- College of Material, Chemistry and Chemical Engineering, Hangzhou Normal University, Hangzhou, China
Iron(III)-promoted rapid three-component deuteration of quinoxalinones with olefins and NaBD4 is reported for the first time, which provides a novel, economic, and efficient method for the rapid synthesis of deuterated quinoxalinones. In this transformation, a radical pathway is involved according to the results of control experiments.
Introduction
In recent years, deuterium-labeled compounds have received much attention because they play an important role in studying chemical and biological processes (Mutlib, 2008; Gómez-Gallego and Sierra, 2011; Konermann et al., 2011; Simmons and Hartwig, 2012; Atzrodt et al., 2018; Pirali et al., 2019). The incorporation of deuterium is a very efficient strategy not only to measure the kinetic isotope effect and track the reaction path in synthetic chemistry but also to change the absorption, distribution, metabolism, and excretion (ADME) properties of drug candidates in pharmaceutical chemistry (Atzrodt et al., 2007; Meanwell, 2011; Guengerich, 2012; Katsnelson, 2013; Gant, 2014). Since the first deuterated drug, deutetrabenazine, for the treatment of chorea associated with Huntington's disease was approved by the Food and Drug Administration in 2017 (Schmidt, 2017), which clearly proved a route for the development of deuterated drugs in clinical medicine (Scheme 1A) (Junk and Catallo, 1997; Gowrisankar et al., 2012; Tolnai et al., 2014; Ray et al., 2018), considerable interests have been devoted to developing novel and efficient methods for the synthesis of such compounds (Yu et al., 2016; Kerr et al., 2017; Liang et al., 2017; Li et al., 2017; Liu et al., 2018; Yang et al., 2018; Han et al., 2019; Shen et al., 2019; Xu et al., 2019; Zhao et al., 2019; Chang et al., 2020; Dong et al., 2020). For instances, in 2016, Chirik and coworkers reported an iron-catalyzed transformation for the deuteration and tritiation of pharmaceuticals (Yu et al., 2016). Kerr's group developed an iridium-catalyzed hydrogen isotope exchange method for the site-selective deuteration of N-heterocycles (Kerr et al., 2017). In 2012, Fe(III)/NaBH4-mediated free radical hydrofluorination of unactivated alkenes was reported by Boger's group (Barker and Boger, 2012) (Scheme 1B). Subsequently, Liu and coworkers reported a similar method with Fe(III)-promoted free-radical hydroheteroarylation of alkenes (Liang et al., 2017) (Scheme 1B). Dai and Yan, respectively developed novel methods for the synthesis of deuterated arenes by a palladium-catalyzed, pyridine-directed remote meta-C–H bond deuteration of arenes (Xu et al., 2019) or ruthenium catalysis (Zhao et al., 2019). In 2019, Wasa and coworkers demonstrated a B(C6F5)3-catalyzed α-deuteration of carbonyl compounds with D2O, providing an efficient protocol for the synthesis of deuterium labeling carbonyl-based pharmaceuticals (Chang et al., 2020). Despite their utilities, there is still a substantial interest in developing novel and efficient methods for the synthesis of such organic compounds.
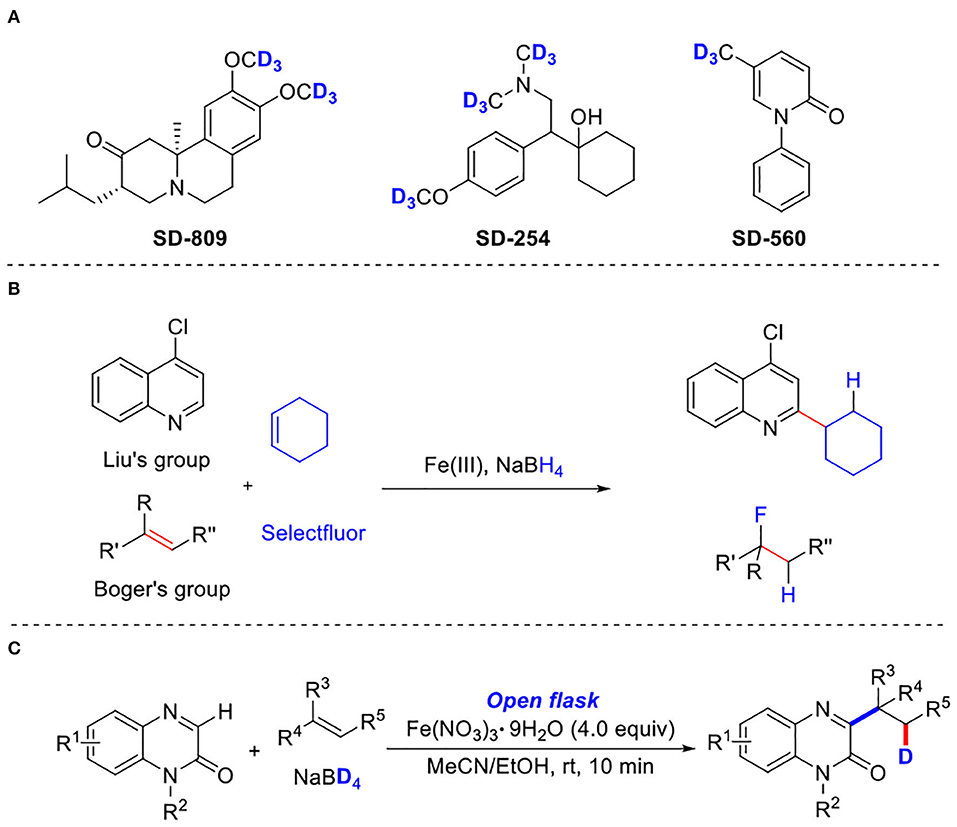
Scheme 1. Three-component deuteration of quinoxalinones. (A) Representative bioactive compounds that contain deuterium. (B) Previous work Fe(III)-promoted three-component deuteration. (C) Present Work: Iron (III)-promoted three-component deuteration.
Multicomponent reactions have become a hot field in modern organic chemistry in recent years because multicomponent reaction can form multiple chemical bonds in one step in comparison with the traditional synthesis method, thus realizing the simple, efficient, and atomic economic synthesis of structural diversity compounds. Quinoxalines and their derivatives are one of the important organic compounds because they have been widely applied in organic synthesis, material chemistry, agrochemical industries, and pharmaceutical chemistry (TenBrink et al., 1994; Monge et al., 1995; Badran et al., 2003; Refaat et al., 2004; Hoogewijs et al., 2013; Nakane et al., 2015; Renault et al., 2017). Although a plenty of two-component reactions for the synthesis of quinoxalinones were achieved (Hong et al., 2019; Jin et al., 2019; Ke et al., 2019; Liu et al., 2019; Wang et al., 2019, 2020; Wei et al., 2019; Xie et al., 2019; Xue et al., 2019; Yan et al., 2019; Zhang H. et al., 2019; Zhang W. et al., 2019; Bao et al., 2020).
Multicomponent transformations were rarely reported. In 2019, Studer and coworkers demonstrated a visible-light-initiated three-component reaction of quinoxalinones, olefins, and perfluoroalkyl iodides (Zheng and Studer, 2019). In the same year, Koley's group disclosed a metal-free domino three-component radical cascde reaction of quinoxalinones, olefins, and sulfinic acids (Dutta et al., 2019).
We also achieved a useful method for the rapid synthesis of quinoxalinone-containing organoazides using three-component cascade reaction of quinoxalinones with olefins and TMSN3 (Shen et al., 2020). Keeping on our interests in developing simple and efficient methods for the synthesis of quinoxalinones (Xu et al., 2019; Zhang H. et al., 2019; Shen et al., 2020), herein, we demonstrated a radical-type three-component deuteration of quinoxalinones with olefins and NaBD4 mediated by Fe(NO3)3•9H2O for the first time (Scheme 1C).
Results and Discussion
Initially, we commenced three-component deuteration of quinoxalinones by the reaction of 1-methylquinoxalin-2(1H)-one (1a), 2.0 equiv of styrene (2a), 1.0 equiv of NaBD4 (3) 4.0 equiv of Fe(NO3)3•9H2O in ethanol at room temperature for 10 min, providing the desired product 4a in 55% yield (Table 1, entry 1). This reaction could not take place if other solvents (MeCN, dichloromethane (DCM), dioxane, dimethylformamide (DMF), dimethyl sulfoxide (DMSO)] (Table 1, entries 2–6) or catalysts (Table 1, entries 12–17) [FeBr3, CuCl2, CuO, (NH4)2Ce(NO3)6, Fe2(ox)3, FeF3], or no catalyst (Table 1, entry 18) were used. However, it surprised us that using the mixed solvent of ethanol and acetonitrile (v/v = 1:1) could improve the reaction yield to 65% (Table 1, entry 7 among entries 7–11). Subsequently, the dosage of styrene 2a and NaBD4 3 were screened (Table 1, entries 19–23). The yield was decreased to 40% when amount of 2a was reduced from 2.0 to 1.0 equiv (Table 1, entry 19). By increasing the amount of NaBD4 3 from 1.0 to 2.0 equiv, a highest yield (73%) was observed (Table 1, entry 23). Furthermore, the product yield could also not be further improved no matter changing the amount of Fe(NO3)3•9H2O (Table 1, entries 24–25) or reaction time (Table 1, entries 26–27). Thus, the highest yield could be obtained when the mixture of 1-methylquinoxalin-2(1H)-one (1a), 2.0 equiv of styrene (2a), 2.0 equiv of NaBD4 (3) in EtOH/CH3CN (4.0 ml, v/v = 1:1) were reacted at 4.0 equiv of Fe(NO3)3•9H2O as oxidant at room temperature for 10 min.
With the optimized reaction conditions in hand, the substrate scope of the three-component deuteration was subsequently explored by using various quinoxalinones (1) with styrene (2a) and NaBD4 (3) (Table 2). To our delight, a wide range of N-protecting groups including N-methyl, N-ethyl, N-butyl, N-cyclopropylmethyl, and N-esteryl groups could work well under standard conditions, affording the target products (4a−4e) in 70–77% yields. Quinoxalinones with various N-benzyl groups or the methoxyl, chloro, bromo, and methyl groups on the benzene ring were also tolerated in this reaction, as demonstrated with products 4f−4q, or 4r−4u in good yields. It was noteworthy that the N-free protecting quinoxalinone was also suitable for the transformation; the product (4v) was obtained in 66% yield. Unfortunately, other N-heterocycles, such as theophylline and 4-hydroxyquinazoline, could not undergo the reaction (see SI).
Some other olefins were then tested by the reaction with 1-methylquinoxalin-2(1H)-one (1a) and NaBD4 (3) (Table 3). It was found that aromatic olefins bearing electron-rich or electron-poor substituents (4aa−4ai) could react smoothly, affording the desired products in good yields. The transformation with nonfunctionalized olefin was also successful, giving the corresponding product 4aj in 75% yield. The multiple substituted olefin (4ak) and cyclic olefin (4al) were also compatible, providing the target products in 60 and 76% yields, respectively (Tang et al., 2015; Yi et al., 2017). In addition, olefins with various ester substituents were also well tolerated, affording the target products (4am−4aq) in good yields. More interestingly, olefins with high-activity functional groups including halo (4ar) and alcohol substituents (4as−4av) also could be converted into corresponding products in good yields (65–79%). However, other olefins containing heteroaromatic ring, such as 2-vinylpyridine, 4-vinylpyridine, and 1-vinyl-2-pyrrolidone could not be transformed into corresponding products (see SI).
To demonstrate the synthetic utility of our method, a gram-scale experiment was performed to synthesize 1-methyl-3-(1-phenylethyl-2-d)quinoxalin-2(1H)-one (4a) in 66% yield (Scheme 2A). It was worth mentioning that the modification of estrone derivative further demonstrated its synthetic utility (Scheme 2B).
To understand the reaction mechanism, the preliminary mechanistic studies was proceeded (Scheme 3). When 2.0 equiv of TEMPO (2,2,6,6-tetramethyl-piperidin-1-oxyl) were used as radical inhibitor, the reaction was completely inhibited (Scheme 3A). In addition, the transformation of 1-methylquinoxalin-2(1H)-one (1a) and diethyl 2,2-diallylmalonate (5a) with NaBD4 (3) performed to give product 6a in 70% yield (Scheme 3B). All these results clearly implied that a radical pathway was responsible for the three-component reaction.
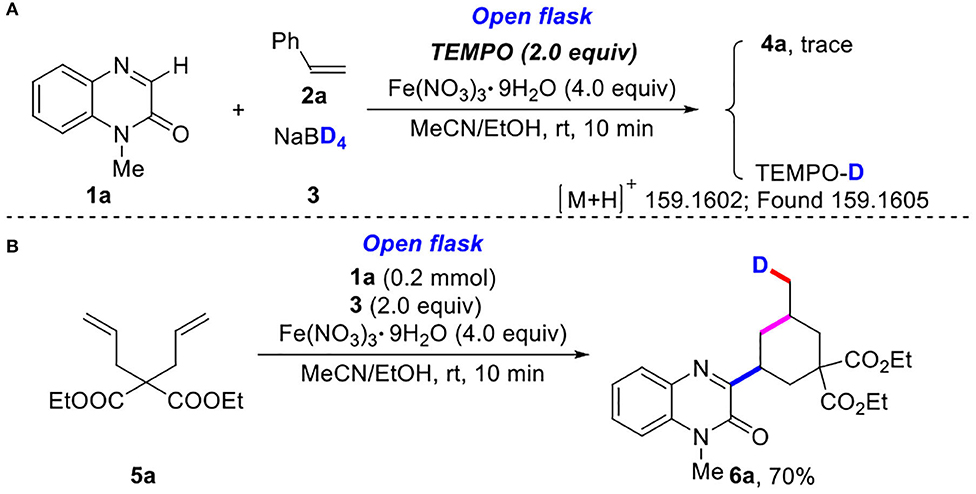
Scheme 3. Control experiments. (A) The experiment of deuterium radical inhibition. (B) The experiment of deuterium radical addition reaction.
Based on the above experimental results and previous reports (Yi et al., 2017; Yan et al., 2019; Shen et al., 2020), a probable radical mechanism for the three-component reaction was proposed (Scheme 4). First, deuterium radical (A) was generated from NaBD4 in the presence of Fe(III). Second, the generated deuterium radical (A) attacked olefin 2a to afford alkyl radical (B). Third, alkyl radical (B) then attacked quinoxalinone 1a to give nitrogen radical (C), which underwent a 1,2-hydrogen shift process to produce carbon radical (D). After the generation of carbon cation (E) from carbon radical (D) by the oxidation of Fe(III), the final product 4a was obtained through a deprotonation process.
Experimental section
General Information
All reagents and deuterated solvents were commercially available and used without further purification. All products were separated by silica gel (200–300 mesh) column chromatography with petroleum ether (PE) (60–90°C) and ethyl acetate (EA). 1H, 13C, and 19F NMR spectra were recorded on a Bruker Advance 500 spectrometer at ambient temperature with CDCl3 as solvent and tetramethylsilane (TMS) as the internal standard. Melting points were determined on an X-5 Data microscopic melting point apparatus. Analytical thin layer chromatography (TLC) was performed on Merk precoated TLC (silica gel 60 F254) plates. Compounds for high-resolution mass spectrometry (HRMS) were analyzed by positive mode electrospray ionization (ESI) using Agilent 6530 QTOF mass spectrometer.
Typical Reaction Procedure for the Cascade Reaction of Quinoxalinones With Unactivated Alkenes and NaBD4
A mixture of quinoxalinones (1) (0.2 mmol), olefins (2) (2.0 equiv), Fe(NO3)3•9H2O (4.0 equiv), and MeCN/EtOH (4.0 ml, v/v = 1:1) in a 15-ml tube was stirred at room temperature for 5 min to make all the components dissolved. Then, NaBD4 (2.0 equiv) was slowly added. The resulting mixture was stirred for another 5 min. After the completion (as indicated by TLC), the reaction mixture was quenched with aqueous NH3•H2O (2 ml) and extracted with EtOAc (5 ml × 3). The collected organic layer was washed with brine and dried with MgSO4. Finally, the organic solvent was removed under reduced pressure, and the obtained residue was purified by silica gel column chromatography (200–300 mesh silica gel, PE/EA = 3:1).
Gram-Scale Synthesis of 1-methyl-3-(1-phenylethyl-2-d)quinoxalin-2(1H)-one
A mixture of quinoxalinones (1) (6.0 mmol), olefins (2) (2.0 equiv), Fe(NO3)3•9H2O (4.0 equiv), and MeCN/EtOH (100 ml, v/v = 1:1) in a 250-ml flask was stirred at room temperature for 5 min to make all the components dissolved. Then, NaBD4 (2.0 equiv) was slowly added. The resulting mixture was stirred for another 5 min. After the completion (as indicated by TLC), the reaction mixture was quenched with aqueous NH3•H2O (50 ml) and extracted with EtOAc (50 ml × 3). The collected organic layer was washed with brine and dried with MgSO4. Finally, the organic solvent was removed under reduced pressure, and the obtained residue was purified by silica gel column chromatography (200–300 mesh silica gel, PE/EA = 3:1) to provide product 4a in 66% yield (1.05 g).
Conclusion
In conclusion, a rapid three-component deuteration of quinoxalinones with olefins and NaBD4 was reported for the first time. Quinoxalinones or olefins bearing various functional groups could undergo the reaction smoothly, producing the target products in moderate to good yields. This transformation gave a novel and efficient method for the synthesis of previously unknown deuterated quinoxalinones.
Data Availability Statement
All datasets presented in this study are included in the article/Supplementary Material.
Author Contributions
WL and PZ contributed conception and design of the study. HC wrote sections of the manuscript. All authors contributed to manuscript revision, read, and approved the submitted version.
Funding
This work was supported by General Scientific Research Projects of Zhejiang Education Department-Special Project on Training Mode Reform of Master of Engineering in Universities (Y201840022).
Conflict of Interest
The authors declare that the research was conducted in the absence of any commercial or financial relationships that could be construed as a potential conflict of interest.
Acknowledgments
We thank the National Natural Science Foundation of China (No. 21871071) and the Key Research and Development Project of Zhejiang (No. 2019C01081) for financial support.
Supplementary Material
The Supplementary Material for this article can be found online at: https://www.frontiersin.org/articles/10.3389/fchem.2020.00606/full#supplementary-material
References
Atzrodt, J., Derdau, V., Kerr, W. J., and Reid, M. (2018). Deuterium- and tritium-labelled compounds: applications in the life sciences. Angew. Chem. Int. Ed. 57, 1758–1784. doi: 10.1002/anie.201704146
Atzrodt, J., Derdau, V. Fey, T., and Zimmermann, J. (2007). The Renaissance of H/D exchange. Angew. Chem. Int. Ed. 46, 7744–7765. doi: 10.1002/anie.200700039
Badran, M. M., Abouzid, K. A. M., and Hussein, M. H. M. (2003). Synthesis of certain substituted quinoxalines as antimicrobial agents (Part II). Arch. Pharm. Res. 26, 107–113. doi: 10.1007/BF02976653
Bao, P., Liu, F., Lv, Y., Yue, H., Li, J.-S., and Wei, W. (2020). Visible-light-promoted acridine red catalyzed aerobic oxidative decarboxylative acylation of α-oxo-carboxylic acids with quinoxalin-2(1H)-ones. Org. Chem. Front. 7, 492–498. doi: 10.1039/C9QO01334B
Barker, T., and Boger, D. (2012). Fe(III)/NaBH4-mediated free radical hydrofluorination of unactivated alkenes. J. Am. Chem. Soc. 134, 13588–13591. doi: 10.1021/ja3063716
Chang, Y., Myers, T., and Wasa, M. (2020). B(C6F5)3-catalyzed α-deuteration of bioactive carbonyl compounds with D2O. Adv. Synth. Catal. 362, 360–364. doi: 10.1002/adsc.201901419
Dong, J., Wang, X., Wang, Z., Song, H., Liu, Y., and Wang, Q. (2020). Formyl-selective deuteration of aldehydes with D2O via synergistic organic and photoredox catalysis. Chem. Sci. 11, 1026–1031. doi: 10.1039/C9SC05132E
Dutta, H. S., Ahmad, A., Khan, A. A., Kumar, M., and Raziullah, K. D. (2019). Metal free benzylation and alkylation of quinoxalin-2(1H)-ones with alkenes triggered by sulfonyl radical generated from sulfinic acids. Adv. Synth. Catal. 361, 5534–5539. doi: 10.1002/adsc.201901212
Gant, T. G. (2014). Using deuterium in drug discovery: leaving the label in the drug. J. Med. Chem. 57, 3595–3611. doi: 10.1021/jm4007998
Gómez-Gallego, M., and Sierra, M. A. (2011). Kinetic isotope effects in the study of organometallic reaction mechanisms. Chem. Rev. 111, 4857–4963. doi: 10.1021/cr100436k
Gowrisankar, S., Neumann, H., and Beller, M. (2012). A convenient and practical synthesis of anisoles and deuterated anisoles by palladium-catalyzed coupling reactions of aryl bromides and chlorides. Chem. Eur. J. 18, 2498–2502. doi: 10.1002/chem.201103347
Guengerich, F. P. (2012). Introduction: use of radioactive compounds in drug discovery and development. Chem. Res. Toxicol. 25, 511–512. doi: 10.1021/tx3000522
Han, X., Yuan, Y., and Shi, Z. (2019). Rhodium-catalyzed selective C-H trideuteromethylation of indole at C7 position using acetic-d6 anhydride. J. Org. Chem. 84, 12764–12772. doi: 10.1021/acs.joc.9b01114
Hong, G., Yuan, J., Fu, J., Pan, G., Wang, Z., Yang, L., et al. (2019). Transition-metal-free decarboxylative C3-difluoroarylmethylation of quinoxalin-2(1H)-ones with α,α-difluoroarylacetic acids. Org. Chem. Front. 6, 1173–1182. doi: 10.1039/C9QO00105K
Hoogewijs, K., Buyst, D., Winne, J. M., Martins, J. C., and Madder, A. (2013). Exploiting furan's versatile reactivity in reversible and irreversible orthogonal peptide labelling. Chem. Commun. 49, 2927–2929. doi: 10.1039/C3CC40588E
Jin, C., Zhuang, X., Sun, B., Li, D., and Zhu, R. (2019). Merging visible-light photoredox and organoamine catalysis for the C-3 difluoroalkylation of quinoxalin-2(1H)-ones. Asian J. Org. Chem. 8, 1490–1494. doi: 10.1002/ajoc.201900369
Junk, T., and Catallo, W. J. (1997). Hydrogen isotope exchange reactions involving C-H (D, T) bonds. Chem. Soc. Rev. 26, 401–406. doi: 10.1039/CS9972600401
Katsnelson, A. (2013). Heavy drugs draw heavy interest from pharma backers. Nat. Med. 19:656. doi: 10.1038/nm0613-656
Ke, Q., Yan, G., Yu, J., and Wu, X. (2019). Recent advances in the direct functionalization of quinoxalin-2(1H)-ones. Org. Biomol. Chem. 17, 5863–5881. doi: 10.1039/C9OB00782B
Kerr, W. J., Lindsay, D. M., Owens, P. K., Reid, M., Tuttle, T., and Campos, S. (2017). Site-selective deuteration of N-heterocycles via iridium-catalyzed hydrogen isotope exchange. ACS Catal. 7, 7182–7186. doi: 10.1021/acscatal.7b02682
Konermann, L., Pan, J., and Liu, Y. H. (2011). Hydrogen exchange mass spectrometry for studying protein structure and dynamics. Chem. Soc. Rev. 40, 1224–1234. doi: 10.1039/C0CS00113A
Li, W., Wang, M.-M., Hu, Y., and Werner, T. (2017). B(C6F5)3-catalyzed regioselective deuteration of electron-rich aromatic and heteroaromatic compounds. Org. Lett. 19, 5768–5771. doi: 10.1021/acs.orglett.7b02701
Liang, B., Wang, Q., and Liu, Z.-Q. (2017). A Fe(III)/NaBH4-promoted free-radical hydroheteroarylation of alkenes. Org. Lett 19, 6463–6465. doi: 10.1021/acs.orglett.7b03313
Liu, C., Chen, Z., Su, C., Zhao, X., Gao, Q., Ning, G.-H., et al. (2018). Controllable deuteration of halogenated compounds by photocatalytic D2O splitting. Nat. Commun. 9:80. doi: 10.1038/s41467-017-02551-8
Liu, L. X., Pan, N., Sheng, W., Su, L., Liu, L., Dong, J. Y., et al. (2019). Visible light-induced regioselective decarboxylative alkylation of the C(sp2)-H bonds of non-aromatic heterocycles. Adv. Synth. Catal. 361, 4126–4132. doi: 10.1002/adsc.201900572
Meanwell, N. A. (2011). Synopsis of some recent tactical application of bioisosteres in drug design. J. Med. Chem. 54, 2529–2591. doi: 10.1021/jm1013693
Monge, A., Martinez-Crespo, F. J., Cerai, A. L., Palop, J. A., Narro, S., Senador, V., et al. (1995). Hypoxia-selective agents derived from 2-quinoxalinecarbonitrile 1,4-Di-iV-oxides. J. Med. Chem. 38, 4488–4494. doi: 10.1021/jm00022a014
Mutlib, A. E. (2008). Application of stable isotope-labeled compounds in metabolism and in metabolism-mediated toxicity studies. Chem. Res. Toxicol. 21, 1672–1689. doi: 10.1021/tx800139z
Nakane, Y., Takeda, T., Hoshino, N., Sakai, K.-I., and Akutagawa, T. (2015). Cation–anion dual sensing of a fluorescent quinoxalinone derivative using lactam–lactim tautomerism. J. Phys. Chem. A 119, 6223–6231. doi: 10.1021/acs.jpca.5b02850
Pirali, T., Serafini, M., Cargnin, S., and Genazzani, A. A. (2019). Applications of deuterium in medicinal chemistry. J. Med. Chem. 62, 5276–5279. doi: 10.1021/acs.jmedchem.8b01808
Ray, P. G., Pawar, Y. D., Singare, D. T., Deshpande, T. N., and Singh, G. P. (2018). Novel process for preparation of tetrabenazine and deutetrabenazine. Org. Process Res. Dev. 22, 520–526. doi: 10.1021/acs.oprd.8b00011
Refaat, H. M., Moneer, A. A., and Khalil, O. M. (2004). Synthesis and antimicrobial activity of certain novel quinoxalines. Arch. Pharm. Res. 27, 1093–1098. doi: 10.1007/BF02975110
Renault, K., Renard, P.-Y., and Sabot, C. (2017). Photophysical properties of quinoxalin-2(1H)-ones: application in the preparation of an azide-based fluorogenic probe for the detection of hydrogen sulphide, New J. Chem. 41, 10432–10437. doi: 10.1039/C7NJ01893B
Schmidt, C. (2017). First deuterated drug approved. Nat. Biotechnol. 35, 493–494. doi: 10.1038/nbt0617-493
Shen, J., Xu, J., Cai, H., Shen, C., and Zhang, P. (2019). Platinum(II)-catalyzed selective para C-H alkoxylation of arylamines through a coordinating activation strategy. Org. Biomol. Chem. 17, 490–497. doi: 10.1039/C8OB02942C
Shen, J., Xu, J., Huang, L., Zhu, Q., and Zhang, P. (2020). Hypervalent iodine(III)-promoted rapid cascade reaction of quinoxalinones with unactivated alkenes and TMSN3. Adv. Synth. Catal. 362, 230–241. doi: 10.1002/adsc.201901314
Simmons, E. M., and Hartwig, J. F. (2012). On the interpretation of deuterium kinetic isotope effects in c-h bond functionalizations by transition-metal complexes. Angew. Chem. Int. Ed. 51, 3066–3072. doi: 10.1002/anie.201107334
Tang, S., Liu, K., Liu, C., and Lei, A. (2015). Olefinic C-H functionalization through radical alkenylation. Chem. Soc. Rev. 44, 1070–1082. doi: 10.1039/C4CS00347K
TenBrink, R. E., Im, W. B., Sethy, V. H., Tang, A. H., and Carter, D. B. (1994). Antagonist, partial agonist, and full agonist imidazo[1,5-a]quinoxaline amides and carbamates acting through the GABAA/benzodiazepine receptor. J. Med. Chem. 37, 758–768. doi: 10.1021/jm00032a008
Tolnai, G. L., Pethö, B., Králl, P., and Novák, Z. (2014). Palladium-catalyzed methoxylation of aromatic chlorides with borate salts. Adv. Synth. Catal. 356, 125–129. doi: 10.1002/adsc.201300687
Wang, J., Sun, B., Zhang, L., Xu, T., Xie, Y., and Jin, C. (2020). Transition-metal-free direct C-3 cyanation of quinoxalin-2(1H)-ones with ammonium thiocyanate as the “CN” source. Org. Chem. Front. 7, 113–118. doi: 10.1039/C9QO01055F
Wang, L., Liu, H., Li, F., Zhao, J., Zhang, H.-Y., and Zhang, Y. (2019). copper-catalyzed C3-H difluoroacetylation of quinoxalinones with ethyl bromodifluoroacetate. Adv. Synth. Catal. 361, 2354–2359. doi: 10.1002/adsc.201900066
Wei, Z., Qi, S., Xu, Y., Liu, H., Wu, J., Li, H.-S., et al. (2019). Visible light-induced photocatalytic C-H perfluoroalkylation of quinoxalinones under aerobic oxidation condition. Adv. Synth. Catal. 361, 5490–5498. doi: 10.1002/adsc.201900885
Xie, L.-Y., Peng, S., Fan, T.-G., Liu, Y.-F., Sun, M., Jiang, L.-L., et al. (2019). Metal-free C3-alkoxycarbonylation of quinoxalin-2(1H)-ones with carbazates as ecofriendly ester sources. Sci. Chin. Chem. 62, 460–464. doi: 10.1007/s11426-018-9446-1
Xu, H., Liu, M., Li, L.-J., Cao, Y.-F., Yu, J.-Q., and Dai, H.-X. (2019). Palladium-catalyzed remote meta-C–H bond deuteration of arenes using a pyridine template. Org. Lett. 21, 4887–4891. doi: 10.1021/acs.orglett.9b01784
Xue, W., Su, Y., Wang, K.-H., Zhang, R., Feng, Y., Cao, L., et al. (2019). Visible-light induced decarboxylative alkylation of quinoxalin-2(1H)-ones at the C3-position. Org. Biomol. Chem. 17, 6654–6661. doi: 10.1039/C9OB01169B
Yan, Z., Sun, B., Zhang, X., Zhuang, X., Yang, J., Su, W., et al. (2019). Construction of C(sp2)-C(sp3) Bond between quinoxalin-2(1H)-ones and N-hydroxyphthalimide esters via photocatalytic decarboxylative coupling. Chem. Asian J. 14, 3344–3349. doi: 10.1002/asia.201900904
Yang, H., Zarate, C., Palmer, W. N., Rivera, N., Hesk, D., and Chirik, P. J. (2018). Site-selective nickel-catalyzed hydrogen isotope exchange in N-heterocycles and its application to the tritiation of pharmaceuticals. ACS Catal. 8, 10210–10218. doi: 10.1021/acscatal.8b03717
Yi, H., Zhang, G., Wang, H., Huang, Z., Wang, J., Singh, A. K., et al. (2017). Recent advances in radical C-H activation/radical cross-coupling. Chem. Rev. 117, 9016–9085. doi: 10.1021/acs.chemrev.6b00620
Yu, R. P., Hesk, D., Rivera, N., Pelczer, I., and Chirik, P. J. (2016). Iron-catalysed tritiation of pharmaceuticals. Nature 529, 195–199. doi: 10.1038/nature16464
Zhang, H., Xu, J., Zhou, M., Zhao, J., Zhang, P., and Li, W. (2019). The visible-light-triggered regioselective alkylation of quinoxalin-2(1H)-ones via decarboxylation coupling. Org. Biomol. Chem. 17, 10201–10208. doi: 10.1039/C9OB02203A
Zhang, W., Pan, Y.-L., Yang, C., Chen, L., Li, X., and Cheng, J.-P. (2019). Metal-free direct C-H cyanoalkylation of quinoxalin-2(1H)-ones by organic photoredox catalysis. J. Org. Chem. 84, 7786–7795. doi: 10.1021/acs.joc.9b00657
Zhao, L.-L., Liu, W., Zhang, Z., Zhao, H., Wang, Q., and Yan, X. (2019). Ruthenium-catalyzed ortho- and meta-H/D exchange of arenes. Org. Lett. 21, 10023–10027. doi: 10.1021/acs.orglett.9b03955
Keywords: deuteration, quinoxalinones, alkenes, three-component, radical pathway
Citation: Li W, Cai H, Huang L, He L, Zhang Y, Xu J and Zhang P (2020) Iron(III)-Mediated Rapid Radical-Type Three-Component Deuteration of Quinoxalinones With Olefins and NaBD4. Front. Chem. 8:606. doi: 10.3389/fchem.2020.00606
Received: 20 April 2020; Accepted: 10 June 2020;
Published: 04 August 2020.
Edited by:
Naohiko Yoshikai, Nanyang Technological University, SingaporeReviewed by:
Ye Wei, Southwest University, ChinaIndubhusan Deb, Indian Institute of Chemical Biology (CSIR), India
Copyright © 2020 Li, Cai, Huang, He, Zhang, Xu and Zhang. This is an open-access article distributed under the terms of the Creative Commons Attribution License (CC BY). The use, distribution or reproduction in other forums is permitted, provided the original author(s) and the copyright owner(s) are credited and that the original publication in this journal is cited, in accordance with accepted academic practice. No use, distribution or reproduction is permitted which does not comply with these terms.
*Correspondence: Wanmei Li, liwanmei@hznu.edu.cn