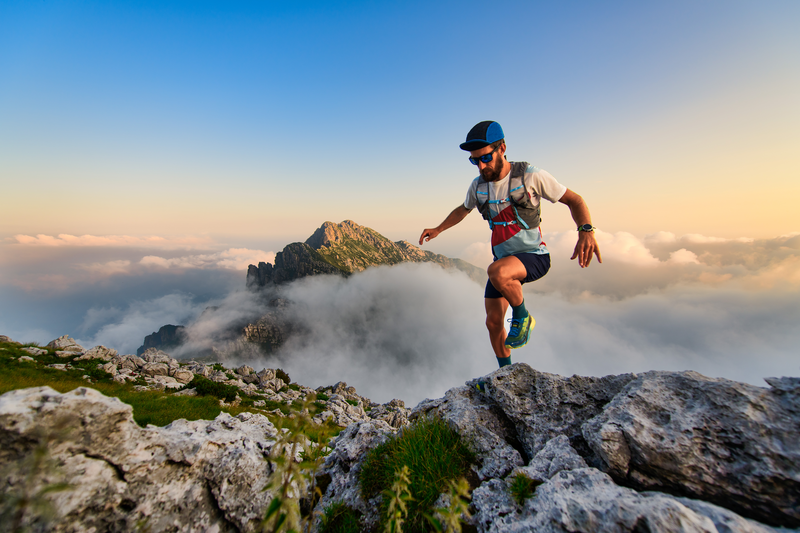
95% of researchers rate our articles as excellent or good
Learn more about the work of our research integrity team to safeguard the quality of each article we publish.
Find out more
MINI REVIEW article
Front. Chem. , 23 December 2021
Sec. Nanoscience
Volume 9 - 2021 | https://doi.org/10.3389/fchem.2021.813973
This article is part of the Research Topic Cutting-Edge Nanotechnologies in Bacterial Infection Control View all 7 articles
Vaginal candidiasis (VC) is a common disease of women and the main pathogen is Candida albicans (C. albicans). C. albicans infection incidence especially its drug resistance have become a global health threat due to the existence of C. albicans biofilms and the low bioavailability of traditional antifungal drugs. In recent years, nanomaterials have made great progresses in the field of antifungal applications. Some researchers have treated fungal infections with inorganic nanoparticles, represented by silver nanoparticles (AgNPs) with antifungal properties. Liposomes, polymeric nanoparticles, metal-organic frameworks (MOFs), and covalent organic frameworks (COFs) were also used to improve the bioavailability of antifungal drugs. Herein, we briefly introduced the recent developments on using above nanomaterials to combat C. albicans in antifungal applications.
Vaginal candidiasis (VC) is the second common mucosal infection of the vagina, with around 80–95% cases caused by Candida species, especially Candida albicans (C. albicans). About 75% of women occur at least once in their lifetime, and at least twice in more than half of patients. Around 5–8% of patients will develop recurrent vulvovaginal candidiasis (at least 4 times per year) (Anderson et al., 2004; Sobel, 2007). 80–95% of these pathogens are C. albicans.
C. albicans is an opportunistic pathogen that colonizes the mucous membranes of the skin and gastrointestinal tract in 30–50% of normal healthy individuals. C. albicans only becomes pathogenic when the local or systemic defense mechanism is damaged. The most common infection sites are oropharynx, esophagus and vagina (Johal et al., 2016). C. albicans has three forms: spore, pseudohyphae and hyphae (Molero et al., 1998). The morphological transformation from spore to mycelium also means that C. albicans has transformed from symbiotic state to pathogenic state, while pseudohyphae is the transitional form of both (Talapko et al., 2021). The hypha invades host tissues through active penetration and induces endocytosis. In this process, hypha synthesizes and secretes various proteins and form biofilms. Actually, most C. albicans infections are associated with the formation of biofilms on the host or abiotic surfaces (Maza et al., 2017). Biofilm is a highly structured and complex microbial community whose formation consists of several successive stages (Figure 1). In the first stage, free C. albicans adhere to the substrate, forming the basal layer of the biofilm. Then albicans cells form elongated projections that continue to grow into filamentous hyphal form. This process is known as the cell proliferation and filamentation, and is also the sign of the beginning of biofilms forming. Extracellular polysaccharide matrix accumulates during the mature stage. Finally, unattached cells disperse between tissues and begin the formation of new biofilms (Talapko et al., 2021). The three-dimensional communities of these surface-attached and tightly packed fungi complicate treatment and can lead to drug resistance and persistent fungal infections (Li et al., 2018). Biofilms can achieve immune escape of fungal cells in the host (Nett, 2014; Vera-González and Shukla, 2020) and secrete a dense extracellular polymer, which can serve as a physical barrier during antifungal therapy, making most antifungal drugs difficult to pass through this barrier and less effective at their target position (Singh et al., 2018; Vera-González and Shukla, 2020; Talapko et al., 2021). In addition, studies have reported that drug resistance may also be related to the thickness of the bacterial cell wall. It has been observed that the thickness of the biofilm is twice that of free cells (Nett et al., 2007; Lima et al., 2019).
FIGURE 1. Biofilm Formation: (A). Candida albicans adhere to the substrate; (B,C). The cells proliferated and formed mycelia while extracellular polysaccharide matrix accumulated; (D). The beginning of new biofilm formation.
VC treatments based on standard antifungal agents such as azole, polyene and echinocandin include oral or vaginal topical administration. Transvaginal agents are absorbed through three main pathways: transcellular absorption, paracellular absorption and active transport. Transcellular and paracellular absorption rely on a intra-/extra-cellular concentration-dependent gradient and tight connections between cells; while active transport depends on vesicles or receptors on the surfaces of cells. The absorption efficiency of drugs is affected by many factors, such as the thickness of the vaginal epithelium, the viscosity of vaginal secretions, pH value and the solubility of drugs (Johal et al., 2016). In addition, drug stability, bioavailability and compliance of patients are also essentisal factors of antifungal therapy. While oral drugs are often difficult to transport to target position because of their poor solubility and bioavailability, resulting in an increased incidence of adverse reactions due to lack of drug selectivity. The common adverse reactions include gastrointestinal symptoms, nephrotoxicity and hepatotoxicity (Paul and Sharma, 2010). And oral administration often fails to produce satisfactory results for severe fungal infections (Johal et al., 2016).
Nanomaterials have developed rapidly in recent years. The application of nanomaterials in the medical field is also popular direction. Nanoparticles have the potential to carry, stabilize and protect therapeutic payloads, penetrate extracellular polymeric substances (EPS), target fungal cells, making nanoparticle therapeutics for the treatment of candida biofilms become a promising strategy (Figure 2). Here we will introduce some advanced developments in antifungal therapy by nanomaterials.
FIGURE 2. Nanoparticles have anti-biofilm effect: (A). Traditional antifungal drugs have difficulty passing through biofilms: (B). Nanoparticles have anti-biofilm effect and can improve the bioavailability of antifungal drugs.
Many inorganic substances have been known to have antibacterial effects. However, due to the lack of targeting and difficult to control the release of inorganic substances, they accumulate in normal tissues and produce toxic and side effects. Inorganic nanoparticles not only have antibacterial effects, but also have the advantages of non-toxicity, hydrophilic, biocompatibility and high stability, which have been gradually paid attention to in the fields of medical imaging diagnosis and drug delivery (Spadari et al., 2017).
Silver nanoparticles (AgNPs), the diameter of which is less than 100 nm, with higher antibacterial activity and larger relative surface areas, show antibacterial activity against the target microbial biofilm at low concentrations (Monteiro et al., 2011; Takamiya et al., 2016). AgNPs attack the membranes of yeast cells and disturb the membrane lipid bilayer, forming pores that lead to leakage of ions and other substances, dissipating the membrane potential (Kim et al., 2009). Hwang et al. found AgNPs kill fungal cells by inducing the accumulation of reactive oxygen radicals, especially hydroxyl radicals (Hwang et al., 2012). Lara used transmission electron microscopy to find that the fungal cell wall was infiltrated and the outer cell wall of the structural layer was destroyed after the treatment of silver nanoparticles. This indicates that the anti-biofilm effect of AgNPs is achieved via cell wall disruption (Lara et al., 2015). In addition, AgNPs can also accumulate outside fungal cells and release silver ions through the interaction between cell components and ionic silver, inducing cell death (Vazquez-Muñoz et al., 2014). Takamiya et al. combined AgNPs with acrylic resin (a material with strong bending strength and antifungal effect) and found that the effect of antifungal biofilm was increased, while the bending strength of acrylic resin itself as usual. In addition, 60 days after the recombinant material was implanted into the subcutaneous tissue of mice, there was no significant difference in inflammatory response compared with control group, suggesting that the AgNPs were biocompatible (Takamiya et al., 2021).
Bismuth is another metallic element, whose compounds have been used in medicine for more than two centuries (Vazquez-Munoz et al., 2020a). Recently several bismuth compounds have been found to have antibacterial and antifungal activities (Ferraz et al., 2013). Some reports indicated that bismuth nanoparticles (BiNPs) display promising anti-microbial activity on bacteria, fungi, and protozoan. However there are few articles about antibacterial activity of nano-bismuth due to their difficulties in synthesizing and low stability. Most recently an American group has described a method for the fast, facile, and inexpensive synthesis of PVP-coated bismuth nanoparticles (PVP-BiNPs), which can be used even in a non-specialist laboratory (Vazquez-Munoz et al., 2020b). PVP-BiNPs are more stable than the bismuth (III) ions and the bismuth-BAL complexes. PVP-BiNPs have been proved to have strong antibacterial activity against both pathogenic Staphylococcus aureus (S. aureus) and conditionally pathogenic yeast C. albicans, whether under plankton or biofilm growth conditions (Vazquez-Munoz et al., 2020a). However, the antibacterial mechanism of BiNPs is unclear and remains to be further studied.
Zinc oxide nanoparticles (ZnO-NPs) have been widely used in many fields due to their optical transparency, electrical conductivity, piezoelectric properties, non-toxicity, wide applicability, low cost and good stability. What’s more, they can also be used to fight bacteria and fungi. ZnO-NPs can be successfully synthesized by homogeneous precipitation method. ZnO-NPs with small particle size and large specific surface area had strong antifungal activity. The antibacterial activity of ZnO-NPs against C. albicans was detected by disc diffusion susceptibility method. It was observed that the inhibition band increased with the increase of ZnO-NPs concentration (Sharma and Ghose, 2015).
Inorganic nanoparticles have achieved satisfactory results in antifungal treatment. However, their high requirements for preparation conditions, high production costs, poor stability and other shortcomings hinder the process of large-scale clinical use. In addition, the antibacterial mechanism of inorganic nanoparticles remains to be further studied.
Liposomes (Garg and K. Goyal, 2014) are mainly composed of non-ionic lipid molecules such as phospholipids and cholesterol, which could form thin lipid films or lipid cakes. In the process of agitation, hydration of lipid will unlock and self-close, forming large multilamellar vesicles. The existence of lipid bilayer and aqueous medium make liposomes both lipophilic and hydrophilic, which means they can be used to encapsulate and deliver both hydrophilic and lipophilic drug molecules. What’s more, liposomes have good solubility and stable structure, which can reduce the phagocytosis of macrophages on drugs, maintain blood drug concentration, reduce the influence of microenvironment on drugs, promote the intracellular transmission of drugs (Garg and K. Goyal, 2014). Encapsulation of antifungals into liposome nanoparticles, which may improve the transport and action efficiency of antifungal drugs, has become one of the research hotspots in recent years.
Liposomal amphotericin B is the first commercially formulated nanoparticle system for antifungal drugs. Host toxicity of amphotericin B was significantly reduced in liposomal amphotericin B (Olson et al., 2008). The ultra-deformable amphotericin B liposomes containing surfactants have stronger epidermal permeability (Perez et al., 2016). VERA-GONZÁLEZET et al. successfully encapsulated anidulafungin (an echinocandin antifungal drug) into liposomes. These liposome formulations can inhibit planktonic C. albicans growth with a similar minimum inhibitory concentration (MIC) to anidulafungin, but localize to the candida cells more quickly and perform better in penetrating fungal biofilms compared with free anidulafungin. What’s more, in comparison with free agents, using liposomes at the same concentration 2 days before infection significantly improved survival rate (Vera-González et al., 2020).
Actually, liposomes can simultaneously transport two or more drugs to the targeted position. For example, C. Carbone et al. have created a liposome formulation which combined with solid lipid nanoparticles, clotrimazole (CLZ) and alphalipolic acid (ALA), marked as CLZ-ALA-loaded SLN. Mean size of nanoparticles were below 150 nm and exhibited slight negative or highly positive zeta potential values, so that the nanoparticles can remain stable in the presence of cationic resin. Furthermore, they confirmed the formation of SLN was able to release drug slowly but steadily in vitro. This research indicates that the dual-delivery SLN developed with ALA protective antifungal agents may be a promising strategy for the treatment of candida (Carbone et al., 2020).
Despite the promising prospect of liposome nanotechnology, its low solubility, short half life, poor stability, allergic reaction, high production cost and low production efficiency are still problems that need to be solved urgently (Garg and K. Goyal, 2014).
Polymeric nanoparticles (polymeric NPs) are composed of organic polymers. According to their structural forms, they can be roughly classified as nanocapsules and nanospheres. Nanocapsules consist of a core that dissolves the drug and a polymer shell that controls its release. Nanospheres are a continuous network of polymers in which drugs are stored inside or adsorbed to the surface (Zielinska et al., 2020). Chitosan and poly (lactic-co-glycolic acid) (PLGA) are two common materials used to prepare polymer nanoparticles.
Chitosan is a kind of biocompatible, biodegradable, selective non-toxic cationic polysaccharide (Ing et al., 2012; Wimardani et al., 2012), which has certain antifungal activity (Dhillon et al., 2014). These advantages make it a popular material the preparation of nanoparticles in recent years. Ferulic acid (FA) and its derivatives have been found to have antibiofilm potential against C. albicans. However, the applicability of FA is limited by its low permeability and instability. Additionally, FA is a plant phenolic compound, which is unable to distinguish between diseased and healthy cells, and may lead to undesirable side effects. Coating them with nanoparticles in polymers allow them to accumulate at the specific targets, preventing harmful effects on normal tissues (Park et al., 2010). Panwar et al. composed FA encapsulated chitosan nanoparticles (FA-CSNPs) exhibited the positive zeta potential, which allowed them to bind to negatively charged fungal membranes and thus destroed their membrane integrity, resulting in intracellular material leakage and biofilm inhibition (Panwar et al., 2016). Chitosan also binded with trace elements to inhibit the normal growth of fungi (Roller and Covill, 1999). In addition, some scholars proposed that chitosan can penetrate fungal cell wall and bind to its DNA, thus inhibiting the synthesis of mRNA and the production of essential fungal proteins and enzymes (Kong et al., 2010).
Curcumin is one of the main components extracted from turmeric, a perennial flowering plant in the rhizome of Zingiberaceae. Recently, curcumin loaded chitosan nanoparticles (CSNP-CUR) have been synthesized and used as antibacterial agents (Samrot et al., 2018). CSNP-CUR has been confirmed to have stronger antimicrobial activity and biofilm resistance than free curcumin. Furthermore, Ma et al. found that CSNP-CUR also showed excellent antibacterial, antifungal and antibiofilm effects on the polymicrobial biofilm, indicating that CSNP-CUR may be a novel strategy for polymicrobial biofilm related infections (Ma et al., 2020).
In addition, other chitosan based nanoparticles with antifungal activity and biofilm potential have been reported, such as chitosan-pentasodium tripolyphosphte (CS-TPP) nanoparticles (Ing et al., 2012) and chitosan based zinc oxide nanoparticles (Dhillon et al., 2014).
Poly (lactic-co-glycolic acid) (PLGA) is another popular material for the preparation of polymeric nanoparticles. Different from chitosan, PLGA is a synthetic polymer organic material, and its advantages are similar to those of chitosan (Cartiera et al., 2009).
Ketoconazole is a common antifungal drug in clinical practice. However, due to its low bioavailability, conventional doses of ketoconazole are difficult to achieve satisfactory antifungal activity, while large doses are likely to cause severe allergic and skin reactions. Sadozai et al. prepared ketoconazole-loaded PLGA nanoparticles (Keto-PLGA NPs) by emulsion/solvent evaporation method. This formulation succeeded in enhancing the solubility of drug. Under experimental conditions, they found that the sustained release of ketoconazole from the nanoparticles resulted in a weaker fungal inhibition than the same dose of free ketoconazole. However, when Keto-PLGA NPs interacted with AgNPs, the largest growth inhibition region is produced, indicating a strong synergistic effect between two materials (Sadozai et al., 2020), which is consistent with previous reports of synergistic effects between AgNPs and other azole-based antimycotic drugs (Sun et al., 2016).
Currently, some polymeric NPs have been successfully prepared, but current research tends to focus on their physical and chemical properties. We still lack data on their ecotoxicology and how the drug relates to the polymeric NPs.
Metal-organic frameworks (MOFs) is a kind of organic-inorganic hybrid crystalline materials, containing positively charged metal ions and organic linking molecules arranged in order. MOFs structures have many good properties, such as ultra-high surface areas, porosities, biocompatibility, biodegradability and stability in water and biological media, which make MOFs a new hotspot in the field of nanocomposites (MOFs (Metal-Organic Frameworks, 2021)). Different from MOFs, COFs is another synthetic material, holding the network of organic ligands covalently bonded to each other. The advantages of COFs are somewhat similar to those of MOFs. Both MOFs and COFs are of permanent porosity and highly ordered structures. Their high degree of controllability in structure, synthesis and function make them the new favorites in the field of organic materials (Geng et al., 2020).
Researches about MOFs and COFs in C. albicans inhibitory applications are developing. Su et al. designed and synthesized voriconazole-Inbuilt zinc 2-methylimidazolates frameworks (V-ZIF) recently. Voriconazole is expected to be embedded in the MOF, and the former can only be responsive-released under the pH of 5 environment of the C. albicans biofilm. What’s more, they found that V-ZIFs provide better antifungal effect and antibiofilm effect than free voriconazole. This could be explained by V-ZIFs binding to C. albicans via electrostatic double-layer attraction, resulting in additional damage to the latter cell wall. The potential cytotoxicity of V-ZIPs and free drugs was compared using human embryonic kidney 293T (HEK 293T) cells and it was found that V-ZIPs yielded significantly less loss of cytotoxicity. V-ZIFs also could accelerate wound healing without obvious side effects in open wounds infected with C. albicans in infectious mice models (Su et al., 2020).
The applications of MOFs and COFs in drug delivery systems for antifungal purpose are still in its infancy, more progresses are expected in the future.
In addition to the antifungal effect of nanoparticles themselves and the use of nanomaterials as a carrier to improve the bioavailability of traditional antifungal drugs, some researchers have used nanomaterials to regulate macrophage-related immune processes to enhance the killing of C. albicans by the body itself. The conventional chemotherapeutic imatinib was encapsulated in dual functional mannosylated chitosan oligosaccharides which can decrease M2 macrophage population by inhibiting the STAT6 phosphorylation pathway and induce M1 macrophage polarization. This process will significantly increase the degree of resistance to C. albicans (Gao et al., 2020).
Photodynamic antimicrobial chemotherapy (PACT) is a new method fighting against bacteria and fungus, which mainly utilizes the absorbtion of specific wavelength of light activating photosensitizers to generate reactive oxygen species (ROS) such as singlet oxygen. Subsequently, ROS can induce a series of reactions and physical effects to achieve the purpose of killing microorganisms. PACT has been proved to be effective against C. albicans recently (Ikono et al., 2019). Khan et al. found that gold nanoparticles (AuNPs) could significantly promote the PACT mediated by methylene blue (MB), an classic photosensitizer, and increase the inhibitory rate of C. albicans biofilm from 81.9 to 95.4% (Khan et al., 2012).
In recent years, C. albicans infection incidence and its drug resistance increased year by year, which make it become a global problem almost. Studies and literature on its infections and treatments are also emerging.
Nanomaterials have become a hot topic in the field of drug delivery systems due to their ability to carry, stabilize and protect therapeutic payloads and target cell binding. The antifungal effect of nanomaterials, such as inorganic nanoparticles, liposomes, polymeric nanoparticles, metal-organic frameworks (MOFs) and covalent organic frameworks (COFs) have been well verified in laboratory conditions. But there is still a long way to go before these materials can be put into clinical use. First of all, due to the high equipment and technology requirements and high cost of producing nanoparticles, it is difficult to achieve large-scale production at present, which limits its development in clinical application to some extent. Secondly, the specific mechanism, biological effects and toxic and side effects of nanoparticles are not clear. We still lack data on whether the nanoparticles themselves and their metabolites are truly environmentally and biologically benign. Moreover, Unlike other skin or digestive systems, the microenvironment of the female reproductive system changes periodically under the influence of reproductive hormones. Whether nanomaterials can adapt to such changes and give full play to their antifungal effects remains to be discussed. Many more laboratory and clinical trials are needed to resolve all these questions, but the promise of nanomaterials as antifungal treatments is unquestionable. In addition, currently, the nanocarriers themselves and their combinations with antifungal drugs or other nanomaterials are still limited. It may also be a promising research direction to find more and more effective combinations as possible.
BL and LP contributed equally to the work and share the first authorship, HZ and LX wrote sections of the manuscript, XW and JS collected literature, XY and YQ directed the writing.
This work was financially supported by the Natural Science Foundation of Zhejiang Province-THZ2 No. LY21H160058 (XY), the Chinese Medicine Science and Technology Project of Zhejiang Province-EGCG No. 2020ZB144 (XY), the Scientific Research Project of Wenzhou Science and Technology Bureau No. Y20190014 (XY) and National Natural Science Foundation of China (NSFC), grant number 22005019 (YQ).
The authors declare that the research was conducted in the absence of any commercial or financial relationships that could be construed as a potential conflict of interest.
All claims expressed in this article are solely those of the authors and do not necessarily represent those of their affiliated organizations, or those of the publisher, the editors and the reviewers. Any product that may be evaluated in this article, or claim that may be made by its manufacturer, is not guaranteed or endorsed by the publisher.
We would like to acknowledge the First Affiliated Hospital of Wenzhou Medical University.
Anderson, M. R., Klink, K., and Cohrssen, A. (2004). Evaluation of Vaginal Complaints. JAMA 291(11), 1368–1379. doi:10.1001/jama.291.11.1368
Carbone, C., Fuochi, V., Zielińska, A., Musumeci, T., Souto, E. B., Bonaccorso, A., et al. (2020). Dual-drugs Delivery in Solid Lipid Nanoparticles for the Treatment of Candida Albicans Mycosis. Colloids Surf. B: Biointerfaces 186, 110705. doi:10.1016/j.colsurfb.2019.110705
Cartiera, M. S., Johnson, K. M., Rajendran, V., Caplan, M. J., and Saltzman, W. M. (2009). The Uptake and Intracellular Fate of PLGA Nanoparticles in Epithelial Cells. Biomaterials 30 (14), 2790–2798. doi:10.1016/j.biomaterials.2009.01.057
Dhillon, G. S., Kaur, S., and Brar, S. K. (2014). Facile Fabrication and Characterization of Chitosan-Based Zinc Oxide Nanoparticles and Evaluation of Their Antimicrobial and Antibiofilm Activity. Int. Nano Lett. 4 (2), 107. doi:10.1007/s40089-014-0107-6
Ferraz, K. S. O., Reis, D. C., Da Silva, J. G., Souza-Fagundes, E. M., Baran, E. J., and Beraldo, H. (2013). Investigation on the Bioactivities of Clioquinol and its Bismuth(III) and platinum(II,IV) Complexes. Polyhedron 63, 28–35. doi:10.1016/j.poly.2013.07.008
Gao, Q., Zhang, J., Chen, C., Chen, M., Sun, P., Du, W., et al. (2020). In Situ Mannosylated Nanotrinity-Mediated Macrophage Remodeling Combats Candida Albicans Infection. ACS Nano 14 (4), 3980–3990. doi:10.1021/acsnano.9b07896
Garg, T., and K. Goyal, A. (2014). Liposomes: Targeted and Controlled Delivery System. Ddl 4, 62–71. doi:10.2174/22103031113036660015
Geng, K., He, T., Liu, R., Dalapati, S., Tan, K. T., Li, Z., et al. (2020). Covalent Organic Frameworks: Design, Synthesis, and Functions. Chem. Rev. 120 (16), 8814–8933. doi:10.1021/acs.chemrev.9b00550
Hwang, I.-s., Lee, J., Hwang, J. H., Kim, K.-J., and Lee, D. G. (2012). Silver Nanoparticles Induce Apoptotic Cell Death in Candida Albicans through the Increase of Hydroxyl Radicals. FEBS J. 279 (7), 1327–1338. doi:10.1111/j.1742-4658.2012.08527.x
Ikono, R., Vibriani, A., Wibowo, I., Saputro, K. E., Muliawan, W., Bachtiar, B. M., et al. (2019). Nanochitosan Antimicrobial Activity against Streptococcus Mutans and Candida Albicans Dual-Species Biofilms. BMC Res. Notes 12 (1), 383. doi:10.1186/s13104-019-4422-x
Ing, L. Y., Zin, N. M., Sarwar, A., and Katas, H. (2012). Antifungal Activity of Chitosan Nanoparticles and Correlation with Their Physical Properties. Int. J. Biomater. 2012, 632698. doi:10.1155/2012/632698
Johal, H. S., Garg, T., Rath, G., and Goyal, A. K. (2016). Advanced Topical Drug Delivery System for the Management of Vaginal Candidiasis. Drug Deliv. 23 (2), 550–563. doi:10.3109/10717544.2014.928760
Khan, A., Khan, fnm., Azam, fnm., and Alam, fnm. (2012). Gold Nanoparticles Enhance Methylene Blue– Induced Photodynamic Therapy: a Novel Therapeutic Approach to Inhibit Candida Albicans Biofilm. Ijn 7, 3245–57. doi:10.2147/ijn.s31219
Kim, K.-J., Sung, W. S., Suh, B. K., Moon, S.-K., Choi, J.-S., Kim, J. G., et al. (2009). Antifungal Activity and Mode of Action of Silver Nano-Particles on Candida Albicans. Biometals 22 (2), 235–242. doi:10.1007/s10534-008-9159-2
Kong, M., Chen, X. G., Xing, K., and Park, H. J. (2010). Antimicrobial Properties of Chitosan and Mode of Action: A State of the Art Review. Int. J. Food Microbiol. 144 (1), 51–63. doi:10.1016/j.ijfoodmicro.2010.09.012
Lara, H. H., Romero-Urbina, D. G., Pierce, C., Lopez-Ribot, J. L., Arellano-Jiménez, M. J., and Jose-Yacaman, M. (2015). Effect of Silver Nanoparticles on Candida Albicans Biofilms: n Ultrastructural Study. J. Nanobiotechnol 13, 91. doi:10.1186/s12951-015-0147-8
Li, W.-S., Chen, Y.-C., Kuo, S.-F., Chen, F.-J., and Lee, C.-H. (2018). The Impact of Biofilm Formation on the Persistence of Candidemia. Front. Microbiol. 9, 1196. doi:10.3389/fmicb.2018.01196
Lima, S. L., Colombo, A. L., and de Almeida Junior, J. N. (2019). Fungal Cell Wall: Emerging Antifungals and Drug Resistance. Front. Microbiol. 10, 2573. doi:10.3389/fmicb.2019.02573
Ma, S., Moser, D., Han, F., Leonhard, M., Schneider-Stickler, B., and Tan, Y. (2020). Preparation and Antibiofilm Studies of Curcumin Loaded Chitosan Nanoparticles against Polymicrobial Biofilms of Candida Albicans and Staphylococcus aureus. Carbohydr. Polym. 241, 116254. doi:10.1016/j.carbpol.2020.116254
Maza, P. K., Bonfim-Melo, A., Padovan, A. C. B., Mortara, R. A., Orikaza, C. M., Ramos, L. M. D., et al. (2017). Candida Albicans: The Ability to Invade Epithelial Cells and Survive under Oxidative Stress Is Unlinked to Hyphal Length. Front. Microbiol. 8, 1235. doi:10.3389/fmicb.2017.01235
MOFs (Metal-Organic Frameworks) (2021). Available from: https://metal-organic-frameworks.com/.
Molero, G., Díez-Orejas, R., Navarro-García, F., Monteoliva, L., Pla, J., Gil, C., et al. (1998). Candida Albicans: Genetics, Dimorphism and Pathogenicity. Int. Microbiol. : official J. Spanish Soc. Microbiol. 1, 95–106.
Monteiro, D. R., Gorup, L. F., Silva, S., Negri, M., de Camargo, E. R., Oliveira, R., et al. (2011). Silver Colloidal Nanoparticles: Antifungal Effect against Adhered Cells and Biofilms ofCandida albicansandCandida Glabrata. Biofouling 27 (7), 711–719. doi:10.1080/08927014.2011.599101
Nett, J. E. (2014). Future Directions for Anti-biofilm Therapeutics targetingCandida. Expert Rev. Anti-infective Ther. 12 (3), 375–382. doi:10.1586/14787210.2014.885838
Nett, J., Lincoln, L., Marchillo, K., Massey, R., Holoyda, K., Hoff, B., et al. (2007). Putative Role of β-1,3 Glucans in Candida Albicans Biofilm Resistance. Antimicrob. Agents Chemother. 51 (2), 510–520. doi:10.1128/aac.01056-06
Olson, J. A., Adler-Moore, J. P., Jensen, G. M., Schwartz, J., Dignani, M. C., and Proffitt, R. T. (2008). Comparison of the Physicochemical, Antifungal, and Toxic Properties of Two Liposomal Amphotericin B Products. Antimicrob. Agents Chemother. 52 (1), 259–268. doi:10.1128/aac.00870-07
Panwar, R., Pemmaraju, S. C., Sharma, A. K., and Pruthi, V. (2016). Efficacy of Ferulic Acid Encapsulated Chitosan Nanoparticles against Candida Albicans Biofilm. Microb. Pathogenesis 95, 21–31. doi:10.1016/j.micpath.2016.02.007
Park, J. H., Saravanakumar, G., Kim, K., and Kwon, I. C. (2010). Targeted Delivery of Low Molecular Drugs Using Chitosan and its Derivatives. Adv. Drug Deliv. Rev. 62 (1), 28–41. doi:10.1016/j.addr.2009.10.003
Paul, W., and Sharma, C. P. (2010). Inorganic Nanoparticles for Targeted Drug Delivery. Biointegration Med. Implant Mater., 204–235. doi:10.1533/9781845699802.2.204
Perez, A. P., Altube, M. J., Schilrreff, P., Apezteguia, G., Celes, F. S., Zacchino, S., et al. (2016). Topical Amphotericin B in Ultradeformable Liposomes: Formulation, Skin Penetration Study, Antifungal and Antileishmanial Activity In Vitro. Colloids Surf. B: Biointerfaces 139, 190–198. doi:10.1016/j.colsurfb.2015.12.003
Roller, S., and Covill, N. (1999). The Antifungal Properties of Chitosan in Laboratory media and Apple Juice. Int. J. Food Microbiol. 47 (1), 67–77. doi:10.1016/s0168-1605(99)00006-9
Sadozai, S. K., Khan, S. A., Karim, N., Becker, D., Steinbrück, N., Gier, S., et al. (2020). Ketoconazole-loaded PLGA Nanoparticles and Their Synergism against Candida Albicans when Combined with Silver Nanoparticles. J. Drug Deliv. Sci. Tech. 56 (5), 101574. doi:10.1016/j.jddst.2020.101574
Samrot, A. V., Burman, U., Philip, S. A., N, S., and Chandrasekaran, K. (2018). Synthesis of Curcumin Loaded Polymeric Nanoparticles from Crab Shell Derived Chitosan for Drug Delivery. Inform. Med. Unlocked 10, 159–182. doi:10.1016/j.imu.2017.12.010
Sharma, R. K., and Ghose, R. (2015). Synthesis of Zinc Oxide Nanoparticles by Homogeneous Precipitation Method and its Application in Antifungal Activity against Candida Albicans. Ceramics Int. 41 (1), 967–975. doi:10.1016/j.ceramint.2014.09.016
Singh, R., Kumari, A., Kaur, K., Sethi, P., and Chakrabarti, A. (2018). Relevance of Antifungal Penetration in Biofilm-Associated Resistance of Candida Albicans and Non-albicans Candida Species. J. Med. Microbiol. 67 (7), 922–926. doi:10.1099/jmm.0.000757
Sobel, J. D. (2007). Vulvovaginal Candidosis. The Lancet 369 (9577), 1961–1971. doi:10.1016/s0140-6736(07)60917-9
Spadari, C. d. C., Lopes, L. B., and Ishida, K. (2017). Potential Use of Alginate-Based Carriers as Antifungal Delivery System. Front. Microbiol. 8, 97. doi:10.3389/fmicb.2017.00097
Su, L. Z., Li, Y., Liu, Y., Ma, R., Liu, Y., Huang, F., et al. (2020). Antifungal‐Inbuilt Metal–Organic‐Frameworks Eradicate Candida Albicans Biofilms. Adv. Funct. Mater. 30 (28), 202000537. doi:10.1002/adfm.202000537
Sun, L., Liao, K., Li, Y., Zhao, L., Liang, S., Guo, D., et al. (2016). Synergy between Polyvinylpyrrolidone-Coated Silver Nanoparticles and Azole Antifungal against Drug-Resistant Candida Albicans. j nanosci nanotechnol 16, 2325–2335. doi:10.1166/jnn.2016.10934
Takamiya, A. S., Monteiro, D. R., Bernabé, D. G., Gorup, L. F., Camargo, E. R., Gomes-Filho, J. E., et al. (2016). In Vitro and In Vivo Toxicity Evaluation of Colloidal Silver Nanoparticles Used in Endodontic Treatments. J. Endodontics 42 (6), 953–960. doi:10.1016/j.joen.2016.03.014
Takamiya, A. S., Monteiro, D. R., Gorup, L. F., Silva, E. A., de Camargo, E. R., Gomes-Filho, J. E., et al. (2021). Biocompatible Silver Nanoparticles Incorporated in Acrylic Resin for Dental Application Inhibit Candida Albicans Biofilm. Mater. Sci. Eng. C 118, 111341. doi:10.1016/j.msec.2020.111341
Talapko, J., Juzbašić, M., Matijević, T., Pustijanac, E., Bekić, S., Kotris, I., et al. (2021). Candida Albicans-The Virulence Factors and Clinical Manifestations of Infection. J. Fungi (Basel) 7 (2), 79. doi:10.3390/jof7020079
Vazquez-Munoz, R., Arellano-Jimenez, M. J., and Lopez-Ribot, J. L. (2020). Bismuth Nanoparticles Obtained by a Facile Synthesis Method Exhibit Antimicrobial Activity against Staphylococcus aureus and Candida Albicans. BMC Biomed. Eng. 2, 11. doi:10.1186/s42490-020-00044-2
Vazquez-Munoz, R., Arellano-Jimenez, M. J., and Lopez-Ribot, J. L. (2020). Fast, Facile Synthesis Method for BAL-Mediated PVP-Bismuth Nanoparticles. MethodsX 7, 100894. doi:10.1016/j.mex.2020.100894
Vazquez-Muñoz, R., Avalos-Borja, M., and Castro-Longoria, E. (2014). Ultrastructural Analysis of Candida Albicans when Exposed to Silver Nanoparticles. PLoS One 9 (10), e108876. doi:10.1371/journal.pone.0108876
Vera-González, N., Bailey-Hytholt, C. M., Langlois, L., de Camargo Ribeiro, F., de Souza Santos, E. L., Junqueira, J. C., et al. (2020). Anidulafungin Liposome Nanoparticles Exhibit Antifungal Activity against Planktonic and Biofilm Candida Albicans. J. Biomed. Mater. Res. A. 108 (11), 2263–2276. doi:10.1002/jbm.a.36984
Vera-González, N., and Shukla, A. (2020). Advances in Biomaterials for the Prevention and Disruption of Candida Biofilms. Front. Microbiol. 11, 538602. doi:10.3389/fmicb.2020.538602
Wimardani, Y. S., Suniarti, D, Freisleben, H.-J., Wanandi, S. I., and Ikeda, M.-A., (2012). Cytotoxic Effects of Chitosan against Oral Cancer Cell Lines Is Molecular-Weightdependent and Cell-type-specific. Int. J. Oral Res. 3, e1. doi:10.2334/josnusd.56.119
Keywords: vaginal candidiasis, Candida albicans, nanomaterials, antifungal applications, biofilms
Citation: Li B, Pan L, Zhang H, Xie L, Wang X, Shou J, Qi Y and Yan X (2021) Recent Developments on Using Nanomaterials to Combat Candida albicans. Front. Chem. 9:813973. doi: 10.3389/fchem.2021.813973
Received: 12 November 2021; Accepted: 02 December 2021;
Published: 23 December 2021.
Edited by:
Fan Huang, China Academy of Chinese Medical Sciences, ChinaReviewed by:
Ruifang Gao, University of Groningen, NetherlandsCopyright © 2021 Li, Pan, Zhang, Xie, Wang, Shou, Qi and Yan. This is an open-access article distributed under the terms of the Creative Commons Attribution License (CC BY). The use, distribution or reproduction in other forums is permitted, provided the original author(s) and the copyright owner(s) are credited and that the original publication in this journal is cited, in accordance with accepted academic practice. No use, distribution or reproduction is permitted which does not comply with these terms.
*Correspondence: Xiaojian Yan, eXhqYmV0dGVyMjAxNkBob3RtYWlsLmNvbQ==; Yu Qi, cWl5dUB1Y2FzLmFjLmNu
†These authors have contributed equally to this work and share first authorship
Disclaimer: All claims expressed in this article are solely those of the authors and do not necessarily represent those of their affiliated organizations, or those of the publisher, the editors and the reviewers. Any product that may be evaluated in this article or claim that may be made by its manufacturer is not guaranteed or endorsed by the publisher.
Research integrity at Frontiers
Learn more about the work of our research integrity team to safeguard the quality of each article we publish.