- 1Faculty of Chemistry, Adam Mickiewicz University, Poznań, Poland
- 2Faculté de Pharmacie, UMR 8076 CNRS, BioCIS, University of Paris-Sud, Univ. Paris-Saclay, Châtenay-Malabry, France
- 3Centre for Advanced Technologies, Adam Mickiewicz University, Poznań, Poland
Mono- and disubstituted 4-CF3 β-lactams at the C-3 position have been obtained stereoselectively under basic conditions. A wide range of function such as alcohols, alkyls, aryls, esters, and double and triple bonds have been introduced.
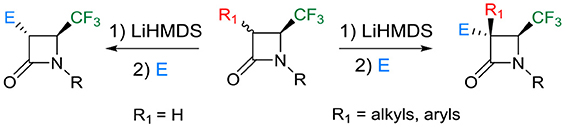
Graphical Abstract. Stereoselective access to C-3 mono- and disubstituted 4-CF3 β-lactams under basic conditions.
Introduction
β-Lactams (2-azetidinones) are of major interest not only for their biological properties, such as antibiotics (Georg, 1993) and enzyme inhibitors (Alcaide et al., 2007), but also for their usefulness as intermediates in organic chemistry, for example, in the synthesis of peptidomimetics and alkaloids (Ojima, 1995). 2-Azetidinones motivated the scientific community to study and exploit them. Thus, several methods of β-lactam synthesis have been developed (Pitts and Letcka, 2014; Hosseyni and Jarrahpour, 2018).
Although fluorine-containing compounds have been widely used in the field of medicinal chemistry (Wang et al., 2014; Zhu et al., 2014; Zhou et al., 2016) due to their pharmacological properties, 4-CF3 monobactams functionalized at C-3 are slightly exploited. The synthesis of 4-CF3 monosubstituted β-lactams at the C-3 position are reported and prepared according to different ways: Staudinger reaction (Guanti et al., 1985; Abouabdellah et al., 1997; Petrik et al., 2011), ring expansion of aziridines via halogen-metal exchange (Decamps et al., 2014), Kinugasa reaction (Kowalski et al., 2016), and the Reformatsky reaction (Trulli et al., 2018). The alkylation reaction with non-fluorinated β-lactams using lithium amide, such as LDA and Li/KHDMS, to obtain the alkylated products at C-3 was well documented in the literature (Kuhlein and Jensen, 1974; Kamath and Ojima, 2012; Deketelaere et al., 2017). Surprisingly, only one example is reported involving the formation of enolate of 4-CF3 β-lactams under the condition of LiHMDS/HMPA with alkyl iodides (Liu et al., 2013) (Scheme 1).
To the best of our knowledge, only few examples of the synthesis of disubstituted β-lactams at the C-3 position have been reported in the literature. We can cite the stereoselective synthesis of 4-CF3-3-fluoroazetidinones via the condensation of 2-fluoropropanethioate lithium enolate with CF3-imines (Ishihara et al., 1996), the addition of enolate to trifluoromethyl-N-para-methoxyphenyl imine that gave a 4:1 diastereomeric mixture of β-lactams (3R,4R, ee: 72%) and (3R,4S, ee: 72%) (Battaglia et al., 2003), and the Wittig rearrangement (Garbi et al., 2001) (Scheme 2).
Results and Discussion
Herein, we disclose the straightforward synthesis of mono- and difunctionalized 4-CF3 β-lactams at the C-3 position. First, 4-CF3 β-lactams 1 and 2 were synthesized according to the literature (Trulli et al., 2018), and the same method was applied to the synthesis of the N-PMBn protected 4-CF3 β-lactam 3. Then, we attempted the C-3 monodeprotonation of the 4-CF3 β-lactams 1–3 (Trulli et al., 2018) with diverse bases such as LDA, LiHMDS, and LTMP. Thus, the addition of benzaldehyde at different conditions (temperature, time, concentration of base) was conducted. The best conditions were achieved when 1.5 eq of LiHMDS in THF at −25°C was applied for 30 min, followed by the addition of 2 eq of aldehyde. The reaction mixture was kept for an additional 2 h at the same temperature and then it was let warm at room temperature overnight. The reaction is stereoselective and the best yields in corresponding 3-functionalized β-lactams 4 were obtained from 1 possessing the para-methoxyphenyl group. From β-lactams 2 and 3, moderate yields were obtained. Our significant results are summarized in the following Table 1. The relative configuration was determined by 1H-NMR according to the coupling constant of cis (3R*, 4S*) (6 Hz) and trans (3S*, 4S*) (3 Hz) between H-3 and H-4. The addition occurred exclusively from the opposite side of the bulky trifluoromethyl substituent. This result has already been observed during our reactions of Li/Br exchange from 3-Br 4-CF3 lactams and aldehydes (Decamps et al., 2014).
With these efficient conditions in hand, other electrophilic reagents have been investigated with the 4-CF3 β-lactam 1 (Table 2). Aldehydes were trapped by the enolate to afford the corresponding 3-functionalized 4-CF3 β-lactams 7 and 8 as a mixture of diastereoisomers. Products were isolated and separated in good yields and with excellent stereoselectivity. The relative configuration of compounds 7 and 8 is trans (3S*, 4S*). Compounds 7 and 8 prepared from Li/Br exchange at −100°C were obtained with an inverse stereoselectivity (Decamps et al., 2014). Next, the reactivity of alkyl halides has been undertaken. When reaction was performed with 1.2 eq of MeI, a mixture of C-3 mono- and disubstituted lactams 9 and 14 was obtained (Table 2, entry 3). Thus, with 2 eq of MeI, the product 14 was exclusively obtained in good yield (68%) (Table 2, entry 4). Similarly, with 1.2 eq of EtI, the same dependence was observed (Table 2, entry 5), but with 2 eq of EtI, C-3 disubstituted product 15 was obtained in much lower yield compared to 14 (Table 2, entry 6), due to the formation of a large number of unexpected products. From 1.2 eq of allyl bromide, C-3 mono- and disubstituted lactams were isolated (Table 2, entry 7). Surprisingly, with the propargyl bromide, only the C-3 monosubstituted azetidinone 12 was obtained (Table 2, entry 8). Regrettably, when 2 eq or more of allyl or propargyl bromide was used, many side products were present in the crude mixture. With another type of electrophile, the ethyl chloroformate, the C-3 monosubstituted lactam was obtained with 1.2 eq, and a mixture of C-3 mono- and disubstituted was observed with 2 eq (Table 2, entries 9 and 10, respectively). No improvement was observed when temperature and solvent, base, and its concentration were modified. Moreover, in almost each case, yields of the products were poor to moderate.
Due to these disappointing results, we moved toward a new pathway that focused on rapid access of C-3 disubstituted 4-CF3 β-lactams from C-3 monosubstituted 4-CF3 β-lactams 9 and 18. Indeed 4-CF3 β-lactams possessing quaternary stereogenic center at C-3 are quite limited. The C-3 monosubstituted 4-CF3 β-lactams 9 and 18 have been prepared using the Reformatsky reaction between CF3-aldimine and methyl- and phenyl-α-bromo esters (Scheme 3). The 3-Me 4-CF3 β-lactams 9 were prepared according to the literature (Trulli et al., 2018) but with shorter reaction time (6 h). For this reason, we observed different ratio of cis (3S*,4S*) and trans (3R*,4S*) isomers (0.5:1, respectively) than was reported. The same method was incorporated to synthesize 3-Ph 4-CF3 β-lactams 18, which were formed also as a mixture of cis (3S*,4S*) and trans (3R*,4S*) isomers (0.8:1, respectively) (Scheme 3).
To synthesize C-3 disubstituted 4-CF3 β-lactams, we started our studies with the previous conditions involving LiHMDS in THF at −25°C and MeI as electrophile with the 3-Me 4-CF3 β-lactams 9. Fortunately, the corresponding C-3 disubstituted compound 14 was obtained in excellent yield (86%). Due to this new result, we investigated first the synthesis of non-symmetrical C-3 disubstituted 4-CF3 β-lactams from 9 (Scheme 4). From alkyl halides such as EtI, allyl, and propargyl bromides corresponding to 4-CF3 β-lactams 19–21 were obtained in reasonable to good yields and in excellent stereochemical purity (Scheme 4). From the ethyl chloroformate, the lactam 22 was obtained in 45% yield. Interestingly, 4-CF3 β-lactams 20–22 are very attractive because of the presence of various functions for functionalizing them or incorporating into bioactive molecules for example. Likewise, the reaction gave very good results with the aldehydes, which led to alcohols 23–25 with an excellent stereoselectivity.
Then, we investigated reactions of 3-Ph 4-CF3 β-lactams 18 with the same electrophiles using the previous conditions (Scheme 5). Unfortunately, with aldehydes, only traces of products were detected. This surprising result could be explained by the steric hindrance of the 3-Ph group in 18 relative to the 3-Me group in 9. Nevertheless, we investigated the reactions with the other electrophiles. In the case of the methyl and ethyl iodides, the reactions occurred to incorporate the methyl and the ethyl group in α position of the phenyl group. Compounds 26 and 27 were isolated in good yield, 73 and 69%, respectively. Reasonable yields were obtained with the allyl and the propargyl bromides, 53% (28) and 47% (29), respectively. The chloroformate reacted with the enolate intermediate, leading to the desired 4-CF3 β-lactam 30 in 50% yield.
All products 14 and 19–30 were isolated pure and with an excellent stereoselectivity. Furthermore, if reactions were performed with lactams 9 or 18 as a cis or trans or in mixture, the results were the same, which means that the enolate intermediate is identical regardless of the ratio cis/trans. Then, the addition of the electrophile occurred at the opposite of the CF3 group. Thus, we can suppose the following mechanism reported in Scheme 6.
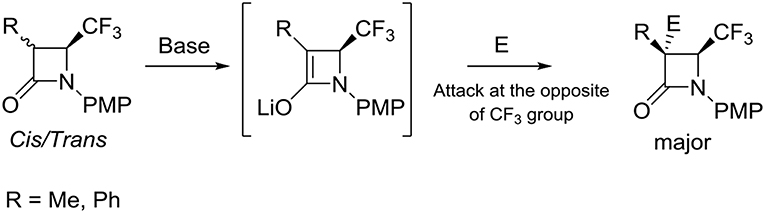
Scheme 6. Stereochemistry of addition of electrophiles to enolate of C-3 substituted 4-CF3 β-lactams.
The configuration of the addition products of different electrophiles to enolate was studied and determined by NMR analysis of compounds 19–30. For each product, 2D 1H-19F HOESY NMR spectra were performed. We observed correlation between the CF3 group and the 3-Me or 3-Ph group and correlation between CF3 and geminal proton due to the bulkiness of this substituent. As an example, for the product 23a, the 1H-19F HOESY spectrum (see Supplementary Figure 1) showed correlations for the both mentioned interactions of the CH3 with CF3 as well as CHCF3 with CF3 on the same intensity level.
Furthermore, the effortless and efficient method of N-PMP deprotection of the selected 4-CF3 β-lactam (14), using ceric ammonium nitrate (CAN) (Jarrahpour and Zarei, 2007), showed great synthetic opportunity toward preparation of β-lactam antibiotics and application in the semi-synthesis of anticancer agents (Zarei et al., 2012) (Scheme 7).
Materials and Methods
Experimental Section
General Methods
1H NMR, 13C NMR, 19F NMR, and 31P NMR spectra were performed on Bruker ASCEND 600 (600 MHz) spectrometers. All 2D and 1D selective NMR spectra were recorded on a Bruker ASCEND 600 (600 MHz) spectrometer. Chemical shifts of 1H NMR were expressed in parts per million downfield from tetramethylsilane (TMS) as an internal standard (δ = 0) in CDCl3. Chemical shifts of 13C NMR were expressed in parts per million downfield and upfield from CDCl3 as an internal standard (δ = 77.0). Chemical shifts of 19F NMR were expressed in parts per million upfield from CFCl3 as an internal standard (δ = 0) in CDCl3. All d.r. were evaluated on the basis of 19F NMR reaction mixture. High-resolution mass spectra were recorded by electron spray (MS-ESI) techniques using a QToF Impact HD Bruker spectrometer. Reagent grade chemicals were used. THF was dried by refluxing with sodium metal-benzophenone (THF) and distilled under argon atmosphere. All moisture-sensitive reactions were carried out under argon atmosphere using oven-dried glassware. Reaction temperatures below 0°C were performed using a cooling bath (liquid N2/n-hexane or liquid N2/i-PrOH). TLC was performed on Merck Kieselgel 60-F254 with EtOAc/n-hexane and MeOH/CHCl3 as developing systems, and products were detected by inspection under UV light (254 nm) and with a solution of potassium permanganate. Merck Kieselgel 60 (0.063–0.200 μm), Merck Kieselgel 60 (0.040–0.063 μm), and Merck Kieselgel 60 (0.015–0.004 μm) were used for column chromatography.
General Procedure of the Synthesis Reformatsky Reaction of PMP-Aldimine With Methyl- and Phenyl-α-Bromo Esters
In a round-bottom flask, zinc (30 mmol) activated by acetic acid, dry THF (15 mL), trifluoromethyl aldimine (25 mmol), and 2-bromo ester (30 mmol) were added under an argon atmosphere. The suspension was warmed to 50 or 60°C and stirred at the same temperature (6 h). The mixture was quenched with saturated aqueous NH4Cl (5 ml) and then extracted with Et2O (7 × 10 ml). The organic layers were washed with brine, dried over MgSO4, filtrated, and concentrated under reduced pressure to give the crude mixture that was purified using column chromatography (hexane/ethyl acetate or cyclohexane/ethyl acetate).
Racemic mixture of (S)-1-(4-methoxyphenyl)-4-(trifluoromethyl)azetidin-2-one (1)
Pale yellow oil (1,530 mg, 65%): The NMR data were in good agreement (Liu et al., 2013).
Racemic mixture of (S)-1-benzyl-4-(trifluoromethyl) azetidin-2-one (2)
Pale yellow oil (1223 mg, 68%): The NMR data were in good agreement (Trulli et al., 2018).
Racemic Mixture of (S)-1-(4-methoxybenzyl)-4-(trifluoromethyl)azetidin-2-one (3)
Pale yellow oil (1004 mg, 71%): 1H NMR (600 MHz, CDCl3) δ = 7.18 (d, J = 8.5 Hz, 2H, Ar), 6.88 (d, J = 8.6 Hz, 2H, Ar), 4.77 (d, J = 15.0 Hz, 1H, CHHPh), 3.92 (d, J = 15.0 Hz, 1H, CHHPh), 3.84–3.76 (m, 4H, CHCF3, OCH3), 3.08 (dd, J = 14.9, 5.3 Hz, 1H, CHHCHCF3), 3.02 (dd, J = 14.9, 1.4 Hz, 1H, CHHCHCF3). 13C NMR (151 MHz, CDCl3) δ = 165.29 (s, C = O), 159.59 (s, Ar), 129.97 (s, Ar), 126.75 (s, Ar), 124.58 (q, J = 279.3 Hz, CF3), 114.45 (s, Ar), 55.39 (s, OCH3), 49.59 (q, J = 35.0 Hz, CHCF3), 45.27 (s, CH2Ar), 38.60 (s, CCHCF3). 19F NMR (565 MHz, CDCl3) δ = −75.29 (d, J = 5.7 Hz). HRMS (ESI) calcd for C12H12F3NO2 ([M+H+]): 260.0898, found: 260.0890.
Racemic mixture of (3S,4S)-1-(4-methoxyphenyl)-3-methyl-4-(trifluoromethyl)azetidin-2-one (cis-9)
Pale yellow oil (353 mg, 25%): The NMR data were in good agreement (Thi et al., 2016).
Racemic mixture of (3R,4S)-1-(4-methoxyphenyl)-3-methyl-4-(trifluoromethyl)azetidin-2-one (trans-9)
Pale yellow oil (692 mg, 49%): The NMR data were in good agreement (Decamps et al., 2014).
Racemic Mixture of (3S,4S)-1-(4-methoxyphenyl)-3-phenyl-4-(trifluoromethyl)azetidin-2-one (cis-18)
Pale yellow oil (609 mg, 34%): 1H NMR (600 MHz, CDCl3) δ = 7.46 (d, J = 8.9 Hz, 2H, Ar), 7.42–7.32 (m, 5H, Ph), 6.93 (d, J = 9.0 Hz, 2H, Ar), 4.94 (d, J = 6.1 Hz, 1H, HCPh), 4.80 (p, J = 6.0 Hz, 1H, CHCF3), 3.81 (s, 3H, OCH3). 13C NMR (151 MHz, CDCl3) δ = 164.56 (s, NC = O), 157.31 (s, Ar), 130.06 (s, Ph), 129.84 (s, Ph), 129.62 (s, Ph), 128.71 (s, Ph), 128.63 (s, Ar), 123.76 (q, J = 281.3 Hz, CF3), 119.97 (s, Ar), 114.57 (s, Ar), 57.19 (q, J = 32.4 Hz, CHCF3), 56.66 (s, CHCHCF3), 55.62 (s, OCH3). 19F NMR (565 MHz, CDCl3) δ = −68.05 (d, J = 5.8 Hz). HRMS (ESI) calcd for C17H14F3NO2 ([M+H+]): 322.1055, found: 322.1039.
Racemic Mixture of (3R,4S)-1-(4-methoxyphenyl)-3-phenyl-4-(trifluoromethyl)azetidin-2-one (trans-18)
Pale yellow oil (771 mg, 43%): 1H NMR (600 MHz, CDCl3) δ = 7.52–7.28 (m, 7H, Ph, Ar), 6.92 (d, J = 8.9 Hz, 2H, Ar), 4.60 (br s, 1H, HCPh), 4.50–4.43 (m, 1H, CHCF3), 3.81 (s, 3H, OCH3). 13C NMR (151 MHz, CDCl3) δ = 163.82 (s, NC = O), 157.35 (s, Ar), 132.75 (s, Ph), 129.72 (s, Ph), 129.38 (s, Ph), 128.62 (s, Ar), 127.48 (s, Ph), 124.34 (q, J = 280.6 Hz, CF3), 119.86 (s, Ar), 114.62 (s, Ar), 59.44 (q, J = 32.4 Hz, CHCF3), 56.25 (d, J = 1.2 Hz, CHCHCF3), 55.65 (s, OCH3). 19F NMR (565 MHz, CDCl3) δ = −72.96 (d, J = 5.8 Hz). HRMS (ESI) calcd for C17H14F3NO2 ([M+H+]): 322.1055, found: 322.1060.
General Procedure of the Reactions of 4-CF3 β-lactams With Electrophiles
In a round-bottom flask, dry THF (2 ml) was cooled to −25°C and then LiHMDS (1.0 M in THF, 0.75 mmol) was added dropwise under an argon atmosphere. Then, the solution of β-lactam (0.5 mmol) in dry THF (1 ml) was added. The suspension was stirred at the same temperature for 30 min and then the electrophile (1 or 0.6 mmol) was added dropwise (p-BrPhCHO was dissolved in 1 ml of dry THF). Then, the reaction mixture was stirred at the same temperature for 2 h and left overnight at room temperature. Then, the reaction mixture was cooled to 0°C and carefully quenched by dropwise addition of saturated aqueous NH4Cl (1 ml), and then extracted with Et2O (2 × 5 ml). The organic layers were washed with brine, dried over MgSO4, filtrated, and concentrated under reduced pressure and purified using column chromatography (hexane/ethyl acetate or cyclohexane/ethyl acetate).
Racemic mixture of 3S,4S)-3-((R)-hydroxy(phenyl) methyl)-1-(4-methoxyphenyl)-4-(trifluoromethyl) azetidin-2-one (4a) and (3S,4S)-3-((S)-hydroxy (phenyl)methyl)-1-(4-methoxyphenyl)-4-(trifluoromethyl)azetidin-2-one (4a and 4b)
Pale yellow oil (145 mg, 83%). The NMR data were in good agreement (Decamps et al., 2014).
Racemic mixture of (3S,4S)-3-((R)-1-benzyl-3-((S)-hydroxy(phenyl)methyl)-4-(trifluoromethyl)azetidin-2-one and (3S,4S)-3-((S)-1-benzyl-3-((S)-hydroxy (phenyl)methyl)-4-(trifluoromethyl)azetidin-2-one (5a and 5b)
Pale yellow oil (95 mg, 57%): The NMR data were in good agreement (Decamps et al., 2014).
Racemic mixture of (3S,4S)-3-((R)-hydroxy(phenyl)methyl)-1-(4-methoxybenzyl)-4-(trifluoromethyl)azetidin-2-one and (3S,4S)-3-((S)-hydroxy(phenyl)methyl)-1-(4-methoxybenzyl)-4-(trifluoromethyl)azetidin-2-one (6a and 6b)
Pale yellow oil (109 mg, 60%): The NMR data were in good agreement (Decamps et al., 2014).
Racemic mixture of (3S,4S)-3-((R)-(4-bromophenyl)(hydroxy)methyl)-1-(4-methoxyphenyl)-4-(trifluoromethyl)azetidin-2-one and (3S,4S)-3-((S)-(4-bromophenyl)(hydroxy)methyl)-1-(4-methoxyphenyl)-4-(trifluoromethyl)azetidin-2-one (7a and 7b)
Pale yellow oil (116 mg, 54%): The NMR data were in good agreement (Decamps et al., 2014).
Racemic mixture of (3S,4S)-3-((R)-hydroxy(4-methoxyphenyl)methyl)-1-(4-methoxyphenyl)-3-methyl-4-(trifluoromethyl)azetidin-2-one and (3S,4S)-3-((S)-hydroxy(4-methoxyphenyl)methyl)-1-(4-methoxyphenyl)-3-methyl-4-(trifluoromethyl)azetidin-2-one (8a and 8b)
Pale yellow oil (109 mg, 57%): 1H NMR (600 MHz, CDCl3) δ = 7.45 (d, J = 8.4 Hz), 7.36 (d, J = 8.6 Hz), 7.34–7.28 (m), 6.95–6.85 (m), 5.30 (br s), 5.08 (br d, J = 4.1 Hz), 4.68–4.60 (m), 4.40 (br s), 3.84–3.77 (m), 3.75 (br s), 3.64 (br s). 13C NMR (151 MHz, CDCl3) δ = 164.52, 163.75, 159.94, 159.52, 157.23, 157.22, 132.62, 131.97, 129.69 (d, J = 7.1 Hz), 128.78, 128.16, 126.57, 124.37 (q, J = 280.2 Hz, CF3), 124.31 (q, J = 280.1 Hz, CF3), 119.98, 119.94, 114.49, 114.26, 114.21, 114.07, 71.34, 68.56, 65.13, 59.33, 58.16, 55.84, 55.60, 55.40, 54.48 (q, J = 34.7 Hz, CHCF3), 52.78 (q, J = 34.8 Hz, CHCF3). 19F NMR (565 MHz, CDCl3) δ = −72.98 (d, J = 4.7 Hz),−72.80 (d, J = 4.7 Hz). HRMS (ESI) calcd for C19H18F3NO4 ([M+Na+]): 404.1086, found: 404.1090.
Racemic mixture of (3R,4S)-3-methyl-1-(4-methoxyphenyl)-4-(trifluoromethyl)azetidin-2-one (9)
Pale yellow oil (19 mg, 15%): The NMR data were in good agreement (Decamps et al., 2014).
Racemic mixture of (3R,4S)-3-ethyl-1-(4-methoxyphenyl)-4-(trifluoromethyl)azetidin-2-one (10)
Pale yellow oil (17 mg, 12%): The NMR data were in good agreement (Gong and Kato, 2001).
Racemic mixture of (3R,4S)-3-allyl-1-(4-methoxyphenyl)-4-(trifluoromethyl)azetidin-2-one (11)
Pale yellow oil (37 mg, 26%): The NMR data were in good agreement (Decamps et al., 2014).
Racemic Mixture of (3R,4S)-1-(4-methoxyphenyl)-3-(prop-2-yn-1-yl)-4-(trifluoromethyl)azetidin-2-one (12)
Pale yellow oil (56 mg, 40%): 1H NMR (600 MHz, CDCl3) δ = 7.36 (d, J = 7.9 Hz, 2H, Ar), 6.89 (d, J = 7.8 Hz, 2H, Ar), 4.46 (br d, J = 4.9 Hz, 1H, CHCF3), 3.80 (s, 3H, OCH3), 3.53 (br t, J = 4.9 Hz, 1H, CHCHCF3), 2.76 (br d, J = 2.7 Hz, 2H, CH2C≡CH), 2.08 (br d, J = 1.4 Hz, 1H, C≡CH). 13C NMR (151 MHz, CDCl3) δ = 163.81 (s, C = O), 157.37 (s, Ar), 129.59 (s, Ar), 124.32 (q, J = 280.3 Hz, CF3), 120.02 (s, Ar), 114.58 (s, Ar), 77.95 (s, C≡CH), 71.96 (s, C≡CH), 56.09 (q, J = 34.8 Hz, CHCF3), 55.64 (s, OCH3), 49.93 (s, CHCHCF3), 17.17 (s, CH2C≡CH). 19F NMR (565 NMR (565 MHz, CDCl3) δ = −72.97 (d, J = 5.0 Hz). HRMS (ESI) calcd for ([M+H+]): 284.0898, found: 284.0894.
Racemic Mixture of Ethyl (3R,4S)-1-(4-methoxyphenyl)-2-oxo-4-(trifluoromethyl)azetidine-3-carboxylate (13)
Pale yellow oil (74 mg, 47%): 1H NMR (600 MHz, CDCl3) δ = 7.35 (d, J = 8.9 Hz, 2H, Ar), 6.90 (d, J = 8.9 Hz, 2H, Ar), 4.86 (dq, J = 5.1, 2.2 Hz, 1H, CHCF3), 4.30 (dq, J = 7.1, 1.1 Hz, 2H, OCH2CH3), 4.21 (d, J = 2.1 Hz, 1H, CHCHCF3), 3.80 (s, 3H, OCH3), 1.34 (t, J = 7.1 Hz, 3H, OCH2CH3). 13C NMR (151 MHz, CDCl3) δ = 164.77 (s, NC = O), 157.72 (s, OC = O), 157.63 (s, Ar), 129.32 (s, Ar), 123.78 (q, J = 280.4 Hz, CF3), 120.05 (s, Ar), 114.62 (s, Ar), 62.95 (s, OCH2CH3), 55.89 (s, CHCHCF3), 55.64 (s, OCH3), 54.26 (q, J = 35.5 Hz, CHCF3), 14.21 (s, OCH2CH3). 19F NMR (565 MHz, CDCl3) δ = −73.15 (d, J = 6.1 Hz). HRMS (ESI) calcd for C14H14F3NO4 ([M+H+]): 318.095318, found: 318.0944.
Racemic Mixture of (S)-1-(4-methoxyphenyl)-3,3-dimethyl-4-(trifluoromethyl)azetidin-2-one (14)
Pale yellow oil (117 mg, 86%): 1H NMR (600 MHz, CDCl3) δ = 7.36 (d, J = 8.9 Hz, 2H, Ar), 6.88 (d, J = 8.9 Hz, 2H, Ar), 4.16 (q, J = 6.4 Hz, 1H, CHCF3), 3.79 (s, 3H, OCH3), 1.48 (s, 3H, CCH3), 1.43 (s, 3H, CCH3). 13C NMR (151 MHz, CDCl3) δ = 170.03 (s, C = O), 157.11 (s, Ar), 130.00 (s, Ar), 124.35 (q, J = 280.9 Hz, CF3), 119.91 (s, Ar), 114.51 (s, Ar), 62.59 (q, J = 32.7 Hz, CHCF3), 55.59 (s, OCH3), 54.02 (s, CCHCF3), 23.38 (s, CCH3), 16.99 (q, J = 2.4 Hz, CCH3). 19F NMR (565 MHz, CDCl3) δ = −68.19 (d, J = 6.0 Hz). HRMS (ESI) calcd for C13H14F3NO2 ([M+H+]): 274.1055, found: 274.1062.
Racemic Mixture of (S)-3-diethyl-1-(4-methoxyphenyl)-4-(trifluoromethyl)azetidin-2-one (15)
Pale yellow oil (49 mg, 33%): 1H NMR (600 MHz, CDCl3) δ = 7.34 (d, J = 7.8 Hz, 2H, Ar), 6.89 (d, J = 7.8 Hz, 2H, Ar), 4.17 (q, J = 5.6 Hz, 1H, CHCF3), 3.79 (s, 3H, OCH3), 2.06 (dq, J = 15.0, 7.8 Hz, 1H, CHHCH3), 1.97 (dq, J = 14.9, 7.6 Hz, 1H, CHHCH3), 1.87–1.72 (m, 2H, CH2CH3), 1.12 (t, J = 7.1 Hz, 3H, CH2CH3), 1.01 (t, J = 7.0 Hz, 3H, CH2CH3). 13C NMR (151 MHz, CDCl3) δ = 169.83 (s, C = O), 157.13 (s, Ar), 129.83 (s, Ar), 124.53 (q, J = 281.0 Hz, CF3), 120.17 (s, Ar), 114.51 (s, Ar), 62.23 (s, CCHCF3), 60.93 (q, J = 32.9 Hz, CHCF3), 55.64 (s, OCH3), 24.92 (s, CH2CH3), 20.62 (d, J = 2.1 Hz, CH2CH3), 8.84 (s, CH2CH3), 8.58 (s, CH2CH3). 19F NMR (565 MHz, CDCl3) δ = −66.86 (d, J = 5.8 Hz). HRMS (ESI) calcd for C15H18F3NO2 ([M+H+]): 302.1368, found: 302.1368.
Racemic Mixture of (S)-3,3-diallyl-1-(4-methoxyphenyl)-4-(trifluoromethyl)azetidin-2-one (16)
Pale yellow oil (69 mg, 43%): 1H NMR (600 MHz, CDCl3) δ = 7.32 (d, J = 8.8 Hz, 2H, Ar), 6.89 (d, J = 8.8 Hz, 2H, Ar), 6.03 (ddt, J = 16.0, 9.3, 5.7 Hz, 1H, HC = CH2), 5.74 (dddd, J = 16.3, 9.9, 8.3, 6.1 Hz, 1H, HC = CH2), 5.29–5.14 (m, 4H, 2 × HC = CH2), 4.29 (q, J = 6.5 Hz, 1H, CHCF3), 3.79 (s, 3H, OCH3), 2.82 (dd, J = 14.3, 4.8 Hz, 1H, CHHCH = CH2), 2.60–2.44 (m, 3H, CHHCH = CH2 and CHHCH = CH2). 13C NMR (151 MHz, CDCl3) δ = 168.36 (s, C = O), 157.31 (s, Ar), 132.45 (s, HC = CH2), 131.78 (s, HC = CH2), 129.40 (s, Ar), 124.52 (q, J = 281.1 Hz, CF3), 120.67 (s, HC = CH2), 120.40 (s, Ar), 119.40 (s, HC = CH2), 114.54 (s, Ar), 59.80 (s, CCHCF3), 59.41 (q, J = 33.3 Hz, CHCF3), 55.64 (s, OCH3), 37.39 (s, CH2CH = CH2), 34.04 (q, J = 2.5 Hz, CH2CH = CH2). 19F NMR (565 MHz, CDCl3) δ = −66.89 (d, J = 6.5 Hz). HRMS (ESI) calcd for C17H18F3NO2 ([M+Na+]): 348.1187, found: 348.1184.
Racemic Mixture of Diethyl (S)-1-(4-methoxyphenyl)-2-oxo-4-(trifluoromethyl)azetidine-3,3-dicarboxylate (17)
Pale yellow oil (68 mg, 35%): 1H NMR (600 MHz, CDCl3) δ = 7.35 (d, J = 8.9 Hz, 2H, Ar), 6.91 (d, J = 9.0 Hz, 2H, Ar), 5.22 (q, J = 6.0 Hz, 1H, CHCF3), 4.43–4.27 (m, 4H, 2 × OCH2CH3), 3.80 (s, 3H, OCH3), 1.34 (t, J = 7.1 Hz, 3H, OCH2CH3), 1.32 (t, J = 7.1 Hz, 3H, OCH2CH3). 13C NMR (151 MHz, CDCl3) δ = 163.00 (s, NC = O), 162.14 (s, OC = O), 158.04 (s, Ar), 156.10 (s, OC = O), 128.58 (s, Ar), 123.07 (q, J = 281.5 Hz, CF3), 121.07 (s, Ar), 114.62 (s, Ar), 70.75 (s, CCHCF3), 64.06 (s, OCH2CH3), 63.34 (s, OCH2CH3), 58.64 (q, J = 35.1 Hz, CHCF3), 55.66 (s, OCH3), 14.07 (s, OCH2CH3), 13.85 (s, OCH2CH3). 19F NMR (565 MHz, CDCl3) δ = −69.36 (d, J = 6.1 Hz). HRMS (ESI) calcd for C17H18F3NO6 ([M+Na+]): 412.0984, found: 412.0982.
Racemic Mixture of (3R, 4S)-3-ethyl-1-(4-methoxyphenyl)-3-methyl-4-(trifluoromethyl)azetidin-2-one (19)
Pale yellow oil (75 mg, 52%): 1H NMR (600 MHz, CDCl3) δ = 7.36 (d, J = 8.9 Hz, 2H, Ar), 6.89 (d, J = 9.0 Hz, 2H, Ar), 4.19 (q, J = 6.5 Hz, 1H, CHCF3), 3.79 (s, 3H, OCH3), 1.88–1.75 (m, 2H, CH2CH3), 1.42 (s, 3H, CCH3), 1.04 (t, J = 7.5 Hz, 3H, CH2CH3). 13C NMR (151 MHz, CDCl3) δ = 169.87 (s, C = O), 157.12 (s, Ar), 129.87 (s, Ar), 124.49 (q, J = 280.9 Hz, CF3), 119.95 (s, Ar), 114.52 (s, Ar), 60.44 (q, J = 32.8 Hz, CHCF3), 58.26 (s, CCHCF3), 55.61 (s, OCH3), 29.56 (s, CH2CH3), 14.51 (q, J = 2.6 Hz, CCH3), 8.91 (s, CH2CH3). 19F NMR (565 MHz, CDCl3) δ = −67.73 (d, J = 6.0 Hz). HRMS (ESI) calcd for C14H16F3NO2 ([M+H+]): 288.1211, found: 288.1214.
Racemic Mixture of (3R,4S)-3-allyl-1-(4-methoxyphenyl)-3-methyl-4-(trifluoromethyl)azetidin-2-one (20)
Pale yellow oil (106 mg, 71%): 1H NMR (600 MHz, CDCl3) δ = 7.34 (d, J = 8.9 Hz, 2H, Ar), 6.88 (d, J = 8.9 Hz, 2H, Ar), 5.83–5.73 (m, 1H, HC = CH2), 5.27–5.18 (m, 2H, HC = CH2), 4.25 (q, J = 6.5 Hz, 1H, CHCF3), 3.78 (s, 3H, OCH3), 2.53 (dd, J = 14.1, 6.5 Hz, 1H, CHHCH = CH2), 2.46 (dd, J = 14.1, 8.1 Hz, 1H, CHHCH = CH2), 1.44 (s, 3H, CCH3). 13C NMR (151 MHz, CDCl3) δ = 169.11 (s, C = O), 157.18 (s, Ar), 131.90 (s, HC = CH2), 129.60 (s, Ar), 124.48 (q, J = 280.9 Hz, CF3), 120.37 (s, HC = CH2), 120.09 (s, Ar), 114.48 (s, Ar), 59.66 (q, J = 32.9 Hz, CHCF3), 57.09 (s, CCHCF3), 55.55 (s, OCH3), 40.84 (s, CH2CH = CH2), 15.32 (q, J = 2.5 Hz, CCH3). 19F NMR (565 MHz, CDCl3) δ = −67.68 (d, J = 6.0 Hz). HRMS (ESI) calcd for C15H16F3NO2 ([M+H+]): 300.1211, found: 300.1216.
Racemic Mixture of (3R, 4S)-1-(4-methoxyphenyl)-3-methyl-3-(prop-2-yn-1-yl)-4-(trifluoromethyl)azetidin-2-one (21)
Pale yellow oil (96 mg, 65%): 1H NMR (600 MHz, CDCl3) δ = 7.36 (d, J = 8.9 Hz, 2H, Ar), 6.89 (d, J = 9.0 Hz, 2H, Ar), 4.51 (q, J = 6.4 Hz, 1H, CHCF3), 3.79 (s, 3H, OCH3), 2.68 (dd, 1H, J = 17.1, 2.4, CHHC≡CH), 2.59 (dd, 1H, J = 17.1, 2.4, CHHC≡CH), 2.09 (t, J = 2.4 Hz, 1H, C≡CH), 1.50 (s, CCH3). 13C NMR (151 MHz, CDCl3) δ = 167.83 (s, C = O), 157.39 (s, Ar), 129.33 (s, Ar), 124.30 (q, J = 280.8 Hz, CF3), 120.47 (s, Ar), 114.54 (s, Ar), 78.15 (s, C≡CH), 72.26 (s, C≡CH), 60.18 (q, J = 33.2 Hz, CHCF3), 56.42 (s, CCHCF3), 55.61 (s, OCH3), 26.26 (s, CH2C≡CH), 15.27 (q, J = 2.4 Hz, CCH3). 19F NMR (565 MHz, CDCl3) δ = −67.88 (d, J = 6.0 Hz). HRMS (ESI) calcd C15H14F3NO2 ([M+H+]): 298.1055, found: 298.1057.
Racemic Mixture of Ethyl (3R,4S)-1-(4-methoxyphenyl)-3-methyl-2-oxo-4-(trifluoromethyl)azetidine-3-carboxylate (22)
Pale yellow oil (98 mg, 45%): 1H NMR (600 MHz, CDCl3) δ = 7.36 (d, J = 8.9 Hz, 2H, Ar), 6.90 (d, J = 8.9 Hz, 2H, Ar), 4.96 (q, J = 6.3 Hz, 1H, CHCF3), 4.33–4.23 (m, 2H, OCH2CH3), 3.80 (s, 3H, OCH3), 1.72 (s, 3H, CCH3), 1.31 (t, J = 7.1 Hz, 3H, OCH2CH3). 13C NMR (151 MHz, CDCl3) δ = 168.17 (s, NC = O), 162.59 (s, OC = O), 157.65 (s, Ar), 129.19 (s, Ar), 123.81 (q, J = 281.1 Hz, CF3), 120.58 (s, Ar), 114.60 (s, Ar), 62.96 (s, OCH2CH3), 62.45 (s, CCHCF3), 58.83 (q, J = 33.7 Hz, CHCF3), 55.63 (s, OCH3), 14.17 (s, OCH2CH3), 13.02 (q, J = 2.5 Hz, CCH3). 19F NMR (565 MHz, CDCl3) δ = −67.70 (d, J = 6.3 Hz). HRMS (ESI) calcd for C15H16F3NO4 ([M+H+]): 332.1110, found: 332.1102.
Racemic mixture of diastereomer A of (3S,4S)-3-(hydroxy(phenyl)methyl)-1-(4-methoxyphenyl)-3-methyl-4-(trifluoromethyl)azetidin-2-one (23a)
Pale yellow oil (86 mg, 44%): 1H NMR (600 MHz, CDCl3) δ = 7.47 (d, J = 7.2 Hz, 2H, Ph), 7.37–7.27 (m, 3H, Ph), 7.14 (d, J = 8.8 Hz, 2H, Ar), 6.80 (d, J = 8.9 Hz, 2H, Ar), 4.85 (s, 1H, HCOH), 4.59 (q, J = 6.5 Hz, 1H, CHCF3), 3.75 (s, 3H, OCH3), 2.87 (br d, J = 15.9 Hz, 1H, OH), 1.39 (s, 3H CCH3). 13C NMR (151 MHz, CDCl3) δ = 168.01 (s, NC = O), 157.36 (s, Ar), 138.90 (s, Ph), 129.03 (s, Ar), 128.74 (s, Ph), 128.55 (s, Ph), 127.45 (s, Ph), 124.54 (q, J = 280.9 Hz, CF3), 120.90 (s, Ar), 114.37 (s, Ar), 76.63 (s, HCOH), 62.33 (s, CCHCF3), 58.03 (q, J = 32.8 Hz, CHCF3), 55.51 (s, OCH3), 13.16 (d, J = 1.8 Hz, CCH3). 19F NMR (565 MHz, CDCl3) δ = −67.43 (d, J = 5.8 Hz). HRMS (ESI) calcd for C19H18F3NO3 ([M+H+]): 366.1317, found: 366.1318.
Racemic mixture of diastereomer B of (3S,4S)-3-(hydroxy(phenyl)methyl)-1-(4-methoxyphenyl)-3-methyl-4-(trifluoromethyl)azetidin-2-one (23b)
Pale yellow oil (43 mg, 23%): 1H NMR (600 MHz, CDCl3) δ = 7.41–7.30 (m, 7H, Ph, Ar), 6.87 (d, J = 9.1 Hz, 2H, Ar), 4.98 (q, J = 6.7 Hz, 1H, CHCF3), 4.87 (s, 1H, HCOH), 3.79 (s, 3H, OCH3), 3.80 (br s, 1H, OH), 1.24 (s, 3H CCH3). 13C NMR (151 MHz, CDCl3) δ = 169.42 (s, NC = O), 157.35 (s, Ar), 139.22 (s, Ph), 129.40 (s, Ar), 128.72 (s, Ph), 127.49 (s, Ph), 126.97 (s, Ph), 124.45 (q, J = 280.8 Hz, CF3), 120.74 (s, Ar), 114.50 (s, Ar), 74.80 (s, HCOH), 62.40 (s, CCHCF3), 56.37 (q, J = 33.0 Hz, CHCF3), 55.59 (s, OCH3), 13.46 (d, J = 1.9 Hz, CCH3). 19F NMR (565 MHz, CDCl3) δ = −67.01 (d, J = 6.3 Hz). HRMS (ESI) calcd for C19H18F3NO3 ([M+H+]): 366.1317, found: 366.1316.
Racemic mixture of diastereomer A of (3S,4S)-3-((4-bromophenyl)(hydroxy)methyl)-1-(4-methoxyphenyl)-3-methyl-4-(trifluoromethyl)azetidin-2-one (24a)
Pale yellow oil (70 mg, 32%): 1H NMR (600 MHz, CDCl3) δ = 7.49 (d, J = 8.4 Hz, 2H, 4-BrPh), 7.38 (d, J = 8.3 Hz, 2H, 4-BrPh), 7.21 (d, J = 8.9 Hz, 2H, 4-MeOPh), 6.85 (d, J = 8.9 Hz, 2H, 4-MeOPh), 4.87 (s, 1H, HCOH), 4.59 (q, J = 6.5 Hz, 1H, CHCF3), 3.78 (s, 3H, OCH3), 2.49 (br s, 1H, OH), 1.37 (s, 3H CCH3). 13C NMR (151 MHz, CDCl3) δ = 167.60 (s, NC = O), 157.51 (s, 4-MeOPh), 137.84 (s, 4-BrPh), 131.83 (s, 4-BrPh), 129.33 (s, 4-BrPh), 129.09 (s, 4-MeOPh), 124.46 (q, J = 280.9 Hz, CF3), 123.01 (s, 4-BrPh), 120.75 (s, 4-MeOPh), 114.52 (s, 4-MeOPh), 76.36 (s, HCOH), 62.03 (s, CCHCF3), 58.17 (q, J = 32.9 Hz, CHCF3), 55.63 (s, OCH3), 13.09 (q, J = 2.6 Hz, CCH3). 19F NMR (565 MHz, CDCl3) δ = −67.40 (d, J = 5.9 Hz). HRMS (ESI) calcd for C19H17BrF3NO3 ([M+H+]): 444.0422 and 446.0402, found: 444.0404 and 446.0386.
Racemic mixture of diastereomer B of (3S,4S)-3-((4-bromophenyl)(hydroxy)methyl)-1-(4-methoxyphenyl)-3-methyl-4-(trifluoromethyl)azetidin-2-one (24b)
Pale yellow oil (87mg, 39%): 1H NMR (600 MHz, CDCl3) δ = 7.51 (d, J = 8.3 Hz, 2H, 4-BrPh), 7.21 (d, J = 8.2 Hz, 2H, 4-BrPh), 7.36 (d, J = 8.9 Hz, 2H, 4-MeOPh), 6.88 (d, J = 8.9 Hz, 2H, 4-MeOPh), 4.91 (q, J = 6.6 Hz, 1H, CHCF3), 4.86 (s, 1H, HCOH), 3.80 (s, 3H, OCH3), 2.96 (br s, 1H, OH), 1.24 (s, 3H CCH3). 13C NMR (151 MHz, CDCl3) δ = 169.02 (s, NC = O), 157.49 (s, 4-MeOPh), 138.19 (s, 4-BrPh), 131.86 (s, 4-BrPh), 129.27 (s, 4-MeOPh), 128.62 (s, 4-BrPh), 124.35 (q, J = 280.6 Hz, CF3), 122.78 (s, 4-BrPh), 120.73 (s, 4-MeOPh), 114.56 (s, 4-MeOPh), 74.22 (s, HCOH), 62.24 (s, CCHCF3), 56.32 (q, J = 33.0 Hz, CHCF3), 55.64 (s, OCH3), 13.39 (d, J = 2.0 Hz, CCH3). 19F NMR (565 MHz, CDCl3) δ = −67.02 (d, J = 6.0 Hz). HRMS (ESI) calcd for C19H17BrF3NO3 ([M+H+]): 444.0422 and 446.0402, found: 444.0416 and 446.0399.
Racemic mixture of (3S,4S)-3-((R)-hydroxy(4-methoxyphenyl)methyl)-1-(4-methoxyphenyl)-3-methyl-4-(trifluoromethyl)azetidin-2-one and (3S,4S)-3-((S)-hydroxy(4-methoxyphenyl)methyl)-1-(4-methoxyphenyl)-3-methyl-4-(trifluoromethyl)azetidin-2-one (25a and 25b)
Pale yellow oil (102 mg, 52%): 1H NMR (600 MHz, CDCl3) δ = 7.43 (d, J = 8.6 Hz), 7.38 (d, J = 8.9 Hz), 7.27 (d, J = 6.9 Hz), 7.22 (d, J = 8.9 Hz), 7.00–6.82 (m), 4.95 (q, J = 6.8 Hz), 4.86 (br d, J = 5.6 Hz), 4.65–4.55 (m), 3.88–3.76 (m), 2.61 (br s), 2.41 (br s), 1.38 (s), 1.25 (s). 13C NMR (151 MHz, CDCl3) δ = 169.35, 167.98, 159.99, 159.94, 157.35, 131.25, 130.99, 129.52, 129.29, 128.86, 128.17, 124.60 (q, J = 280.9 Hz, CF3), 124.53 (q, J = 281.0 Hz, CF3), 120.75, 120.69, 114.51, 114.44, 114.10, 114.04, 76.59, 74.71, 65.19, 62.48, 62.45, 58.15 (q, J = 32.9 Hz, CHCF3), 56.45 (q, J = 32.9 Hz, CHCF3), 55.63, 55.60, 55.43, 13.47 (q, J = 2.0 Hz, CCH3), 13.18 (q, J = 2.3 Hz, CCH3). 19F NMR (565 MHz, CDCl3) δ = −67.41 (d, J = 6.1 Hz),−66.96 (d, J = 6.4 Hz). HRMS (ESI) calcd for C20H20F3NO4 ([M+Na+]): 418.1242, found: 418.1243.
Racemic Mixture of (3S,4S)-1-(4-methoxyphenyl)-3-methyl-3-phenyl-4-(trifluoromethyl)azetidin-2-one (26)
Pale yellow oil (122 mg, 73%): 1H NMR (600 MHz, CDCl3) δ = 7.54–7.31 (m, 7H, Ph, Ar), 6.93 (d, J = 9.0 Hz, 2H, Ar), 4.46 (q, J = 6.0 Hz, 1H, CHCF3), 3.81 (s, 3H, OCH3), 1.90 (s, 3H, CH3). 13C NMR (151 MHz, CDCl3) δ = 168.41 (s, C = O), 157.40 (s, Ar), 135.19 (s, Ph), 129.80 (s, Ph), 128.51 (s, Ph), 128.29 (s, Ar), 127.59 (s, Ph), 123.60 (q, J = 281.2 Hz, CF3), 120.50 (s, Ar), 114.53 (s, Ar), 64.41 (q, J = 32.0 Hz, CHCF3), 61.49 (s, CCHCF3), 55.61 (s, OCH3), 24.12 (s, CCH3). 19F NMR (565 MHz, CDCl3) δ = −68.39 (d, J = 5.7 Hz). HRMS (ESI) calcd for C18H16F3NO2 ([M+H+]): 336.1211, found: 336.1211.
Racemic Mixture of (3S,4S)-3-ethyl-1-(4-methoxyphenyl)-3-phenyl-4-(trifluoromethyl)azetidin-2-one (27)
Pale yellow oil (120 mg, 69%): 1H NMR (600 MHz, CDCl3) δ = 7.54–7.30 (m, 7H, Ph, Ar), 6.92 (d, J = 8.9 Hz, 2H, Ar), 4.45 (q, J = 5.8 Hz, 1H, CHCF3), 3.81 (s, 3H, OCH3), 2.37 (dq, J = 14.8, 7.4 Hz, 1H, CHHCH3), 2.27 (dq, J = 14.4, 7.3 Hz, 1H, CHHCH3), 0.98 (t, J = 7.3 Hz, 3H, CH2CH3). 13C NMR (151 MHz, CDCl3) δ = 167.77 (s, C = O), 157.38 (s, Ar), 134.23 (s, Ph), 129.63 (s, Ph), 128.52 (s, Ph), 128.20 (s, Ar), 127.88 (s, Ph), 123.86 (q, J = 281.3 Hz, CF3), 120.56 (s, Ar), 114.55 (s, Ar), 65.89 (s, CCHCF3), 62.57 (q, J = 31.8 Hz, CHCF3), 55.66 (s, OCH3), 31.34 (s, CH2CH3), 9.18 (s, CH2CH3). 19F NMR (565 MHz, CDCl3) δ = −68.08 (d, J = 5.6 Hz). HRMS (ESI) calcd for C19H18F3NO2 ([M+Na+]): 372.1187, found: 372.1192.
Racemic Mixture of (3S,4S)-3-allyl-1-(4-methoxyphenyl)-3-phenyl-4-(trifluoromethyl)azetidin-2-one (28)
Pale yellow oil (96 mg, 53%): 1H NMR (600 MHz, CDCl3) δ = 7.56–7.29 (m, 7H, Ph, Ar), 6.91 (d, J = 8.9 Hz, 2H, Ar), 5.84–5.73 (m, 1H, HC = CH2), 5.31 (br d, J = 17.0 Hz, 1H, HC = CHH), 5.20 (br d, J = 10.2 Hz, 1H, HC = CHH), 4.53 (q, J = 6.0 Hz, 1H, CHCF3), 3.81 (s, 3H, OCH3), 2.95 (d, J = 7.2 Hz, 2H, CH2CH = CH2). 13C NMR (151 MHz, CDCl3) δ = 167.12 (s, C = O), 157.53 (s, Ar), 134.64 (s, Ph), 131.87 (s, HC = CH2), 129.16 (s, Ph), 128.58 (s, Ph), 128.35 (s, Ar), 127.75 (s, Ph), 123.96 (q, J = 281.3 Hz, CF3), 121.17 (s, HC = CH2), 121.05 (s, Ar), 114.54 (s, Ar), 64.74 (s, CCHCF3), 61.07 (q, J = 32.0 Hz, CHCF3), 55.65 (s, OCH3), 41.72 (s, CH2CH = CH2). 19F NMR (565 MHz, CDCl3) δ = −67.92 (d, J = 5.6 Hz). HRMS (ESI) calcd for C20H18F3NO2 ([M+Na+]): 384.1187, found: 384.1193.
Racemic Mixture of (3S,4S)-1-(4-methoxyphenyl)-3-phenyl-3-(prop-2-yn-1-yl)-4-(trifluoromethyl)azetidin-2-one (29)
Pale yellow oil (84 mg, 47%): 1H NMR (600 MHz, CDCl3) δ = 7.55–7.32 (m, 7H, Ph, Ar), 6.92 (d, J = 9.0 Hz, 2H, Ar), 4.90 (q, J = 6.1 Hz, 1H, CHCF3), 3.81 (s, 3H, OCH3), 3.17 (dd, J = 17.3, 2.6, 1H, CHHC≡CH), 2.97 (dd, J = 17.3, 2.4, 1H, CHHC≡CH), 2.10 (t, J = 2.5 Hz, 1H, C≡CH). 13C NMR (151 MHz, CDCl3) δ = 166.17 (s, C = O), 157.66 (s, Ar), 133.69 (s, Ph), 128.91 (s, Ar), 128.78 (s, Ph), 128.73 (s, Ph), 127.62 (s, Ph), 123.78 (q, J = 280.9 Hz, CF3), 121.33 (s, Ar), Ar), 114.49 (s, Ar), 78.21 (s, C≡CH), 72.55 (s, C≡CH), 63.68 (s, CCHCF3), 61.39 (q, J = 32.4 Hz, CHCF3), 55.61 (s, OCH3), 26.86 (s, CH2C≡CH). 19F NMR (565 MHz, CDCl3) δ = −68.05 (d, J = 6.3 Hz). HRMS (ESI) calcd for C20H16F3NO2 ([M+Na+]): 382.1030 found: 382.1022.
Racemic Mixture of Ethyl (3R,4S)-1-(4-methoxyphenyl)-2-oxo-3-phenyl-4-(trifluoromethyl)azetidine-3-carboxylate (30)
Pale yellow oil (74 mg, 50%): 1H NMR (600 MHz, CDCl3) δ = 7.50–7.35 (m, 7H, Ph, Ar), 6.92 (d, J = 8.9 Hz, 2H, Ar), 5.39 (q, J = 5.8 Hz 1H, CHCF3), 4.34–4.20 (m, 2H, OCH2CH3), 3.81 (s, 3H, OCH3), 1.20 (t, J = 7.1 Hz, 3H, OCH2CH3). 13C NMR (151 MHz, CDCl3) δ = 167.50 (s, NC = O), 160.67 (s, OC = O), 157.79 (s, Ar), 130.49 (s, Ph), 129.17 (s, Ph), 129.05 (s, Ph), 128.81 (s, Ph), 128.39 (s, Ar), 123.26 (q, J = 281.3 Hz, CF3), 120.92 (s, Ar), 114.57 (s, Ar), 71.52 (s, CCHCF3), 63.36 (s, OCH2CH3), 60.36 (q, J = 32.6 Hz, CHCF3), 55.64 (s, OCH3), 13.99 (s, OCH2CH3). 19F NMR (565 MHz, CDCl3) δ = −68.00 (d, J = 5.5 Hz). HRMS (ESI) calcd for C20H18F3NO4 ([M+H+]): 394.1266, found: 394.1262.
Procedure of the N-PMP deprotection of 4-CF3 β-lactams
A solution of β-lactam 14 (0.25 mmol) in MeCN (1.5 ml) was cooled to 0°C and then CAN (0.75 mmol) in water (1 ml) was added dropwise. The mixture was stirred at 0°C for AN additional 30 min. Then, water (5 ml) was added and the mixture was extracted with EtOAc (4 × 5 ml) and washed with 10% aqueous NaHCO3 (10 ml). The aqueous layer was extracted again with EtOAc (10 ml) and all organic layers were combined and washed successively with 10% NaHSO3 (2 × 5 ml), 10% NaHCO3 (5 ml), and brine (5 ml) and then dried over MgSO4. After filtration and evaporation of the solvent in vacuo, the crude product 31 was purified by column chromatography (cyclohexane/ethyl acetate; 25:75, v/v).
Racemic Mixture of (S)-3,3-dimethyl-4-(trifluoromethyl)azetidin-2-one (31)
Pale yellow oil (31 mg, 73%): 1H NMR (600 MHz, CDCl3) δ = 6.05 (br s, NH), 3.74 (q, J = 6.8 Hz, 1H, CHCF3), 1.44 (s, 3H, CCH3), 1.35 (s, 3H, CCH3). 13C NMR (151 MHz, CDCl3) δ = 172.87 (s, C = O), 124.44 (q, J = 278.9 Hz, CF3), 59.03 (q, J = 33.6 Hz, CHCF3), 56.05 (s, CCHCF3), 23.45 (s, CCH3), 16.79 (q, J = 2.4 Hz, CCH3). 19F NMR (565 MHz, CDCl3) δ = −72.20 (d, J = 6.5 Hz). HRMS (ESI) calcd for C6H8F3NO ([M+H+]): 168.0636, found: 168.0637.
Conclusion
In conclusion, we developed a convenient and highly diastereoselective synthesis of C-3 mono- and disubstituted 4-CF3 β-lactams. We showed that the enolate of 4-CF3 β-lactams can be formed under basic conditions and then undergoes reaction with various electrophiles. These wide ranges of 4-CF3 β-lactams variously functionalized are excellent synthons for constructing products of interest or for incorporating them into bioactive molecules.
Data Availability
All datasets generated for this study are included in the manuscript/Supplementary Files.
Author Contributions
TC and BC carried out manuscript writing. MS carried out chemical synthesis, characterization, and manuscript writing. TM and MK contributed to manuscript writing and revision. BC, TC, and HK designed and managed the study. All authors listed have made substantial, direct, and intellectual contributions to the work, and approved it for publication.
Funding
This work was supported by grant no. POWR.03.02.00-00-I023/17 co-financed by the European Union through the European Social Fund under the Operational Program Knowledge Education Development.
Conflict of Interest Statement
The reviewer AP-L declared a shared affiliation, with no collaboration, with one of the authors BC to the handling editor at time of review.
The remaining authors declare that the research was conducted in the absence of any commercial or financial relationships that could be construed as a potential conflict of interest.
Acknowledgments
MS acknowledges the French Government for Ph.D. fellowship, funded from the program of the French Government Scholarship (BGF), Doctorat en Cotutelle.
Supplementary Material
The Supplementary Material for this article can be found online at: https://www.frontiersin.org/articles/10.3389/fchem.2019.00526/full#supplementary-material
References
Abouabdellah, A., Bégué, J.-P., Bonnet-Delpon, D., and Thanh Nga, T. T. (1997). J. Org. Chem. 62, 8826–8833. doi: 10.1021/jo971381a
Alcaide, B., Almendros, P., and Aragoncillo, C. (2007). β-Lactams: versatile building blocks for the stereoselective synthesis of non-β-lactam products. Chem. Rev. 107, 4437–4492. doi: 10.1021/cr0307300
Battaglia, A., Bernacki, R. J., Bertucci, C., Bombardelli, E., Cimitan, S., Ferlini, C., et al. (2003). Synthesis and biological evaluation of 2′-methyl taxoids derived from baccatin III and 14β-OH-baccatin III 1,14-carbonate. J. Med. Chem. 46, 4822–4825. doi: 10.1021/jm034146v
Decamps, S., Sevaille, L., Ongeri, S., and Crousse, B. (2014). Access to novel functionalized trifluoromethyl β-lactams by ring expansion of aziridines. Org. Biomol. Chem. 12, 6345–6348. doi: 10.1039/C4OB01262C
Deketelaere, S., Van Nguyen, T., Stevens, C. V., and D'hooghe, M. (2017). Synthetic approaches toward monocyclic 3-amino-β-lactams. ChemistryOpen 6, 301–319. doi: 10.1002/open.201700051
Garbi, A., Allain, L., Chorki, F., Ourévitch, M., Crousse, B., Bonnet-Delpon, D., et al. (2001). Novel [1,2]- and [2,3]-wittig rearrangements of α-benzyloxy β-CF3-β-lactam enolates. Org. Lett. 3, 2529–2531. doi: 10.1021/ol016198p
Gong, Y., and Kato, K. (2001). Nucleophilic reactions with α-trifluoromethyl imine and N,O-disubstituted aminal: synthesis of β-trifluoromethyl β-anilino esters. J. Fluor. Chem. 111, 77–80. doi: 10.1016/S0022-1139(01)00437-7
Guanti, G., Banfi, L., Narisano, E., Scolastico, C., and Bosone, E. (1985). Synthesis and properties of 2-amino-3-oxo-3H-azepines. Synthesis 1985, 609–611. doi: 10.1055/s-1985-34142
Hosseyni, S., and Jarrahpour, A. (2018). Recent advances in β-lactam synthesis. Org. Biomol. Chem. 16, 6840–6852. doi: 10.1039/C8OB01833B
Ishihara, T., Ichihara, K., and Yamanaka, H. (1996). Stereoselective synthesis of 3-fluoro azetidinones via the condensation of 2-fluoropropanethioate lithium enolate with imines. Tetrahedron 52, 255–262. doi: 10.1016/0040-4020(95)00869-A
Jarrahpour, A., and Zarei, M. (2007). Synthesis of novel N-(4-Ethoxyphenyl) azetidin-2-ones and their oxidative N-deprotection by ceric ammonium nitrate. Molecules 12, 2364–2379. doi: 10.3390/12102364
Kamath, A., and Ojima, I. (2012). Advances in the chemistry of β-lactam and its medicinal applications. Tetrahedron 68, 10640–10664. doi: 10.1016/j.tet.2012.07.090
Kowalski, M. K., Mloston, G., Obijalska, E., Linden, A., and Heimgartner, H. (2016). First application of fluorinated nitrones for the synthesis of fluoroalkylated β-lactams via the Kinugasa reaction. Tetrahedron 72, 5305–5313. doi: 10.1016/j.tet.2016.06.073
Kuhlein, K., and Jensen, H. (1974). Substitutionsreaktionen an cephamderivaten. Justus Liebigs Ann. Chem. 1974, 369–402. doi: 10.1002/jlac.197419740305
Liu, Y., Chen, J.-L., Wang, G.-H., Sun, P., Huang, H., and Qing, F.-L. (2013). 4-CF3-ezetimibe analogs: design, synthesis, and biological evaluation of cholesterol absorption inhibitions. Tetrahedron Lett. 54, 5541–5543. doi: 10.1016/j.tetlet.2013.08.027
Ojima, I. (1995). Asymmetric syntheses by means of the β-lactam synthon method. Adv. Asym. Synth. 1:95.
Petrik, V., Röschenthaler, G.-V., and Cahard, D. (2011). Diastereoselective synthesis of trans-trifluoromethyl-β-lactams and α-alkyl-β-trifluoromethyl-β-amino esters. Tetrahedron 67, 3254–3259. doi: 10.1016/j.tet.2011.03.001
Pitts, R. C., and Letcka, T. (2014). Chemical synthesis of β-lactams: Asymmetric catalysis and other recent advances. Chem. Rev. 114, 7930–7953. doi: 10.1021/cr4005549
Thi, H. D., Danneels, B., Desmet, T., Van Hecke, K., Nguyen, T. V., and D'hooghe, M. (2016). Synthesis and applications of 3-methylene-4-(trifluoromethyl)azetidin-2-ones as building blocks for the preparation of mono- and spirocyclic 4-CF3-β-lactams. Asian J. Org. Chem. 5, 1480–1491. doi: 10.1002/ajoc.201600387
Trulli, L., Raglione, V., and Fioravanti, S. (2018). Selective synthesis of trifluoromethyl β-lactams by a Zn-promoted 2-bromo ester addition on C-CF3-substituted aldimines. Eur. J. Org. Chem. 2018, 3743–3749. doi: 10.1002/ejoc.201800168
Wang, J., Sánchez-Rosell,ó, M., Aceña, J. L., del Pozo, C., Sorochinsky, A. E., Fustero, S., et al. (2014). Fluorine in pharmaceutical industry: fluorine-containing drugs introduced to the market in the last decade (2001–2011). Chem. Rev. 114, 2432–2506. doi: 10.1021/cr4002879
Zarei, M., Jarrahpour, A., Ebrahimi, E., Aye, M., and Badrabady, S. A. T. (2012). andOn-column N-dearylation of 2-azetidinones by silica-supported ceric ammonium nitrate. Tetrahedron 68, 5505–55012. doi: 10.1016/j.tet.2012.04.088
Zhou, Y., Wang, J., Gu, Z., Wang, S., Zhu, W., Aceña, J. L., et al. (2016). Next generation of fluorine-containing pharmaceuticals, compounds currently in phase II–III clinical trials of major pharmaceutical companies: new structural trends and therapeutic areas. Chem. Rev. 116, 422–518. doi: 10.1021/acs.chemrev.5b00392
Keywords: lactams, fluorine, alkylation, deprotonation, functionalization
Citation: Skibińska M, Kaźmierczak M, Milcent T, Cytlak T, Koroniak H and Crousse B (2019) Direct Access to Substituted 4-CF3 β-Lactams at the C-3 Position. Front. Chem. 7:526. doi: 10.3389/fchem.2019.00526
Received: 17 May 2019; Accepted: 10 July 2019;
Published: 06 August 2019.
Edited by:
Hyun-Joon Ha, Hankuk University of Foreign Studies, South KoreaReviewed by:
Renzo Luisi, University of Bari Aldo Moro, ItalyAlejandro Perez-Luna, Sorbonne Universités, France
Copyright © 2019 Skibińska, Kaźmierczak, Milcent, Cytlak, Koroniak and Crousse. This is an open-access article distributed under the terms of the Creative Commons Attribution License (CC BY). The use, distribution or reproduction in other forums is permitted, provided the original author(s) and the copyright owner(s) are credited and that the original publication in this journal is cited, in accordance with accepted academic practice. No use, distribution or reproduction is permitted which does not comply with these terms.
*Correspondence: Tomasz Cytlak, Y3l0bGFrJiN4MDAwNDA7YW11LmVkdS5wbA==; Benoit Crousse, YmVub2l0LmNyb3Vzc2UmI3gwMDA0MDt1LXBzdWQuZnI=