- School of Light Industry and Engineering, Qi Lu University of Technology, Shandong Academy of Sciences, Jinan, China
Diethyl malonate-based fluorescent probe NE-N2H4 was constructed for monitoring hydrazine (N2H4). The novel probe NE-N2H4 exhibits good properties, such as large Stokes shift (about 125 nm), good selectivity, and low cytotoxicity. This sensing probe NE-N2H4 can be operated to detect hydrazine in living HeLa cells. Especially after soaking in probe solution, the thin-layer chromatography (TLC) plate could detect the vapor of hydrazine. Therefore, the probe NE-N2H4 might be used to monitor hydrazine in biosamples and environmental problem.
Introduction
Hydrazine (N2H4) and its substituted derivatives are usually applied in the aerospace industry as rocket propellant due to the distinctive properties of flammability and explosion (Serov and Kwak, 2010). This molecule N2H4 has also been employed as a catalyst, corrosion inhibitor, and reducing agent in many different fields including pharmaceutical, agricultural, and applied chemical industries (Kean et al., 2006; Khaled, 2006; Rosca and Koper, 2008). Due to its high toxicity, it is also considered as a terrible pollutant to creatures and humans, which could make the lungs, livers, and kidneys cancerous (Garrod et al., 2005). Hence, 10 ppb is the upper line (CDC, 1988). That is why it is important to develop good methods for sensing N2H4 in real-time detection and environmental pollution.
In modern times, chromatography–mass spectrometry, titrimetric, and electrochemical methods have been reported for monitoring N2H4 (Karimi-Maleh et al., 2014; McAdam et al., 2015). During the past few years, molecular probes have been developed for biological imaging with good properties of high sensitivity, large Stokes shift, good selectivity, good biocompatibility, and real-time detection, etc., which were regarded as the most practical method (Lakowicz, 2006; Li et al., 2014; Tang et al., 2015; Zhou X. et al., 2015).
During the last few decades, a series of turn-on probes were applied to detect N2H4 in living biosamples (Cui et al., 2014; Goswami et al., 2014a,b, 2015; Liu et al., 2014, 2019; Qian et al., 2014; Qu et al., 2014; Raju et al., 2014; Sun et al., 2014, 2015; Xiao et al., 2014; Jin et al., 2015; Nandi et al., 2015; Yu et al., 2015; Zhang et al., 2015; Zhou J. et al., 2015; Dai et al., 2016; Reja et al., 2016; Chen et al., 2017; Li et al., 2017, 2018, 2019; Ma et al., 2017; Mahapatra et al., 2017; Jung et al., 2019; Paul et al., 2019; Shi et al., 2019; Xing et al., 2019; Fang et al., 2020; Han et al., 2020; Hou et al., 2020; Vijay and Velmathi, 2020), a few of which were constructed by cleavage of C = C bond (Sun et al., 2014; Reja et al., 2016; Li et al., 2017, 2018, 2019; Liu et al., 2019; Hou et al., 2020). Many examples were developed by the deprotection group from the fluorescent group (Cui et al., 2014; Goswami et al., 2014a, 2015; Liu et al., 2014; Qian et al., 2014; Qu et al., 2014; Raju et al., 2014; Jin et al., 2015; Sun et al., 2015; Yu et al., 2015; Zhang et al., 2015; Zhou J. et al., 2015; Chen et al., 2017; Ma et al., 2017; Mahapatra et al., 2017; Shi et al., 2019; Xing et al., 2019; Fang et al., 2020; Vijay and Velmathi, 2020). Additionally, the rest of the fluorescent molecules were used to monitor N2H4 using the property of special nucleophilicity of N2H4 (Goswami et al., 2014b; Xiao et al., 2014; Nandi et al., 2015; Dai et al., 2016; Jung et al., 2019; Paul et al., 2019; Han et al., 2020). That is why it is necessary to construct a powerful molecule for monitoring N2H4 by the way of cleavage of C = C bond.
In this report, a novel fluorescent probe, NE-N2H4, has been constructed to monitor N2H4 with improved properties including good selectivity, low cytotoxicity, and large Stokes shift over other analytes by cleavage of C = C bond (Scheme 1). The probe NE-N2H4 was applied to imaging N2H4 in living HeLa cells. Besides, the probe NE-N2H4 could monitor vapor of N2H4 by way of thin-layer chromatography (TLC) plate after soaking in solution of probe NE-N2H4. Therefore, this novel probe NE-N2H4 could be regarded as a powerful pool for monitoring N2H4 in biosystems and environmental pollution.
Experiment
Synthesis of Probe NE-N2H4
Here, 6-hydroxy-2-naphthaldehyde (1.0 mmol, 172.0 mg) and diethyl malonate (1.2 mmol, 192.2 mg) were added to EtOH (5.0 ml). Then, piperidine (1.2 mmol, 102.2 mg) was added to the above solution. After reacting at 25°C for 12 h, distilled H2O (10.0 ml) was added to the above reaction, which was extracted with dichloromethane (DCM) (50 ml) 3 times. All the extracts were washed with saturated aqueous sodium chloride solution and dried over MgSO4. The solid residue was dealt with flash column chromatography. The probe NE-N2H4 was obtained (83% yield). 1H NMR (400 MHz, CDCl3): 7.86 (s, 1H), 7.81 (s, 1H), 7.64 (d, J = 8.8 Hz, 1H), 7.52 (d, J = 8.8 Hz, 1H), 7.40 (dd, J1 = 2.0 Hz, J2 = 8.8 Hz, 1H), 7.09–7.04 (m, 2H), 4.41 (dd, J1 = 6.8, J2 = 14.0, 2H), 4.33 (dd, J1 = 7.2, J2 = 14.0, 2H), 1.37–1.32 (m, 6H); 13C NMR (100 MHz, CDCl3): 167.5, 164.5, 155.3, 142.7, 135.6, 131.2, 130.7, 128.3, 128.0, 127.0, 125.9, 124.4, 118.8, 109.5, 61.9, 61.7, 14.2, 14.0; high-resolution mass spectrometry (HRMS) [electrospray ionization (ESI)] m/z calcd for C18H18O5 (M+H)+: 315.1230; found 315.1228.
Vapor Gas Detection
TLC plates were soaked in the probe NE-N2H4 solution [0.1 mM, in dimethylsulfoxide (DMSO)]. After the NE-N2H4 probe-loaded TLC plates were dried over air-blast drying box, the plates were put onto a series of flasks with different concentrations of N2H4 for 10 min. Then, the color of probe-loaded TLC plates was observed under UV light of 365 nm.
Cell Imaging
HeLa cells were grown in modified Eagle's medium (MEM) replenished with 10% fetal bovine serum (FBS) with the atmosphere of 5% CO2 and 95% air at 37°C for 24 h. The HeLa cells were washed with phosphate buffered saline (PBS) three times when used. HeLa cells were treated with NE-N2H4 (20.0 μM) for 30 min, then with N2H4 (200.0 μM) for 30 min at 37°C. The ideal fluorescence images were acquired with a Nikon A1MP confocal microscopy with the equipment of a CCD camera.
Results and Discussion
Design and Synthesis of Probe NE-N2H4
As we all know, aldehyde group was easily reacted with nucleophile to construct C=C bond. Therefore, the simple compound of 6-hydroxy-2-naphthaldehyde was modified simply as the fluorescent group. The turn-on probe NE-N2H4 was developed by modifying a novel recognition site of diethyl malonate with functional aldehyde group in Scheme 2. The structure of the NE-N2H4 was characterized by 1H, 13C NMR, and HRMS (Supplementary Figures 8–10).
The Spectral Property of Probe NE-N2H4
This developed probe NE-N2H4 was applied to measure spectral properties with the addition of N2H4 including absorption spectroscopy and fluorescence spectroscopy. The probe NE-N2H4 exhibited no fluorescence under excitation at 320 nm without addition of N2H4 (Supplementary Figure 1, Figure 1). In contrary, strong fluorescence emission appeared at 445 nm after adding N2H4 to the solution of NE-N2H4, with a quantum yield of 0.35. When the addition of N2H4 was up to 200 equivalent, the fluorescence enhancement emerged to the high point (Figure 1). Therefore, the probe NE-N2H4 was easy to respond to N2H4, which was suitable for sensing N2H4 as a powerful pool with a large Stokes shift. The pH effect of PBS buffer was examined in Supplementary Figure 2. The fluorescent intensity increased from acid to basic rapidly. The main reason is that the nucleophilic substitution to the probe NE-N2H4 reacted easily in alkaline conditions.
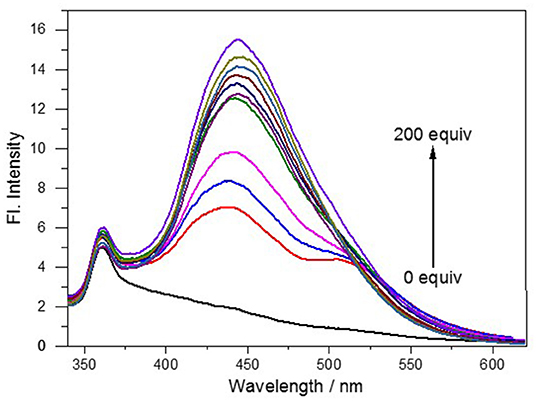
Figure 1. Fluorescence spectra of NE-N2H4 (10 μM) in pH 7.4 phosphate buffered saline (PBS)/dimethyl sulfoxide (DMSO0 (v/v = 1/1) in the absence or presence of N2H4.
Mechanism
The sensing mechanism was examined by adding N2H4 to the solution of probe NE-N2H4. The reaction solution was detected by HRMS. When probe NE-N2H4 (20 μM) was treated with N2H4 (200 μM), a peak at m/z 187.0879 emerged in HRMS spectrum in accordance with the predicted NE-N2H4-adduct (Supplementary Figure 4). The NE-N2H4-adduct was constructed in one step easily characterized by 1H NMR and HRMS (Supplementary Figures 5, 6). Additionally, the absortion spectra of NE-N2H4 (10 μM) in absence or presence of N2H4 (10 equiv) and the synthetic NE-N2H4-adduct in pH 7.4 PBS/DMSO (v/v = 1/1) were listed in Supplementary Figure 3, which is consistent with the proposed mechanism (Scheme 1).
Response Rate and Selectivity of Probe NE-N2H4
The time course of probe NE-N2H4 was measured after the addition of N2H4 (10 equiv) (Figures 2A,B). Notably, the fluorescence enhancement was increased obviously as time goes on. That is to say, the sensing probe NE-N2H4 could be fit for imaging N2H4 in living cells. Another important factor is selectivity research of NE-N2H4 compared to other interfering species. It is very crucial whether the sensing molecule NE-N2H4 is suitable for cell imaging in the biosystem. The selectivity research was performed in Figure 3 over other competitive molecules. We find that fluorescence intensity showed almost no change after adding N2H4, when the solution of probe NE-N2H4 was added with other competitive molecules including , , , I−, Br−, Fe2+, H2O2, NO, Li+, Zn2+, Ni2+, Cys, GSH, S2−, and N2H4. In conclusion, the sensing probe NE-N2H4 could be suitable for the response to N2H4 with good selectivity over other interfering molecules in the biosamples.
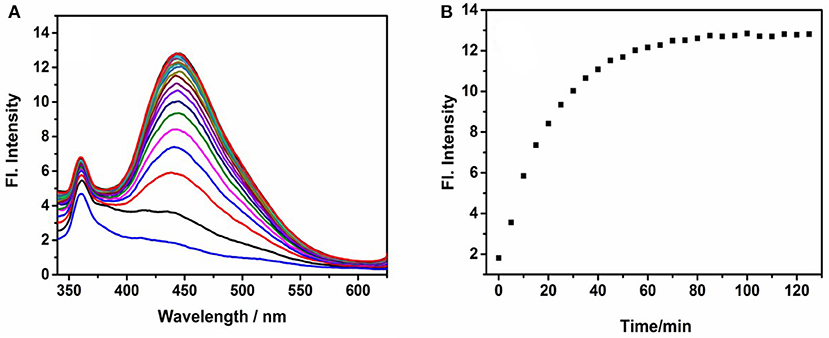
Figure 2. Fluorescence spectra of NE-N2H4 (10 μM) in pH 7.4 phosphate buffered saline (PBS)/dimethylsulfoxide (DMSO) (v/v = 1/1) in the absence or presence of N2H4 (10 equiv). (A,B) were depicted in Response Rate and Selectivity of Probe NE-N2H4.
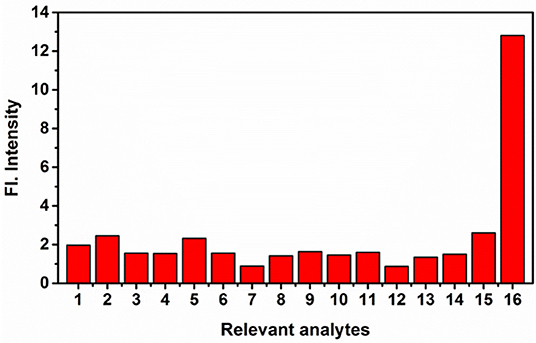
Figure 3. The fluorescence intensity of NE-N2H4 (10 μM) in the presence of various analytes (10 equiv) in phosphate buffered saline (PBS) buffer [pH 7.4 PBS/dimethylsulfoxide (DMSO) (v/v = 1/1)]. 1: None; 2: ; 3: ; 4: ; 5: I−; 6: Br−; 7: Fe2+; 8: H2O2; 9: NO; 10: Li+; 11: Zn2+; 12: Ni2+; 13: Cys; 14: GSH; 15: S2−; 16: N2H4.
Application in Gas Detection
According to the above research data, the application of gas detection was operated. The free TLC plates were soaked in the solution of NE-N2H4 (0.1 mM, in DMF). The TLC plates loaded with probe NE-N2H4 were prepared to discriminate N2H4 (gas) in different concentrations after drying with a vacuum dryer. Distinctive fluorescence color changes of plates were obtained under UV 365 nm light (Figure 4) after exposing TLC plates to the N2H4 (gas) for 10 min. Obviously, no obvious change was exhibited in the distilled water (Figure 4a). The experimental result indicated that the sensing probe NE-N2H4 may be a practical method to detect N2H4 in industrial pollution.

Figure 4. Photographs of thin-layer chromatography (TLC) plates, soaked in the solution of NE-N2H4, followed by addition of different amounts of hydrazine. (a) Water, (b) 10% N2H4, (c) 20% N2H4, (d) 30% N2H4, (e) 40% N2H4, (f) 50% N2H4.
Cytotoxicity and Imaging
Encouraged by the good fluorescent properties of probe NE-N2H4 including sensitive response, good selectivity, and large Stokes shift, a laser confocal microscope was applied to test the potential applications in cell imaging. The cytotoxicity of probe NE-N2H4was tested for imaging MTT assays in living cells. The living HeLa cells were operated for imaging fluorescent experiments by means of confocal laser scanning microscopy.
MTT assays were operated on living HeLa cells incubated with probe NE-N2H4 (see Supplementary Figure 4). The data indicated that this probe NE-N2H4 at different concentrations was almost nontoxic to the living cells [>90% HeLa cells survived after 24 h with NE-N2H4 (10.0 μM) incubation]. Therefore, this probe is fit for imaging N2H4 in living HeLa cells.
The probe NE-N2H4 was operated to incubate living HeLa cells for bioimaging of N2H4 due to the improved properties. Firstly, the solution of probe NE-N2H4 was used for incubating living HeLa cells for 30 min. No obvious fluorescence emerged in blue channel collected with Nikon A1MP confocal microscopy with a CCD camera (Figures 5a–c). Then, probe NE-N2H4 was used to incubate the living HeLa cells for 30 min and treated with N2H4 for another 30 min, obvious fluorescence exhibited in blue channel (Figures 5d–f). The experimental data indicated that the probe NE-N2H4 was fit for imaging N2H4 in living HeLa cells.
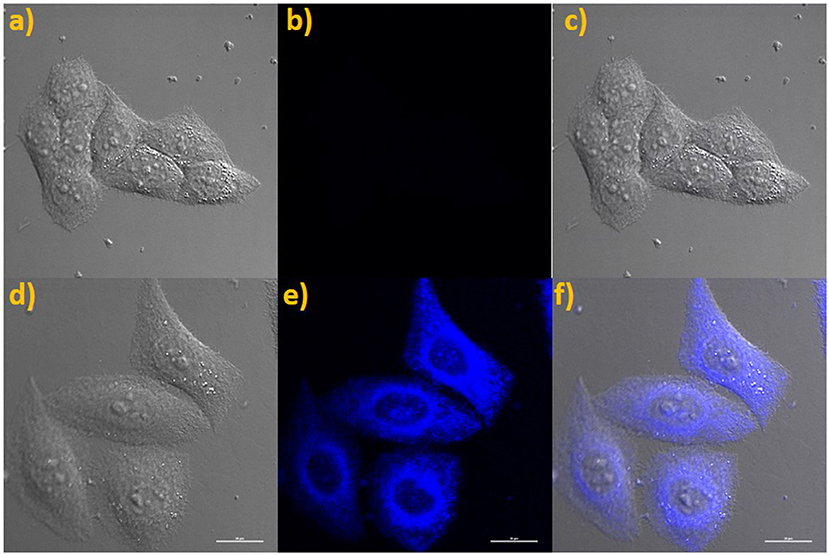
Figure 5. (a) Brightfield image of living HeLa cells costained only with NE-N2H4. (b) Fluorescence images of (a) from blue channel. (c) Overlay of (a,b). (d) Brightfield image of living HeLa cells costained with NE-N2H4 and N2H4. (e) Fluorescence image of (d) from blue channel. (f) Overlay of the brightfield image (d) and blue channels (e).
Conclusion
In conclusion, an organic fluorescent probe has been constructed using diethyl malonate as a recognition site for sensing N2H4 with good selectivity and large Stokes shift (125 nm). This novel probe NE-N2H4 was developed for sensing N2H4 in living HeLa cells. In addition, this probe NE-N2H4 was applied for gas detection by probe-loaded TLC plate. The above results indicate that the probe NE-N2H4 may be powerful for monitoring N2H4 in biosystems and environmental problem.
Data Availability Statement
The original contributions presented in the study are included in the article/Supplementary Materials, further inquiries can be directed to the corresponding author/s.
Author Contributions
J-YW: synthesize and characterize the dyes. JQ: supervise the project, review, and edit manuscript. HZ: supervise the project, review, and edit manuscript. Z-HZ and Z-TW: design, synthesize, characterize the dyes, write and edit manuscript, and manage the research project. All authors: contributed to the article and approved the submitted version.
Funding
This work was financially supported by the Natural Science Foundation of China (21801145), Shandong Provincial Key R&D Program/Major Science and Technology Innovation Project of Shandong Province (2019JZZY020230), and Natural Science Foundation of Shandong Province (ZR2017BB012).
Conflict of Interest
The authors declare that the research was conducted in the absence of any commercial or financial relationships that could be construed as a potential conflict of interest.
Supplementary Material
The Supplementary Material for this article can be found online at: https://www.frontiersin.org/articles/10.3389/fchem.2020.602125/full#supplementary-material
References
CDC (1988). Occupational Safety and Health Guideline for Hydrazine Potential Human Carcinogen. U.S Department of Health and Human Services; Centers for Disease Control.
Chen, W., Liu, W., Liu, X. J., Kuang, Y. Q., Yu, R. Q., and Jiang, J. H. (2017). A novel fluorescent probe for sensitive detection and imaging of hydrazine in living cells. Talanta 162, 225–231. doi: 10.1016/j.talanta.2016.10.026
Cui, L., Ji, C., Peng, Z., Zhong, L., Zhou, C., Yan, L., et al. (2014). Unique tri-output optical probe for specific and ultrasensitive detection of hydrazine. Anal. Chem. 86, 4611–4617. doi: 10.1021/ac5007552
Dai, X., Wang, Z. Y., Du, Z. F., Miao, J. Y., and Zhao, B. X. (2016). A simple but effective near-infrared ratiometric fluorescent probe forhydrazine and its application in bioimaging. Sens. Actuators B 232, 369–374. doi: 10.1016/j.snb.2016.03.159
Fang, Q., Yang, L., Xiong, H., Han, S., Zhang, Y., Wang, J., et al. (2020). Coumarinocoumarin-based fluorescent probe for the sensitive and selective detection of hydrazine in living cells and zebra fish. Chin. Chem. Lett. 31, 129–132. doi: 10.1016/j.cclet.2019.04.021
Garrod, S., Bollard, M. E., Nicholls, A. W., Connor, S. C., Connelly, J., Nicholson, J. K., et al. (2005). Integrated metabonomic analysis of the multiorgan effects of hydrazine toxicity in the rat. Chem. Res. Toxicol. 18, 115–122. doi: 10.1021/tx0498915
Goswami, S., Aich, K., Das, S., Roy, S. B., Pakhira, B., and Sarkar, S. (2014a). A reaction based colorimetric as well as fluorescence “turn on” probe for the rapid detection of hydrazine. RSC Adv. 4, 14210–14214. doi: 10.1039/c3ra46663a
Goswami, S., Das, S., Aich, K., Sarkar, D., and Mondal, T. K. (2014b). A coumarin based chemodosimetric probe for ratiometric detection of hydrazine. Tetrahedron Lett. 55, 2695–2699. doi: 10.1016/j.tetlet.2014.03.041
Goswami, S., Paul, S., and Manna, A. (2015). Fast and ratiometric “naked” eye detection of hydrazine for both solid, vapour phase sensing. New J. Chem. 39, 2300–2305. doi: 10.1039/C4NJ02220C
Han, J., Yue, X., Wang, J., Zhang, Y., Wang, B., and Song, X. (2020). A ratiometric merocyanine-based fluorescent probe for detecting hydrazine in living cells and zebra fish. Chin. Chem. Lett. 31, 1508–1510. doi: 10.1016/j.cclet.2020.01.029
Hou, J.-T., Wang, B., Wang, S., Wu, Y., Liao, Y. X., and Ren, W. (2020). Detection of hydrazine via a highly selective fluorescent probe: a case study on the reactivity of cyano-substituted C=C bond. Dyes Pigm. 178:108366. doi: 10.1016/j.dyepig.2020.108366
Jin, X., Liu, C., Wang, X., Huang, H., Zhang, X., and Zhu, H. (2015). A flavone-based ESIPT fluorescent sensor for detection of N2H4 in aqueous solution and gas state and its imaging in living cells. Sens. Actuators B 216, 141–149. doi: 10.1016/j.snb.2015.03.088
Jung, Y., Ju, I. G., Choe, Y. H., Kim, Y., Park, S., Hyun, Y. M., et al. (2019). Hydrazine exposé: the next-generation fluorescent probe. ACS Sens. 4, 441–449. doi: 10.1021/acssensors.8b01429
Karimi-Maleh, H., Moazampour, M., Ensafi, A. A., Mallakpour, S., and Hatami, M. (2014). An electrochemical nanocomposite modified carbon paste electrode as a sensor for simultaneous determination of hydrazine and phenol in water and wastewater samples. Environ. Sci. Pollut. Res. Int. 21, 5879–5888. doi: 10.1007/s11356-014-2529-0
Kean, T., Miller, J. H. M., Skellern, G. G., and Snodin, D. (2006). Acceptance criteria for levels of hydrazine in substances for pharmaceutical use and analytical methods for its determination. Pharmeur. Sci. Notes 2, 23–33.
Khaled, K. F. (2006). Experimental and theoretical study for corrosion inhibition of mild steel in hydrochloric acid solution by some new hydrazine carbodithioic acid derivatives. Appl. Surf. Sci. 252, 4120–4128. doi: 10.1016/j.apsusc.2005.06.016
Lakowicz, J. R. (2006). Principles of Fluorescence Spectroscopy. New York, NY: Springer. doi: 10.1007/978-0-387-46312-4
Li, J., Cui, Y., Bi, C., Feng, S., Yu, F., Yuan, E., et al. (2019). Oligo(ethylene glycol)-functionalized ratiometric fluorescent probe for the detection of hydrazine in vitro and in vivo. Anal. Chem. 91, 7360–7365. doi: 10.1021/acs.analchem.9b01223
Li, X., Gao, X., Shi, W., and Ma, H. (2014). Design strategies for water-soluble small molecular chromogenic and fluorogenic probes. Chem. Rev. 114, 590–659. doi: 10.1021/cr300508p
Li, Z., Ren, M., Wang, L., and Lin, W. (2018). Ethyl cyanoacetate based turn-on fluorescent probe for hydrazine and its bio-imaging and environmental applications. Anal. Methods 10, 4016–4019. doi: 10.1039/C8AY01336E
Li, Z., Zhang, W., Liu, C., Yu, M., Zhang, H., Guo, L., et al. (2017). A colorimetric and ratiometric fluorescent probe for hydrazine and itsapplication in living cells with low dark toxicity. Sens. Actuators B 241, 665–671. doi: 10.1016/j.snb.2016.10.141
Liu, B., Liu, Q., Shah, M., Wang, J., Zhang, G., and Pang, Y. (2014). Fluorescence monitor of hydrazine in vivo by selective deprotection of flavonoid. Sens. Actuators B 202, 194–200. doi: 10.1016/j.snb.2014.05.010
Liu, Y., Ren, D., Zhang, J., Li, H., and Yang, X. F. (2019). A fluorescent probe for hydrazine based on a newly developed 1-indanonefused coumarin scaffold. Dyes Pigm. 162, 112–119. doi: 10.1016/j.dyepig.2018.10.012
Ma, J., Fan, J., Li, H., Yao, Q., Xia, J., Wang, J., et al. (2017). Probing hydrazine with a near-infrared fluorescent chemodosimeter. Dyes Pigm. 138, 39–46. doi: 10.1016/j.dyepig.2016.11.026
Mahapatra, A. K., Karmakar, P., Manna, S., Maiti, K., and Mandal, D. (2017). Benzthiazole-derived chromogenic fluorogenic and ratiometric probes for detection of hydrazine in environmental samples and living cells. J. Photochem. Photobiol. A 334, 1–12. doi: 10.1016/j.jphotochem.2016.10.032
McAdam, K., Kimpton, H., Essen, S., Davis, P., Vas, C., Wright, C., et al. (2015). Analysis of hydrazine in smokeless tobacco products by gas chromatography-mass spectrometry. Chem. Cent. J. 9, 13–26. doi: 10.1186/s13065-015-0089-0
Nandi, S., Sahana, A., Mandal, S., Sengupta, A., Chatterjee, A., Safin, D. A., et al. (2015). Hydrazine selective dual signaling chemodosimetric probe in physiological conditions and its application in live cells. Anal. Chim. Acta 893, 84–90. doi: 10.1016/j.aca.2015.08.041
Paul, S., Nandi, R., Ghoshal, K., Bhattacharyya, M., and Maiti, D. K. (2019). A smart sensor for rapid detection of lethal hydrazine in human blood and drinking water. New J. Chem. 43, 3303–3308. doi: 10.1039/C8NJ06230G
Qian, Y., Lin, J., Han, L., Lin, L., and Zhu, H. (2014). A resorufin-based colorimetric and fluorescent probe for live-cell monitoring of hydrazine. Biosens. Bioelectron. 58, 282–286. doi: 10.1016/j.bios.2014.02.059
Qu, D. Y., Chen, J. L., and Di, B. (2014). A fluorescence “switch-on” approach to detect hydrazine in aqueous solution at neutral pH. Anal. Methods 6, 4705–4709. doi: 10.1039/C4AY00533C
Raju, M. V. R., Prakash, E. C., Chang, H. C., and Lin, H. C. (2014). A facile ratiometric fluorescent chemodosimeter for hydrazine based on Ing-Manske hydrazinolysis and its applications in living cells. Dyes Pigm. 103, 9–20. doi: 10.1016/j.dyepig.2013.11.015
Reja, S. I., Gupta, N., Bhalla, V., Kaur, D., Arora, S., and Kumar, M. (2016). A charge transfer based ratiometric fluorescent probe for detection of hydrazine in aqueous medium and living cells. Sens. Actuators B 222, 923–929. doi: 10.1016/j.snb.2015.08.078
Rosca, V., and Koper, M. T. M. (2008). Electrocatalytic oxidation of hydrazine on platinum electrodes in alkaline solutions. Electrochim. Acta 53, 5199–5205. doi: 10.1016/j.electacta.2008.02.054
Serov, A., and Kwak, C. (2010). Direct hydrazine fuel cells: a review. Appl. Catal. B Environ. 98:1–9. doi: 10.1016/j.apcatb.2010.05.005
Shi, X., Huo, F., Chao, J., Zhang, Y., and Yin, C. (2019). An isophorone-based NIR probe for hydrazine in real water sample and hermetic space. New J. Chem. 43, 10025–10029. doi: 10.1039/C9NJ01661A
Sun, M., Guo, J., Yang, Q., Xiao, N., and Li, Y. (2014). A new fluorescent and colorimetric sensor for hydrazine and its application in biological systems. J. Mater. Chem. B 2, 1846–1851. doi: 10.1039/C3TB21753A
Sun, Y., Zhao, D., Fan, S., and Duan, L. (2015). A 4-hydroxynaphthalimide-derived ratiometric fluorescent probe for hydrazine and its in vivo applications. Sens. Actuators B 208, 512–517. doi: 10.1016/j.snb.2014.11.057
Tang, Y., Lee, D., Wang, J., Li, G., Yu, J., Lin, W., et al. (2015). Development of fluorescent probes based on protection-deprotection of the key functional groups for biological imaging. Chem. Soc. Rev. 44, 5003–5015. doi: 10.1039/C5CS00103J
Vijay, N., and Velmathi, S. (2020). Near-Infrared-Emitting probes for detection of nanomolar hydrazine in a complete aqueous medium with real-time application in bioimaging and vapor-phase hydrazine detection. ACS Sust. Chem. Eng. 8, 4457–4463. doi: 10.1021/acssuschemeng.9b07445
Xiao, L., Tu, J., Sun, S., Pei, Z., Pei, Y., Pang, Y., et al. (2014). A fluorescent probe for hydrazine and its in vivo applications. RSC Adv. 4, 41807–41811. doi: 10.1039/C4RA08101C
Xing, M., Wang, K., Wu, X., Ma, S., Cao, D., Guan, R., et al. (2019). A coumarin chalcone ratiometric fluorescent probe for hydrazine based on deprotection addition and subsequent cyclization mechanism. Chem. Commun. 55, 14980–14983. doi: 10.1039/C9CC08174G
Yu, S., Wang, S., Yu, H., Feng, Y., Zhang, S., Zhu, M., et al. (2015). A ratiometric two-photon fluorescent probe for hydrazine and its applications. Sens. Actuators B 220, 1338–1345. doi: 10.1016/j.snb.2015.07.051
Zhang, J., Ning, L., Liu, J., Wang, J., Yu, B., Liu, X., et al. (2015). Naked-eye and near-infrared fluorescence probe for hydrazine and its applications in in vitro and in vivo bioimaging. Anal. Chem. 87, 9101–9107. doi: 10.1021/acs.analchem.5b02527
Zhou, J., Shi, R., Liu, J., Wang, R., Xu, Y., and Qian, X. (2015). An ESIPT-based fluorescent probe for sensitive detection of hydrazine in aqueous solution. Org. Biomol. Chem. 13, 5344–5348. doi: 10.1039/C5OB00209E
Keywords: fluorescent probe, hydrazine, Stokes shift, gas detection, cell imaging
Citation: Qu J, Zhang Z-H, Zhang H, Weng Z-T and Wang J-Y (2021) Diethyl Malonate-Based Turn-On Chemical Probe for Detecting Hydrazine and Its Bio-Imaging and Environmental Applications With Large Stokes Shift. Front. Chem. 8:602125. doi: 10.3389/fchem.2020.602125
Received: 02 September 2020; Accepted: 14 December 2020;
Published: 18 March 2021.
Edited by:
Zhenzhong Guo, Wuhan University of Science and Technology, ChinaCopyright © 2021 Qu, Zhang, Zhang, Weng and Wang. This is an open-access article distributed under the terms of the Creative Commons Attribution License (CC BY). The use, distribution or reproduction in other forums is permitted, provided the original author(s) and the copyright owner(s) are credited and that the original publication in this journal is cited, in accordance with accepted academic practice. No use, distribution or reproduction is permitted which does not comply with these terms.
*Correspondence: Jian-Yong Wang, wjy@qlu.edu.cn