported aerobic oxidative C–CN bond cleavage of arylacetonitriles leading to various esters with catalysis of Fe and Ru, respectively (Scheme 1C) (Kong et al., 2016; Eisele et al., 2019). In general, ester compounds could be achieved using acylcyanides as acylating agents. Cu-catalyzed esterification using acyl cyanides with alcohols to yield the corresponding cyano-substituted esters is also reported (Scheme 1D) (Chen et al., 2020).
Furthermore, esterification reactions could proceed using photochemical strategies, in which reaction mechanisms involve mainly single electron transfer, energy transfer, or other radical procedures (Deng et al., 2021). A novel and metal-free method for the synthesis of α-ketoesters from β-ketonitriles and alcohols was reported, but under visible light irradiation conditions (Xu et al., 2018). Besides, the acyloxycarbonyl compounds could be obtained by TBAI-catalyzed acyloxylation of ketones (Uyanik et al., 2011; Guo et al., 2014), which the excess TBHP was required for the reaction process (Scheme 1E). Very recently, Subaramannian et al. reported t-BuOK catalyzed esterification using acyl cyanides with alcohols (Scheme 1F); however, their applicability was limited to N-heteroaryl methanols (Subaramanian et al., 2020). In addition, transition metal-free activation of amides by cleavage of C–N bond to obtain the aryl esters is well known (Li et al., 2018; Li and Szostak, 2020). Therefore, these reported esterification protocols involve the metal catalysts, lack of step efficiency, conditions of light irradiation, limited substrate scope of N-heterocyclic compounds, and need of oxidants. In our continuous effort in the construction of N-heteroaryl compounds (Lai et al., 2018; Zhai et al., 2018), we disclose herein an efficient and new protocol for the base-promoted esterification of N-heteroaryl methanols via C–C bond cleavage of arylacetonitriles acyl cyanides as acylating sources (Scheme 1G). The present protocol is simple to handle and does not involve any metal catalyst detrimental to environmental safety.
Result and discussion
Our initial optimization using 2-pyrazinylmethanol 1a and benzoylacetonitrile 2a as the model substrates revealed that the reaction proceeded as anticipated with 1 equiv of t-BuOK in toluene according to the reported conditions (Roy et al., 2019; Subaramanian et al., 2020), affording the desired product 3a in 10% yield (Table 1, entry 1). No product was detected in the absence of base (Table 1, entry 2), which suggests that it played a crucial role. Further screening of other bases indicated that Na2CO3 was obviously superior to the others, providing the desired product 3a in 25% isolated yield (Table 1, entries 3–9 vs. entry 1). Interestingly, an improved yield (61%) was obtained when the reaction was conducted in the presence of 15-crown-5 (Table 1, entry 10).The role of crown ether in the reaction is increasing the solubility of base in solvent and known to be effective for trapping the potassium and sodium ions (Liotta et al., 1974; Lutz et al., 1988; Hay et al., 1993; Reuter et al., 1999). Subsequently, the evaluation of additives was conducted, and the results indicated that none of the screened additives (PPh3, 1,10-Phen, and TMEDA) benefited the outcome (29%–32%, Table 1, entries 11–13 vs. 10). Thus, 15-crown-5 was selected as the additive to assess the effect of solvents, including 1,4-dioxane, DMF, DMSO, DCE, and THF (Table 1, entries 14–18). All of the attempts did not show any improvement on the reaction yield compared with toluene (Table 1, entry 10). Then, we explored the other reaction parameters including the reaction temperature, the mol ratio of 1a and 2a, and the amount of additive. The yield was not much improved compared with the yield of 140°C (51%) when the reaction was conducted at 150°C (62%, Table 1, entry 20 vs. 10). Therefore, the reaction temperature remains unchanged. Gratifyingly, when the mole ratio of 1a and 2a was modified from 1:1 to 2.5:1, the reaction provided the best yield (85%) of 3a (Table 1, entry 22 vs. entry 10). Then, the effect of the amount of additive and reaction time was examined; unfortunately, no better results were obtained (Table 1, entries 23–25). Finally, the optimal reaction conditions were identified as follows: The mixture of 1a (0.5 mmol), 2a (0.2 mmol), Na2CO3 (0.2 mmol), and 15-crown-5 (0.2 mmol) was stirred in toluene at 140°C under N2 atmosphere for 24 h.
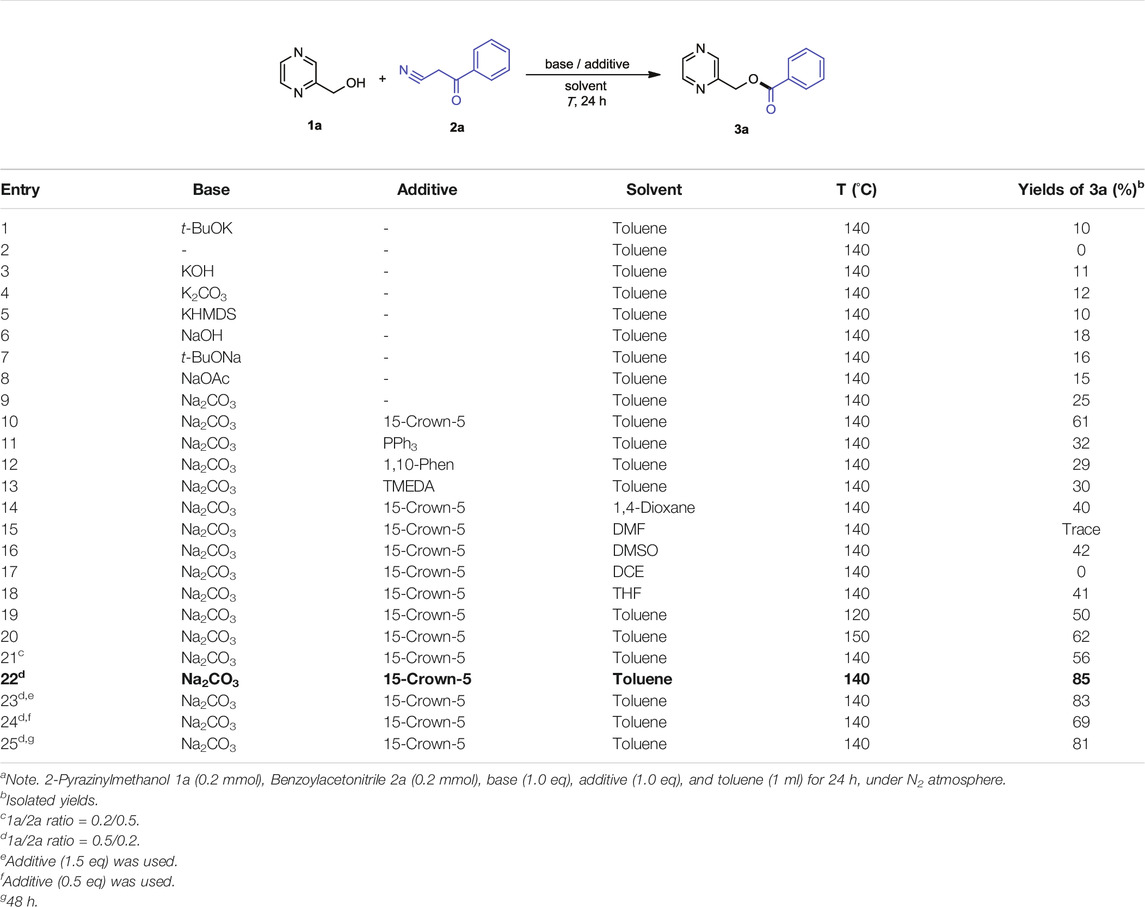
TABLE 1. Optimization of reaction conditionsa.
With the optimized reaction conditions in hand, we next sought to generalize the protocol on a range of diverse substrates. As shown in Scheme 2, various commercially available N-heteroaryl methanols and benzoylacetonitrile were exposed to the standard reaction conditions, and the desired heteroarenemethyl benzoates were successfully afforded with good yields. Initially, pyrazinylmethanol was substituted by an electron-donating group such as Me− or MeO−, and we obtained the desired products with moderate to good yields (3b, 85%) and (3c, 76%), respectively, while, the electron-withdrawing substituent Cl− also gave the corresponding ester 3d at a moderate yield of 77%. 2-pyridinylmethanols with 3-methyl, 6-methyl, 6-methoxy, 4-Br, 5-Br, 6-Cl, and 6-Br groups were converted to the corresponding products with lower yields (3e-3l, 62%–80% yields). It was worth mentioning that the yields were slightly decreased by the presence of different substituents on the 2-pyridinylmethanols. However, 3- and 4-pyridinylmethanol exhibited good reactivity obtaining the esterification products 3m and 3n in 90% and 83% yield, respectively. 4-Pyridinylmethanol bearing electron-withdrawing substituent (2-Br) revealed the lower yield of desired product (3o, 73%). The reactions of benzoylacetonitrile with furanmethanols, thiophenemethanols, and 4-quinolylmethanol also smoothly afforded the corresponding products 3p–3t in yields of 77%–85%. As we expected, both α-methyl-2-pyrazinemethanol, 2-pyrazinylethyl alcohol, and 2-pyridinylethyl alcohol worked well to give the corresponding products 3u–3w in 78%–83% yield. Interestingly, simple alcohols such as benzyl and aliphatic (1v–1y) could be used as substrates under the present conditions, and the desired products (3x–3z) were obtained at the yields of 81%, 92%, and 88%, respectively. The results indicated that the simple unhindered aliphatic alcohols show the excellent nucleophilic activity.
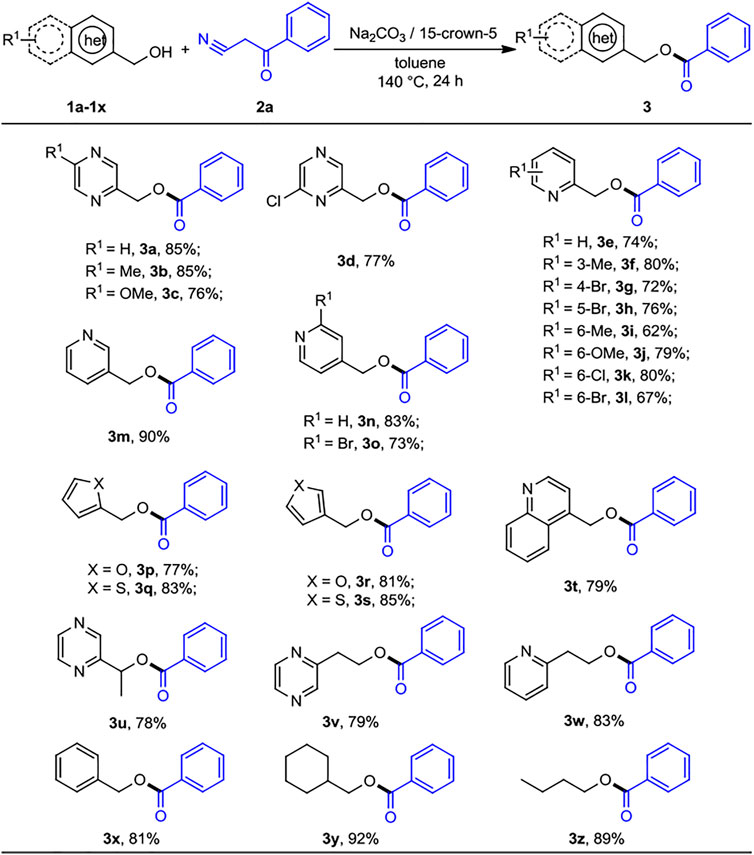
SCHEME 2. Substrate scope of heteroaryl methanols for the acylation reactions. a,bReaction conditions: aheteroaryl methanols 1 (0.5 mmol), benzoylacetonitrile 2 (0.2 mmol), Na2CO3 (0.2 mmol), 15-crown-5 (0.2 mmol), and toluene (1 ml) at 140°C for 24 h, under N2 atmosphere. bIsolated yields.
Next, the scope of the reaction was evaluated on various benzoylacetonitrile derivatives under the optimized reaction conditions, and the results are summarized in Scheme 3. Benzoylacetonitrile containing electron-donating groups methoxy (2b, 2g) and methyl (2f) were successfully transformed to the corresponding esters to give the products (4a, 4e, and 4f) at good yields of 76%–81%. Slight lower yields were obtained when electron-withdrawing groups 4-F, 4-Cl, 4-Br, 3-Cl, and 3-CF3 substituted benzoylacetonitriles with 2-pyrazinylmethanol were subjected to this transformation (4b–4d, 4g–4h, 64%–78% yields). Interestingly, 2- and 4-pyridinylmethanol bearing the OMe group and halogen atom (Cl, Br, and CF3) at the para or meta position are well tolerated, as the products of pyridin-2-ylmethyl and -4-ylmethyl substituted benzoates, 4i–4t were obtained in 73%–85% yields. The reactions of benzoylacetonitriles substituted at the para or meta position by various groups (OMe, Cl, and Br) with 2-furanmethanol and 2-thiophenemethanol underwent a smooth reaction to provide the products 4u–4b′ in good to excellent yields. Moreover, pyvaolylacetonitrile displayed the good reactivity, as 4c′ was isolated in 89% yield.
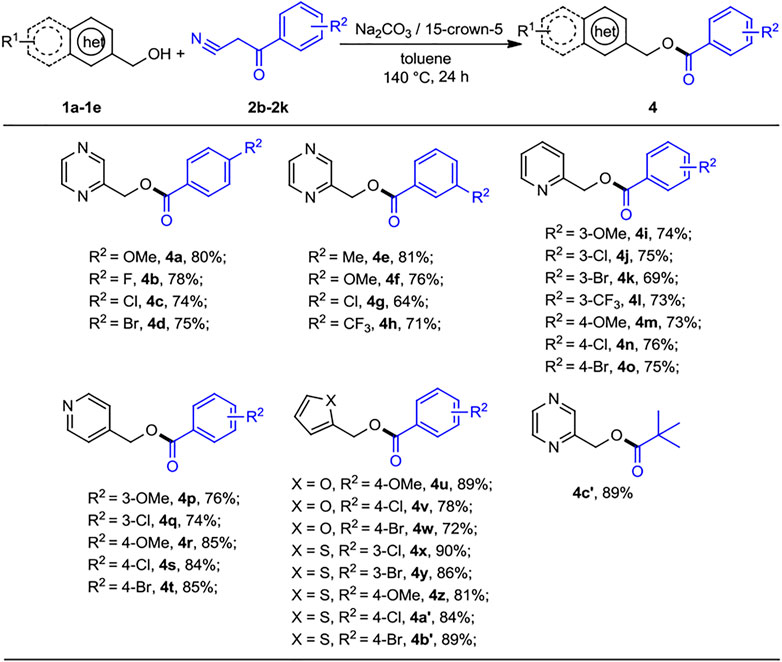
SCHEME 3. Substrate scope of benzoylacetonitriles for the acylation reactions. a,bReaction conditions: aheteroaryl methanols 1 (0.5 mmol), benzoylacetonitrile derivatives 2 (0.2 mmol), Na2CO3 (0.2 mmol), 15-crown-5 (0.2 mmol), and toluene (1 ml) at 140°C for 24 h, under N2 atmosphere. bIsolated yields.
Finally, we also briefly set out to evaluate the scope of aryl cyanides (Scheme 4). As expected, these reactions proceeded smoothly when 2-pyrazinylmethanol and pyridinylmethanols with benzoyl cyanides served as the substrates, leading the corresponding products 5a–5h in moderate to good yields (41%–80%). 3-furanmethanol and 3-thiophenemethanol could undergo the expected acylation with 2-methoxy-α-oxo-benzeneacetonitrile (2m) in moderate yields (5i–5j, 51%–60%). We were pleased to observe that α-oxo-1-naphthaleneacetonitrile was readily reacted with 1a in moderate yield (5k, 60%). It was satisfying to discover that the protocol was amenable for most benzoyl cyanides substrates, which is different from the work of Subaramannian et al. involving the single-electron transfer (SET) in the catalytic transformation (Subaramanian et al., 2020). This was probably because the nature of reaction mechanism of these two methods is distinct.
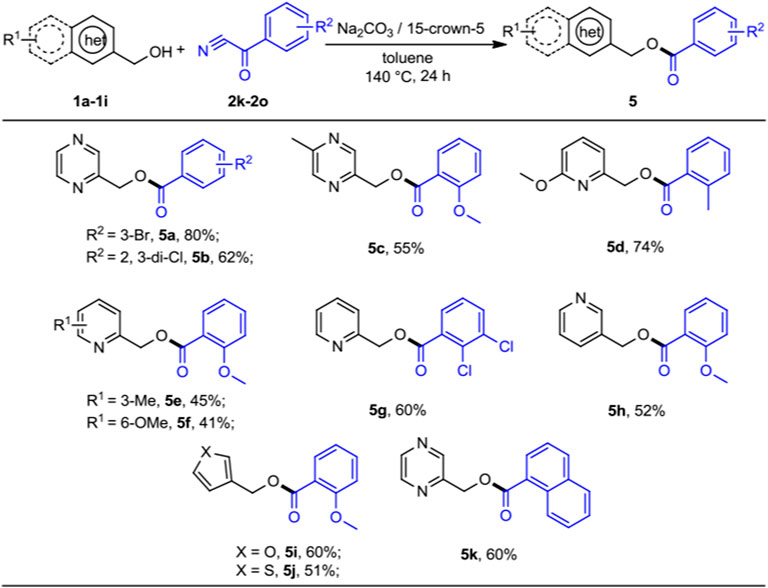
SCHEME 4. Substrate scope of benzoyl cyanides for the acylation reactions. a,bReaction conditions: aheteroaryl methanols 1 (0.5 mmol), benzoyl cyanides 2 (0.2 mmol), Na2CO3 (0.2 mmol), 15-crown-5 (0.2 mmol), and toluene (1 ml) at 140°C for 24 h, under N2 atmosphere. bIsolated yields.
The gram-scale reaction of 1a (16 mmol, 1.761 g) and 2k (6.4 mmol, 0.801 g) was carried out to demonstrate the practicability of this protocol, which could give the desired product 4c′ in 84% yield (Scheme 5). As shown in Scheme 6, radical trapping experiments were performed through the addition of TEMPO, BHT, p-benzoquinone, and 1,1-diphenylethylene to the reaction system in the standard conditions, and the formation of 3a was not suppressed. These results inferred that this transformation did not occur via a radical mechanism.
A tentative mechanism was proposed and is shown in Scheme 7 on the basis of the results presented above and previous reports (Xie et al., 2014; Kong et al., 2016). The reaction initiated with Na2CO3 associated with 15-crown-5 induced deprotonation of 2-pyrazinylmethanol 1a to form 2-pyrazinyl alkoxy anion A. Then anion A undergoes a nucleophilic attack to the carbonyl group of benzoylacetonitrile 2a and affords intermediate B. Moreover, by abstracting a proton from the in situ formed NaHCO3, the thermodynamic favorable C–C bond cleavage of B would provide the desired product 3a, in which the side product CH3CN is released.
Conclusion
In summary, Na2CO3/15-crown-5 couple-mediated direct acylation of N-heteroaryl methanols with acyl cyanides via C–C bond cleavage is reported. This new synthetic protocol proceeds under metal-free conditions and offers broad substrate scope in high efficiency. A variety of N-heteroaryl esters including pyrazines, pyridines, quinolines, furans, and thiophenes, which are key molecules in pharmaceuticals, natural products, dyes, or flavors, have thus been efficiently synthesized with good yields.
Data Availability Statement
The original contributions presented in the study are included in the article/Supplementary Material, Further inquiries can be directed to the corresponding author.
Author Contributions
ML and MZ contributed to the conception and design of the study. The synthetic work and data collection were carried out by FS, MW, and GZ. JH contributed to the article revision. All authors read and approved the submitted version.
Conflict of Interest
The authors declare that the research was conducted in the absence of any commercial or financial relationships that could be construed as a potential conflict of interest.
Publisher’s Note
All claims expressed in this article are solely those of the authors and do not necessarily represent those of their affiliated organizations, or those of the publisher, the editors, and the reviewers. Any product that may be evaluated in this article, or claim that may be made by its manufacturer, is not guaranteed or endorsed by the publisher.
Supplementary Material
The Supplementary Material for this article can be found online at: https://www.frontiersin.org/articles/10.3389/fchem.2021.822625/full#supplementary-material
References
Akhlaghinia, B., Safaei, E., and Larki, P. (2010). Chemoselective Protocol for O-Acylation with a New Catalyst. Trends Org. Chem. 14, 93–106. doi:10.1039/toc100814a
Armani, E., Amari, G., Rizzi, A., Fanti, R. D., Ghidini, E., Capaldi, C., et al. (2014). Novel Class of Benzoic Acid Ester Derivatives as Potent PDE4 Inhibitors for Inhaled Administration in the Treatment of Respiratory Diseases. J. Med. Chem. 57, 793–816. doi:10.1021/jm401549m
Arzumanyan, A. V. (2017). Iron-catalyzed C C Bond Activation/C O Bond Formation: Direct Conversion of Ketones to Esters. Tetrahedron Lett. 58, 4667–4671. doi:10.1016/j.tetlet.2017.10.068
Balalaie, S., Mahdidoust, M., and Eshaghi-Najafabadi, R. (2008). ChemInform Abstract: 2-1h-Benzotriazole-1-Yl-1,1,3,3-Tetramethyluronium Tetrafluoro Borate (TBTU) as an Efficient Coupling Reagent for the Esterification of Carboxylic Acids with Alcohols and Phenols at Room Temperature. ChemInform 39, 1141–1144. doi:10.1002/chin.200839075
Bayout, I., Bouzemi, N., Guo, N., Mao, X., Serra, S., Riva, S., et al. (2020). Natural Flavor Ester Synthesis Catalyzed by Lipases. Flavour Fragr J. 35, 209–218. doi:10.1002/ffj.3554
Bourne-Branchu, Y., Gosmini, C., and Danoun, G. (2017). Cobalt-Catalyzed Esterification of Amides. Chem. Eur. J. 23, 10043–10047. doi:10.1002/chem.201702608
Chen, X., Liu, M., Liu, Y., Chen, Y., Au, C.-T., and Yin, S.-F. (2018). Copper-Catalyzed Esterification of N -Heteroaryl Methanes by Oxidative Dehydrogenation. Asian J. Org. Chem. 7, 1825–1829. doi:10.1002/ajoc.201800421
Chen, Z., Wang, B., Zhang, J., Yu, W., Liu, Z., and Zhang, Y. (2015). Transition Metal-Catalyzed C-H Bond Functionalizations by the Use of Diverse Directing Groups. Org. Chem. Front. 2, 1107–1295. doi:10.1039/c5qo00004a
Chen, Z., Wen, X., Zheng, W., He, R., Chen, D., Cao, D., et al. (2020). Acyl Cyanides as Bifunctional Reagent: Application in Copper-Catalyzed Cyanoamidation and Cyanoesterification Reaction. J. Org. Chem. 85, 5691–5701. doi:10.1021/acs.joc.9b03500
Cheng, L., Sun, Y., Wang, W., Yao, C., and Li, T.-J. (2019). Ligand-Free Copper(I)-Catalyzed Benzylic Acyloxylation of 2-Alkylpyridines under Aerobic Conditions. J. Org. Chem. 84, 3074–3082. doi:10.1021/acs.joc.8b02794
Chun, S., and Chung, Y. K. (2017). Transition-Metal-Free Poly(thiazolium) Iodide/1,8-Diazabicyclo[5.4.0]undec-7-ene/Phenazine-Catalyzed Esterification of Aldehydes with Alcohols. Org. Lett. 19, 3787–3790. doi:10.1021/acs.orglett.7b01617
Deng, Y., Yang, T., Wang, H., Yang, C., Cheng, L., Yin, S.-F., et al. (2021). Recent Progress on Photocatalytic Synthesis of Ester Derivatives and Reaction Mechanisms. Top. Curr. Chem. (Z) 379, 42–94. doi:10.1007/s41061-021-00355-5
Dong, D.-Q., Zhang, H., and Wang, Z.-L. (2017). Synthesis of Benzyl Esters from the Commercially Available Alcohols Catalyzed by TBAI via C(sp3)-H Bond Functionalization. RSC Adv. 7, 3780–3782. doi:10.1039/C6RA26387A
Dong, J., Chen, X., Ji, F., Liu, L., Su, L., Mo, M., et al. (2021). Copper‐mediated Simple and Direct Aerobic Oxidative Esterification of Arylacetonitriles with Alcohols/phenols. Appl. Organomet. Chem. 35, 6073–6083. doi:10.1002/aoc.6073
Eisele, P., Bauder, M., Hsu, S. F., and Plietker, B. (2019). A Cyanide‐Free Synthesis of Acylcyanides through Ru‐Catalyzed C(sp 3 )‐H‐Oxidation of Benzylic Nitriles. ChemistryOpen 8, 689–691. doi:10.1002/open.201900130
Guo, C., Xu, C., Zhang, N.-N., Li, X.-j., Ge, Y.-q., and Diao, P.-h. (2018). Visible Light Promotes Decyanation Esterification Reaction of β-Ketonitriles with Dioxygen and Alcohols to α-Ketoesters. Synlett 29, 1065–1070. doi:10.1055/s-0036-1591943
Guo, S., Yu, J.-T., Dai, Q., Yang, H., and Cheng, J. (2014). The Bu4NI-Catalyzed Alfa-Acyloxylation of Ketones with Benzylic Alcohols. Chem. Commun. 50, 6240–6242. doi:10.1039/C4CC01652A
Hay, B. P., Rustad, J. R., and Hostetler, C. J. (1993). Quantitative Structure-Stability Relationship for Potassium Ion Complexation by crown Ethers. A Molecular Mechanics and Ab Initio Study. J. Am. Chem. Soc. 115, 11158–11164. doi:10.1021/ja00077a013
Hie, L., Baker, E. L., Anthony, S. M., Desrosiers, J.-N., Senanayake, C., and Garg, N. K. (2016). Nickel-Catalyzed Esterification of Aliphatic Amides. Angew. Chem. Int. Ed.Angew. Chem. 55128, 15129–15132. doi:10.1002/anie.201607856
Huang, X., Li, X., Zou, M., Song, S., Tang, C., Yuan, Y., et al. (2014). From Ketones to Esters by a Cu-Catalyzed Highly Selective C(CO)-C(alkyl) Bond Cleavage: Aerobic Oxidation and Oxygenation with Air. J. Am. Chem. Soc. 136, 14858–14865. doi:10.1021/ja5073004
Huang, Z., Huang, X., Li, B., Mou, C., Yang, S., Song, B.-A., et al. (2016). Access to P-Stereogenic Phosphinates via N-Heterocyclic Carbene-Catalyzed Desymmetrization of Bisphenols. J. Am. Chem. Soc. 138, 7524–7527. doi:10.1021/jacs.6b04624
Jiang, H., Chen, H., Wang, A., and Liu, X. (2010). Palladium-catalyzed Acetoxylation of Sp3 C-H Bonds Using Molecular Oxygen. Chem. Commun. 46, 7259–7261. doi:10.1039/c0cc00841a
Kong, W., Li, B., Xu, X., and Song, Q. (2016). Fe-Catalyzed Aerobic Oxidative C-CN Bond Cleavage of Arylacetonitriles Leading to Various Esters. J. Org. Chem. 81, 8436–8443. doi:10.1021/acs.joc.6b01594
Lai, M., Zhai, K., Cheng, C., Wu, Z., and Zhao, M. (2018). Direct Thiolation of Aza-Heteroaromatic N-Oxides with Disulfides via Copper-Catalyzed Regioselective C-H Bond Activation. Org. Chem. Front. 5, 2986–2991. doi:10.1039/C8QO00840J
Li, G., Lei, P., and Szostak, M. (2018). Transition-Metal-Free Esterification of Amides via Selective N-C Cleavage under Mild Conditions. Org. Lett. 20, 5622–5625. doi:10.1021/acs.orglett.8b02323
Li, G., and Szostak, M. (2020). Transition‐Metal‐Free Activation of Amides by N−C Bond Cleavage. Chem. Rec. 20, 649–659. doi:10.1002/tcr.201900072
Liotta, C. L., Harris, H. P., McDermott, M., Gonzalez, T., and Smith, K. (1974). Chemistry of "naked" Anions II. Reactions of the 18-crown-6 Complex of Potassium Acetate with Organic Substrates in Aprotic Organic Solvents. Tetrahedron Lett. 15, 2417–2420. doi:10.1016/S0040-4039(01)92273-7
Liu, B., Hu, F., and Shi, B.-F. (2015a). Recent Advances on Ester Synthesis via Transition-Metal Catalyzed C-H Functionalization. ACS Catal. 5, 1863–1881. doi:10.1021/acscatal.5b00050
Liu, C., Yuan, J., Gao, M., Tang, S., Li, W., Shi, R., et al. (2015b). Oxidative Coupling between Two Hydrocarbons: an Update of Recent C-H Functionalizations. Chem. Rev. 115, 12138–12204. doi:10.1021/cr500431s
Lu, P., Hou, T., Gu, X., and Li, P. (2015). Visible-Light-Promoted Conversion of Alkyl Benzyl Ether to Alkyl Ester or Alcohol via O-α-Sp3 C-H Cleavage. Org. Lett. 17, 1954–1957. doi:10.1021/acs.orglett.5b00663
Lutz, H. D., Jung, M., and Beckenkamp, K. (1988). Nature of the strong Basicity of Superbases, Unsolvated Hydroxide Ions? J. Mol. Struct. 175, 257–261. doi:10.1016/S0022-2860(98)80085-9
McMurray, L., O'Hara, F., and Gaunt, M. J. (2011). Recent Developments in Natural Product Synthesis Using Metal-Catalysed C-H Bond Functionalisation. Chem. Soc. Rev. 40, 1885–1898. doi:10.1039/c1cs15013h
Mukaiyama, T., Kikuchi, W., and Shintou, T. (2003). Preparation of Various Carboxylic Acid Esters from Bulky Alcohols and Carboxylic Acids by a New Type Oxidation-Reduction Condensation Using 2,6-Dimethyl-1,4-Benzoquinone. Chem. Lett. 32, 300–301. doi:10.1246/cl.2003.300
Rammurthy, B., Peraka, S., Vasu, A., Krishna Sai, G., Divya Rohini, Y., and Narender, N. (2021). Metal‐free Catalytic Esterification of Aryl Alkyl Ketones with Alcohols via Free‐radical Mediated C(sp 3 )−H Bond Oxygenation. Asian J. Org. Chem. 10, 594–601. doi:10.1002/ajoc.202000691
Reuter, C., Wienand, W., Hübner, G. M., Seel, C., and Vögtle, F. (1999). High-Yield Synthesis of Ester, Carbonate, and Acetal Rotaxanes by Anion Template Assistance and Their Hydrolytic Dethreading. Chem. Eur. J. 5, 2692–2697. doi:10.1002/(SICI)1521-3765(19990903)5:9<2692:AID-CHEM2692>3.0.CO;2-F
Roy, B. C., Ansari, I. A., Samim, S. A., and Kundu, S. (2019). Base‐Promoted α‐Alkylation of Arylacetonitriles with Alcohols. Chem. Asian J. 14, 2215–2219. doi:10.1002/asia.201900285
Subaramanian, M., Ramar, P. M., Rana, J., Gupta, V. K., and Balaraman, E. (2020). Catalytic Conversion of Ketones to Esters via C(O)-C Bond Cleavage under Transition-Metal Free Conditions. Chem. Commun. 56, 8143–8146. doi:10.1039/d0cc03312j
Tamaddon, F., Amrollahi, M. A., and Sharafat, L. (2005). A green Protocol for Chemoselective O-Acylation in the Presence of Zinc Oxide as a Heterogeneous, Reusable and Eco-Friendly Catalyst. Tetrahedron Lett. 46, 7841–7844. doi:10.1016/j.tetlet.2005.09.005
Tang, S., Yuan, J., Liu, C., and Lei, A. (2014). Direct Oxidative Esterification of Alcohols. Dalton Trans. 43, 13460–13470. doi:10.1039/c4dt01133c
Trotier-Faurion, A., Dézard, S., Taran, F., Valayannopoulos, V., de Lonlay, P., and Mabondzo, A. (2013). Synthesis and Biological Evaluation of New Creatine Fatty Esters Revealed Dodecyl Creatine Ester as a Promising Drug Candidate for the Treatment of the Creatine Transporter Deficiency. J. Med. Chem. 56, 5173–5181. doi:10.1021/jm400545n
Uyanik, M., Suzuki, D., Yasui, T., and Ishihara, K. (2011). In Situ Generated (Hypo)Iodite Catalysts for the Direct α-Oxyacylation of Carbonyl Compounds with Carboxylic Acids. Angew. Chem. Int. Ed. 50, 5331–5334. doi:10.1002/anie.201101522
Wang, C.-S., Roisnel, T., Dixneuf, P. H., and Soulé, J.-F. (2017). Synthesis of 2-Pyridinemethyl Ester Derivatives from Aldehydes and 2-Alkylheterocycle N-Oxides via Copper-Catalyzed Tandem Oxidative Coupling-Rearrangement. Org. Lett. 19, 6720–6723. doi:10.1021/acs.orglett.7b03446
Wencel-Delord, J., and Glorius, F. (2013). C-H Bond Activation Enables the Rapid Construction and Late-Stage Diversification of Functional Molecules. Nat. Chem 5, 369–375. doi:10.1038/nchem.1607
Xie, F., Yan, F., Chen, M., and Zhang, M. (2014). Base-catalyzed Retro-Claisen Condensation: a Convenient Esterification of Alcohols via C-C Bond Cleavage of Ketones to Afford Acylating Sources. RSC Adv. 4, 29502–29508. doi:10.1039/c4ra04618h
Xu, Y., Zhao, J., Liu, X., Zhang, C., Zhao, Z., Li, X., et al. (2022). Flavor Mystery of Chinese Traditional Fermented Baijiu: The Great Contribution of Ester Compounds. Food Chem. 369, 130920–130932. doi:10.1016/j.foodchem.2021.130920
Yan, F.-X., Zhang, M., Wang, X.-T., Xie, F., Chen, M.-M., and Jiang, H. (2014). Efficient Ruthenium-Catalyzed α-alkylation of Ketones Using Pyridyl Methanols. Tetrahedron 70, 1193–1198. doi:10.1016/j.tet.2013.12.065
Yasin, F. M., Boulos, R. A., Hong, B. Y., Cornejo, A., Iyer, K. S., Gao, L., et al. (2012). Microfluidic Size Selective Growth of Palladium Nano-Particles on Carbon Nano-Onions. Chem. Commun. 48, 10102–10206. doi:10.1039/c2cc35017c
Zeng, R., Sheng, H., Zhang, Y., Feng, Y., Chen, Z., Wang, J., et al. (2014). Heterobimetallic Dinuclear Lanthanide Alkoxide Complexes as Acid-Base Difunctional Catalysts for Transesterification. J. Org. Chem. 79, 9246–9252. doi:10.1021/jo5016536
Zhai, K., Lai, M., Wu, Z., Zhao, M., Jing, Y., and Liu, P. (2018). Synthesis and Initial thermal Behavior Investigation of 2-alkenyl Substituted Pyrazine N-Oxides. Catal. Commun. 116, 20–26. doi:10.1016/j.catcom.2018.07.017
Zhang, C., and Wang, Y. (2019). Chloride‐Induced Highly Active Au Catalyst for Methyl Esterification of Alcohols. Chin. J. Chem. 37, 249–254. doi:10.1002/cjoc.201800374
Keywords: N-heteroaryl esters, C-C bond cleavage, acyl cyanides, transition-metal free synthesis, N-heteroaryl methanols
Citation: Lai M, Su F, Hu J, Wang M, Zhao M and Zhang G (2022) Synthesis of N-Heteroarenemethyl Esters via C–C Bond Cleavage of Acyl Cyanides Under Transition Metal-Free Conditions. Front. Chem. 9:822625. doi: 10.3389/fchem.2021.822625
Received: 26 November 2021; Accepted: 15 December 2021;
Published: 26 January 2022.
Edited by:
Florent Allais, AgroParisTech Institut des Sciences et Industries du Vivant et de L’Environnement, FranceReviewed by:
Muhammet Uyanik, Nagoya University, JapanMichal Szostak, The State University of New Jersey, United States
Copyright © 2022 Lai, Su, Hu, Wang, Zhao and Zhang. This is an open-access article distributed under the terms of the Creative Commons Attribution License (CC BY). The use, distribution or reproduction in other forums is permitted, provided the original author(s) and the copyright owner(s) are credited and that the original publication in this journal is cited, in accordance with accepted academic practice. No use, distribution or reproduction is permitted which does not comply with these terms.
*Correspondence: Mingqin Zhao, zhaomingqin@126.com
†These authors have contributed equally to this work