- 1College of Chemistry and Material Sciences, South-Central University for Nationalities, Wuhan, China
- 2School of Pharmaceutical Sciences, South-Central University for Nationalities, Wuhan, China
Eleven new polycyclic polyprenylated acylphloroglucinols (PPAPs, 1–11) and three new monocyclic polyprenylated acylphloroglucinols (MPAPs, 12–14), together with ten known analogues were isolated from the fruits of Garcinia multiflora. These PPAPs belong to three types including the bicyclic polyprenylated acylphloroglucinols (BPAPs), the caged PPAPs, and the complicated PPAPs. Their structures and absolute configurations were determined through HRESIMS, NMR spectroscopy data, electronic circular dichroism (ECD) calculations, and gauge-independent atomic orbital (GIAO) NMR calculations with DP4+ analyses. Moreover, compounds 2 and 7 exhibited moderate cytotoxicity against three human cancer lines (MCF-7, T98, and HepG2) with IC50 values ranging from 9.81 ± 1.56 to 17.00 ± 2.75 μM.
Introduction
The plants of Guttiferae and Hypericaceae family mainly including the genus Garcinia and Hypericum are well-known for producing structurally diverse and biologically polycyclic polyprenylated acylphloroglucinols (PPAPs). Previous phytochemical studies indicated that more than 500 PPAPs have been isolated the plants of Guttiferae family with diverse structural scaffolds including the bicyclic polyprenylated acylphloroglucinols (BPAPs), the caged PPAPs, and the complicated PPAPs. BPAPs comprise approximate 60% of PPAPs which share a bicyclo [3.3.1]nonane-2,4,9-trione core (Yang et al., 2018). Depending on the relative position of the acyl group connected to the phloroglucinol core, BPAPs are categorized into type A or B. Type A BPAPs contain a C-1 acyl group which is next to C-8 quaternary center, while type B BPAPs contain a C-3 acyl group (Ciochina and Grossman, 2006). BPAPs have attracted noticeable attention from both natural product and medicinal chemists due to their fascinating chemical structures and intriguing biological activities (Phang et al., 2020).
Garcinia multiflora Champ belongs to the genus of Garcinia, which is mainly distributed in the southern region of China. The fruit can be eaten raw when it is ripe, which possesses a high nutritional value and contains pharmacologically active compounds (Liu et al., 2017a; Xu et al., 2017). Previous research results indicated that the fruits, stems, roots, leaves, and twigs of G. multiflora might be an important source of PPAPs (Chien et al., 2008; Chen et al., 2009; Liu et al., 2010; Ting et al., 2012; Ting et al., 2014; Fan et al., 2015; Fu et al., 2015; Tian et al., 2016; Fan et al., 2016; Cheng et al., 2018a; Cheng et al., 2018b; Wang et al., 2018). In our previous study, four new complicated PPAPs with new carbon skeletons tricyclo [3.3.1.1.4,8]decane, 14 new-caged PPAPs, and two new cyclohexanone—monocyclic polycyclic polyprenylated acylphloroglucinols (MPAPs) from the fruits of G. multiflora were reported (Chen et al., 2019a; Chen et al., 2019b; Teng et al., 2019; Teng et al., 2020). As part of ongoing research, phytochemical investigations of an extract of the fruits of G. multiflora afforded 11 new PPAPs and three new MPAPs (Figure 1). This study reported the isolation, structure identification, and biological activity of these compounds.
Materials and Methods
General Experimental Procedures
Optical rotations were determined in MeOH using an Autopol IV polarimeter (Rudolph Research Analytical, Hackettstown, NJ, United States). UV spectra were obtained using a UH5300 UV-VIS Double-Beam spectrophotometer (Hitachi Co., Tokyo, Japan). 1D and 2D NMR spectra were recorded with a Bruker AVANCE IIITM 600 MHz spectrometer (Bruker, Ettlingen, Germany) in CDCl3 using TMS as internal standard. HR-ESIMS data were obtained using a Thermo Fisher Scientific Q Exactive Orbitrap LC-MS/MS System (Thermo Fisher Scientific, Waltham, MA, United States). An Ultimate 3000 HPLC system (Dionex Co., Sunnyvale, CA, United States) with an Ultimate 3,000 pump and an Ultimate 3,000 Variable Wavelength Detector was used to perform semipreparative HPLC, with a Nacalai Tesque 5C18-MS-II column (250 × 10 mm, 5 μm). Silica gel for CC (200–300 mesh and 300–400 mesh) was obtained from the Qingdao Hai Yang Chemical Group Co. (Qingdao, China). The human tumor cell lines HepG2, T98G, and MCF-7 were purchased from the cell bank of the Chinese Academy of Sciences (Shanghai, China). Cisplatin was purchased from Sigma-Aldrich (Saint Louis, MO, United States). The Cell Counting Kit (CCK-8) was purchased from Beyotime Biotechnology (Shanghai, China). Dulbecco’s modified Eagle’s medium (DMEM) and penicillin–streptomycin solution were purchased from GE Healthcare Life Sciences (Logan, UT, United States). Fetal bovine serum (FBS) was purchased from Gibco and Life Technologies (Grand Island, NY, United States). Reagent grade DMSO was purchased from Vetec and Sigma Chemical Co. (St Louis, MO, United States). The absorbance was recorded with a Multiskan GO microplate reader (Thermo Fisher Scientific, Inc., Waltham, MA, United States). The organic solvents were obtained from Sinopharm Chemical Reagent Co., Ltd. (Shanghai, China).
Plant Material
The fruits of G. multiflora were purchased from Nanning, Guangxi Zhuang Autonomous Region, P. R. China, and identified by Prof. Hongli Teng, Guangxi Zhuang Medicine International Hospital. The voucher specimen (2014091201) was deposited in the herbarium of School of Pharmaceutical Sciences, South Central University for Nationalities.
Extraction and Isolation
The dried fruits of G. multiflora Champ (5.2 kg) were powdered and extracted with 95% EtOH at room temperature for three times (each times for 24 h) to obtain EtOH extract 2.21 kg and then successively partitioned with petroleum ether (PE), EtOAc, and n-BuOH to get PE extract 125 g, EtOAc extract 166 g, and n-BuOH extract 80 g. The PE extract (125 g) was chromatographed on a silica gel column (200–300 mesh) eluted successively with PE/acetone gradient (50:1, 25:1, 10:1, 7:3, 1:1, and 0:1) to obtain six fractions (Fr. 1-Fr. 6). Fr. 2 (42.5 g) was chromatographed on a silica gel column (200–300 mesh) eluted successively with PE/CH2Cl2 gradient (10:1 to 0:1) to obtain 11 fractions (Fr. 2.1–Fr. 2.11). Fr. 2.7 (9.2 g) was separated on an ODS column, eluted with H2O-MeOH (7:3 to 0:1), and repeated semi-preparative HPLC to afford compounds 20 (20.0 mg; CH3CN-H2O, 78:22, tR 31.7 min); 21 (3.2 mg; CH3CN-H2O, 84:16, tR 91.5 min); 23 (3.4 mg; CH3CN-H2O, 85:15, tR 26.3 min); and 24 (15.9 mg; CH3CN-H2O, 85:15, tR 56.6 min). Fr. 2.9 (2.3 g) was further separated by silica gel CC (PE/CH2Cl2/MeOH, 10:1:0.1 to 0:1:0.1) and repeated semi-preparative HPLC to afford compounds 10 (1.2 mg; CH3CN-H2O, 93:7, tR 20.0 min); 13 (2.2 mg; CH3CN-H2O, 93:7, tR 54.0 min); and 17 (1.0 mg; CH3CN-H2O, 90:10, tR 25.0 min). Fr. 2.10 (4.6 g) was further purified by semi-preparative HPLC (CH3CN-H2O, 87:13) to afford compounds 1 (2.2 mg) at tR 55.1 min, 12 (1.7 mg) at tR 64.2 min, and 15 (7.5 mg) at tR 70.8 min. Fr. 3 (31.0 g) was subjected to repeated silica gel CC with PE/CH2Cl2 (50:1 to 0:1), ODS CC with H2O-MeOH (7:3 to 0:1), and semi-preparative HPLC to afford compounds 3 (1.4 mg; MeOH-H2O, 90:10, tR 18.0 min); 5 (2.0 mg; MeOH-H2O, 93:7, tR 12.9 min); 8 (2.6 mg; CH3CN-H2O, 75:25, tR 40.0 min); 9 (15.1 mg; CH3CN-H2O, 93:7, tR 16.5 min); 16 (7.8 mg; CH3CN-H2O, 80:20, tR 19.2 min); and 18 (5.0 mg; CH3CN-H2O, 90:10, tR 17.5 min). The EtOAc extract (166 g) was chromatographed on a silica gel column (200–300 mesh) eluted successively with PE/EtOAc gradient (20:1 to 0:1) to obtain nine fractions (Fr. 1–Fr. 9). Fr. 1 (5.7 g) was subjected to ODS CC with H2O-MeOH (7:3 to 0:1), a silica gel CC with PE/CH2Cl2 (10:1 to 0:1), and semi-preparative HPLC to afford compounds 4 (2.3 mg; CH3CN-H2O, 85:15, tR 20.1 min); 6 (11.4 mg; CH3CN-H2O, 87.7:12.3, tR 59.3 min); and 14 (4.3 mg; CH3CN-H2O, 85:15, tR 27.9 min). Fr. 2 (15.6 g) was subjected to a silica gel CC with PE/CH2Cl2 (10:1 to 0:1) and semi-preparative HPLC to afford compounds 11 (11 mg; CH3CN-H2O, 74:26, tR 61.7 min) and 19 (10 mg; CH3CN-H2O, 74:26, tR 55.9 min). Fr. 4 (18.7 g) was subjected to a silica gel CC with PE/EtOAc (10:1 to 0:1), ODS CC with H2O-MeOH (7:3 to 0:1), and semi-preparative HPLC to afford compounds 2 (24.5 mg; CH3CN-H2O, 83:17, tR 31.7 min); 7 (3.8 mg; CH3CN-H2O, 80:20, tR 32.4 min); and 22 (5.5mg; CH3CN-H2O, 83:17, tR 23.8 min).
Spectroscopic Data
Garcimultinone D (1): white amorphous powder; [α]20 D +186.27 (c 0.02, MeOH); UV (MeOH) λmax (log ε) 215 (3.71) and 245 (3.89), 280 (3.81) nm; ECD (MeOH) λ (θ) 204 (−13.49), 229 (+0.59), 249 (−3.23), 274 (+8.77), 300 (+3.02), and 317 (+4.37) nm; 1H and 13C NMR (CDCl3), see Tables 1 and 2; HRESIMS m/z 571.3781 [M+H]+ (calcd for C38H51O4, 571.3782).
Garcimultinone E (2): white amorphous powder; [α]20 D +11.22 (c 0.15, MeOH); UV (MeOH) λmax (log ε) 235 (3.18) and 310 (3.17) nm; ECD (MeOH) λ (θ) 248 (+0.33), 274 (−0.39), and 311 (+0.96) nm; 1H and 13C NMR (CDCl3), see Tables 1 and 2; HRESIMS m/z 603.3679 [M+H]+ (calcd for C38H51O6, 603.3641).
Garcimultinone F (3): white amorphous powder; [α]20 D +117.78 (c 0.02, MeOH); UV (MeOH) λmax (log ε) 245 (4.04) and 290 (4.01) nm; ECD (MeOH) λ (θ) 225 (+4.85), 249 (−13.36), 284 (+12.82), 318 (−0.05), and 338 (+1.39) nm; 1H and 13C NMR (CDCl3), see Tables 1 and 2; HRESIMS m/z 587.37311 [M+H]+ (calcd for C38H51O5, 587.3731).
Garcimultinone G (4): colorless oil; [α]20 D +159.26 (c 0.02, MeOH); UV (MeOH) λmax (log ε) 245 (4.02) and 280 (3.97) nm; ECD (MeOH) λ (θ) 222 (+4.07), 248 (−11.65), 273 (+14.02), 319 (+0.62), and 331 (+1.66) nm; 1H and 13C NMR (CDCl3), see Tables 1 and 2; HRESIMS m/z 549.3209 [M+H]+ (calcd for C34H45O6, 549.3211).
Garcimultinone H (5): colorless oil; [α]20 D +35.0 (c 0.02, MeOH); UV (MeOH) λmax (log ε) 210 (3.51) nm; ECD (MeOH) λ (θ) 223 (+1.54), 256 (−1.26), 281 (−0.32), 306 (−0.62), and 349 (+0.57) nm; 1H and 13C NMR (CDCl3), see Tables 1 and 2; HRESIMS m/z 585.3587 [M-H]− (calcd for C38H49O5, 585.3586).
Garcimultinone I (6): colorless oil; [α]20 D +73.90 (c 0.09, MeOH); UV (MeOH) λmax (log ε) 235 (3.38) and 290 (3.35) nm; ECD (MeOH) λ (θ) 207 (−10.10), 218 (+9.57), 247 (−27.76), 266 (−1.51), 276 (−4.48), and 305 (+10.00) nm; 1H and 13C NMR (CDCl3), see Tables 1 and 2; HRESIMS m/z 571.3778 [M+H]+ (calcd for C38H51O4, 571.3782).
Garcimultinone J (7): colorless oil; [α]20 D −5.07 (c 0.05, MeOH); UV (MeOH) λmax (log ε) 210 (3.44), 230 (3.39), and 275 (3.48) nm; ECD (MeOH) λ (θ) 225 (+0.51), 250 (+0.07), 267 (+0.41), 298 (−0.97), and 329 (+0.34) nm; 1H and 13C NMR (CDCl3), see Tables 1 and 2; HRESIMS m/z 603.3676 [M+H]+ (calcd for C38H51O6, 603.3641).
Garcimultinone K (8): pale yellow oil; [α]20 D−12.00 (c 0.05, MeOH); UV (MeOH) λmax (log ε) 220 (3.58), 245 (3.61), and 280 (3.50) nm; ECD (MeOH) λ (θ) 219 (+2.00), 250 (−15.45), 269 (−6.47), 284 (−11.22), and 321 (+5.44) nm; 1H and 13C NMR (CDCl3), see Tables 1 and 3; HRESIMS m/z 617.3488 [M-H]− (calcd for C38H49O7, 617.3484).
Garcimultinone L (9): white amorphous powder; [α]20 D +110.00 (c 0.04, MeOH); UV (MeOH) λmax (log ε) 305 (3.24) nm; ECD (MeOH) λ (θ) 204 (−3.44), 218 (+6.00), 250 (−15.64), 272 (−1.72), 284 (−3.25), and 314 (+7.34) nm; 1H and 13C NMR (CDCl3), see Tables 1 and 3; HRESIMS m/z 587.3726 [M+H]+ (calcd for C38H51O5, 587.3731).
Garcimultinone M (10): white amorphous powder; [α]20 D −114.44 (c 0.02, MeOH); UV (MeOH) λmax (log ε) 240 (4.03) nm; ECD (MeOH) λ (θ) 221 (+12.96), 272 (−11.16), 302 (+2.51), 322 (−0.79), and 351 (+2.08) nm; 1H and 13C NMR (CDCl3), see Tables 1 and 3; HRESIMS m/z 571.3780 [M+H]+ (calcd for C38H51O4, 571.3782).
Garcimultinone N (11): white amorphous powder; [α]20 D +12.59 (c 0.04, MeOH); UV (MeOH) λmax (log ε) 210 (3.48) and 245 (3.44) nm; ECD (MeOH) λ (θ) 210 (−2.47), 248 (+13.52), 289 (−8.74), and 323 (+2.92) nm; 1H and 13C NMR (CDCl3), see Tables 1 and 3; HRESIMS m/z 619.3630 [M+H]+ (calcd for C38H51O7, 619.3629).
Garcimultinone O (12): white amorphous powder; [α]20 D +71.43 (c 0.03, MeOH); UV (MeOH) λmax (log ε) 230 (3.73) and 355 (3.50) nm; ECD (MeOH) λ (θ) 215 (+2.95), 230 (−1.46), 254 (+5.15), 281 (−1.13), and 359 (+2.31) nm; 1H and 13C NMR (CDCl3), see Tables 1 and 3; HRESIMS m/z 503.3158 [M+H]+ (calcd for C33H43O4, 503.3156).
Garcimultinone P (13): white amorphous powder; [α]20 D −76.67 (c 0.04, MeOH); UV (MeOH) λmax (log ε) 235 (3.75) and 350 (3.81) nm; ECD (MeOH) λ (θ) 227 (+5.26), 257 (−11.64), 295 (+3.38), and 364 (−3.93) nm; 1H and 13C NMR (CDCl3), see Tables 1 and 3; HRESIMS m/z 571.3780 [M+H]+ (calcd for C38H51O4, 571.3782).
Garcimultinone Q (14): yellow powder; [α]20 D +41.60 (c 0.04, MeOH); UV (MeOH) λmax (log ε) 235 (3.63) and 315 (3.65) nm; ECD (MeOH) λ (θ) 220 (+1.21), 250 (−0.54), and 279 (+2.23) nm; 1H and 13C NMR (CDCl3), see Tables 1 and 3; HRESIMS m/z 435.2532 [M+H]+ (calcd for C28H35O4, 435.2530).
NMR Calculations
The calculated NMR data were acquired using the Gauge-Including Atomic Orbitals (GIAO) method at the mPW1PW91/6–311+G (2 d,p) level in CHCl3 with the IEFPCM model (the detailed NMR calculations are described in the Supplementary information).
ECD Calculations
The ECD calculation was conducted using time-dependent density functional theory (TD-DFT) in methanol by the IEFPCM model (the detailed ECD calculations are described in the Supplementary information).
Antiproliferative Activity Bioassay
The antiproliferative activities against HepG2, T98, and MCF-7 cell lines of isolated compounds were measured by the CCK-8 method using cisplatin as the positive control, according to the protocol described previously (Teng et al., 2019).
Results and Discussion
PPAPs
Depending on the relative configuration at C-7 relative to C-1/C-5, BPAPs can also be subclassified into endo- and exo-subtypes. In case of endo-BPAPs, the chemical shifts of C-7 and Me-38 (axial position) appeared at 45–49 and 26–27 ppm, respectively. In case of exo-BPAPs, the chemical shifts of C-7 and Me-38 (axial position) displayed upfield signals at 41–44 and 16–17 ppm resulting from a γ-gauche effect between Me-38 and the CH2-32. Therefore, the analysis of 13C-NMR data is a powerful tool to distinguish the two types of BPAPs (Marti et al., 2010; Phang et al., 2020).
Type A BPAPs
Compound 1 was isolated as a white amorphous powder with the molecular formula of C38H50O4 implied by the HRESIMS at m/z 571.3781 [M + H]+ (calcd 571.3782) suggesting 14 degrees of unsaturation. Its 1H NMR spectrum (Table 2) contained signals of three olefinic protons (δH 5.09, 1H, br t, J = 7.2 Hz; 5.03, 1H, br t, J = 7.2 Hz; 5.00, 1H, br t, J = 6.0 Hz), 10 singlet methyls (δH1.15–1.74), and one unsubstituted phenyl group (δH 7.52, 2H, d, J = 7.2 Hz; 7.24, 2H, t, J = 7.2 Hz; 7.39, 1H, t, J = 7.2 Hz). Detailed analysis of 13C-NMR, DEPT, and HSQC spectrum indicated the presence of characteristic peaks of the bicyclo[3.3.1]nonane skeleton, including a methylene at δC 40.7 (C-6), a methine at δC 42.8 (C-7), three sp3 quaternary carbons at δC 79.3 (C-1), 51.2 (C-5), and 48.0 (C-8), a conjugated carbonyl carbon at δC 193.9 (C-10), a non-conjugated carbonyl carbon at δC 208.4 (C-9), and an enolized 1,3-diketo group at δC 193.0 (C-2), 124.7 (C-3), and 167.3 (C-4). The chemical shift of C-1 at δC 79.3 and HMBC correlations (Figure 2) from Me-37 and Me-38 to C-8 (δC 48.0) and C-1 suggested that compound 1 might belong to Type A BPAPs. By comparison of NMR data of 1 with those of garcimultiflorone A suggested that their structures were closely resembled (Chen et al., 2009). The major difference between 1 and garcimultiflorone A was that the resonances for C-7 (δC 42.8) and C-38 (δC 16.3) in 1 were shifted upfield compared to C-7 (δC 47.8) and C-38 (δC 26.8) in garcimultiflorone A, suggesting that 1 was the 7-epimer of garcimultiflorone A which was further supported by ROESY correlations of H2-32/H3-38 and H-7/H3-37 (Figure 3). To further determine the relative configuration of C-23, two possible isomers (1S*, 5R*, 7R*, and 23R*)-1a and (1S*, 5R*, 7R*, and 23S*)-1b were calculated by the DP4+ method. The results revealed that the experimental NMR data for 1 gave the best match of DP4+ probability 100% with the (1S*, 5R*, 7R*, and 23R*)-1a isomer. To ascertain the absolute configuration of 1 (1S, 5R, 7R, and 23R)-1a, and its enantiomer 1a′ were calculated by the time-dependent density functional theory (TDDFT) method. As a result, the experimental ECD spectrum of 1 matched well with the calculated ECD spectrum of (1S, 5R, 7R, and 23R)-1a (Figure 4). Thus, the absolute configuration of 1 was determined as (1S, 5R, 7R, and 23R). Finally, the structure of 1 was elucidated to be exo-BPAPs and named as garcimultinone D.
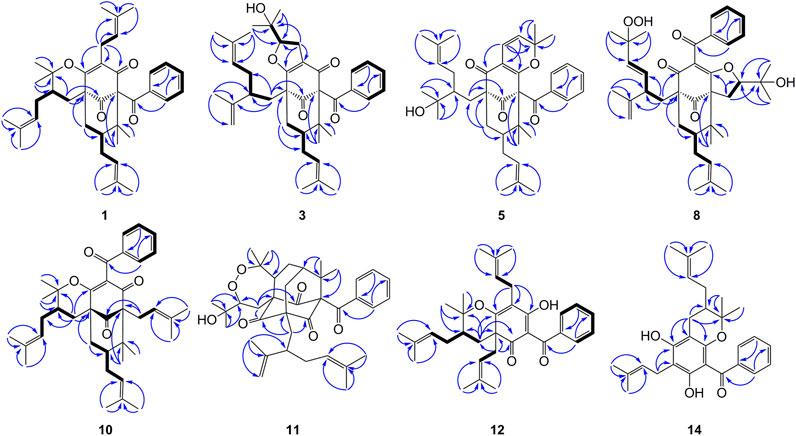
FIGURE 2. Key HMBC for compounds 1, 3, 5, 8, 10–12, and 14, and 1H–1H COSY correlations for 1, 3, 8, 10, 12, and 14.
Compound 2 was isolated as a white amorphous powder. The molecular formula was established as C38H50O6 based on a pseudo molecular ion peak at m/z 603.3679 [M + H]+ (calcd 603.3641), indicating 32 mass units more than 1. The NMR data (Tables 1 and 2) of 2 closely matched those of 1, except for the presence of a 3,4-dihydroxybenzoyl group at C-1, replacing a benzoyl group at C-1 in 1, which was supported by HMBC correlations H-12 and H-16 to C-10. The relative configuration of 2 was established as the same as that of 1 by ROESY spectrum (Supplementary Figure S138, Supplementary information) and 13C NMR data. The absolute configuration of 2 was finally assigned as (1S, 5R, 7R, and 23R) by comparing the experimental and calculated ECD spectra (Supplementary Figure S155, Supplementary information). Thus, the structure of compound 2 was elucidated, as shown in Figure 1, and named as garcimultinone E.
Compound 3 was obtained as a white amorphous powder and showed a pseudo molecular ion peak at m/z 587.3733 [M + H]+ (calcd 587.3731) in the HRESIMS, corresponding to the molecular formula C38H50O5. The 1H and 13C NMR data of 3 closely resembled those of hyperattenin C with the only difference being the presence of isogeranyl located at C-5 in 3, instead of geranyl located at C-5 in hyperattenin C (Li et al., 2015). The assignment was further corroborated by the HMBC correlations from H2-22 to C-5 and C-9 (Figure 2). The relative configuration of 3 was determined by ROESY spectrum (Supplementary Figure S138, Supplementary information) and the 13C NMR data (Table 1) analysis. Compared to 1 and 2, the chemical shift of C-7 (δC 48.9) and C-38 (δC 27.0) were shifted downfield. These findings suggested that 3 belonged to endo-BPAPs, which was confirmed by ROESY correlations of H2-32/H3-37 and H-7/H3-38. By comparison of NMR data of 3 with those of hyperattenin C and otogirinin D (Ishida et al., 2010) indicated that the chemical shifts of C-18, 19, 20, and 21 of 3 were consistent with those of hyperattenin C, suggesting the β-orientation of H-18. Many PPAPs with the isogeranyl group have been isolated from G. multiflora (Wang et al., 2018; Chen et al., 2019b; Teng et al., 2019). Owing to the characteristics of structural flexibility of the isogeranyl group, it is difficult to solve the absolute configuration of C-23 of the isogeranyl group by conventional structural elucidation methods. Thus, the relative configuration of compound 3 except C-23 was determined, named as garcimultinone F. The relative configuration of C-23 of 3 and its absolute configuration are discussed later together with compounds 5–7.
Compound 4 was obtained as a colorless oil and showed a pseudo molecular ion peak at m/z 549.3209 [M + H]+ (calcd 549.3211) in the HRESIMS, corresponding to the molecular formula C34H44O6. The 1H NMR and 13C NMR spectrum of 4 and hyperattenin D were highly similar, except for the additional methoxy and prenyl groups and the absence of ethoxy and geranyl groups in 4 (Li et al., 2015). HMBC correlations (Supplementary Figure S137, Supplementary information) from MeO to C-17 and H2-22 to C-5 and C-9 suggested that methoxy and prenyl groups were located at C-17 and C-5, respectively. The relative configuration of 4 was established as the same as that of hyperattenin D by ROESY spectrum (Figure 3) and 13C NMR data (Table 1). The absolute configuration of 4 was defined to be 1R, 5S, 7S, 17S, and 18S by the ECD calculation (Figure 4). Thus, the structure of compound 4 was elucidated, as shown in Figure 1, and named as garcimultinone G.
Compound 5 was obtained as colorless oil. The molecular formula of 5 was deduced to be C38H50O5 based on its negative-ion HRESIMS data at m/z 585.3587 [M - H]− (calcd for C38H49O5, 585.3586). Comparison of the NMR spectroscopic data (Tables 1, 2) of 5 with those of garcimultine A implied that they possessed a similar structure, except for the presence of an oxygenated tertiary carbon C-24 (δC 74.2) and a methyl [δH 1.19 (3H, s); δC 29.8] in 5, replacing the terminal double bond Δ24(26) in garcimultine A. These findings suggested that 5 was a Δ24(26)-hydrate of garcimultine A (Liu et al., 2017b), which was ascertained by HMBC cross-peaks (Figure 2) from H3-25/H3-26 to C-23 (δC 46.9) and C-24 (δC 74.2). Compound 5 was defined as endo-BPAPs based on the analyses of ROESY spectrum (Figure 3) and 13C-NMR data (Table 1). Therefore, the relative configuration of 5 except C-23 was defined and named as garcimultinone H.
Compound 6 isolated as a colorless oil and gave the molecular formula C38H50O4 as revealed by its HRESIMS at m/z 571.3778 [M + H]+ (calcd for C38H51O4, 571.3782). Comparison of the NMR data of 6 and hypersampsone T indicated that their structures were highly similar, except for the C-5 substituent. Obviously, isogeranyl NMR signals of 6 replaced those for a prenyl group in hypersampsone T (Tian et al., 2016). This deduction was further confirmed by HMBC correlations from H2-22 to C-4, C-5, C-9, C-23, and C-24 (Supplementary Figure S138, Supplementary information). Thus, the relative configuration of 6 except C-23 was defined and named as garcimultinone I.
Compound 7 were isolated as a colorless oil and gave the molecular formula C38H50O6, as revealed by its HRESIMS at m/z 603.3676 [M + H]+ (calcd C38H51O6, 603.3641), indicating 32 mass units more than 6. Comparison of NMR data (Tables 1, 2) of 6 and 7 showed many similarities with two major differences. Firstly, the presence of a 3,4-dihydroxybenzoyl group at C-1 in 7 instead of a benzoyl group at C-1 in 6 was observed in NMR data, which was further confirmed by HMBC spectrum (Supplementary Figure S138, Supplementary information). Secondly, the chemical shifts of C-7 and CH3-38 were both shifted upfield 6.2 and 11.2 ppm, respectively, compared to 6, suggesting to be exo-BPAPs which was further supported by ROESY spectrum. Thus, the relative configuration of 7 except C-23 was established, and named as garcimultinone J.
Compounds 3 and 5–7 all contain the isogeranyl group featuring a stereocenter at C-23, which is a challenge for the determination of the absolute configuration. Recently, the absolute configuration of C-23 of the isogeranyl of PPAPs bearing exocyclic stereocenters such as guttiferone F has been determined and revised by total synthesis and X-ray diffraction, and a preliminary conclusion has been drawn that BPAP-bearing exocyclic stereocenters from natural sources mostly carries S configuration of the isogeranyl. According to this rule, all (23R)-endo-type B BPAPs with a isogeranyl at C-5 are corrected to be (23S)-endo-type B BPAPs, including garcimultiflorones D–F, 18-hydroxygarcimultiflorone D, isogarcimultiflorone F, and garcimultiflorone J isolated from the same plant (Wang et al., 2021; Zheng et al., 2021). Combined with the biosynthetic pathway and this rule, the configuration of C-23 could be tentatively determined as S*. Therefore, the relative configuration of 3 and 5–7 was defined as (1R*, 5R*, 7R*, 18R*, and 23S*), (1S*, 5S*, 7R*, and 23S*), (1S*, 5R*, 7R*, and 23S*), and (1S*, 5R*, 7S*, and 23S*), respectively. Subsequently, by comparing the calculated ECD spectrum of compounds 3 and 5–7 with the experimental ECD spectrum, the absolute configurations of compounds 3 and 5–7 were determined as to be (1S, 5S, 7S, 18S, and 23R), (1R, 5R, 7S, and 23R), (1R, 5S, 7S, and 23R), and (1R, 5S, 7R, and 23R), respectively.
Type B BPAPs
Compound 8 was obtained as pale yellow oil. The HRESIMS data at m/z 617.3488 [M - H]− of 8 together with 13C-NMR and DEPT indicated molecular formula of C38H50O7. The 1H, 13C NMR, HSQC, and HMBC spectrum of 8 disclosed characteristic signals of the Type B BPAPs skeleton, including a methylene at δC 43.4 (C-6), a methine at δC 48.8 (C-7), three sp3 quaternary carbon at δC 69.3 (C-1), 61.9 (C-5), and 49.7 (C-8), a conjugated carbonyl carbon at δC 192.5 (C-10), a non-conjugated carbonyl carbon at δC 208.4 (C-9), an enolized 1,3-diketo group at δC 173.2 (C-2), 119.1 (C-3), and 192.5 (C-4), and HMBC correlations from Me-37 and Me-38 to C-8 (δC 49.7) and C-1 (δC 69.3). The NMR data (Tables 1, 3) of 8 was highly similar to those of hyperibone I except for the replacement of a prenyl group at C-5 in hyperibone I by a E-5-methyl-2-(1-methylethenyl)-5-hydroperoxy-hex-3-enyl group in 8 (Matsuhisa et al., 2002). The key HMBC correlations from Me-30 and Me-31 to C-28 and C-29, from Me-25 to C-23, C-24, and C-26, and from H2-22 to C-4, C-5, C-23, C-24, and C-27 (Figure 2), together with 1H-1H COSY of H2-22/H-23/H-27/H-28 confirmed this conclusion. The double bond of Δ27(28) was assigned the E configuration based on their coupling constant values 16.2 Hz (Zhang et al., 2016). By comparison of 13C-NMR data of 8 and hyperibone I suggested that the relative configurations C-1, C-5, and C-18 of 8 was consistent with hyperibone I, which was further confirmed by ROESY correlations H-18/H3-37 and H3-37/H2-32 (Figure 3). The determination of its absolute configuration will be discussed with compound 9.
Compound 9 was obtained as a white amorphous powder. The HRESIMS data at m/z 587.37262 [M + H]+ of 9 together with 13C-NMR and DEPT indicated molecular formula of C38H50O5. The NMR data of 9 was highly similar to those of 8 (Tables 1, 3), except for the presence of a prenyl group attached to C-23 in 9, instead of E-3-methyl-3-hydroperoxy-but-1-enyl group in 8. The key HMBC correlations from Me-30 and Me-31 to C-28 (δC 123.1) and C-29 (δC 131.9) confirmed this conclusion. Compound 9 was also determined as endo-type B BPAPs based on the chemical shift of C-7 (δC 48.9) and C-38 (δC 26.8) and ROESY correlation between Me-37 and H2-32. The NMR difference between compounds 8 and 9 mainly lied in the chemical shift and splitting of H-18 [(δH 4.03, t, J = 10.8 Hz) in 9 (δH 4.68, dd, J = 10.8, 7.8 Hz) in 8], suggesting the orientation of H-18 in 9 was opposite to 8, which was further confirmed by ROESY correlation of Me-37/Me-21. As with the above discussion of compounds 3 and 5–7, the relative configurations of compounds 8 and 9 could be determined as (1S*, 5R*, 7R*, 18R*, and 23R*) and (1S*, 5S*, 7R*, 18S*, and 23S*), respectively. Owing to the change of the priority order of functional groups at C-23, the relative configuration of C-23 of 8 changed from S* to R*. The calculated ECD spectrum of (1R, 5R, 7S, 18R, and 23R)-9b was in good agreement with the experimental ECD spectrum of 9, establishing the absolute configuration of 9 as 1R, 5R, 7S, 18R, and 23R (Figure 4). Owing to the similarity of experimental ECD curves between 8 and 9, the absolute configuration of 8 was defined as 1R, 5S, 7S, 18S, and 23S. Thus, the structures of 8 and 9 were established as depicted in Figure 1, and named as garcimultinones K and L, respectively.
Compound 10 was obtained as a white amorphous powder. The HRESIMS data at m/z 571.37780 [M + H]+ of 10, together with 13C-NMR and DEPT indicated molecular formula of C38H50O4. Its NMR data were highly similar to those of isogarcinol (Marti et al., 2010; Gustafson et al., 1992), except for the presence of a benzoyl group in 10, instead of 3,4-dihydroxybenzoyl group in isogarcinol. Thus, 10 was a 13,14-didehydoxy of isogarcinol, which was further confirmed by the HMBC correlations (Figure 2). In a previous report, 13,14-didehydoxyisogarcinol was isolated from the fruit of G. multiflora (Chen et al., 2009), which has been corrected to 13,14-didehydoxy-7-epi-isogarcinol (Yang et al., 2018). The relative configuration of 10 was deduced as the same as that of isogarcinol from the ROESY spectrum (Supplementary Figure S139, Supplementary information). In the experimental ECD spectrum, compound 10 showed positive Cotton effect (CE) at 220 nm and negative CE at 270 nm, establishing the absolute configuration of 10 as the same as that of isogarcinol (Socolsky and Plietker, 2015). This deduction was further confirmed by ECD calculations (Supplementary Figure S159, Supplementary information). Thus, the structures of 10 were established as 13,14-didehydoxyisogarcinol and named as garcimultinone M. Compared to 8 and 9, compound 10 display the different side chain orientations of the bicyclo[3.3.1]nonane moiety. However, it is noteworthy that the corresponding compounds show the same CD spectrum. Compounds 8–9 are the presence of an enolized C-2 via ether ring closure and C-4 keto form, while compound 10 is the presence of C-2 keto form and an enolized C-4 via ether ring closure. These findings could imply that the position of an enolized 1,3-diketo group in the core structure might affect the molecular conformation and hence the ECD curves (Le et al., 2016; Sukandar et al., 2020).
Caged PPAPs
The HRESIMS data of 11 displayed an [M + H]+ ion at m/z 619.3630 (calcd for 619.3629), corresponding to the molecular formula of C38H50O7. The 1H, 13C NMR, HSQC, and HMBC spectrum of 11 contained characteristic signals of a homo-adamantane PPAPs skeleton, including three non-conjugated carbonyls at δC 208.2 (C-4), 204.1 (C-2), and 204.3 (C-9), four quaternary carbons at δC 82.1 (C-1), 66.6 (C-3), 69.1 (C-5), and 48.7 (C-8), two methines at δC 43.3 (C-7) and 41.5 (C-33), two methylenes at δC 37.8 (C-6) and 28.8 (C-32), and HMBC correlations (Figure 2) from Me-37 and Me-38 to C-8 (δC 48.7), C-7 (δC 43.3), and C-1 (δC 82.1). Comparison of the NMR data of 11 with those of garcimultiflorone G disclosed that the planar structure of 11 was identical to that of garcimultiflorone G (Ting et al., 2014). Compared to garcimultiflorone G, the chemical shifts of C-6 and C-32 was shielded from δC 45.1 (C-6) and 31.6 (C-32) in garcimultiflorone G to δC 37.8 (C-6) and 28.8 (C-32) in 11. Therefore, H-33 was determined as β-oriented. The ΔδC between Me-35 and Me-36 was about 10 ppm, suggesting the relative configuration of H-18 and H-33 taken as cis-oriented (Ye et al., 2019). This deduction was further confirmed by ROESY spectrum (Figure 3). Therefore, the relative configuration of 11 except C-23 can be determined as (1S*, 3S*, 5R*, 7R*, 18S*, and 33S*). The carbon skeleton of 11 was different from the abovementioned compounds, and this rule might be not suitable for determining the configuration of C-23. Thus, The ECD calculations for (1S, 3S, 5R, 7R, 18S, 23S, and 33S)-11a, (1S, 3S, 5R, 7R, 18S, 23R, and 33S)-11b, and their enantiomers 11a′ and 11b′ were performed using the TDDFT/ECD method at the B3LYP/6–31+G(d) level. As a result, the calculated ECD curves of 11a′ and 11b′ matched well the experimental ECD spectra of 11 (Figure 4). Consequently, the absolute configuration of 11 except C-23 was established, as shown in Figure 1, and named as garcimultinone N.
MPAPs
Compound 12 was obtained as a white amorphous powder. The molecular formula of 12 was established as C33H42O4 by the 13C NMR, DEPT, and HRESIMS data at m/z [M + H]+ 503.3158 (calcd for C33H43O4, 503.3156). The 1H- and 13C-NMR spectra (Tables 1, 3) revealed the existence the characteristic signals of the phloroglucinol core, including one sp3 quaternary carbon at δC 52.7 (C-2), an enolized 1,3-diketo group at δC 196.3 (C-1), 107.6 (C-6), and 189.0 (C-5), and an enolic moiety at δC 171.9 (C-3) and 116.6 (C-4). Comparison of the NMR data 12 with those of hypelodin A indicated that the E-4-methylpent-1,3-dienyl group attached to C-9 in hypelodin A was replaced by the methyl group at C-9 in 12 (Hashida et al., 2014), which was further confirmed by HMBC correlations from Me-37 and Me-38 to C-8 (δC 41.0) and C-9 (δC 85.6). In the ROESY spectrum of 12 (Figure 3), the correlations of H-8/H2-22, H-8/H3-37, and H3-38/H2-17 demonstrated that H-8, 2-prenyl, and CH3-37 were cofacial and arbitrarily assigned as β-oriented. The calculated ECD spectrum of (2R and 8S)-12a matched well with the experimental ECD spectrum of 12 (Figure 4). Thus, the absolute configuration of 12 was established as (2R, 8S), and compound 12 was named as garcimultinone O.
Compound 13 was obtained as a white amorphous powder. The molecular formula of 13 was established as C38H50O4 by the 13C NMR, DEPT, and HRESIMS data at m/z [M + H]+ 571.3780 (calcd for C38H51O4, 571.3782). Comparison of the NMR data 13 with those of 12 indicated the presence of an isogeranyl group at C-2 in 13 as opposed to a prenyl group at C-2 in 12. However, the chemical shifts of C-2 and C-8 were shifted downfield from C-2 (δC 52.7) and C-8 (δC 41.0) in 12 to C-2 (δC 56.5) and C-8 (δC 44.2) in 13, suggesting that 8-prenyl and 2-isogeranyl groups were taken as cis relationship (Xu et al., 2019). ROESY correlations H-17/H2-22/Me-38 supported this deduction (Supplementary Figure S139, Supplementary information). The relative configuration of C-23 remained undetermined. Thus, the ECD calculations for (2R, 8R, and 23R)-13a, (2R, 8R, and 23S)-13b, and their enantiomers 13a′ and 13b′ were carried out. These result showed that the calculated ECD curves of 13a′ and 13b′ were in good agreement with experimental ECD data (Supplementary Figure S160, Supplementary information). Thus, the absolute configuration of 13 except C-23 was established as (2S and 8S), and compound 13 was named as garcimultinone P.
Compound 14 was obtained as a yellow powder, which possessed a molecular formula of C28H34O4 as determined by the HRESIMS data at m/z 435.2532 [M + H]+ (calcd for C28H35O4, 435.2530) in accordance with its 13C NMR data. The 1H- and 13C-NMR spectra (Tables 1 and 3) showed the characteristic signals for the phloroglucinol core, including three sp2 quaternary carbons at δC 104.6 (C-2), δC 100.5 (C-4), and δC 105.2 (C-6), three sp2 oxygenated quaternary carbons at δC 161.2 (C-1), δC 160.3 (C-3), and δC 154.5 (C-5), which constructed six substituted benzene ring. The 1H- and 13C-NMR data of 14 were very similar to those of vismiaguianones A (Seo et al., 2000), except for the presence of an additional prenyl group in 14 and the chemical shift of C-8 being shifted upfield from C-8 (δC 68.7) in vismiaguianones A to C-8 (δC 40.9) in 14. Thus, the hydroxy group in vismiaguianones A was replaced by the prenyl group in 14. This deduction was subsequently confirmed by the correlations of H2-22 (δH 2.10, 1.68) with C-7 (δC 22.1) and C-8 (δC 40.9) in the HMBC spectrum (Figure 2). The absolute configuration of 14 was established as 8S by comparing the calculated and experimental ECD data (Supplementary Figure S161, Supplementary information), and compound 14 was named as garcimultinone Q.
The ten known analogues were identified as garcimultiflorone A (15) (Chen et al., 2009), hyperscabrone M (16) (Gao et al., 2016), 13,14-didehydoxy-7-epi-isogarcinol (17) (Chen et al., 2009), xerophenone C (18) (Thoison et al., 2005), garcimultiflorone G (19) (Ting et al., 2014), garcimultiflorone P (20) and garcimultiflorone N (21) (Wang et al., 2018), garciniagifolone A (22) (Shan et al., 2012), garcibracteatone (23) (Thoison et al., 2005), and nemorosonol (24) (Oya et al., 2015).
All isolated compounds were evaluated for their inhibitory effects against the human T98, HepG2, and MCF-7 cancer cell lines by the CCK-8 method. Compounds 2 and 7 displayed evident antiproliferative activity against three tested cell lines (Supplementary Table S1, Supplementary information). The IC50 values of antiproliferative activities of compound 2 on T98, HepG2, and MCF-7 cancer cell lines were 13.23 ± 4.24, 13.53 ± 0.17, and 9.81 ± 1.56 μM, respectively. Compound 7 showed antiproliferative activity against T98, HepG2, and MCF-7 cancer cell lines with IC50 values of 17.00 ± 2.75, 12.84 ± 1.59, and 15.68 ± 1.65 μM, respectively. However, the other compounds were inactive showing IC50 values in excess of 20 μM. According to the structure type and biological activity of the isolated compounds, it could be preliminarily inferred that 3,4-dihydroxybenzoyl substituents in the structures of BPAPs are important for their anticancer activities (Yang et al., 2018).
Conclusion
In summary, the phytochemical investigation of the fruits of G. multiflora resulted in the isolation and structure elucidation of 24 structurally diverse polyprenylated acylphloroglucinols (PAPs) including 11 new PPAPs (1–11) and 3 new MPAPs (12–14). These PPAPs belong to three types including the bicyclic polyprenylated acylphloroglucinols (BPAPs, 1–10 and 15–18), the caged PPAPs (11 and 19–22), and the complicated PPAPs (23 and 24). Interestingly, most of PAPs are linked with the isogeranyl or its derivatives. The BPAPs with 3,4-dihydroxybenzoyl were found to exhibit effectively antiproliferative activity. These findings indicated that the fruits of G. multiflora are an important source of structural diversity PAPs, which deserve further study.
Data Availability Statement
The original contributions presented in the study are included in the article/Supplementary Material; further inquiries can be directed to the corresponding authors.
Author Contributions
YC and GY conceived, designed the experiments, were responsible for structure elucidation, and revised the manuscript. HT carried out the isolation of compounds, NMR, and ECD calculation, and wrote the original draft. QL contributed to cytotoxicity testing. ZM carried out the isolation of compounds. XL and WX carried out the experiments and data analyses. All authors have read and approved the published version of the manuscript.
Funding
This work was financially supported by the National Key Research and Development Program of China (2018YFC1708004), the Major Scientific and Technological Project of Hubei Province (2020ACA007), and the Special Fund for Basic Scientific Research of Central Colleges, South-Central University for Nationalities (CZP18004)
Conflict of Interest
The authors declare that the research was conducted in the absence of any commercial or financial relationships that could be construed as a potential conflict of interest.
Publisher’s Note
All claims expressed in this article are solely those of the authors and do not necessarily represent those of their affiliated organizations, or those of the publisher, the editors and the reviewers. Any product that may be evaluated in this article, or claim that may be made by its manufacturer, is not guaranteed or endorsed by the publisher.
Supplementary Material
The Supplementary Material for this article can be found online at: https://www.frontiersin.org/articles/10.3389/fchem.2021.756452/full#supplementary-material
References
Chen, J.-J., Ting, C.-W., Hwang, T.-L., and Chen, I.-S. (2009). Benzophenone Derivatives from the Fruits of Garcinia Multiflora and Their Anti-inflammatory Activity. J. Nat. Prod. 72, 253–258. doi:10.1055/s-0029-123498710.1021/np8006364
Chen, Y., Ma, Z., Teng, H., Gan, F., Xiong, H., Mei, Z., et al. (2019a). Adamantyl and Homoadamantyl Derivatives from Garcinia Multiflora Fruits. RSC Adv. 9, 12291–12299. doi:10.1039/c9ra01279f
Chen, Y., Ma, Z., Teng, H., Gan, F., Xiong, H., Mei, Z., et al. (2019b). Four Intricately Caged Polycyclic Polyprenylated Acylphloroglucinols from Garcinia Multiflora Fruits. Org. Chem. Front. 6, 3085–3092. doi:10.1039/c9qo00586b
Cheng, L.-Y., Chen, C.-L., Kuo, Y.-H., Chang, T.-H., Lin, I.-W., Wang, S.-W., et al. (2018a). Polyprenylated Polycyclic Acylphloroglucinol: Angiogenesis Inhibitor from Garcinia Multiflora. Bioorg. Med. Chem. Lett. 28, 1860–1863. doi:10.1016/j.bmcl.2018.04.006
Cheng, L.-Y., Tsai, Y.-C., Fu, S.-L., Cheng, M.-J., Sung, P.-J., Chung, M.-I., et al. (2018b). Acylphloroglucinol Derivatives from Garcinia Multiflora with Anti-inflammatory Effect in LPS-Induced RAW264.7 Macrophages. Molecules 23, 2587–2398. doi:10.3390/molecules23102587
Chien, S.-C., Chyu, C.-F., Chang, I.-S., Chiu, H.-L., and Kuo, Y.-H. (2008). A Novel Polyprenylated Phloroglucinol, Garcinialone, from the Roots of Garcinia Multiflora. Tetrahedron Lett. 49, 5276–5278. doi:10.1016/j.tetlet.2008.06.102
Ciochina, R., and Grossman, R. B. (2006). Polycyclic Polyprenylated Acylphloroglucinols. Chem. Rev. 106, 3963–3986. doi:10.1021/cr0500582
Fan, Y.-M., Yi, P., Li, Y., Yan, C., Huang, T., Gu, W., et al. (2015). Two Unusual Polycyclic Polyprenylated Acylphloroglucinols, Including a Pair of Enantiomers from Garcinia Multiflora. Org. Lett. 17, 2066–2069. doi:10.1021/acs.orglett.5b00588
Fan, Y. M., Tian, D. S., Wei, G., Huang, L. J., Yuan, C. M., and Hao, X. J. (2016). Chemical Constituents from the Leaves and Twigs of Garcinia Multiflora and Their Cytotoxic as Well as Anti-inflammatory Activity. Nat. Prod. Res. Dev. 28, 222–227. doi:10.16333/j.1001-6880.2016.2.009
Fu, W., Wu, M., Zhu, L., Lao, Y., Wang, L., Tan, H., et al. (2015). Prenylated Benzoylphloroglucinols and Biphenyl Derivatives from the Leaves of Garcinia Multiflora Champ. RSC Adv. 5, 78259–78267. doi:10.1039/c5ra09213b
Gao, W., Hu, J.-W., Xu, F., Wei, C.-J., Shi, M.-J., Zhao, J., et al. (2016). Polyisoprenylated Benzoylphloroglucinol Derivatives from Hypericum Scabrum. Fitoterapia 115, 128–134. doi:10.1016/j.fitote.2016.10.003
Gustafson, K. R., Blunt, J. W., Munro, M. H. G., Fuller, R. W., Mckee, T. C., Cardellina, J. H., et al. (1992). The Guttiferones, HIV-Inhibitory Benzophenones from Symphonia Globulifera, Garcinia Livingstonei, Garcinia Ovalifolia and Clusia Rosea. Tetrahedron 48, 10093–10102. doi:10.1016/S0040-4020(01)89039-6
Hashida, C., Tanaka, N., Kawazoe, K., Murakami, K., Sun, H.-D., Takaishi, Y., et al. (2014). Hypelodins A and B, Polyprenylated Benzophenones from Hypericum Elodeoides. J. Nat. Med. 68, 737–742. doi:10.1007/s11418-014-0853-9
Ishida, Y., Shirota, O., Sekita, S., Someya, K., Tokita, F., Nakane, T., et al. (2010). Polyprenylated Benzoylphloroglucinol-type Derivatives Including Novel Cage Compounds from Hypericum Erectum. Chem. Pharm. Bull. 58, 336–343. doi:10.1248/cpb.58.336
Le, D. H., Nishimura, K., Takenaka, Y., Mizushina, Y., and Tanahashi, T. (2016). Polyprenylated Benzoylphloroglucinols with DNA Polymerase Inhibitory Activity from the Fruits of Garcinia Schomburgkiana. J. Nat. Prod. 79, 1798–1807. doi:10.1021/acs.jnatprod.6b00255
Li, D., Xue, Y., Zhu, H., Li, Y., Sun, B., Liu, J., et al. (2015). Hyperattenins A-I, Bioactive Polyprenylated Acylphloroglucinols from Hypericum Attenuatum Choisy. RSC Adv. 5, 5277–5287. doi:10.1039/c4ra11675e
Liu, B., Zhang, X., Bussmann, R. W., Hart, R. H., Li, P., Bai, Y., et al. (2017a). Garcinia in Southern china: Ethnobotany, Management, and Niche Modeling. Econ. Bot. 70, 416–430. doi:10.1007/s12231-016-9360-0
Liu, H., Gan, F., Jin, S., Li, J., Chen, Y., and Yang, G. (2017b). Acylphloroglucinol and Tocotrienol Derivatives from the Fruits of Garcinia Multiflora. RSC Adv. 7, 29295–29301. doi:10.1039/c7ra04208f
Liu, X., Yu, T., Gao, X.-M., Zhou, Y., Qiao, C.-F., Peng, Y., et al. (2010). Apoptotic Effects of Polyprenylated Benzoylphloroglucinol Derivatives from the Twigs of Garcinia Multiflora. J. Nat. Prod. 73, 1355–1359. doi:10.1021/np100156w
Marti, G., Eparvier, V., Moretti, C., Prado, S., Grellier, P., Hue, N., et al. (2010). Antiplasmodial Benzophenone Derivatives from the Root Barks of Symphonia Globulifera (Clusiaceae). Phytochemistry 71, 964–974. doi:10.1016/j.phytochem.2010.03.008
Matsuhisa, M., Shikishima, Y., Takaishi, Y., Honda, G., Ito, M., Takeda, Y., et al. (2002). Benzoylphloroglucinol Derivatives from Hypericum Scabrum. J. Nat. Prod. 65, 290–294. doi:10.1021/np010310a
Oya, A., Tanaka, N., Kusama, T., Kim, S.-Y., Hayashi, S., Kojoma, M., et al. (2015). Prenylated Benzophenones from Triadenum Japonicum. J. Nat. Prod. 78, 258–264. doi:10.1021/np500827h
Phang, Y., Wang, X., Lu, Y., Fu, W., Zheng, C., and Xu, H. (2020). Bicyclic Polyprenylated Acylphloroglucinols and Their Derivatives: Structural Modification, Structure-Activity Relationship, Biological Activity and Mechanism of Action. Eur. J. Med. Chem. 205, 112646–112673. doi:10.1016/j.ejmech.2020.112646
Seo, E.-K., Wani, M. C., Wall, M. E., Navarro, H., Mukherjee, R., Farnsworth, N. R., et al. (2000). New Bioactive Aromatic Compounds from Vismia Guianensis. Phytochemistry 55, 35–42. doi:10.1016/S0031-9422(00)00208-9
Shan, W.-G., Lin, T.-S., Yu, H.-N., Chen, Y., and Zhan, Z.-J. (2012). Polyprenylated Xanthones and Benzophenones from the Bark of Garcinia Oblongifolia. Hca 95, 1442–1448. doi:10.1002/hlca.201200019
Socolsky, C., and Plietker, B. (2015). Total Synthesis and Absolute Configuration Assignment of MRSA Active Garcinol and Isogarcinol. Chem. Eur. J. 21, 3053–3061. doi:10.1002/chem.201406077
Sukandar, E. R., Kaennakam, S., Aree, T., Nöst, X., Rassamee, K., Bauer, R., et al. (2020). Picrorhizones A-H, Polyprenylated Benzoylphloroglucinols from the Stem Bark of Garcinia Picrorhiza. J. Nat. Prod. 83, 2102–2111. doi:10.1021/acs.jnatprod.9b01106
Teng, H., Ma, Z., Teng, H., Du, Y., Chen, X., Chen, Y., et al. (2021). Two Novel Cyclohexanone-Monocyclic Polycyclic Polyprenylated Acylphloroglucinols from Garcinia Multiflora Fruits. Nat. Product. Res. doi:10.1080/14786419.2020.1788559
Teng, H., Ren, Y., Ma, Z., Tan, X., Xu, J., Chen, Y., et al. (2019). Homoadamantane Polycyclic Polyprenylated Acylphloroglucinols from the Fruits of Garcinia Multiflora. Fitoterapia 137, 104245–104251. doi:10.1016/j.fitote.2019.104245
Thoison, O., Cuong, D. D., Gramain, A., Chiaroni, A., Hung, N. V., and Sévenet, T. (2005). Further Rearranged Prenylxanthones and Benzophenones from Garcinia Bracteata. Tetrahedron 61, 8529–8535. doi:10.1016/j.tet.2005.05.091
Tian, D. S., Yi, P., Xia, L., Xiao, X., Fan, Y. M., Gu, W., et al. (2016b). Garmultins A-G, Biogenetically Related Polycyclic Acylphloroglucinols from Garcinia Multiflora. Org. Lett. 18, 5904–5907. doi:10.1021/acs.orglett.6b03004
Tian, W.-J., Qiu, Y.-Q., Jin, X.-J., Chen, H.-F., Yao, X.-J., Dai, Y., et al. (2016a). Hypersampsones S-W, New Polycyclic Polyprenylated Acylphloroglucinols from Hypericum Sampsonii. RSC Adv. 6, 50887–50894. doi:10.1039/c5ra26332h
Ting, C.-W., Hwang, T.-L., Chen, I.-S., Cheng, M.-J., Sung, P.-J., Yen, M.-H., et al. (2014). Garcimultiflorone G, a Novel Benzoylphloroglucinol Derivative fromGarcinia Multiflorawith Inhibitory Activity on Neutrophil Pro-inflammatory Responses. Chem. Biodiversity 11, 819–824. doi:10.1002/cbdv.201300278
Ting, C.-W., Hwang, T.-L., Chen, I.-S., Yen, M.-H., and Chen, J.-J. (2012). A New Benzoylphloroglucinol Derivative with an Adamantyl Skeleton and Other Constituents from Garcinia Multiflora: Effects on Neutrophil Pro-inflammatory Responses. Chem. Biodiversity 9, 99–105. doi:10.1002/cbdv.201100006
Wang, X., Phang, Y., Feng, J., Liu, S., Zhang, H., and Fu, W. (2021). Stereodivergent Strategy in Structural Determination: Asymmetric Total Synthesis of Garcinol, Cambogin, and Related Analogues. Org. Lett. 23, 4203–4208. doi:10.1021/acs.orglett.1c01139Xu
Wang, Z.-Q., Li, X.-Y., Hu, D.-B., and Long, C.-L. (2018). Cytotoxic Garcimultiflorones K-Q, Lavandulyl Benzophenones from Garcinia Multiflora Branches. Phytochemistry 152, 82–90. doi:10.1016/j.phytochem.2018.04.019
Xu, H., Lin, X., Xu, G., and Zheng, C. (2017). Studies on the Chemistry and Bioactivities of Chinese Garcinia Plants. Shanghai: Shanghai Scientific and Technical Publisher, 9–10.
Xu, W.-J., Tang, P.-F., Lu, W.-J., Zhang, Y.-Q., Wang, X.-B., Zhang, H., et al. (2019). Hyperberins A and B, Type B Polycyclic Polyprenylated Acylphloroglucinols with Bicyclo[5.3.1]hendecane Core from Hypericum Beanii. Org. Lett. 21, 8558–8562. doi:10.1021/acs.orglett.9b03098
Yang, X.-W., Grossman, R. B., and Xu, G. (2018). Research Progress of Polycyclic Polyprenylated Acylphloroglucinols. Chem. Rev. 118, 3508–3558. doi:10.1021/acs.chemrev.7b00551
Ye, Y., Yang, X.-W., Zhou, Y., and Xu, G. (2019). Homo-Adamantane Type Polycyclic Polyprenylated Acylphloroglucinols from Hypericum Hookerianum. Fitoterapia 133, 43–50. doi:10.1016/j.fitote.2018.12.014
Zhang, H., Zheng, D., Ding, Z.-J., Lao, Y.-Z., Tan, H.-S., and Xu, H.-X. (2016). UPLC-PDA-QTOFMS-guided Isolation of Prenylated Xanthones and Benzoylphloroglucinols from the Leaves of Garcinia Oblongifolia and Their Migration-Inhibitory Activity. Sci. Rep. 6, 35789–35800. doi:10.1038/srep35789
Keywords: Garcinia multiflora, Garcinia, polyprenylated acylphloroglucinols, NMR calculations, antiproliferative activity
Citation: Teng H, Li Q, Ma Z, Li X, Xie W, Chen Y and Yang G (2021) Polyprenylated Acylphloroglucinols With Different Carbon Skeletons From the Fruits of Garcinia multiflora. Front. Chem. 9:756452. doi: 10.3389/fchem.2021.756452
Received: 10 August 2021; Accepted: 21 September 2021;
Published: 26 October 2021.
Edited by:
Xiaoxiao Huang, Shenyang Pharmaceutical University, ChinaReviewed by:
Wen-Yu Zhao, Dalian Medical University, ChinaLe Zhou, South China Sea Institute of Oceanology, (CAS), China
Copyright © 2021 Teng, Li, Ma, Li, Xie, Chen and Yang. This is an open-access article distributed under the terms of the Creative Commons Attribution License (CC BY). The use, distribution or reproduction in other forums is permitted, provided the original author(s) and the copyright owner(s) are credited and that the original publication in this journal is cited, in accordance with accepted academic practice. No use, distribution or reproduction is permitted which does not comply with these terms.
*Correspondence: Yu Chen, chenyuwh888@126.com; Guangzhong Yang, yanggz888@126.com
†These authors have contributed equally to this work