- College of Chemistry and Materials Engineering, Wenzhou University, Wenzhou, China
An efficient enantioselective NMR analysis of atropisomeric quinolines in the promotion of chiral phosphoric acid is described, in which a variety of racemic 4-aryl quinolines were well-recognized with up to 0.17 ppm ΔΔδ value. Additionally, the optical purities of different nonracemic substrates could be evaluated fast via NMR analysis with high accuracy.
Introduction
Axial chirality is one of the important types of molecular asymmetry created from restriction of carbon–carbon or carbon–nitrogen single-bond rotation. Since Christie and Kenner reported the first detection of atropisomerism in 1922 (Christie and Kenner, 1922), axial chirality was found in a lot of natural products and pharmaceutical compounds as exemplified by michellamines (Manfredi et al., 1991; Bringmann et al., 1993) and vancomycin(Nicolaou et al., 1999). Besides, many chiral ligands and catalysts, such as BINOL, BINAP, and phosphoric acids, have been developed based on axially chiral biaryl scaffolds(Miyashita et al., 1980; Akutagawa, 1995; Kumobayashi et al., 2001; Brunel, 2005; Brunel, 2007; Genet et al., 2014). It is well-known that the enantiopurities of chiral ligands and catalysts are critical to their enantiocontrol, and atropisomers of bioactive molecules always exhibit different pharmacodynamic and pharmacokinetic behavior both in vivo and in vitro (Eichelbaum and Gross, 1996; Clayden et al., 2009). Thus, the development of efficient methods to recognize and determine atropisomeric compounds becomes an interesting target and is always in high demand. As key analysis methods, GC (Schurig and Nowotny, 1990), IR (Reetz et al., 1998), HPLC (Han, 1997), circular dichroism (Ding et al., 1999; Nieto et al., 2008; Ghosn and Wolf, 2009; Nieto et al., 2010), fluorescence spectroscopy (James et al., 1995; Mei and Wolf, 2004; Pu, 2004; Zhao et al., 2004; Tumambac and Wolf, 2005; Liu et al., 2009), electrophoresis technologies (Reetz et al., 2000), and NMR spectroscopy have been efficiently employed in chiral determinations. Among these classic technologies, NMR analysis affords an ideal platform to explore efficient chiral analysis strategies because of its mild condition, easy operation, fast evaluation, high sample tolerance, etc. Over the past few decades, a lot of chiral shift reagents (CSRs) (Frazer et al., 1971; Goering et al., 1971; Yeh et al., 1986; Ghosh et al., 2004; Yang et al., 2005; Mori et al., 2013) or chiral solvating reagents (CSAs) (Pirkle, 1966; Lancelot et al., 1969; Parker, 1991; Wenzel and Wilcox, 2003; Seco et al., 2004; Lovely and Wenzel, 2006; Ema et al., 2007; Wenzel, 2007; Iwaniuk and Wolf, 2010; Moon et al., 2010; Gualandi et al., 2011; Pham and Wenzel, 2011; Quinn et al., 2011; Wenzel and Chisholm, 2011; Ma et al., 2012; Labuta et al., 2013; Zhou et al., 2015; Bian et al., 2016a; Akdeniz et al., 2016; Bian et al., 2016b; Huang et al., 2016) were successfully designed and employed in chiral NMR analysis. Encouraged by these achievements and our continuous efforts to study chiral interactions, we were particularly interested in exploring a novel NMR-based chiral analysis method for our synthetic targets: In 2017, we reported an enantioselective NMR analysis of indoloquinazoline alkaloid–type tertiary alcohols with chiral phosphoric acid (CPA) (Akiyama et al., 2006; Akiyama, 2007; Akiyama and Mori, 2015) promotion, in which a fast reaction condition optimization of amino acid metal salt–catalyzed asymmetric aldol reaction was also achieved (Liu et al., 2017); besides, a variety of racemic 4-aryl quinazolinones, such as afloqualone and IC-87114, were also well-recognized, and the optical purities of different nonracemic substrates could be evaluated fast with high accuracy (Wu et al., 2018). Encouraged by these results and our recent research on the catalytic asymmetric construction of atropisomeric quinolines, we wish to report an efficient chiral recognition of quinoline atropisomers by chiral phosphoric acid: In the presence of 1 equivalent of α-naphthyl phosphoric acid, a variety of racemic quinolines were well-recognized with up to 0.17 ppm ΔΔδ value; additionally, the corresponding analysis system can also be employed in the accurate determination of enantioselectivities of axial chiral quinolines.
Results and Discussion
As shown in Figure 1, the methyl peak on the benzyl position of racemic 1-(6-chloro-4-(2-fluorophenyl)-2-methylquinolin-3-yl) ethan-1-one 1a is unimodal on 1H NMR spectrum in the absence of chiral phosphoric acid. Generally, the addition of 1 equivalent of chiral phosphoric acid brought obvious chemical shift nonequivalences of this methyl peak of 1a, suggesting the strong chiral interaction between chiral phosphoric acids and 4-aryl quinoline. It was shown that the substituents on phosphoric acids had obvious influence on the recognition. For example, 3,3’-α-naphthyl–substituted phosphoric acid C1 afforded a baseline resolution and the largest chemical shift nonequivalence (ΔΔδ = 0.03) of a methyl H signal of 1a in CD3OD at 25°С, while 3,3’-phenyl–substituted phosphoric acid C7 failed to differentiate atropisomers of 1a. Besides, deuterated solvents also played an important role in chiral recognition. As shown in Table 1, chemical shift nonequivalence of methyl H of 1a’s atropisomers was observed when CPA C1 and 1a were combined in CD2Cl2, acetone-D6, CD3CN, and C6D6, while highly polar solvent, such as DMF-D7 and DMSO-D6, seemed to break the interaction between the chiral sensor and analyte, resulting in no differentiation of atropisomers. Besides, different peaks overlapped together when CDCl3 was employed as solvent. Significantly, C6D6 enabled the best chiral recognition of up to ΔΔδ 0.1 ppm, albeit with poor solubility of CPA and quinoline analytes. Considering the fact that CPA and quinoline mixture dissolve well in CDCl3, binary solvents of CD3OD and CDCl3 (5/1) were chosen as analysis media in the purpose of balancing solubility and recognition, offering eminent solubility and baseline resolution (entry 16). Additionally, the amount of 1a also influenced differentiation; for example, baseline resolution was not achieved when a 0.5 equivalent of chiral phosphoric acid C1 was used, while increasing the amount of C1 to 2 equivalent resulted in larger chemical shift nonequivalence (ΔΔδ = 0.05). Finally, under the balance of atom economy and recognition, 1 equivalent of (R)-C1 was employed as a chiral sensor (entry 17).
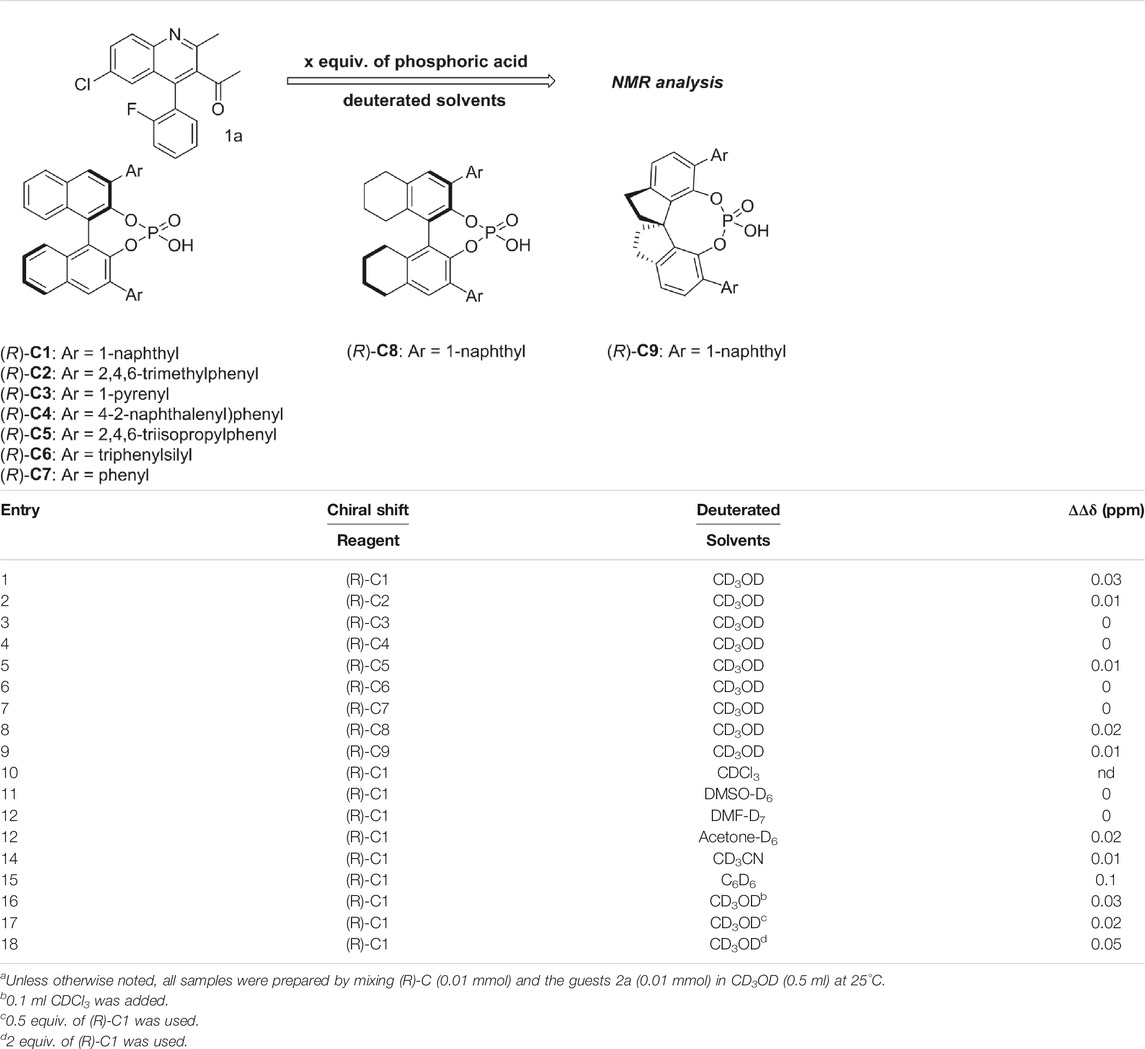
TABLE 1. Evaluating the chiral recognition abilities of chiral phosphoric acids (R)-C with 1a.a
Under optimized conditions, a series of 4-aryl quinoline guests were tested. First, the influence of substituents on quinoline (ring 1) was evaluated. It was shown that different electron-withdrawing groups on ring 1 were fit well under standard conditions, providing baseline resolutions and 0.02–0.17 ppm ΔΔδ values, respectively (Table 2, entries 1–5). Besides, different R3 groups on quinoline such as acetyl, ethyl formate, methyl formate and trifluoroacetyl were also tested, all of which led to clear recognition of atropisomers with up to 0.07 ppm ΔΔδ values. Subsequently, different 4-aryl groups (ring 2) were also studied. As shown in Table 2, a variety of electron-withdrawing or electron-donating groups on ring 2 were well-tolerated, and substituents with either moderate or bulky size on the 2’-position of ring 2 all resulted in clear baseline resolution with good chemical shift nonequivalences. Noticeably, when 1-{4-[(1,1’-biphenyl)-2-yl]-2-methylquinolin-3-yl} ethan-1-one 1g was employed as analyte, the largest chemical shift nonequivalence of 0.17 ppm ΔΔδ was obtained. Interestingly, when 1k-1n were employed as guests, obvious split peaks on α-H of oxygen were observed. It is also worth noting that nitro-substituted substrates 1b and 1g also afforded good differentiation results (chemical shift nonequivalence of 0.11 and 0.17 ppm ΔΔδ, respectively), possibly due to the steric hindrance effect of nitro group.
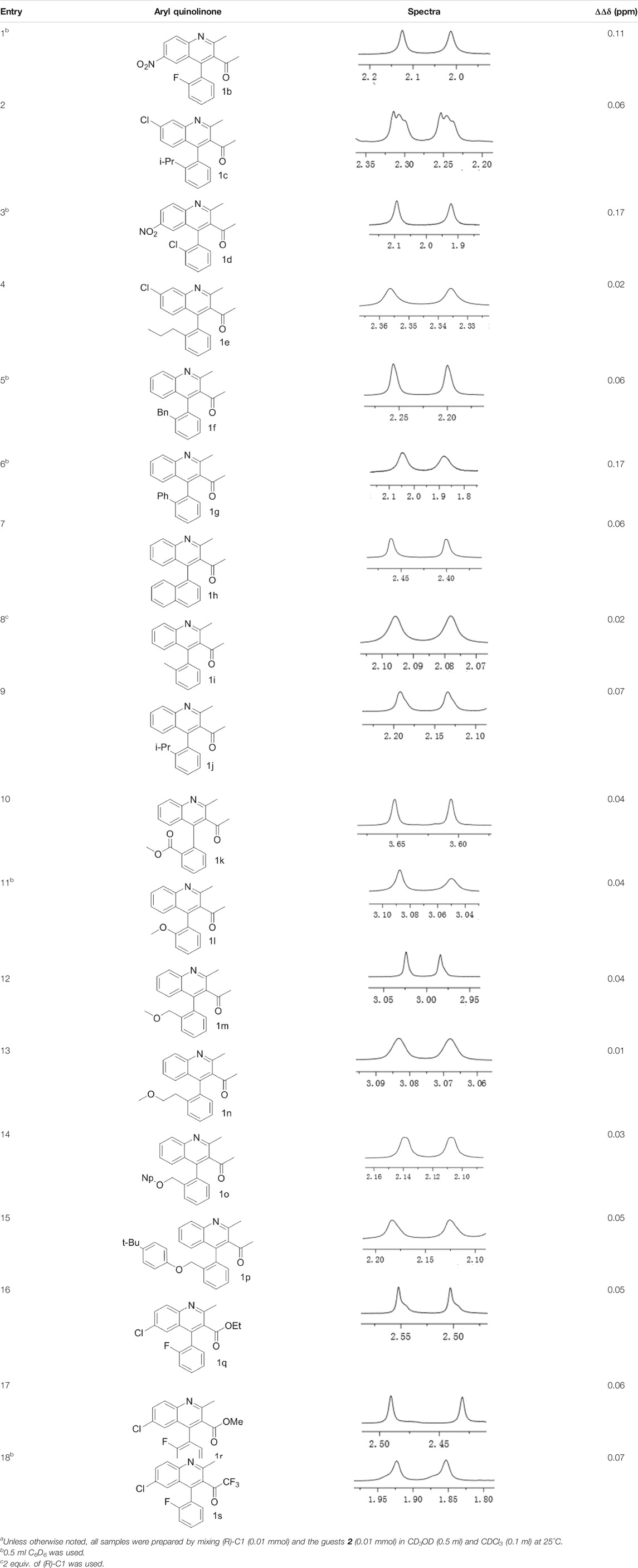
TABLE 2. Measurements of 1H chemical shift nonequivalences (DDd) of racemic aryl quinolinones.a
With this optimal recognition condition, the possibility of our methodology in the enantiomeric determination of various nonracemic 1j samples was explored. As shown in Figure 2, 1j samples with different enantiopurities was combined with 1 equivalent of CPA C1 and then monitored by NMR. It was revealed that the optical purities of 2a could be accurately obtained by integrating the corresponding H signals of the methyl group of 1j, which were very close to the exact results measured by HPLC. Compared with those data obtained from chiral HPLC analysis, an excellent linear relationship of a correlation coefficient R2 0.9996 and up to 0.03% absolute error was obtained.
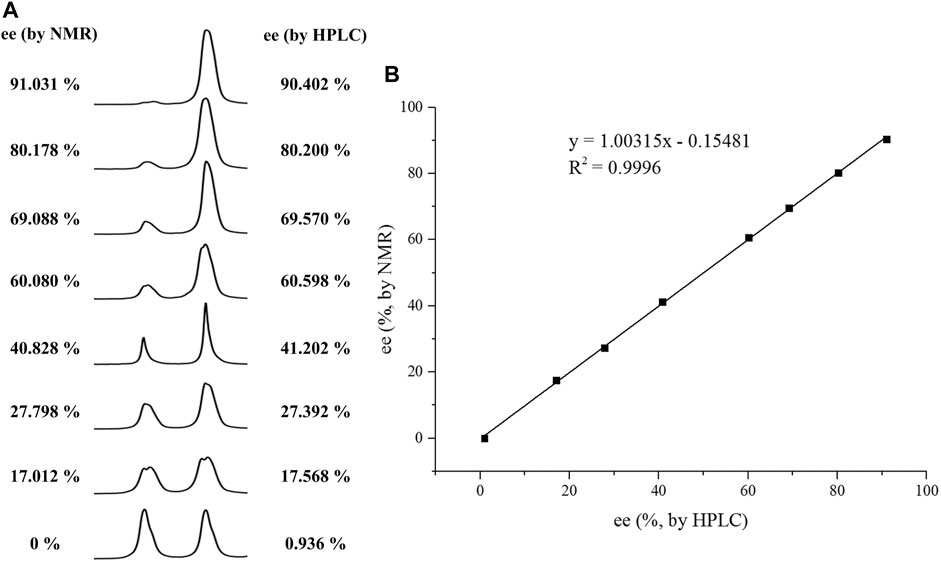
FIGURE 2. (A) Selected regions of the 1H NMR spectra of nonracemic aryl quinolinone samples (varied ee values) with (R)-C1 in 0.5 ml CD3OD and 0.1 ml CDCl3; (B) linear correlation between ee values determined by HPLC and NMR ee values, R2 = correlation coefficient.
Conclusion
In conclusion, an efficient phosphoric acid–promoted chiral recognition of atropisomeric quinolines via NMR analysis was successfully developed. With this method, atropisomers of various quinolines were well-discriminated with base resolution; besides, the optical purities of different nonracemic quinoline 1j could be evaluated fast with high accuracy. This method broadens the chiral analysis ability of chiral phosphoric acids, which encourages us to further explore the interaction of chiral acids with different analytes.
Data Availability Statement
The original contributions presented in the study are included in the article/Supplementary Material, further inquiries can be directed to the corresponding author.
Author Contributions
All authors listed have made a substantial, direct and intellectual contribution to the work, and approved it for publication.
Funding
This research was financially supported by Major Research Plan of Wenzhou City (No. ZG2017027).
Conflict of Interest
The authors declare that the research was conducted in the absence of any commercial or financial relationships that could be construed as a potential conflict of interest.
Supplementary Material
The Supplementary Material for this article can be found online at: https://www.frontiersin.org/articles/10.3389/fchem.2021.672704/full#supplementary-material
References
Akdeniz, A., Minami, T., Watanabe, S., Yokoyama, M., Ema, T., and Anzenbacher, P. (2016). Determination of Enantiomeric Excess of Carboxylates by Fluorescent Macrocyclic Sensors. Chem. Sci. 7, 2016–2022. doi:10.1039/C5SC04235F
Akiyama, T., and Mori, K. (2015). Stronger Brønsted Acids: Recent Progress. Chem. Rev. 115, 9277–9306. doi:10.1021/acs.chemrev.5b00041
Akiyama, T., Itoh, J., and Fuchibe, K. (2006). Recent Progress in Chiral Brønsted Acid Catalysis. Adv. Synth. Catal. 348, 999–1010. 10.1002/adsc.200606074
Akutagawa, S. (1995). Asymmetric Synthesis by Metal BINAP Catalysts. Appl. Catal. A: Gen. 128, 171–207. doi:10.1016/0926-860X(95)00097-6
Bian, G., Yang, S., Huang, H., Zong, H., and Song, L. (2016a). A Bisthiourea-Based 1 H NMR Chiral Sensor for Chiral Discrimination of a Variety of Chiral Compounds. Sensors Actuators B: Chem. 231, 129–134. doi:10.1016/j.snb.2016.03.002
Bian, G., Yang, S., Huang, H., Zong, H., Song, L., Fan, H., et al. (2016b). Chirality Sensing of Tertiary Alcohols by a Novel strong Hydrogen-Bonding Donor - Selenourea. Chem. Sci. 7, 932–938. doi:10.1039/C5SC03780H
Bringmann, G., Zagst, R., Schäffer, M., Hallock, Y. F., Cardellina, J. H., and Boyd, M. R. (1993). The Absolute Configuration of Michellamine B, a "Dimeric", Anti-HIV-active Naphthylisoquinoline Alkaloid. Angew. Chem. Int. Ed. Engl. 32, 1190–1191. doi:10.1002/anie.199311901
Brunel, J. M. (2005). BINOL: a Versatile Chiral Reagent. Chem. Rev. 105, 857–898. doi:10.1021/cr040079g
Brunel, J. M. (2007). Update 1 of: BINOL: a Versatile Chiral Reagent. Chem. Rev. 107, PR1–PR45. doi:10.1021/cr078004a
Christie, G. H., and Kenner, J. (1922). LXXI.-The Molecular Configurations of Polynuclear Aromatic Compounds. Part I. The Resolution of γ-6 : 6′-dinitro- and 4 : 6 : 4′ : 6′-Tetranitro-Diphenic Acids into Optically Active Components. J. Chem. Soc. Trans. 121, 614–620. doi:10.1039/CT9222100614
Clayden, J., Moran, W. J., Edwards, P. J., and LaPlante, S. R. (2009). The challenge of Atropisomerism in Drug Discovery. Angew. Chem. Int. Ed. 48, 6398–6401. doi:10.1002/anie.200901719
Ding, K., Ishii, A., and Mikami, K. (1999). Super High Throughput Screening (SHTS) of Chiral Ligands and Activators: Asymmetric Activation of Chiral Diol-Zinc Catalysts by Chiral Nitrogen Activators for the Enantioselective Addition of Diethylzinc to Aldehydes. Angew. Chem. Int. Ed. 38, 497–501. doi:10.1002/(sici)1521-3773(19990215)38:4<497:aid-anie497>3.0.co;2-g
Eichelbaum, M., and Gross, A. S.(1996). Stereochemical Aspects of Drug Action and Disposition. Adv. Drug Res. 28, 1–64. doi:10.1016/S0065-2490(96)80003-7
Ema, T., Tanida, D., and Sakai, T. (2007). Versatile and Practical Macrocyclic Reagent with Multiple Hydrogen-Bonding Sites for Chiral Discrimination in NMR. J. Am. Chem. Soc. 129, 10591–10596. doi:10.1021/ja073476s
Frazer, R. R., Petit, M. A., and Saunders, J. K. (1971). Determination of Enantiomeric Purity by an Optically Active Nuclear Magnetic Resonance Shift Reagent of Wide Applicability. J. Chem. Soc. Chem. Commun., 1971, 1450–1451. doi:10.1039/c29710001450
Genet, J.-P., Ayad, T., and Ratovelomanana-Vidal, V. (2014). Electron-deficient Diphosphines: the Impact of DIFLUORPHOS in Asymmetric Catalysis. Chem. Rev. 114, 2824–2880. doi:10.1021/cr4003243
Ghosh, I., Zeng, H., and Kishi, Y. (2004). Application of Chiral Lanthanide Shift Reagents for Assignment of Absolute Configuration of Alcohols. Org. Lett. 6, 4715–4718. doi:10.1021/ol048061f
Ghosn, M. W., and Wolf, C. (2009). Chiral Amplification with a Stereodynamic Triaryl Probe: Assignment of the Absolute Configuration and Enantiomeric Excess of Amino Alcohols. J. Am. Chem. Soc. 131, 16360–16361. doi:10.1021/ja907741v
Goering, H. L., Eikenberry, J. N., and Koermer, G. S. (1971). Tris[3-(trifluoromethylhydroxymethylene)-d-camphorato]Europium(III). Chiral Shift Reagent for Direct Determination of Enantiomeric Compositions. J. Am. Chem. Soc. 93, 5913–5914. doi:10.1021/ja00751a065
Gualandi, A., Grilli, S., Savoia, D., Kwit, M., and Gawroński, J. (2011). C-hexaphenyl-substituted Trianglamine as a Chiral Solvating Agent for Carboxylic Acids. Org. Biomol. Chem. 9, 4234–4241. doi:10.1039/c0ob01192d
Han, S. M. (1997). Direct Enantiomeric Separations by High Performance Liquid Chromatography Using Cyclodextrins. Biomed. Chromatogr. 11, 259–271. doi:10.1002/(sici)1099-0801(199709)11:5<259:aid-bmc701>3.0.co;2-u
Huang, H., Bian, G., Zong, H., Wang, Y., Yang, S., Yue, H., et al. (2016). Chiral Sensor for Enantiodiscrimination of Varied Acids. Org. Lett. 18, 2524–2527. doi:10.1021/acs.orglett.6b00088
Iwaniuk, D. P., and Wolf, C. (2010). A Versatile and Practical Solvating Agent for Enantioselective Recognition and NMR Analysis of Protected Amines. J. Org. Chem. 75, 6724–6727. doi:10.1021/jo101426a
James, T. D., Samankumara Sandanayake, K. R. A., and Shinkai, S. (1995). Chiral Discrimination of Monosaccharides Using a Fluorescent Molecular Sensor. Nature 374, 345–347. doi:10.1038/374345a0
Kumobayashi, H., Miura, T., Sayo, N., Saito, T., and Zhang, X. (2001). Recent Advances of BINAP Chemistry in the Industrial Aspects. Synlett 2001 (Special Issue), 1055–1064. doi:10.1055/s-2001-14625
Labuta, J., Ishihara, S., Šikorský, T., Futera, Z., Shundo, A., Hanyková, L., et al. (2013). NMR Spectroscopic Detection of Chirality and Enantiopurity in Referenced Systems without Formation of Diastereomers. Nat. Commun. 4, 2188. doi:10.1038/ncomms3188
Lancelot, C. J., Harper, J. J., and Schleyer, P. v. R. (1969). Participation by Neighboring Aryl Groups. II. Accurate Determinations of Inductive and Anchimeric Assistance Effects by a Hammett-Taft Correlation. J. Am. Chem. Soc. 91, 4294–4296. doi:10.1021/ja01043a051
Liu, H.-L., Hou, X.-L., and Pu, L. (2009). Enantioselective Precipitation and Solid-State Fluorescence Enhancement in the Recognition of α-Hydroxycarboxylic Acids. Angew. Chem. Int. Ed. 48, 382–385. doi:10.1002/anie.200804538
Liu, C.-X., Zheng, L., Zhu, L., Xiao, H.-P., Li, X., and Jiang, J. (2017). Efficient Chiral 1H NMR Analysis of Indoloquinazoline Alkaloids Phaitanthrin A, Cephalanthrin-A and Their Analogues with a Chiral Phosphoric Acid. Org. Biomol. Chem. 15, 4314–4319. doi:10.1039/C7OB00823F
Lovely, A. E., and Wenzel, T. J. (2006). Chiral NMR Discrimination of Secondary Amines Using (18-Crown-6)-2,3,11,12-tetracarboxylic Acid. Org. Lett. 8, 2823–2826. doi:10.1021/ol0609558
Ma, Q., Ma, M., Tian, H., Ye, X., Xiao, H., Chen, L.-h., et al. (2012). A Novel Amine Receptor Based on the Binol Scaffold Functions as a Highly Effective Chiral Shift Reagent for Carboxylic Acids. Org. Lett. 14, 5813–5815. doi:10.1021/ol3027686
Manfredi, K. P., Blunt, J. W., Cardellina, J. H., McMahon, J. B., Pannell, L. L., Cragg, G. M., et al. (1991). Novel Alkaloids from the Tropical Plant Ancistrocladus Abbreviatus Inhibit Cell Killing by HIV-1 and HIV-2. J. Med. Chem. 34, 3402–3405. doi:10.1021/jm00116a011
Mei, X., and Wolf, C. (2004). A Highly Congested N,N′-dioxide Fluorosensor for Enantioselective Recognition of Chiral Hydrogen Bond Donors. Chem. Commun., 2004, 2078–2079. doi:10.1039/B407718K
Miyashita, A., Yasuda, A., Takaya, H., Toriumi, K., Ito, T., Souchi, T., et al. (1980). Synthesis of 2,2'-Bis(diphenylphosphino)-1,1'-Binaphthyl (BINAP), an Atropisomeric Chiral Bis(triaryl)phosphine, and its Use in the Rhodium(I)-catalyzed Asymmetric Hydrogenation of .alpha.-(acylamino)acrylic Acids. J. Am. Chem. Soc. 102, 7932–7934. doi:10.1021/ja00547a020
Moon, L. S., Pal, M., Kasetti, Y., Bharatam, P. V., and Jolly, R. S. (2010). Chiral Solvating Agents for Cyanohydrins and Carboxylic Acids†. J. Org. Chem. 75, 5487–5498. doi:10.1021/jo100445d
Mori, K., Ichikawa, Y., Kobayashi, M., Shibata, Y., Yamanaka, M., and Akiyama, T. (2013). Prediction of Suitable Catalyst by 1H NMR: Asymmetric Synthesis of Multisubstituted Biaryls by Chiral Phosphoric Acid Catalyzed Asymmetric Bromination. Chem. Sci. 4, 4235–4239. doi:10.1039/c3sc52142g
Nicolaou, K. C., Boddy, C. N. C., Bräse, S., and Winssinger, N. (1999). Chemistry, Biology, and Medicine of the Glycopeptide Antibiotics. Angew. Chem. Int. Ed. 38, AID-ANIE2096>3.0.CO, 2096–2152. doi:10.1002/(sici)1521-3773(19990802)38:15<2096:aid-anie2096>3.0.co;2-f
Nieto, S., Lynch, V. M., Anslyn, E. V., Kim, H., and Chin, J. (2008). High-Throughput Screening of Identity, Enantiomeric Excess, and Concentration Using MLCT Transitions in CD Spectroscopy. J. Am. Chem. Soc. 130, 9232–9233. doi:10.1021/ja803443j
Nieto, S., Dragna, J. M., and Anslyn, E. V. (2010). A Facile Circular Dichroism Protocol for Rapid Determination of Enantiomeric Excess and Concentration of Chiral Primary Amines. Chem. Eur. J. 16, 227–232. doi:10.1002/chem.200902650
Parker, D. (1991). NMR Determination of Enantiomeric Purity. Chem. Rev. 91, 1441–1457. doi:10.1021/cr00007a009
Pham, N. H., and Wenzel, T. J. (2011). A Water-Soluble Calix[4]resorcinarene with α-Methyl-l-prolinylmethyl Groups as a Chiral NMR Solvating Agent. J. Org. Chem. 76, 986–989. doi:10.1021/jo102197w
Pirkle, W. H. (1966). The Nonequivalence of Physical Properties of Enantiomers in Optically Active Solvents. Differences in Nuclear Magnetic Resonance Spectra. I. J. Am. Chem. Soc. 88, 1837. doi:10.1021/ja00960a060
Pu, L. (2004). Fluorescence of Organic Molecules in Chiral Recognition. Chem. Rev. 104, 1687–1716. doi:10.1021/cr030052h
Quinn, T. P., Atwood, P. D., Tanski, J. M., Moore, T. F., and Folmer-Andersen, J. F. (2011). Aza-crown Macrocycles as Chiral Solvating Agents for Mandelic Acid Derivatives. J. Org. Chem. 76, 10020–10030. doi:10.1021/jo2018203
Reetz, M. T., Becker, M. H., Kühling, K. M., and Holzwarth, A. (1998). Time-Resolved IR-Thermographic Detection and Screening of Enantioselectivity in Catalytic Reactions. Angew. Chem. Int. Edition 37, 2647–2650. doi:10.1002/(sici)1521-3773(19981016)37:19<2647:aid-anie2647>3.0.co;2-i
Reetz, M. T., Kühling, K. M., Deege, A., Hinrichs, H., and Belder, D. (2000). Super-High-Throughput Screening of Enantioselective Catalysts by Using Capillary Array Electrophoresis. Angew. Chem. Int. Ed. 39, 3891–3893. doi:10.1002/1521-3773(20001103)39:21%3C3891:AID-ANIE3891%3E3.0.CO;2-1
Schurig, V., and Nowotny, H.-P. (1990). Gas Chromatographic Separation of Enantiomers on Cyclodextrin Derivatives. Angew. Chem. Int. Ed. Engl. 29, 939–957. doi:10.1002/anie.199009393
Seco, J. M., Quiñoá, E., and Riguera, R. (2004). The Assignment of Absolute Configuration by NMR†. Chem. Rev. 104, 17–118. doi:10.1021/cr000665j
Tumambac, G. E., and Wolf, C. (2005). Enantioselective Analysis of an Asymmetric Reaction Using a Chiral Fluorosensor. Org. Lett. 7, 4045–4048. doi:10.1021/ol0516216
Wenzel, T. J., and Chisholm, C. D. (2011). Assignment of Absolute Configuration Using Chiral Reagents and NMR Spectroscopy. Chirality 23, 190–214. doi:10.1002/chir.20889
Wenzel, T. J., and Wilcox, J. D. (2003). Chiral Reagents for the Determination of Enantiomeric Excess and Absolute Configuration Using NMR Spectroscopy. Chirality 15, 256–270. doi:10.1002/chir.10190
Wu, C., Liu, H., Li, J., Xiao, H.-P. H.-P., Li, X., and Jiang, J. (2018). Chiral 1H NMR of Atropisomeric Quinazolinones with Enantiopure Phosphoric Acids. Front. Chem. 6, 300. doi:10.3389/fchem.2018.00300
Yang, D., Li, X., Fan, Y.-F., and Zhang, D.-W. (2005). Enantioselective Recognition of Carboxylates: A Receptor Derived from α-Aminoxy Acids Functions as a Chiral Shift Reagent for Carboxylic Acids. J. Am. Chem. Soc. 127, 7996–7997. doi:10.1021/ja051072z
Yeh, H. J. C., Balani, S. K., Yagi, H., Greene, R. M. E., Sharma, N. D., Boyd, D. R., et al. (1986). Use of Chiral Lanthanide Shift Reagents in the Determination of Enantiomer Composition and Absolute Configuration of Epoxides and Arene Oxides. J. Org. Chem. 51, 5439–5443. doi:10.1021/jo00376a080
Zhao, J., Fyles, T. M., and James, T. D. (2004). Chiral Binol-Bisboronic Acid as Fluorescence Sensor for Sugar Acids. Angew. Chem. Int. Ed. 43, 3461–3464. doi:10.1002/anie.200454033
Keywords: chiral recognition, 1H NMR analysis, quinolines, chiral phosphoric acid, chiral shift reagents
Citation: Wan J, Jiang J and Li J (2021) Chiral Phosphoric Acid Promoted Chiral 1H NMR Analysis of Atropisomeric Quinolines. Front. Chem. 9:672704. doi: 10.3389/fchem.2021.672704
Received: 26 February 2021; Accepted: 27 April 2021;
Published: 10 June 2021.
Edited by:
Toshifumi Dohi, Ritsumeikan University, JapanReviewed by:
Keiji Mori, Tokyo University of Agriculture and Technology, JapanRavi Kumar, J. C. Bose University of Science and Technology, YMCA, India
Copyright © 2021 Wan, Jiang and Li. This is an open-access article distributed under the terms of the Creative Commons Attribution License (CC BY). The use, distribution or reproduction in other forums is permitted, provided the original author(s) and the copyright owner(s) are credited and that the original publication in this journal is cited, in accordance with accepted academic practice. No use, distribution or reproduction is permitted which does not comply with these terms.
*Correspondence: Juan Li, anVhbmxpQHd6dS5lZHUuY24=