- 1College of Biology and Environmental Science, Jishou University, Jishou, China
- 2School of Medicine, Jishou University, Jishou, China
- 3School of Pharmaceutical Sciences, Jishou University, Jishou, China
- 4Tujia Medicine Research Center in Hunan (Jishou University), Jishou, China
The bioassay-guided phytochemical study of an ethnic medicinal plant Aspidopterys obcorda ta Hemsl. var. obcordata results in the isolation of eight new polyoxypregnane derivatives, named aspidatasides A–H (1–8), along with ten known analogs (9–18). The series polyoxypregnane derivatives were screened for their cytoxic activity against HL-60 cells, and compound 2 showed the highest potency with an IC50 8.03 μM. Preliminary structure–activity relationship studies displayed that the sugar chain and double bond could notably impact their biological activity.
1 Introduction
Natural products, as major chemical resources, make up significant agents in modern drug discovery, and play an important role in treating and preventing diseases with novel molecular mechanisms over the last 200 years (Hanson, 2017). The latest report shows that half of the new drugs in the market come from natural products or structural modifications based on a natural chemical framework (Newman and Cragg, 2020).
The polyoxypregnanes and their derivatives as valuable therapeutic agents are naturally occurring C21 steroidal skeleton or substituted by oligosaccharide chain, benzoyl, acylated tigloyl ester groups, as well as an extra epoxide ring (Bai et al., 2007; Zheng et al., 2014), which exhibited diverse biological and medicinal activities, such as anti-inflammatory effect, immune-suppressive effect; chondro-protective effect; and antifungal, antioxidant, antifertility, anti-AchE, and anti-HIV activities (Abe et al., 2000; Niranjan et al., 2002; Plaza et al., 2004; Deng et al., 2005; Li et al., 2007; Sanyacharernkul et al., 2009; Ni and Ye, 2010; Wang et al., 2010; Itthiarbha et al., 2012; Pang et al., 2015; Zhang et al., 2015; Gu and Hao, 2016; Wang et al., 2016; Pang et al., 2017; Zhan et al., 2019). In particular, many of them have shown prominent anticarcinogenic or cancer inhibitory activities with great research and development potential, and received widespread attention by pharmacologists (Plaza et al., 2005; Panda et al., 2006; Bai et al., 2009; Shen and Zhang, 2010; Zhang et al., 2010; Xue et al., 2012; Sun et al., 2014; Yao et al., 2014; Zhang et al., 2015; Liu et al., 2018; Song et al., 2018). Natural monomeric polyoxypregnanes have been recognized as characteristic constituents in Asclepiadaceae plants, and there have been effective reports on the use of Marsdenia tenacissima (Asclepiadaceae) as a raw material in the treatment of leukemia, gastric carcinoma, liver cancer, prostate cancer, and lung cancer in China, notably (Zhao et al., 2007; Tong et al., 2015; Ge et al., 2019; Wang et al., 2019; Yu, 2019; Pu et al., 2020; Zhu et al., 2020). However, polyoxypregnanes are rarely isolated from the other families. Meanwhile, their antitumor mechanism and the structure–activity relationship of polyoxypregnane derivatives from the plant are not clearly demonstrated.
A. obcordata is a woody liana herb, mainly distributed in sunny and rainfall areas in Xishuangbanna, Yunnan Province. As a “Dai Medicine,” A. obcordata has been used frequently and commonly in the Dai Minority Hospital of Xishuangbanna, Southwest China, for the treatment of various diseases, such as cystitis, chronic nephritis, urinary tract infections, rheumatic bone pain, and postpartum body deficiency (Chen and Funston, 1997; Li et al., 2011). Although the effectiveness of the medicinal plant has been verified in clinic, there are few related studies about its material basis (Li et al., 2016; Li et al., 2019). In a continuous attempt to search for structurally diverse cytotoxicity, interesting C21 steroids from A. obcordata, eight new polyoxypregnane derivatives, named aspidatasides A–H (1–8), together with ten known analogs (9–18) (Figure 1) were obtained from the 95% EtOH extract of plant’s vines, which exhibited moderate antitumor effects, especially its antihuman myeloid leukemia (HL-60) activity. Herein, the isolated procedures, structural elucidation of the new compounds, as well as their inhibitory activity evaluation against HL-60 were reported in order to understand the structure–activity relationship (SAR).
2 Results and Discussion
2.1 Isolation and Structure Elucidation
Compound 1 was isolated as a white amorphous powder. Its IR spectrum exhibited absorptions for the groups of hydroxyl (3,382 cm−1) and carbonyl (1,717 cm−1). The molecular formula was established as C42H64O15 based on the molecular ion peak at m/z [M + Na]+ 831.4139 in the HR–ESI–MS (calculated for 831.4143 C42H64O15Na). In the 1H NMR spectrum (Table 1), signals for three angular methyl protons at δH 1.29 (3H, s, CH3-18), 1.32 (3H, s, CH3-19), and 2.16 (3H, s, CH3-21), and one olefinic proton signal at δH 5.36 (H-6) in conjunction with three carbons at δC 216.5, 140.6, and 119.5 (Table 2) suggested the basic skeleton of pregen-5-en-20-one (Wang et al., 2010; Zhang et al., 2015; Wang et al., 2019). The proton signals at δH 1.81 (3H, s, 11-OAc) and 6.73 (1H, q, J = 7.2 Hz, Tig-H-3), 1.57 (1H, d, J = 7.2 Hz, Tig-H-4), and 1.43 (3H, s, Tig-H-5), together with carbon resonances at δC 21.0, 172.9 (11-OAc), and δC 168.4, 129.9, 140.7, 14.8, and 12.3 (Tig-C), suggested the presence of acetoxyl function and tigloyl unit in the molecular structure. Two anomeric signals at δH 4.65 (1H, d, J = 9.6 Hz) and 4.72 (1H, d, J = 7.8 Hz) indicated the existence of two sugar moieties in compound 1. Two methoxyls at δH 3.39 (s) and 3.60 (s) were attributed to the sugar moiety. The IR and 1H and 13C-NMR spectra data identified that compound 1 is a polyoxypregnane glycoside derivative.
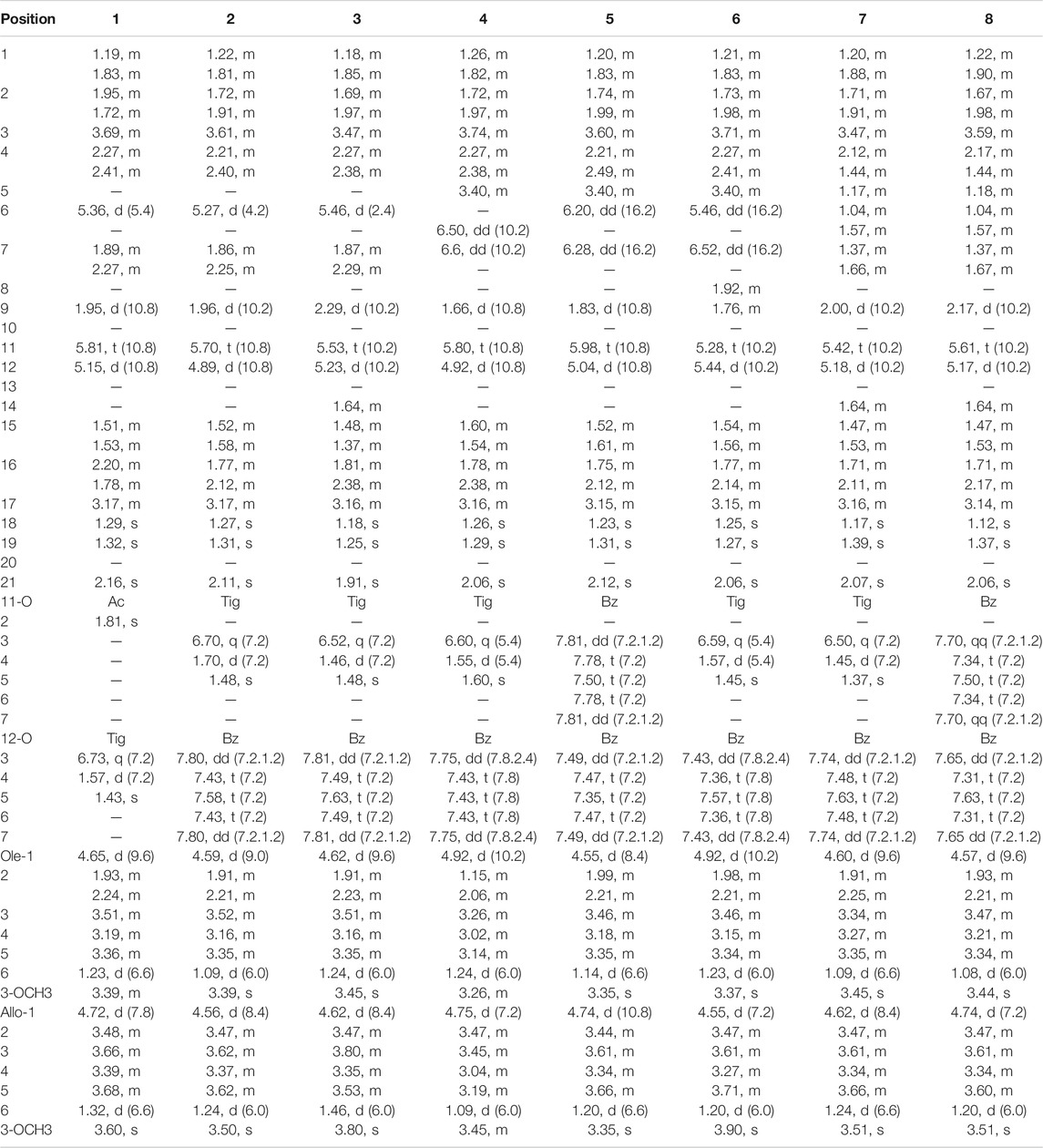
TABLE 1. 1H NMR spectroscopic data (600 MHz, 1 in MeOD and 2–8 in DMSO) for compounds 1–8 (δH in ppm, J in Hz).
All assignments of proton signals were assigned by its HSQC spectrum (Supporting Information, Supplementary Figure S3). The connectivities of compound 1 were deduced mainly by 1H-1H COSY and HMBC spectra (Figure 2). The correlations among H-1/H-2/H-3/H-4, H-6/H-7, H-9/H-11/H-12, and H-15/H-16/H-17 were found in the 1H-1H COSY spectrum. The position of acetoxyl and tigloyl groups was determined at C-11 and C-12, respectively, as the HMBC correlations between δH5.81 (1H, t, J = 10.8 Hz, H-11) and δC 172.9 (11-OAc), δH5.15 (1H, d, J = 10.8 Hz, H-12), and δC 168.4 (Tig-C-1). Two hydroxyl substituents were located at C-8 and C-14, respectively, based on their chemical shifts (δC 76.8 and 86.5) together with the molecular formula above. The existence of D-oleandrose (Ole) and 6-deoxy-3-O-methyl-D-allopyranose (Allo) units was confirmed by co-TLC and further followed by gas chromatography in comparison with standard monosaccharides. The sugar sequence and linkage position were established by the HMBC correlations of δH 4.72 (H-1 of Allo) to δC 84.2 (C-4 of Ole) and δH 4.65 (H-1 of Ole) to δC 79.3 (C-3 of aglycone), as shown in Figure 2.
Meanwhile, the NOE correlations of H-3/H-1α, H3-19/H-1β, H-12/H-9, and H-12/H-17 indicated the α-orientations of H-3/12/17. The 3J coupling constant (J = 10.8 Hz) between H-11 and H-12 also confirmed their anti-periplanar relationship. Meanwhile, the β configurations of the two sugars were established based on the large coupling constants (each 3J1, 2 > 7.0 Hz). Thus, the structure of 1 was established and named aspidataside A.
Compound 2 was obtained as a white amorphous powder. Its molecular formula was established as C47H66O15 according to the HR–ESI–MS spectrum at m/z [M + Na]+ 893.4305 (calculated for C47H66O15Na, 893.4299). An analysis of the 1H and 13CNMR data (Table 1; Table 2) displayed that the structure of 2 was similar to that of 1, except for the OAc group in 1 was absent in 2, extra signals of a benzoyl group at δH7.80 (2H, dd, J = 7.2, 1.2 Hz), 7.43 (2H, t, J = 7.2 Hz), 7.58 (1H, t, J = 7.2 Hz), and δC 165.9, 128.0, 129.2, 129.0, and 129.3 were found in 2. In the HMBC spectrum, the correlations from δH 5.70 (H-11) to the carbonyl signal at δC 166.9 (Tig-1), and from δH 4.89 (H-12) to δC 165.9 indicated that the acetoxyl group at C-11 in 1 was replaced by the tigloyl group in 2, while the tigloyl group at C-12 was changed to the benzoyl group. The absolute configuration of 2 was established by the analysis of the NOESY and CD spectra. Therefore, compound 2 was identified and named aspidataside B.
Compound 3 was suggested to possess the molecular formula C47H66O14, based on the m/z 877.4348 [M + Na]+ (calculated for C47H66O14Na, 877.4350), in the HR–ESI–MS, which was 16 amu less than that of 2. In the NMR spectra of 3 and 2 (Table 1; Table 2), the structure of 3 corresponded to 2, except a hydroxyl group absent at C-14. The up-field chemical shift of C-14 at δC 48.0 in 3 compared with C-14 (δC 84.5) in 2 together with the molecular weight of 3 in the HR–ESI–MS exhibited the disappearance of hydroxyl function at C-14 in 3. The difference was fully confirmed by the 2D NMR experiments. With the analysis of NOESY data (Supporting Information, Supplementary Figure S23), compound 3 was determined as shown and named aspidataside C.
Compound 4, with the molecular formula of C47H66O15 determined by the HR–ESI–MS ion at m/z [M + H]+ 871.4461 (calculated for C47H66O15Na, 871.4480), was an isomer of 2. NMR data analysis between 4 and 2 suggested that they had almost identical structures except for the location of double bond in the B-ring portion. The double bond located at C-6/C-7 was confirmed by the correlations from δH 3.40 (m, H-5) to δC 55.2 (C-5), δH 6.50 (dd, J = 10.2 Hz, H-6) to δC 131.1 (C-7), and δH 6.60 (dd, J = 10.2 Hz, H-7) to δC 129.4 (C-7) in the HSQC spectrum, and correlations from H-6 to C-5 and C-8 in the HMBC experiment. By the combined use of 1H-1H COSY and NOESY experiments (Supporting Information, Supplementary Figures S31, S32), the structure of 4 was finally elucidated and named aspidataside D.
Compound 5 with the molecular formula of C49H64O15 was determined by the HR–ESI–MS (m/z 893.4305, calcd for C49H64O15Na [M + H]+, 893.4323) and had the similar structure as 4 based on comparison of their NMR data (Table 1; Table 2). The differences displayed that the tigloyl group in 4 was replaced by a benzoyl unit in 5. In the 13C NMR data, the typical signals at δC 166.1, 133.6, 128.8, 128.5, 130.5, 164.9, 129.6, 128.2, 129.1, and 133.2, and the absence signals at δC 14.6 and 12.1 supported the differences above. The additional benzoyl function was linked to C-12, based on the correlations of the HMBC spectrum. As a result, the structure of 5 was illustrated and named aspidataside E.
Compound 6 exhibited its molecular ion peak at m/z 877.3334 [M + Na]+, corresponding to the molecular formula of C47H66O14. The 1H NMR and 13C NMR (APT) data were strongly related to those of 4 (Table 1; Table 2). The difference was that the signal at C-8 (δC 77.8) in 4 was changed to δC 30.8 in 6. Considering the loosening of 16 units in molecular weight of 6, it was deduced that the hydroxyl group at C-8 in 4 was missed in 6, which was fully supported by the 2D NMR spectra (Supporting Information, Supplementary Figures S47–S50). Taken together with the NOESY data, compound 6 was established and named aspidataside F.
Compound 7 was given a molecular formula as C47H68O14 based on the HR–ESI–MS ion peak at m/z 875.4196 (calcd for C47H68O14Na [M + Na]+, 875.4207). Its NMR data were almost identical to those of 3, except for one less degree of unsaturation, which suggested the disappearance of one double bond. In the 13CNMR data (Table 2), the carbon signals at δC 74.4 (C-3), 36.6 (C-4), 56.2 (C-5), 26.3 (C-6), 36.6(C-7), and 18.3 (C-19) indicated that there was no double bond between C-5 and C-6 in 7. In the HMBC spectrum, the correlations from δH 1.17 (1H, m, H-5) to δC 26.3 (C-6) and 36.6(C-7) confirmed the difference. As a result, compound 7 was a ductile product of compound 3. Therefore, compound 7 was finally established and named aspidataside G.
Compound 8 was determined as C49H66O14 according to the HR–ESI–MS at m/z 905.4664 (calcd for C49H66O14Na [M + Na]+, 905.4750). The 1H NMR and 13C APT spectra displayed that 8 was analogous to 7 (Table 1; Table 2), except for the tigloyl group at C-11 in 7 was substituted by a benzoyl group (δC 165.4, 129.3, 128.9, 128.5, and 133.3) in 8. The 2D NMR of HMBC experiments fully confirmed the difference (Supporting Information, Supplementary Figures S65–S68). Together with its NOESY data, the structure of compound 8 was fully identified and named aspidataside H.
So far, the skeleton connections of A, B, C, and D rings in all pregnanes were trans/trans/cis types, and the CH3-18, CH3-19, 8-H, and 14-H configurations in all the structures were β-oriented (Liu et al., 2017; Pang et al., 2017; Li et al., 2019). Considering the identical biosyntenic relationship, and the comparison of the experimental and calculated CD data (Supporting Information, Supplementary Figures S49–S56), the absolute configurations of the chiral carbons in the pregnane skeleton were determined to be 3S, 8S, 9S, 10R, 11S, 12S, 13S, 14R, and 17S.
The known polyoxypregnanes were identified as sinomarinoside E (9) (Chen et al., 1999), deacylmetaplexigein (10) (Chen et al., 1999), incisagenin (11) (Chen et al., 1999), drevogenin P (12) (Niranjan et al., 2002), obcordata G (13) (Li et al., 2019), obcordata H (14) (Li et al., 2019), obcordata I (15) (Li et al., 2019), sarcostin (16) (Ma et al., 2017), caretroside A (17) (Halim and Khalil, 1996), and 3-O-[6-deoxy-3-O-methyl-β-allopyanosyl (1→4)-β-oleandropyranosyl]-5,6-dihydro gen-11α,12β-di-O-tigloyl-17β-marsdenin (18) (Liu et al., 2017), based on the comparison of the observed spectroscopic data with those reported in the literature.
2.2 Inhibitory Effects of Compounds 1–18 on Human HL-60 Cell Line
The cytotoxic activity of the isolates (1–18) against HL-60 human promyelocytic acute leukemia cell in vitro was evaluated using the MTT method with the positive control doxorubicin IC50 3.87 μM, and the results are shown in Table 3. Compounds 2 and 9 exhibited potent activities with IC50 values of 8.03 ± 0.35 and 9.25 ± 0.45 μM, respectively. Compounds 1 and 3 displayed moderate activity with IC50 values ranging from 10.53 to 13.27 μM, and approximately two-fold more active than the compounds 7–8, 13–15, 17, and 18, and about three-fold more active comparing with the compounds 4–6, 10, and 11. Compounds 12 and 16 showed week cytotoxic activity against anti–HL-60 cell (IC50 > 50 μM).
2.3 Structure–Activity Relationships
The structure–activity relations of polyoxypregnane derivatives were described, as shown in Figure 3. It was found that all the isolates exhibited moderate inhibitory activity against HL-60 cell lines, which may be due to their unique pregen-5-en-20-one skeleton. It was reported that pregnane, as a component of steroids, plays an important role in regulating the metabolism of life. However, the presence of sugar moieties in the side chain also influenced the cytotoxic activity. Compounds 10–12, and 16 without sugar chain on the hydroxyl at C-3 displayed weak activity (39.0 < IC50 < 60.0 μM) when comparing with possessing oligosaccharides located at C-3, such as compounds 1–8, 9, 13–15, 17, and 18 with IC50 values ranging from 8.03 to 32.80 μM. These results indicated that the presence of sugar moiety at C-3 maybe improving the solubility of structures and could significantly enhance the cytotoxic activity, and played important roles in the structure.
Four (1–3, and 9, 8.03 < IC50 < 13.27 μM) compounds with a double bond between C-5 and C-6 showed the best potency among the 14 isolated constituents with sugar side chain. Compounds 7–8, 13–15, and 17–18 (21.68 < IC50 < 29.45 μM, vs. 1–3, and 9) characterizing without a double bond in skeleton exhibited moderate cytotoxic activity. In addition, the cytotoxic activities were obviously decreased due to a double bond at C-6/C-7, such as compounds 4–6 with the IC50 values from 30.72 to 32.80 μM comparing with 1–3 and 9. Thus, it is likely that the presence and location of double bond was important to the activity in this type of compounds. Furthermore, substituent groups, including O-Bz, O-Ac, and O-Tig, located at C-11 and C-12 were related with antitumor activity. More diverse modifications at C-11 and C-12 are warranted. These findings suggested that the sugar chain, the location of double bond, and polyester substitution in the C-11 and C-12 can substantially contribute to improve their cytotoxic activity.
In summary, the most promising compound, that is, compound 2, exerted significant cytotoxicity against the tested cancerous cell, and might be considered as a potential candidate for drug development and worthy of more detailed studies.
3 Conclusion
In conclusion, chemical investigation of the stems of A. obcordata has led to the discovery of eight new polyoxypregnanes (1–8) and ten known derivatives (9–18). In the in vitro assays, compounds 4–8 and 10–18 showed weak activity against HL-60 cells, while compounds 1–3 and 9 displayed strong inhibitory effects toward HL-60. Especially, compound 2 exhibited the best inhibition of HL-60 with an IC50 value of 8.03 μM, which was likely due to its hydrophilic sugar chains and bulky hydrophobic aliphatic chains linked with the complex conjugated system by SAR analysis. Nevertheless, the antitumor mechanism of action of compound 2 as anti–HL-60 agent is necessary for further studies.
4 Experimental
4.1 General Experimental Procedures
Optical rotations were measured with a Perkin-Elmer 341 digital polarimeter (PerkinElmer, Norwalk, CT, United States). UV and IR spectra were obtained on Shimadzu UV2550 and FTIR-8400S spectrometers (Shimadzu, Kyoto, Japan), respectively. CD spectra were obtained using a JASCO J-815 spectropolarimeter (Tokyo, Japan). NMR spectra were obtained using a Bruker AV III 600 NMR spectrometer with chemical shift values presented as δ values with TMS as the internal standard (Bruker, Billerica, German). HR–ESI–MS were performed on an LTQ-Orbitrap XL spectrometer (Thermo Fisher Scientific, Boston, MA, United States). Column chromatography (CC) was performed using silica gel (100–200 and 200–300 mesh, Qingdao Marine Chemical Plant, Qingdao, China). TLC analyses were carried out on silica gel GF254 precoated plates (Zhi Fu Huang Wu PilotPlant of Silica Gel Development, Yantai, China) with detection accomplished by spraying with 5% H2SO4 followed by heating at 100°C. Preparative HPLC was performed on a Lumtech K-1001 analytic LC equipped with two K-501 pumps, a K-2600 UV detector, and a YMC Pack C18 semi-preparative column (250 mm × 10 mm, i. d., 5 μM, YMC Co. Ltd., Japan), and eluted with CH3OH–H2O at a flow rate of 2 ml/min. All solvents used were of analytical grade (Beijing Chemical Works).
4.2 Plant Material
The vines of A. obcordata were collected in September 2016 from Jinghong, Yunnan Province, People’s Republic of China and identified by Prof. Dai-Gui Zhang, at the School of Biological Resources and Environmental Science, Jishou University, where a voucher specimen (JS161248) was deposited.
4.3 Extraction and Isolation
The dried and powdered vines of A. obcordata (4.0 kg) were extracted with 95% EtOH (20 L) three times (each time for 1 h). The solvents were filtrated and evaporated under reduced pressure to give the total extract (249.0 g), and dissolved successively with water (1.5 L), in the order, petroleum ether (MSO), dichloromethane (DCM), ethyl acetate (EtOAc), and n-butanol (NBA) to re-extract the water solution of the crude extract, and then have yielded different fractions. EtOAc fraction was selected for further separation due to its better inhibitory effect against HL-60 cells.
The EtOAc fraction (112.0 g) was subjected to CC over silica gel (100–200 mesh, 10 × 150 cm), eluting with a gradient of CH2Cl2–MeOH (from 1:0 to 0:1), and obtained twelve fractions, A–L. Fraction A (7.8 g) was subjected to chromatography using ODS MPLC elution with MeOH–H2O (30:70, 70:30, 90:10, and 100:0; v/v), to yield four fractions (Fr. A1–4), and Fr. A2 (0.5280 g) was separated through semi-preparative HPLC using a mobile phase of MeOH–H2O (70:30, v/v) to afford 12 (14.6 mg, Rt 23.5 min) and 16 (13.8 mg, Rt 28.8 min). Compounds 10 (17.2 mg, Rt 20.4) and 11 (13.2 mg, Rt 25.6) were isolated from Fr. A3 (0.2468 g) purified with MeOH–H2O (75:25, v/v). Fraction D (10.4 g) was subjected over ODS column chromatography elution with MeOH–H2O in a gradient manner (30:70, 50:50, 70:30, 80:20, and 100:0; v/v) to give five sub-fractions (Fr. D1–D5). Fr. D2 (0.348 g) was purified by HPLC with an isocratic of 55% MeOH–H2O on a YMC C18 column to get compounds 1 (8.5 mg, Rt 13.1 min), 2 (9.8 mg, Rt 16.3 min), 6 (10.4 mg, Rt 21.6 min), and 9 (7.5 mg, Rt 28.4 min). Compounds 3 (8.6 mg, Rt 18.2 min), 4 (7.5 mg, Rt 22.4 min), and 5 (12.2 mg, Rt 27.8 min) were yielded from Fr. D3 (0.41 g) separated by semi-preparative HPLC with MeOH–H2O (50:50, v/v). Similarly, Fr. E (5.8 g) was isolated through ODS MPLC elution with MeOH–H2O (30:70, 50:50, 70:30, 90:10; 100:0, v/v) to give Fr. E1–5, and Fr. E2 (0.55 g) was prepared by semi-preparative HPLC elution with MeOH–H2O (55:45, v/v), to obtain compounds 7 (14.5 mg, Rt 18.2 min), 8 (10.6 mg, Rt 22.3 min), 13 (8.2 mg, Rt 28.5 min), and 18 (14.8 mg, Rt 31.5 min). Compounds 14 (15.4 mg, Rt 17.5 min), 15 (7.8 mg, Rt 21.5 min), and 17 (13.5 mg, Rt 27.8 min) were yielded from Fr. E3 (0.3550 g) separated by semi-preparative HPLC with MeOH–H2O (50:50, v/v).
4.4 New Compounds
4.4.1 Aspidataside A
C42H64O15, white amorphous powder; [α]20D+35o (c 0.15, MeOH); UV λmax (MeOH) nm (log ε): 225 and 272; IR (KBr) νmax cm−1: 3,423, 1,660; HR–ESI–MS m/z 831.4139 (calculated for 831.4143 C42H64NaO15). 1H-NMR (MeOD, 600 MHz) and 13C-NMR (MeOD, 150 MHz) (see Table 1; Table 2).
4.4.2 Aspidataside B
C47H66O15, white amorphous powder; [α]20D+42.5o (c 0.12, MeOH); UV λmax (MeOH) nm (log ε): 272; IR (KBr) νmax cm−1: 3,350, 1,665, 1,605, 1,525; HR–ESI–MS m/z [M + Na]+ 893.4305 (calculated for C47H66NaO15, 893.4299). 1H-NMR (MeOD, 600 MHz) and 13C-NMR (DMSO, 150 MHz) (see Table 1; Table 2).
4.4.3 Aspidataside C
C47H66O14, white amorphous powder; [α]20D+40.2o (c 0.15, MeOH); UV λmax (MeOH) nm (log ε): 275; IR (KBr) νmax cm−1: 3,318, 1,680, 1,600, 1,524; HR–ESI–MS m/z 877.4348 [M + Na]+ (calculated for C47H66NaO14, 877.4350). 1H-NMR (MeOD, 600 MHz) and 13C-NMR (DMSO, 150 MHz) (see Table 1 and Table 2).
4.4.4 Aspidataside D
C47H66O15, white amorphous powder; [α]20D+52.5o (c 0.12, MeOH); UV λmax (MeOH) nm (log ε): 274; IR (KBr) νmax cm−1: 3,320, 1,675, 1,604, 1,525; HR–ESI–MS m/z 871.4461 [M + H]+ (calculated for C47H66NaO15, 871.4480). 1H-NMR (MeOD, 600 MHz) and 13C-NMR (DMSO, 150 MHz) see Table 1 and Table 2.
4.4.5 Aspidataside E
C49H64O15, white amorphous powder; [α]20D+44.8o (c 0.10, MeOH); UV λmax (MeOH) nm (log ε): 275; IR (KBr) νmax cm−1: 3,315, 1,678, 1,605, 1,520; HR–ESI–MS m/z 893.4305 [M + H]+ (calculated for C49H64NaO15, 893.4323). 1H-NMR (MeOD, 600 MHz) and 13C-NMR (DMSO, 150 MHz) see Table 1 and Table 2.
4.4.6 Aspidataside F
C47H66O14, white amorphous powder; [α]20D+50.3o (c 0.15, MeOH); UV λmax (MeOH) nm (log ε): 272; IR (KBr) νmax cm−1: 3,324, 1,692, 1,600, 1,524; HR–ESI–MS m/z877.3334 [M + Na]+ (calculated for C47H66NaO14, 877.3350). 1H-NMR (MeOD, 600 MHz) and 13C-NMR (DMSO, 150 MHz) (see Table 1 and Table 2).
4.4.7 Aspidataside G
C47H68O14, white amorphous powder; [α]20D+60.4o (c 0.15, MeOH); UV λmax (MeOH) nm (log ε): 275; IR (KBr) νmax cm−1: 3,384, 1,689, 1,610, 1,532; HR–ESI–MS m/z 875.4196 [M + Na]+ (calculated for C47H68NaO14, 875.4207). 1H-NMR (MeOD, 600 MHz) and 13C-NMR (DMSO, 150 MHz) (see Table 1 and Table 2).
4.4.8 Aspidataside H
C49H66O14, white amorphous powder; [α]20D+45.2o (c 0.15, MeOH); UV λmax (MeOH) nm (log ε): 275; IR (KBr) νmax cm−1: 3,372, 1,674, 1,538; HR–ESI–MS m/z 905.4664 [M + Na]+ (calculated for C49H66NaO14, 905.4750). 1H-NMR (MeOD, 600 MHz) and 13C-NMR (DMSO, 150 MHz) (see Table 1 and Table 2).
Acid hydrolysis of compounds was accomplished by the procedure described previously (Wang et al., 2019).
4.6 In vitro Cytotoxicity Bioassay
Compounds 1–18 were assessed for cytotoxicity against human cancer cell line HL-60. This used the MTT method as described in the previously published literature (Halim and Khalil, 1996). Briefly, cells were grown in DMEM supplied with 10% fetal bovine serum and cultured at a density of 1.1 × 105 cells/ml per well in a 96-well plate at 37°C in a 5% CO2 incubator overnight. Each concentration was tested in triplicate. Then, the cells were incubated with 10 µL of MTT (5 mg/ml) for additional 4 h. The residual liquid was removed, and 200 µL DMSO was added. The absorbance was recorded on a microplate reader at a wavelength of 570 nm. The experiments were conducted a minimum of three times.
4.7 Statistical Analysis
Each experiment was repeated for at least three times. Results were expressed as mean ± SD. The statistical significance of differences between groups was evaluated by the unpaired Student’s t-test and indicated with (**) p < 0.01, (*) p < 0.05.
Statistical software SPSS version 15.0 was used for statistical analysis. ANOVA analysis was used with Fisher’s LSD multiple comparison test for multiple comparisons. All p-values < 0.05 were considered statistically significant.
Data Availability Statement
The original contributions presented in the study are included in the article/Supplementary Materials; further inquiries can be directed to the corresponding author.
Author Contributions
HW was responsible for the experimental design. H-WG was responsible for compound isolation. JH and J-XW were responsible for cytotoxic activity testing. Y-GT and Y-HL were responsible for structure elucidation. HL was responsible for compound identities. All authors have read and agreed to the published version of the manuscript.
Funding
This work was supported by the National Natural Science Foundation of China (No. 81960774).
Conflict of Interest
The authors declare that the research was conducted in the absence of any commercial or financial relationships that could be construed as a potential conflict of interest.
Publisher’s Note
All claims expressed in this article are solely those of the authors and do not necessarily represent those of their affiliated organizations, or those of the publisher, the editors, and the reviewers. Any product that may be evaluated in this article, or claim that may be made by its manufacturer, is not guaranteed or endorsed by the publisher.
Supplementary Material
The Supplementary Material for this article can be found online at: https://www.frontiersin.org/articles/10.3389/fchem.2021.799911/full#supplementary-material
References
Abe, F., Yamauchi, T., Honda, K., and Hayashi, N. (2000). Marstomentosides O-T, Polyoxypregnane Glycosides from Marsdenia Tomentosa. Chem. Pharm. Bull. 48 (1), 154–156. doi:10.1248/cpb.48.154
Bai, H., Li, W., Asada, Y., Satou, T., Wang, Y., and Koike, K. (2009). Twelve Pregnane Glycosides from Cynanchum Atratum. Steroids 74, 198–207. doi:10.1016/j.steroids.2008.10.007
Bai, H., Wang, Y. S., and Liu, A. Q. (2007). Advances Study on the C21 Steroid Constituents of Cynanchum Plants. Nat. Prod. Res. Dev. 19, 879–904. doi:10.16333/j.1001-6880.2007.05.041
Chen, J.-J., Zhang, Z.-X., Zhou, J., and Li, B.-T. (1999). New Pregnane Glycosides from Sinomarsdenia Incisa. J. Nat. Prod. 62, 829–832. doi:10.1021/np9804684
Deng, J., Liao, Z., and Chen, D. (2005). Marsdenosides A-H, Polyoxypregnane Glycosides from Marsdenia Tenacissima. Phytochemistry 66, 1040–1051. doi:10.1002/chin.20054420110.1016/j.phytochem.2005.03.018
Ge, Y. N., Zhang, G. Z., Liu, Z. Z., and Zheng, Z. D. (2019). The Clinical Efficacy and Adverse Effects of Xiao'aiping Injection Combined with S-1 Capsule in the Treatment of Elderly Patients with Advanced Gastric Cancer. Anti-tumor Pharm. 9, 892–896. doi:10.3969/j.issn.2095-1264.2019.06.12
Gu, X. J., and Hao, D. C. (2016). Recent Advances in Phytochemistry and Pharmacology of C21 Steroid Constituents from Cynanchum Plants. Chin. J. Nat. Med. 14, 321–334. doi:10.3724/SP.J.1009.2016.00321
Halim, A. F., and Khalil, A. T. (1996). Pregnane Glycosides from Caralluma Retrospiciens. Phytochemistry 42, 1135–1139. doi:10.1016/0031-9422(96)00029-5
Hanson, J. R. (2017). A Hundred Years in the Elucidation of the Structures of Natural Products. Sci. Progress 100, 63–79.
Itthiarbha, A., Phitak, T., Sanyacharernkul, S., Pothacharoen, P., Pompimon, W., and Kongtawelert, P. (2012). Polyoxypregnane Glycoside from Dregea Volubilis Extract Inhibits IL-1β-induced Expression of Matrix Metalloproteinase via Activation of NF-Κb in Human Chondrocytes. In Vitro Cell.Dev.Biol.-Animal 48, 43–53. doi:10.1007/s11626-011-9475-7
Li, H., Peng, C. Z., Guan, Y. H., Niu, Y. F., and Zhang, L. X. (2011). Resources Investigation on Aspidopterys Obcordata. Shi Zhen Guo Yi Guo Yao 22, 2999–3000. doi:10.1016/S0254-6272(16)30052-8
Li, Q. F., Wang, X. L., Ding, L. S., and Zhang, C. (2007). Polyoxypregnanes from the Stems of Marsdenia Tenacissima. Chin. Chem. Lett. 18, 831–834. doi:10.1016/j.cclet.2007.05.014
Li, Y., Li, G., Song, M., Li, X., Zhan, X., Lu, J., et al. (2016). Acute Toxicity Study of Aspidopterys Obcordata Aqueous Extract in Sprague-Dawley Rats. J. Tradit. Chin. Med. 36, 377–381. doi:10.1016/s0254-6272(16)30052-8
Li, Y., Ma, G., Lv, Y., Su, J., Li, G., and Chen, X. (2019). Efficacy of Obcordata A from Aspidopterys Obcordata on Kidney Stones by Inhibiting NOX4 Expression. Molecules 24, 1957. doi:10.3390/molecules24101957
Liu, T. X., Gan, Z., Huang, Q., and Zeng, T. (2018). Research Advances on the Antitumor Activity of C21 Steroid Constituents of Asclepiadaceae in the Last 5 Years. Chin. J. Mod. Drug Appl. 12, 217–218. doi:10.3724/SP.J.1009.2016.00321
Liu, X.-J., Shi, Y., Jia, S.-H., Deng, Y.-L., Lv, F., and Dai, R.-J. (2017). Six New C-21 Steroidal Glycosides from Dregea Sinensis Hemsl. J. Asian Nat. Prod. Res. 19, 745–753. doi:10.1080/10286020.2017.1281912
Liu, X., Zhang, Y., Huang, W., Luo, J., Li, Y., Tan, W., et al. (2018). Development of High Potent and Selective Bcl-2 Inhibitors Bearing the Structural Elements of Natural Product Artemisinin. Eur. J. Med. Chem. 159, 149–165. doi:10.1016/j.ejmech.2018.09.059
Ma, L.-F., Miao, C.-T., Qian, H.-Y., Wang, P.-F., Wang, F.-S., Zhan, Z.-J., et al. (2017). New C21 Steroidal Glycosides, Cynotophyllosides M-O, from Cynanchum Otophyllum. J. Chem. Res. 41, 195–198. doi:10.3184/174751917X14894997017414
Newman, D. J., and Cragg, G. M. (2020). Natural Products as Sources of New Drugs over the Nearly Four Decades from 01/1981 to 09/2019. J. Nat. Prod. 83, 770–803. doi:10.1021/acs.jnatprod.9b01285
Ni, Y., and Ye, Y. P. (2010). Distribution of C21 Steroidal Glycosides in Plants of Asclepiadaceae and Their Pharmacological Activities. Chin. Trad. Herbal. Drugs 41, 162–166.
Niranjan, P. S., Nilendu, P., Nirup, B. M., Banerjee, S., Koike, K., and Nikaido, T. (2002). Polyoxypregnane Glycosides from the Flowers of Dregea Volubilis. Phytochem 61, 383–388. doi:10.1016/S0031-9422(02)00260-1
Panda, N., Banerjee, S., Mandal, N. B., and Sahu, N. P. (2006). Pregnane Glycosides. Nat. Product. Commun. 1, 1934578X0600100–695. doi:10.1177/1934578X0600100813
Pang, X., Kang, L.-P., Fang, X., Zhao, Y., Yu, H.-S., Han, L.-F., et al. (2017). Polyoxypregnane Glycosides from the Roots of Marsdenia Tenacissima and Their Anti-HIV Activities. Planta Med. 83, 126–134. doi:10.1055/s-0042-108057
Pang, X., Kang, L.-P., Yu, H.-S., Zhao, Y., Han, L.-F., Zhang, J., et al. (2015). New Polyoxypregnane Glycosides from the Roots of Marsdenia Tenacissima. Steroids 93, 68–76. doi:10.1016/j.steroids.2014.11.004
Plaza, A., Perrone, A., Balestrieri, M., Felice, F., Balestrieri, C., Hamed, A., et al. (2005). New Unusual Pregnane Glycosides with Antiproliferative Activity from. Steroids 70, 594–603. doi:10.1016/j.steroids.2005.02.019
Plaza, A., Piacente, S., Perrone, A., Hamed, A., Pizza, C., and Bifulco, G. (2004). Stemmosides C and D, Two Novel Unusual Pregnane Glycosides from Solenostemma Argel: Structural Elucidation and Configurational Study by a Combined NMR-Quantum Mechanical Strategy. Tetrahedron 60, 12201–12209. doi:10.1016/j.tet.2004.10.021
Pu, Z. J., Yang, A. Z., Qing, S. K., Xu, D., Chen, H. Y., Xu, H. J., et al. (2020). Study on Apoptosis of HepG-2 Cells Induced by Xiaoaiping Injection Combined with Oxaliplatin and its Related Mechanism. Hubei J. TCM. 42, 3–6.
Sanyacharernkul, S., Itghiarbha, A., Kongtawele, P., Meepowpan, P., Nuntasaen, N., and Pompimon, W. (2009). A New Polyoxypregnane Glycoside from the Roots of Dregea Volubilis (L.F) Benth. Ex Hook. F and its Chondroprotective Effect. Am. J. Biochem. Biotechnol. 5, 202–209. doi:10.3844/ajbbsp.2009.202.209
Shen, W. C., and Zhang, R. S. (2010). Asclepiadaceae Plants C_(21) Steroidal Glycosides Pharmacologic. Chin. J. Ethnomed. Ethnopharm. 21, 46–48. doi:10.1515/revneuro.2010.21.1.29
Song, J., Dai, R., Deng, Y., and Lv, F. (2018). Rapid Structure Prediction by HPLC-ESI-MS N of Twenty-Five Polyoxypregnane Tetraglycosides from Dregea Sinensis with NMR Confirmation of Eight Structures. Phytochemistry 147, 147–157. doi:10.1016/j.phytochem.2017.12.021
Sun, D. F., Sun, J. Y., Fan, H. X., and Yao, Q. Q. (2014). Advances in Studies on C21 Steroidal Glycosides of Plants in Asclepiadaceae. Chin.Trad. Herbal Drugs 45, 1491–1495. doi:10.7501/j.issn.0253-2670.2014.10.02
Tong, L., Dong, J. J., Wang, M. S., Zhou, Y. C., Zhong, Z. L., Tan, Z., et al. (2015). Treatment of Non-M3 Acute Myeloid Leukemia by Combination of Xiaoaiping Injection and Chemotherapy. Hebei J. TCM 37, 95–97. doi:10.3969/j.issn.1002-2619.2015.01.038
Wang, X.-L., Peng, S.-L., and Ding, L.-S. (2010). Further Polyoxypregnane Glycosides from Marsdenia Tenacissima. J. Asian Nat. Prod. Res. 12, 654–661. doi:10.1080/10286020.2010.495330
Wang, X. G., Tian, N., Zhang, S. Y., Chen, J. H., Zhou, Y., and Chen, W. T. (2019). Clinical Study on Rectal Administration of Xiao'aiping Injection Combined with Chemotherapy in the Treatment of Prostate Cancer. China Pharmaceuticals 28, 48–50. doi:10.3969/j.issn.1006-4931.2019.16.015
Wang, Y., Qi, Z., Qi, M., Hu, Z., and Wu, W. (2016). Effects of Periplocoside P from Periploca Sepium on the Midgut Transmembrane Potential of Mythimna Separata Larvaeffects of Periplocoside P from Periploca Sepium on the Midgut Transmembrane Potential of Mythimna Separata Larvae. Sci. Rep. 6, 36982. doi:10.1038/srep36982
Xue, H. L., Huang, X. D., He, D., Lin, S. J., Wang, S., and Niu, T. (2012). Effects of Marsdenia Tenacissima Extract on Proliferation and Apoptosis of Hematologic Neoplasm Cell Line Cells. Sichuan Da Xue Xue Bao Yi Xue Ban 43, 174–179. doi:10.13464/j.scuxbyxb.2012.02.014
Yao, S., To, K. K.-W., Wang, Y.-Z., Yin, C., Chai, S., et al. (2014). Polyoxypregnane Steroids from the Stems of Marsdenia Tenacissima. J. Nat. Prod. 77, 2044–2053. doi:10.1016/10.1021/np500385b
Yu, H. J. (2019). Clinical Efficacy and Safety Evaluation of Xiaoaiping Combined with Chemotherapy in the Treatment of Lung Cancer. Chin. J. Mod. Drug Appl. 13, 86–87.
Zhan, Z. J., Bao, S. M., Zhang, Y., Qiu, F. J., Shan, W. G., and Ma, L. F. (2019). New Immunomodulating Polyhydroxypregnane Glycosides from the Roots of Cynanchum Otophyllum C.K. Schneid. C&B 16, e1900062. doi:10.1002/cbdv.201900062
Zhang, H., Tan, A.-M., Zhang, A.-Y., Chen, R., Yang, S.-B., and Huang, X. (2010). Five New C21 Steroidal Glycosides from the Stems of Marsdenia Tenacissima. Steroids 75, 176–183. doi:10.1016/j.steroids.2009.11.003
Zhang, M., Li, X., Xiang, C., Qin, Y., He, J., Li, B. C., et al. (2015). Cytotoxicity of Pregnane Glycosides of Cynanchum Otophyllum. Steroids. 104, 49–60. doi:10.1016/j.steroids.2015.08.010
Zhang, X., Zhou, Y., Zuo, J., and Yu, B. (2015). Total Synthesis of Periploside A, a Unique Pregnane Hexasaccharide with Potent Immunosuppressive Effects. Nat. Commun. 6, 5879. doi:10.1038/ncomms6879
Zhao, X. M., Hou, W., Yan, J. Y., Li, Z. L., Wang, M. S., Wu, S. Y., et al. (2007). Xiaoaiping Injection Treatment of 6 Cases of Acute Leukemia. JETCM 16, 105.
Zheng, K., Zhang, G., Jiang, N., Yang, S., Li, C., Meng, Z., et al. (2014). Analysis of the Transcriptome of Marsdenia Tenacissima Discovers Putative Polyoxypregnane Glycoside Biosynthetic Genes and Genetic Markers. Genomics 104, 186–193. doi:10.1016/j.ygeno.2014.07.013
Keywords: Aspidopterys obcordata, ethnic medicine, polyoxypregnane derivatives, HL-60, structure–activity relationship
Citation: Guo H-W, Tian Y-G, Liu Y-H, Huang J, Wang J-X, Long H and Wei H (2022) Discovery of Polyoxypregnane Derivatives From Aspidopterys obcordata With Their Potential Antitumor Activity. Front. Chem. 9:799911. doi: 10.3389/fchem.2021.799911
Received: 22 October 2021; Accepted: 06 December 2021;
Published: 05 January 2022.
Edited by:
Zhendong Jin, The University of Iowa, United StatesReviewed by:
Guoxu Ma, Chinese Academy of Medical Sciences and Peking Union Medical College, ChinaWei Gao, Capital Medical University, China
Copyright © 2022 Guo, Tian, Liu, Huang, Wang, Long and Wei. This is an open-access article distributed under the terms of the Creative Commons Attribution License (CC BY). The use, distribution or reproduction in other forums is permitted, provided the original author(s) and the copyright owner(s) are credited and that the original publication in this journal is cited, in accordance with accepted academic practice. No use, distribution or reproduction is permitted which does not comply with these terms.
*Correspondence: Hua Wei, d2VpaHVhMjBAMTI2LmNvbQ==