- Heterocyclic Chemistry Research Laboratory, Chemistry Department, Iran University of Science and Technology, Tehran, Iran
In this protocol, Fucoidan (FU), a fucose-rich sulfated polysaccharide extracted from brown algae Fucus vesiculosus was used for in situ preparation of magnetic Fe3O4@FU. Nanoco magnetic properties of Fe3O4@FU were investigated by energy dispersive X-ray (EDX) spectroscopy, X-ray diffraction (XRD), Fourier transform infrared spectroscopy (FT-IR), field emission scanning electron microscopy (FESEM), transmission electron microscopy (TEM), Brunauer–Emmett–Teller (BET) adsorption method, and vibrating sample magnetometer (VSM). The catalytic activity of Fe3O4@FU was employed for the synthesis of tri- and tetra-substituted imidazoles through three- and four-component reactions respectively, between benzyl, aldehydes, NH4OAc and benzyl, aldehydes, NH4OAc, and amine under reflux in ethanol. It is worth nothing that excellent yields, short reaction times, chromatography-free purification, and environmental friendliness are highlighted features of this protocol.
Introduction
Through extensive applications and potential in the chemical industry and preservation of the environment, the recently supported solid nanocatalysis has been faced with various attentions in catalysis science and technology (Amirnejat et al., 2013; Fereshteh and Shahrzad, 2020). To overcome the difficulty of catalyst separation, some magnetic heterogeneous catalysts with unique features and advanced functionalities suitable for a range of applications, including biological and environmental applications have been made (Pourian et al., 2018; Zaheri et al., 2018). The magnetic materials have gained more attention due to their combined physicochemical characteristics such as high surface area, high thermal and chemical stability, excellent biocompatibility and biodegradability, and efficient super magnetic behavior (Dolatkhah et al., 2018; Piri et al., 2019).
Natural biopolymers as an effective tool have given rise to a new method of producing degradable materials. Meanwhile, marine polysaccharides exhibit a vast variety of structures and could be considered as a novel natural source (Dekamin et al., 2016; Alipour et al., 2018; Dolatkhah et al., 2019). Nanomaterials based on marine polysaccharides have been considered as one of the most important topics of research in recent years, especially in chemical and bio-based research, due to biocompatibility and biodegradability, cheapness, non-toxicity, and abundance (Hemmati et al., 2016; Amirnejat et al., 2020a,b,c). Fucoidan refers to a type of polysaccharide which contains substantial percentages of L-fucose and sulfated ester groups, mainly derived from brown seaweed which has been extensively studied due to its numerous interesting biological activities (Gomez-Zavaglia et al., 2019; Zayed and Ulber, 2019, 2020).
In recent decades, it has been extensively represented that multi-component reaction (MCR) is an ideal tool for creating molecular diversity and complexity (Graebin et al., 2019). Meanwhile imidazoles, polar in nature and with a five-member ring structure, are one of the most important compounds showing a wealthy source of biologically important features such as inhibitors, fungicides, herbicides plant, anti-inflammatory, anticancer, antimicrobial, analgesic, and anti-tubercular activity (Shalini et al., 2010; Varzi and Maleki, 2019). Numerous approaches have been developed for the synthesis of 1,2,4,5-tetrasubstituted imidazoles, which can be prepared by a four-component cyclo condensation consisting of aldehyde, benzil, a primary amine and ammonium acetate in the presence of different catalysts such as BF3·SiO2 (Sadeghi et al., 2008), and silica gel/NaHSO4 (Karimi et al., 2006), while other main components are achieved by synthesis of tri-substituted imidazoles by the condensation of benzil derivatives, aryl aldehydes, and ammonium acetate catalyzed by different catalysts such as ZrCl4 (Shitole et al., 2015), sulfanilic acid (Gadekar et al., 2009), and chitosan (Zheng et al., 2019). However, some of these methodologies have some drawbacks, such as low yields, long reaction times, severe reaction conditions, and work up procedure. Herein, we report the in situ synthesis of a novel eco-friendly magnetic heterogeneous catalyst Fe3O4@FU for the synthesis of tri- and tetra-substituted imidazoles under reflux condition in ethanol (Scheme 1). Easy work up and separation, high product yields and short reaction times made this method effective and advantageous.
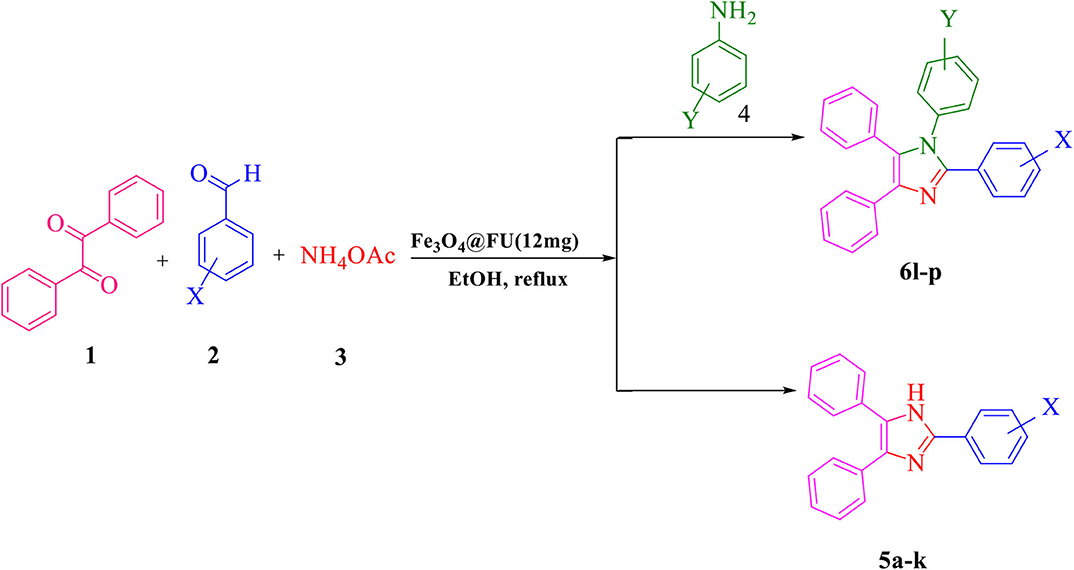
Scheme 1. The synthesis of 2,4,5-triaryl-1H-imidazoles 5a-k and 1,2,4,5- tetraaryl-1H-imidazoles 6l-p via catalytic application of Fe3O4@FU.
Experimental
Materials and Methods
All solvents and chemicals were purchased from Merck and Aldrich. All reactions and the purity of the products were monitored by thin-layer chromatography (TLC) using aluminum plates coated with silica gel F254 plates (Merck) using ethyl acetate and n-hexane as eluents. UV light with a wavelength of 254 nm was used for the detection of products. By using an Electro thermal 9100, melting points were determined in open capillaries. IR spectra were run on a 400s Shimadzu FTIR Spectrophotometer (as KBr pellets). 1H and 13C NMR spectra were recorded on a 500 MHz Bruker Avance DRX Spectrometer instrument using TMS as an internal standard and CDCl3, DMSO-d6 as a solvent. The XRD patterns were obtained on an X-ray diffractometer (Holland, Philips Xpert, Co K, radiation, λ = 0.178897 nm). A Field Emission Scanning Electron Microscope (FE-SEM) with 15 KV, Mira3, Tescan), Thermal Gravimetric Analysis (TGA D-32609 from Hullhorst), and Transmission electron microscope (TEM, Philips –CM120, 100 KV) were used. An ultrasonic probe watt ultrasonic homogenizer 400 from Topsonics Co was used in room temperature for optimization of the reaction.
Preparations of Fucoidan Powder
2.5 gr of algae Fucus vesiculosus was finely ground by a ball mill for 5 min and was placed in a round bottom 200 ml flask containing 100 ml of 96% ethanol, and was stirred for 12 h. Then, the suspension was centrifuged at 4,000 rpm for 15 min and the resulting powder was dried at 50°C for 1 h.
Synthesis of Magnetic Fe3O4@FU Nanocomposite
For the preparation of the Fe3O4@FU, 0.2 g of fucoidan powder, FeCl2.4H2O (2 g, 0.01 mol) and (5.5 g, 0.02 mol) of FeCl3 were used. 6H2O was dissolved in 100 ml of distilled water. Then the mixture was vigorously stirred under a nitrogen atmosphere at 80°C for 15 min to reach a uniform solution. The pH of the solution was then adjusted to 12 by the dropwise addition of an aqueous ammonia solution (25%). The mixture was stirred at 80°C for 45 min. The prepared magnetic nanoparticles were separated by an external magnet, finally washed with ethanol and DI, and dried for 6 h at 60°C (Scheme 2).
General Procedure for the Preparation of 2, 4, 5-Trisubstituted Imidazoles Derivatives
A mixture of benzil (210 mg, 1 mmol), aldehydes (1 mmol), NH4OAc (154 mg, 2 mmol), and Fe3O4@FU NPs (12 mg) in 3 ml EtOH was stirred under reflux conditions for the appropriate times. The progress of the reactions was monitored by TLC (eluent: EtOAc / n-hexane, 1: 3). After completion of the reaction, the catalyst was easily separated by an external magnet and then reused as such for the following experiment after being washed with EtOH and dried. The pure products were obtained by recrystallization from hot EtOH and then dried at 60°C for 1 h.
General Procedure for the Preparation of 1, 2, 4, 5-Tetrasubstituted Imidazoles Derivatives
Benzil (210 mg, 1 mmol), aldehydes (1 mmol), NH4OAc (77 mg, 1 mmol), amine (1 mmol), and Fe3O4@FU NPs (12 mg) in ethanol (3 mL) was stirred under reflux conditions until completion of the reaction which was monitored by TLC (eluent: EtOAc/n-hexane, 1:3). The catalyst was separated from the mixture by an external magnet, washed and dried for use in the next run. The crude products were filtered and recrystallized from ethanol.
Results and Discussion
Fe3O4@FU magnetic nanoparticles as a heterogeneous catalyst were characterized by Fourier transform infrared (FT-IR) spectral analysis. One strong broad band at 3,500 cm−1 was attributed to the stretching vibration due to the O-H of fucoidan and water. The appearance of the peak at 1,624 cm−1, attributed to significant polysaccharide chains (Figure 1a), is stronger than magnetic fucoidan (Figure 1b). The absorption band at 1,029 cm−1 indicated hemiacetal vibration at alcohol and ether functional groups in fucoidan structure. Furthermore, the peak at 1,240–1,255 cm−1 was related to the stretching vibration of S=O from the SO3H group. The presence of the metal oxide peaks of 570 and 455 cm−1 also exhibited in FT-IR of Fe3O4@FU acknowledged that the chemical structure of the magnetic nanoparticles have been preserved after the functionalization.
Primarily, the size, structure, and morphology of FU, and the as prepared nanocomposite were investigated by SEM analyses (Figures 2a,b). As can be seen, Fe3O4@FU nanocomposites have a cauliflower-shaped morphology in which the average size distribution was around 24–33 nm. TEM analysis of the as-synthesized Fe3O4@FU showed that the Fe3O4@FU NPs have a core–shell structure (Figure 2c). Simultaneously, the elemental composition of Fe3O4@FU and FU were studied by EDX analysis (Figures 2d,e) which confirmed the presence of O, C, Fe, and S elements constituted in the nanocomposite.
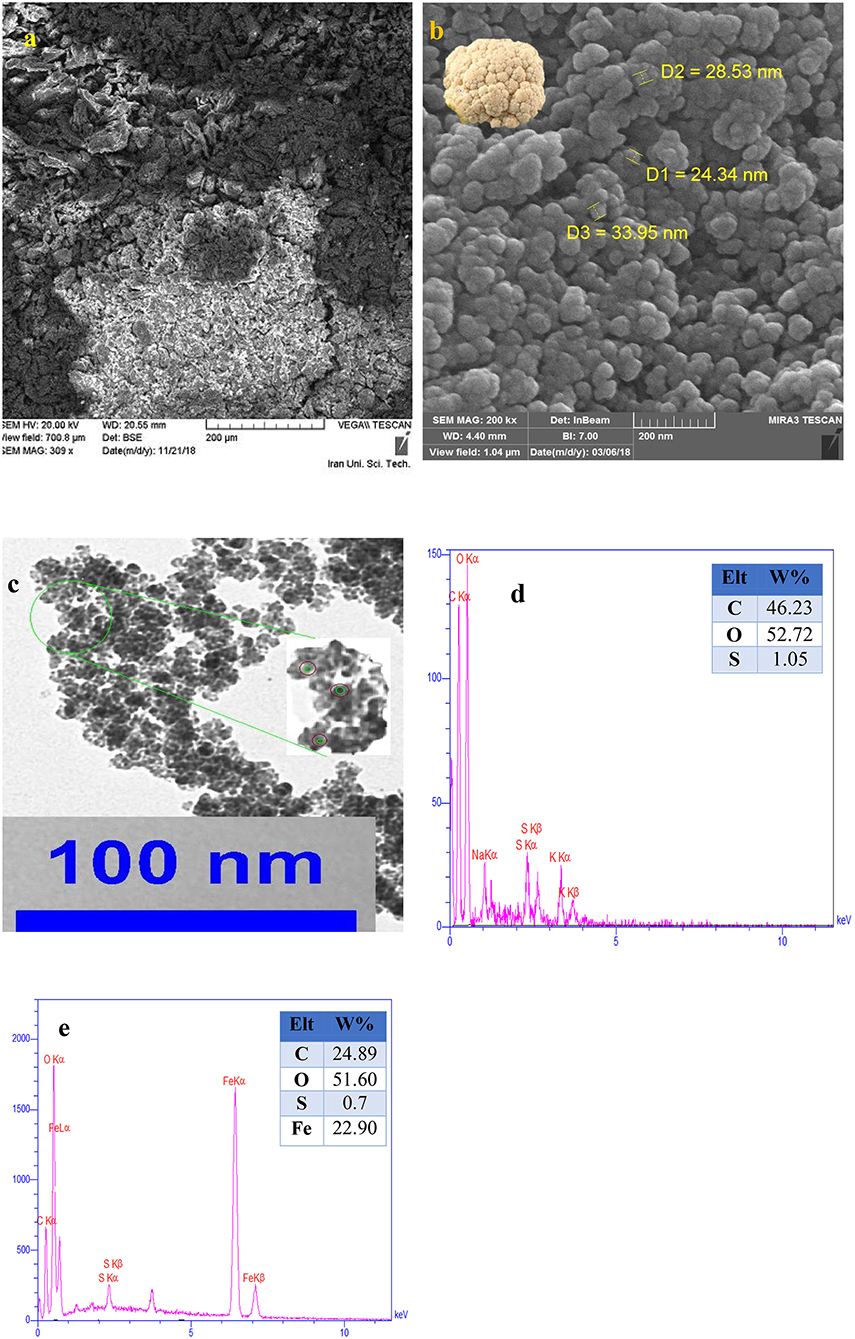
Figure 2. SEM images of (a) FU, (b) Fe3O4@FU, and (c) TEM images of Fe3O4@FU NPs. EDX analysis of (d) FU, and (e) Fe3O4@FU NPs.
The surface area and pore size distributions of the Fe3O4@FU were analyzed by N2 adsorption-desorption analysis. As shown in Figure 3, Fe3O4@FU NPs have type IV isotherms and type H3 hysteresis loops. The BET surface area, average pore diameter and the total pore volume were calculated to be 55.65 m2/g, 11 nm, and 1.749 cm3/g, respectively.
The XRD patterns of Fe3O4@FU NPs are presented in Figure 4. As can be seen, diffraction peaks at 2θ values = 30.4°, 35.8°, 43.7°, 55.3°, 57.8°, and 63.3° could be indexed to the presence of all the crystal planes such as (220), (311), (400), (422), (511), and (440) attributed to the cubic inverse spinel structure of Fe3O4 which matched with the standard pattern (JCPDS-PDF, No. 01-087-2334). These results proved that the crystalline structure of Fe3O4 was maintained after its decoration with fucoidan polysaccharide.
The magnetization curves of Fe3O4 and Fe3O4@FU NPs measured at room temperature with a vibrating sample magnetometer (VSM) were shown in Figure 5. The hysteresis loops of Fe3O4@FU NPs showed the superparamagnetic behavior of Fe3O4@FU NPs. The saturation magnetization (Ms) values for the Fe3O4 and the Fe3O4@FU NPs were 51, 35 emu/g, respectively. It is important to note that the saturation magnetization remains sufficient after covering by FU.
The thermogravimetric analysis (TGA) curve of FU and Fe3O4@FU NPs showed three-stage weight loss in the temperature range from 100 to 500°C (Figure 6). The first weight loss of around 2 wt% ensues at 100°C indicating the evaporation of water or solvent. The next weight loss of about 12 wt% occurs at 240°C and the third weight loss about 42 wt% at 440°C for the decomposition of polysaccharide. Accordingly, the TGA studies showed improved stability for Fe3O4@FU NPs.
Catalytic Activity of Magnetic Fe3O4@FU NPs
The catalytic efficacy of Fe3O4@FU NPs as a proficient heterogeneous catalyst was investigated in the one-pot reaction between benzil (1 mmol), benzaldehyde (1 mmol), and ammonium acetate (2 mmol) as a model reaction for the synthesis of imidazole derivatives. To determine the role of the catalyst, the model reaction was performed in the absence of the catalyst. The anticipated product was not shown after a long reaction time. The results reveal that the presence of the catalyst has a considerable effect on the formation of these compounds. The model reactions were carried out in the presence of different solvents such as EtOH, H2O, THF, and Toluene, CH3CN and solvent-free conditions. As the results show, Ethanol (3 ml) was found to be the most effective solvent. To evaluate the optimum catalyst concentration, the model reaction was carried out in the presence of various amounts of catalyst (5, 10, 12, and 15 mg). Consequently, the best yield is accessible in the presence of just 12 mg catalyst, and use of extra amounts of the catalyst (15 mg) did not increase the result to a significant level (Table 1). The model reaction was carried out with FU, Fe3O4, and Fe3O4@FU. These results endorsed that Fe3O4@FU was more suitable for this reaction. Overall, the most significant conditions for the desired products were achieved at reflux under ethanol in the presence of 12 mg magnetic nanocomposite.
For total assessment of the synthesis of 2,4,5-trisubstituted and 1,2,4,5-tetrasubstituted imidazoles after the mentioned optimized conditions, various aromatic aldehydes and anilines were evaluated which can be seen in Table 2, including both electron-donating and electron-withdrawing substitutions which were studied in these reactions. While the presence of electron-donating groups resulted in the corresponding products being prepared with lower reaction yields, in addition, electron-withdrawing functionalities led to the higher yields with shorter reaction times. Most of the products directly crystallized from the mixture of reaction with high purity with good to excellent yields (80–96%). It should be mentioned that all the products were confirmed by melting points, and some of the products were characterized by NMR spectral data.
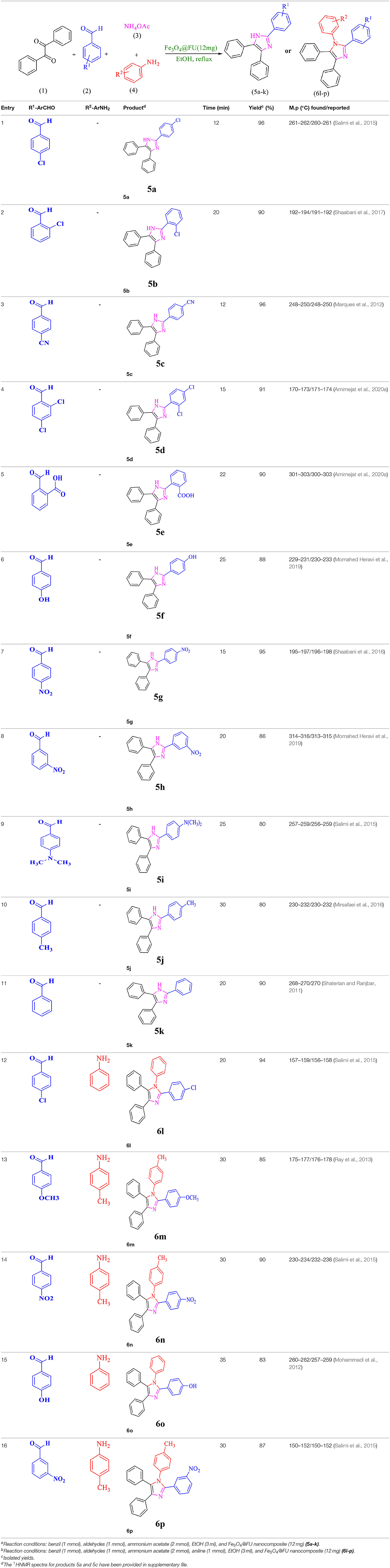
Table 2. Synthesis of 2,4,5-triaryl (5a-k)a and 1,2,4,5- tetraaryl−1H-imidazoles (6l-p)b catalyzed by Fe3O4@FU nanocomposite.
To evaluate the generality of this catalyst in comparison to previously reported results in the literature, Fe3O4@FU acts as an appropriate green biocatalyst due to the yields of products, reaction time and temperature (Table 3).
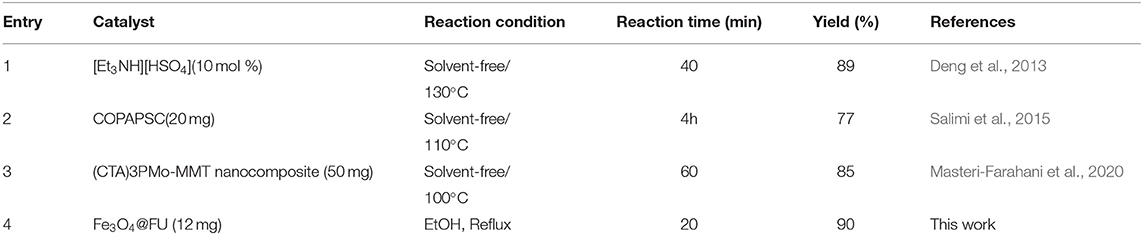
Table 3. Comparison of the activity of the catalysts in synthesis 5k with some of the other catalysts reported in the literature.
The proposed mechanism for the synthesis of tri-substituted imidazoles was shown in Scheme 3. In the first step, the aldehyde and benzil group were activated by the formation of a hydrogen bond with the functional group of fucoidan, followed by the nucleophilic attack of ammonia, coming from the ammonium acetate, the intermediate imine (II) is formed after the removal of H2O. Intermediate (II) is then added to benzil forming intermediate (III). Dehydration of intermediate (III) afforded the intermediate (IV), which rearranges via 1,5 H-shift which, followed by deprotonation, gives tri substituted imidazole (5).
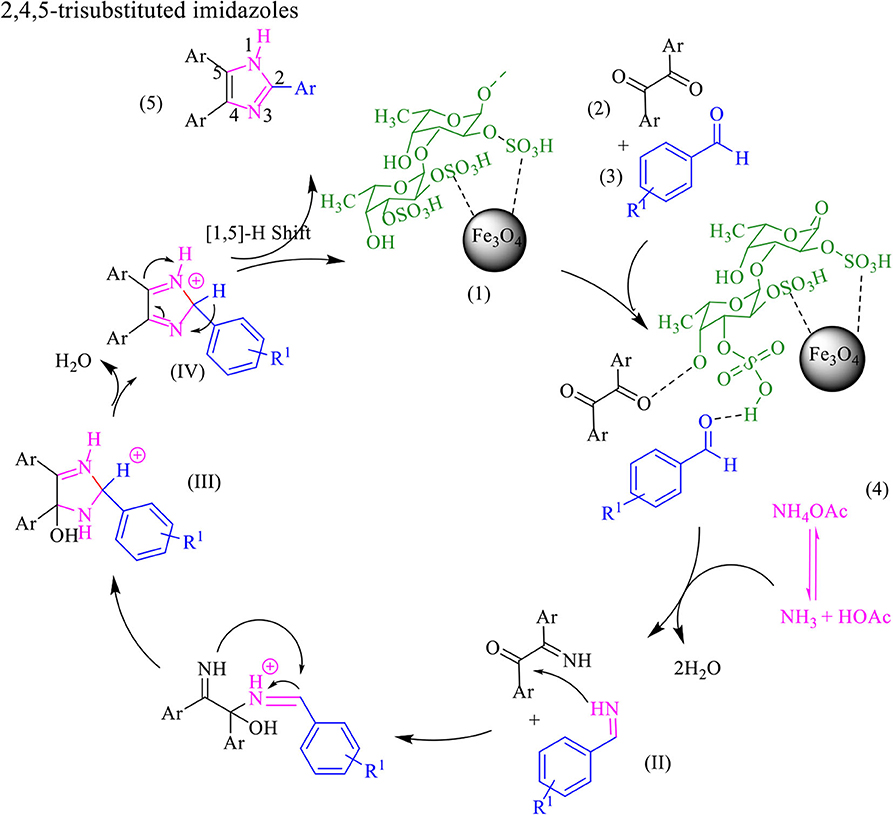
Scheme 3. The proposed mechanism for the synthesis of 2,4,5-trisubstituted imidazoles by using Fe3O4@FU nanocomposite.
The proposed mechanism for the preparation of four one-pot reactions of benzil, aldehyde, and ammonium acetate and amine is shown in Scheme 4. Aldehyde and 1, 2-diketone were first activated by Fe3O4@FU, then amine was added to the aldehydes forming an imine intermediate which was attacked by ammonia (released from the ammonium acetate) to form the amine intermediate (II). On the other hand, the amine intermediate (II) reacted with the activated carbonyl groups of benzil to form the intermediate (III). Finally, the imidazole derivative was formed after dehydration, followed by a 1,5 H-shift.
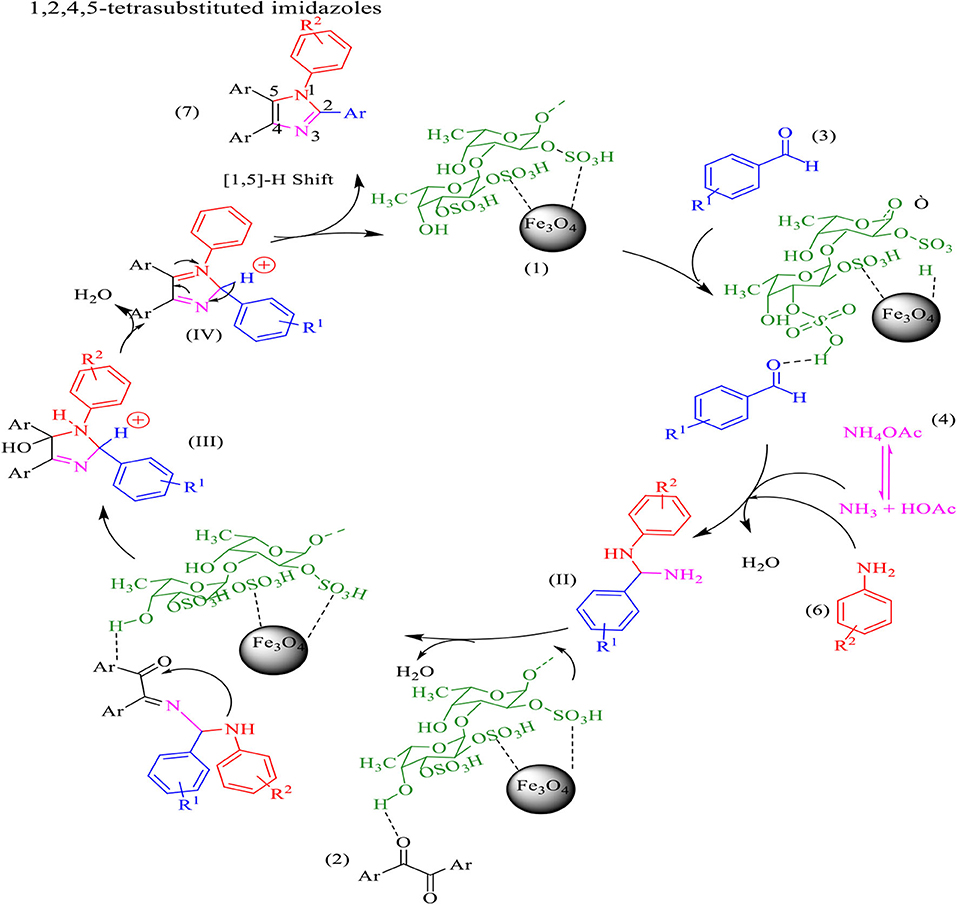
Scheme 4. The proposed mechanism for the synthesis of1, 2,4,5-tetra-substituted imidazoles by using Fe3O4@FU nanocomposite.
The main concerns from an economic and environmental aspect, such as recyclability and the ability to reuse the catalyst, were also surveyed. In this regard, after the reaction was completed, the catalyst was collected by an external magnet and then washed with ethyl acetate, n-hexane and ethanol and dried at 50°C in an oven. The recycled catalyst was used six consecutive times in the reaction. According to the results, no appreciable reduction in the efficiency of the catalysts is observed (Figure 7).
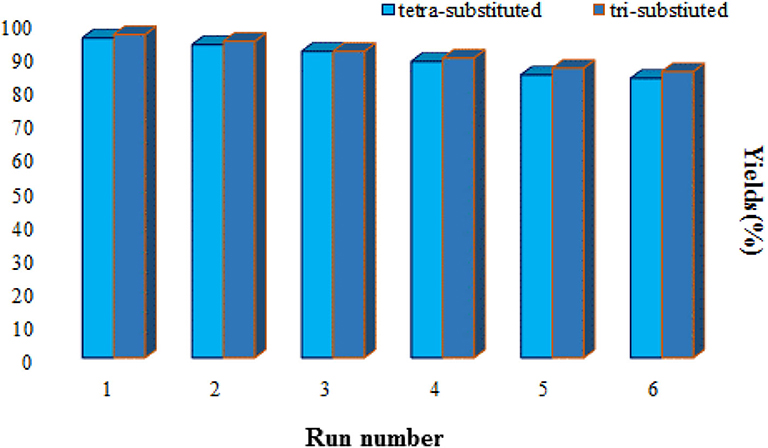
Figure 7. Reusability of Fe3O4@FU for the synthesis of tri-substituted (5a) and tetra-substituted (6l) imidazoles.
The recycled catalyst was identified by EDX and FT-IR analysis. The comparison of the FT-IR spectrum of Fe3O4@FU before and after six consecutive runs confirms that no definite change in its structure was seen, which can therefore be considered as a recyclable and stable biocatalyst in organic reactions (Figure 8). However, the EDX analysis of the recovered catalyst (Figure 9) showed that a degree of catalyst desulfation and leaching occurred after six runs, which explains the decrease in the yield of reactions.
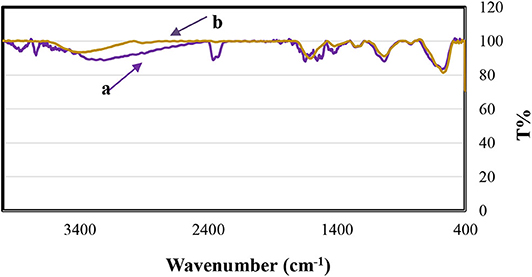
Figure 8. FT-IR spectra of Fe3O4@FU (a) before being used in the reaction, (b) after being used six times in the reaction.
Conclusion
In summary, magnetic core-shell structured fucoidan coated Fe3O4 NPs were in situ synthesized and their structural and magnetic properties were investigated. The catalytic property of Fe3O4@FU was studied in the synthesis of imidazoles derivatives. Outstanding catalytic activity alongside a simple synthesis method, easy processing and separation, a high product yield, and short reaction time make Fe3O4@FU an attractive bio-based heterogeneous catalyst.
Data Availability Statement
The raw data supporting the conclusions of this article will be made available by the authors, without undue reservation.
Author Contributions
MB and SA: investigation and writing—original draft preparation. SJ: project administration, conceptualization, resources, writing—review, and editing. All authors contributed to the article and approved the submitted version.
Conflict of Interest
The authors declare that the research was conducted in the absence of any commercial or financial relationships that could be construed as a potential conflict of interest.
Acknowledgments
The authors wish to express their gratitude for the partial financial support provided by the Research Council of Iran University of Science and Technology (IUST), Tehran, Iran.
Supplementary Material
The Supplementary Material for this article can be found online at: https://www.frontiersin.org/articles/10.3389/fchem.2020.596029/full#supplementary-material
References
Alipour, A., Javanshir, S., and Peymanfar, R. (2018). Preparation, characterization and antibacterial activity investigation of hydrocolloids based Irish moss/ZnO/CuO bio-based nanocomposite films. J. Cluster Sci. 29, 1329–1330. doi: 10.1007/s10876-018-1449-4
Amirnejat, S., Movahedi, F., Masrouri, H., Mohadesi, M., and Kassaee, M. (2013). Silica nanoparticles immobilized benzoylthiourea ferrous complex as an efficient and reusable catalyst for one-pot synthesis of benzopyranopyrimidines. J. Mol. Catal. A Chem. 378, 135–141. doi: 10.1016/j.molcata.2013.05.023
Amirnejat, S., Nosrati, A., and Javanshir, S. (2020c). Superparamagnetic Fe3O4@ Alginate supported L-arginine as a powerful hybrid inorganic–organic nanocatalyst for the one-pot synthesis of pyrazole derivatives. Appl. Organomet. Chem. 34:e5888. doi: 10.1002/aoc.5888
Amirnejat, S., Nosrati, A., Javanshir, S., and Naimi-Jamal, M. R. (2020a). Superparamagnetic alginate-based nanocomposite modified by L-arginine: an eco-friendly bifunctional catalysts and an efficient antibacterial agent. Int. J. Biol. Macromol. 152, 834–845. doi: 10.1016/j.ijbiomac.2020.02.212
Amirnejat, S., Nosrati, A., Peymanfar, R., and Javanshir, S. (2020b). Synthesis and antibacterial study of 2-amino-4H-pyrans and pyrans annulated heterocycles catalyzed by sulfated polysaccharide-coated BaFe12O19 nanoparticles. Res. Chem. Intermed. 46, 3683–3701. doi: 10.1007/s11164-020-04168-x
Dekamin, M. G., Peyman, S. Z., Karimi, Z., Javanshir, S., Naimi-Jamal, M. R., and Barikani, M. (2016). Sodium alginate: an efficient biopolymeric catalyst for green synthesis of 2-amino-4H-pyran derivatives. Int. J. Biol. Macromol. 87, 172–179. doi: 10.1016/j.ijbiomac.2016.01.080
Deng, X., Zhou, Z., Zhang, A., and Xie, G. (2013). Brønsted acid ionic liquid [Et 3 NH][HSO 4] as an efficient and reusable catalyst for the synthesis of 2, 4, 5-triaryl-1H-imidazoles. Res. Chem. Intermed. 39, 1101–1108. doi: 10.1007/s11164-012-0669-8
Dolatkhah, Z., Javanshir, S., Bazgir, A., and Hemmati, B. (2019). Palladium on magnetic Irish moss: a new nano-biocatalyst for suzuki type cross-coupling reactions. Appl. Organomet. Chem. 33:e4859. doi: 10.1002/aoc.4859
Dolatkhah, Z., Javanshir, S., Bazgir, A., and Mohammadkhani, A. (2018). Magnetic isinglass a nano-bio support for copper immobilization: Cu–IG@ Fe3O4 a heterogeneous catalyst for triazoles synthesis. ChemistrySelect 3, 5486–5493. doi: 10.1002/slct.201800501
Fereshteh, N., and Shahrzad, J. (2020). Magnetic γFe2O3@ Sh@ Cu2O: an efficient solid-phase catalyst for reducing agent and base-free click synthesis of 1, 4-disubstituted-1, 2, 3-triazoles. BMC Chem. 14:9. doi: 10.1186/s13065-019-0657-9
Gadekar, L. S., Mane, S. R., Katkar, S. S., Arbad, B. R., and Lande, M. K. (2009). Scolecite as an efficient heterogeneous catalyst for the synthesis of 2, 4, 5-triarylimidazoles. Cent. Eur. J. Chem. 7, 550–554. doi: 10.2478/s11532-009-0050-y
Gomez-Zavaglia, A., Prieto Lage, M. A., Jimenez-Lopez, C., Mejuto, J. C., and Simal-Gandara, J. (2019). The potential of seaweeds as a source of functional ingredients of prebiotic and antioxidant value. Antioxidants 8:406. doi: 10.3390/antiox8090406
Graebin, C. S., Ribeiro, F. V., Rogério, K. R., and Kümmerle, A. E. (2019). Multicomponent reactions for the synthesis of bioactive compounds: a review. Curr. Org. Synth. 16, 855–899. doi: 10.2174/1570179416666190718153703
Hemmati, B., Javanshir, S., and Dolatkhah, Z. (2016). Hybrid magnetic Irish moss/Fe3O4 as a nano-biocatalyst for synthesis of imidazopyrimidine derivatives. RSC Adv. 6, 50431–50436. doi: 10.1039/C6RA08504K
Karimi, A. R., Alimohammadi, Z., Azizian, J., Mohammadi, A. A., and Mohammadizadeh, M. (2006). Solvent-free synthesis of tetrasubstituted imidazoles on silica gel/NaHSO4 support. Catal. Commun. 7, 728–732. doi: 10.1016/j.catcom.2006.04.004
Marques, M. V., Ruthner, M. M., Fontoura, L. A., and Russowsky, D. (2012). Metal chloride hydrates as Lewis acid catalysts in multicomponent synthesis of 2, 4, 5-triarylimidazoles or 2, 4, 5-triaryloxazoles. J. Braz. Chem. Soc. 23, 171–179. doi: 10.1590/S0103-50532012000100024
Masteri-Farahani, M., Ezabadi, A., Mazarei, R., Ataeinia, P., Shahsavarifar, S., and Mousavi, F. (2020). A new nanocomposite catalyst based on clay-supported heteropolyacid for the green synthesis of 2, 4, 5-trisubstituted imidazoles. Appl. Organomet. Chem. 34:e5727. doi: 10.1002/aoc.5727
Mirsafaei, R., Heravi, M. M., Ahmadi, S., and Hosseinnejad, T. (2016). Synthesis and properties of novel reusable nano-ordered KIT-5-N-sulfamic acid as a heterogeneous catalyst for solvent-free synthesis of 2, 4, 5-triaryl-1 H-imidazoles. Chem Pap. 70, 418–429. doi: 10.1515/chempap-2015-0228
Mohammadi, A., Keshvari, H., Sandaroos, R., Maleki, B., Rouhi, H., Moradi, H., et al. (2012). A highly efficient and reusable heterogeneous catalyst for the one-pot synthesis of tetrasubstituted imidazoles. Appl. Catal. A. 429, 73–78. doi: 10.1016/j.apcata.2012.04.011
Momahed Heravi, M., Karimi, N., and Pooremami, S. (2019). One-pot three components synthesis of 2, 4, 5-triaryl-imidazoles catalyzed by Caro's acid-silica gel under solvent–free condition. Adv. J. Chem. A. 2, 73–78. doi: 10.29088/SAMI/AJCA.2019.2.7378
Piri, T., Peymanfar, R., Javanshir, S., and Amirnejat, S. (2019). Magnetic BaFe12O19/Al2O3: an efficient heterogeneous lewis acid catalyst for the synthesis of α-aminophosphonates (Kabachnik–Fields Reaction). Catal. Lett. 149, 3384–3394. doi: 10.1007/s10562-019-02910-8
Pourian, E., Javanshir, S., Dolatkhah, Z., Molaei, S., and Maleki, A. (2018). Ultrasonic-assisted preparation, characterization, and use of novel biocompatible core/shell Fe3O4@ GA@ isinglass in the synthesis of 1, 4-dihydropyridine and 4 H-pyran derivatives. ACS Omega 3, 5012–5020. doi: 10.1021/acsomega.8b00379
Ray, S., Das, P., Bhaumik, A., Dutta, A., and Mukhopadhyay, C. (2013). Covalently anchored organic carboxylic acid on porous silica nano particle: a novel organometallic catalyst (PSNP-CA) for the chromatography-free highly product selective synthesis of tetrasubstituted imidazoles. Appl. Catal. A. 458, 183–195. doi: 10.1016/j.apcata.2013.03.024
Sadeghi, B., Mirjalili, B. B. F., and Hashemi, M. M. (2008). BF3SiO2: an efficient reagent system for the one-pot synthesis of 1, 2, 4, 5-tetrasubstituted imidazoles. Tetrahedron Lett. 49, 2575–2577. doi: 10.1016/j.tetlet.2008.02.100
Salimi, M., Nasseri, M. A., Chapesshloo, T. D., and Zakerinasab, B. (2015). (Carboxy-3-oxopropylamino)-3-propylsilylcellulose as a novel organocatalyst for the synthesis of substituted imidazoles under solvent-free conditions. RSC Adv. 5, 33974–33980. doi: 10.1039/C5RA01909E
Shaabani, A., Afshari, R., and Hooshmand, S. E. (2017). Crosslinked chitosan nanoparticle-anchored magnetic multi-wall carbon nanotubes: a bio-nanoreactor with extremely high activity toward click-multi-component reactions. New J. Chem. 41, 8469–8481. doi: 10.1039/C7NJ01150D
Shaabani, A., Sepahvand, H., Hooshmand, S. E., and Borjian Boroujeni, M. (2016). Design, preparation and characterization of Cu/GA/Fe3O4@ SiO2 nanoparticles as a catalyst for the synthesis of benzodiazepines and imidazoles. Appl. Organomet. Chem. 30, 414–421. doi: 10.1002/aoc.3448
Shalini, K., Sharma, P. K., and Kumar, N. (2010). Imidazole and its biological activities: a review. Der Chemica Sinica 1, 36–47.
Shaterian, H. R., and Ranjbar, M. (2011). An environmental friendly approach for the synthesis of highly substituted imidazoles using Brønsted acidic ionic liquid, N-methyl-2-pyrrolidonium hydrogen sulfate, as reusable catalyst. J. Mol. Liq. 160, 40–49. doi: 10.1016/j.molliq.2011.02.012
Shitole, B. V., Shitole, N. V., Ade, S. B., and Kakde, G. K. (2015). Microwave-induced one-pot synthesis of 2, 4, 5-trisubstituted imidazoles using rochelle salt as a green novel catalyst. Orbital Electron. J. Chem. 7, 240–244. doi: 10.17807/orbital.v7i3.720
Varzi, Z., and Maleki, A. (2019). Design and preparation of ZnS-ZnFe2O4: a green and efficient hybrid nanocatalyst for the multicomponent synthesis of 2, 4, 5-triaryl-1H-imidazoles. Appl. Organomet. Chem. 33:e5008. doi: 10.1002/aoc.5008
Zaheri, H. M., Javanshir, S., Hemmati, B., Dolatkhah, Z., and Fardpour, M. (2018). Magnetic core–shell Carrageenan moss/Fe3O4: a polysaccharide-based metallic nanoparticles for synthesis of pyrimidinone derivatives via Biginelli reaction. Chem. Cent. J. 12:108. doi: 10.1186/s13065-018-0477-3
Zayed, A., and Ulber, R. (2019). Fucoidan production: approval key challenges and opportunities. Carbohydr. Polym. 211, 289–297. doi: 10.1016/j.carbpol.2019.01.105
Zayed, A., and Ulber, R. (2020). Fucoidans: downstream processes and recent applications. Mar. Drugs 18:170. doi: 10.3390/md18030170
Keywords: fucoidan, heterogeneous catalyst, imidazoles, superparamagnetic iron oxide nanoparticles, sulfated polysaccharides
Citation: Banazadeh M, Amirnejat S and Javanshir S (2020) Synthesis, Characterization, and Catalytic Properties of Magnetic Fe3O4@FU: A Heterogeneous Nanostructured Mesoporous Bio-Based Catalyst for the Synthesis of Imidazole Derivatives. Front. Chem. 8:596029. doi: 10.3389/fchem.2020.596029
Received: 18 August 2020; Accepted: 07 October 2020;
Published: 01 December 2020.
Edited by:
Ruquan Ye, City University of Hong Kong, Hong KongReviewed by:
Viorica Parvulescu, Romanian Academy, RomaniaShilpi Ghosh, University of Marburg, Germany
Copyright © 2020 Banazadeh, Amirnejat and Javanshir. This is an open-access article distributed under the terms of the Creative Commons Attribution License (CC BY). The use, distribution or reproduction in other forums is permitted, provided the original author(s) and the copyright owner(s) are credited and that the original publication in this journal is cited, in accordance with accepted academic practice. No use, distribution or reproduction is permitted which does not comply with these terms.
*Correspondence: Shahrzad Javanshir, shjavan@iust.ac.ir