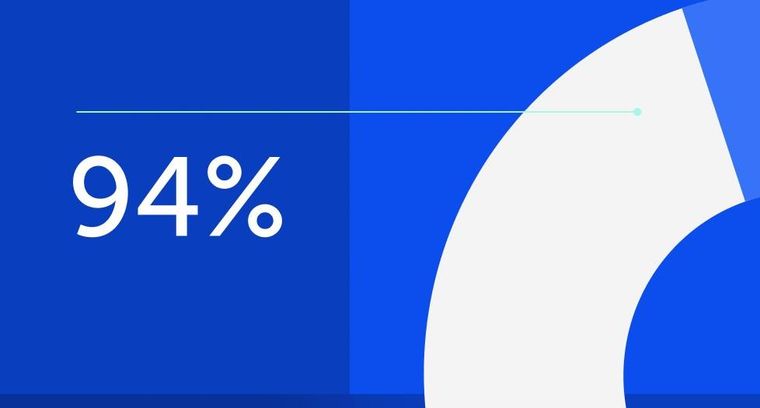
94% of researchers rate our articles as excellent or good
Learn more about the work of our research integrity team to safeguard the quality of each article we publish.
Find out more
MINI REVIEW article
Front. Chem. Biol., 31 January 2024
Sec. Bioinorganic Chemistry
Volume 3 - 2024 | https://doi.org/10.3389/fchbi.2024.1337372
This article is part of the Research TopicNew Developments in Bioinorganic and Bioorganic ChemistryView all 5 articles
Metal centres provide unique foci for varied biological modes of action that often but not exclusively involve redox or metal-ligand reactions. Metal complexes offer alternative and flexible coordination geometries, electron and proton transfer sites, inner and outer sphere reactivities, sites for redox-active, hemi-labile, and non-innocent ligands, and a variety of potentially controllable properties for exploitation in a therapeutic or biological context. The discovery of the first anticancer, the metal-based compound cisplatin in 1965 by Barnett Rosenberg was a historical outstanding breakthrough and led to a new area of metal-drug discovery. Some metal-based compounds have FDA approval for clinical use, while some undergo clinical trials for various medical therapies. This mini-review focuses on recent progress on Mn-based complexes with potential anticancer, antibacterial, and antifungal activities.
Since the discovery of cisplatin and its analogs as anticancer drugs, the coordination chemistry of metal-based drugs has become immensely popular among scientists due to its various applications not only in cancer but also in viral, microbial, or fungal treatment (Liu et al., 2013; Dasari Tchounwou, 2014; Zhao et al., 2016). However, currently, scientists are mostly focused on the synthesis of various candidates for anticancer therapy, especially inorganic compounds with such metal ions as Cu, Pd, Au, and Ru. Many of them are now under comprehensive investigation for potential clinical applications (Dyson and Sava, 2006; Jakupec, et al., 2008; Singh et al., 2023). Nevertheless, until now there have been only a limited number of articles on the antitumor, antibacterial, or antifungal activity of manganese compounds. We believe that Mn inorganic compounds can be an interesting alternative for many toxic drugs and/or those that become inactive.
Manganese (Mn), found in all tissues and fluids (Schmidt and Husted, 2019), is one of the biocompatible core elements of metal enzymes playing many physiological roles in vivo (Wang et al., 2022). This essential trace element plays a variety of biological roles in the human body such as regulation of (i) immunity functions, (ii) blood glucose levels, (iii) cellular energy, (iv) growth of bones, and promotes cholesterol synthesis and coagulation of blood. It also plays a significant role in the body as a reactive oxygen species (ROS) antioxidant (Emsley, 2001; Bae and Kim, 2008; Horning et al., 2015; Alejandro et al., 2020; Kongot et al., 2020). Those facts cause an interest increase in manganese-based nanomaterials (e.g., MnO) (Hao et al., 2017; Kalaiselvan et al., 2022; Zheng et al., 2022) and potential therapeutics in anticancer, antibacterial, and antifungal therapies (Kalaiselvan et al., 2022).
Among several biologically abundant metals, manganese complexes are well known to participate in a diversity of significant biochemical processes (Liu et al., 2013; Dasari Tchounwou, 2014; Zhao et al., 2016). Interestingly, Mn received particular attention in the field of biological applications due to its different oxidation states. The most common manganese valence states are Mn2+, Mn3+, and Mn4+. Among them, Mn2+ ions show the best stability compared with Mn3+ ions and Mn4+ ions (Lin, et al., 2018; Ding, et al., 2019; Kalaiselvan, et al., 2022). The Mn(II) complexes are considered to be very effective and efficient antioxidants against ROS leading to lessen oxidative stress in the human body (Allardyce and Dyson, 2016). An increasing number of mono- and binuclear Mn(III/II) complexes with catalase activity have been reported in the literature, most of them with Schiff base ligands. Following the first publication of the Mn(II) complexes possessing bipyridine (bipy) and phenanthroline (phen) ligands by Fukuda and Sone in 1970, a large number of such compounds with different metals using mainly the same ligands were synthesized and published (Fukuda and Sone, 1970; Bailey, et al., 1980; Paulovicova, et al., 2001; Madalan, et al., 2004). Because of Mn compounds’ potential use as catalytic scavengers of H2O2 against oxidative stress, several mono- and binuclear inorganic compounds with various ligands were obtained and characterized in an effort to mimic the active sites of enzymes (Triller, et al., 2002; Reddig, et al., 2004; Wu, et al., 2004; Signorella, et al., 2007; Biava, et al., 2009; Biju and Rajasekharan, 2011; Vazquez-Fernandez, et al., 2011; Signorella and Hureau, 2012).
Currently, the synthesis/production of Mn nanomaterials has gained limitless attention in the medical sector. Interest in Mn and MnO nanoparticles (NPs) is increasing due to their specific physicochemical properties (Haque, et al., 2021). However, in literature, we can still find Mn complexes possessing interesting biological properties leading to the conclusion that Mn can be used in medicine not only in the form of nanomaterials but also as an inorganic compound. In this mini-review, we have selected publications presenting fifty Mn inorganic compounds possessing anticancer, antimicrobial, and antifungal properties with a description of their first insight into action modes, which have appeared as the most relevant in the ScienceDirect database. Additionally, we described the action mode for selected Mn complexes. The purpose of this review is to show what types of ligands and oxidation states of Mn are usually selected by researchers to obtain therapeutic Mn compounds.
The anticancer properties of manganese inorganic compounds are underexplored. So far only several anticancer manganese (II/III) complexes containing Schiff base, porphyrin, flavonoids, and polypyridyl ligands have been characterized (Ansari et al., 2009a; Ansari et al., 2009b; Zhang, et al., 2009; Chen, et al., 2010). The cellular action mode of such complexes is extremely dependent on the nature of the ligands (Prudhomme, 2013). However, it has been proven in many publications that Mn coordination complexes increase intracellular ROS generation leading to oxidative stress in various cell types (e.g., cancer, bacterial or fungal) (Ansari et al., 2009a; Ansari et al., 2009b; Slator, et al., 2017; Kellet, et al., 2011; Liu, et al., 2015; Farghadani, et al., 2017). Moreover, it was reported that the Mn(II) compounds exhibit similar or higher cytotoxic effects, for instance on the human lung adenocarcinoma (A549), breast cancer (MCF-7) cell lines or breast cancer cell stems 3D cultures, in comparison to the Cu(II) and Fe(III) ones with phenanthroline and pyridine derivatives with simultaneous nontoxicity towards normal cell lines, e.g., epithelial isolated from the mammary gland (MCF-10A) (Eskandari and Suntharalingam, 2019; Icsel, et al., 2020). Therefore, by using Mn compounds over other complexes with more common endogenous metal ions such as Cu and Fe, it will be possible to take advantage of the many physiologically accessible oxidation states of Mn and its ability to undergo Fenton-type reactions to potentially kill cancer cells via efficient ROS production with better selectivity. (Eskandari and Suntharalingam, 2019; Icsel, et al., 2020).
Indeed, a series of di-nuclear manganese (II)-phenanthroline complexes exhibited a significant increase in intracellular ROS levels leading to high cytotoxicity in human-derived colorectal cancer lines (Kellet, et al., 2011). Similarly to a novel, Mn(II) complex (Figure 1; 1) (Ghosh, et al., 2013) with N-(2-hydroxy acetophenone)glycinate synthesized by R.D. Ghosh and coworkers. This compound can induce apoptosis in multidrug-resistant CEM/ADR5000 leukemia cells, most probably through reactive oxygen species production. The authors proved that complex 1 exhibits significant efficacy in overcoming drug-resistant cancer. Moreover, the application of 1 at non-toxic doses caused a significant increase in the lifespan of Swiss albino mice bearing sensitive and doxorubicin-resistant subline of Ehrlich ascites carcinoma cells (Ghosh, et al., 2013). Two Mn complexes ([(Etdpa)MnCl2] (Figure 1; 2) and [(Adpa)Mn(Cl) (H2O)] (Figure 1; 3)) with different ligands: ethyl bis(2-pyridylmethyl)amino-2-propionate (Etdpa) and bis(2-pyridylmethyl)amino-2-propionic acid (Adpa) showed significant activity against the four cancer cells (MCF-7, ECA-109 (esophageal squamous cell carcinoma), U251 (glioblastoma), HeLa (cervical carcinoma)) (Qiu-Yun, et al., 2010). Complex 3 turned out to be more active and inhibited the proliferation of glioma cells (with IC50 9.5 μM) MCF-7 cells (with IC50 6.5 μM) and U251 cells (with IC50 9.5 μM) with a much lower concentration than complex 2. Such high activity was linked by Qiu-Yun and coauthors with the significant decrease of mitochondrial membrane potential (MMP) by studied complexes, especially 3. The binding mode between this manganese(II) complexes (2) and (3) and ct-DNA was weak, however, the authors demonstrated that such compounds inhibited the induced swelling of Ca2+-loaded mitochondria and caused the decrease of mitochondria membrane potential (Δψm). Importantly, it was shown that MMP values determined for tumor cells were higher than those calculated for normal cells. Therefore, with higher permeability of the mitochondria membrane in cancer cells, the Mn compounds 2 and 3 could more efficiently accumulate and interact with the specified organelle. This phenomenon can be one of the main factors in determining the selectivity of Mn complexes (Qiu-Yun, et al., 2010).
K.R. Enikeeva and coworkers have synthesized manganese(II) complexes (Enikeeva, et al., 2023) (Figure 1; 4, 5, 6) with Mn2Cl2 and more rare Mn2O2 cores based on the diisopropyl (pyridin-2-yl)phosphine oxide and diethyl (pyridin-2-yl)phosphine oxide ligands. The cytotoxic effect of these Mn complexes on the HeLa (cervical carcinoma) and HuTu80 (intestine) cancer cell lines and Chang liver normal cell lines has been studied. It was found that Mn(II) complex 5 with the Mn2O2 core was the effective hydrogen catalyst and exhibited high cytotoxicity towards the cancer HuTu80 cell line (selectivity index = 7.6) and very low toxicity against healthy Chang liver cells, making it very promising for further biomedical studies (Enikeeva, et al., 2023). However, the Authors did not study the precise mode of action of those complexes, so it is hard to speculate if ROS had any impact on cancer cell death. Another mononuclear complex [Mn(NO3) (sac) (H2O) (bzimpy)]·2DMF (Figure 1; 7) (Icsel, et al., 2020), (sac = saccharinate and bzimpy = 2,6-bis(2-benzimidazolyl)pyridine) was synthesized by C. Icsel and coworkers. The authors assessed the anticancer effect of compound 7 against a few cancer cell lines: A549 (lung), MCF-7 (breast), HT29 (colon), and one healthy one: MCF10A (normal human breast epithelial). The obtained results were compared with those of the well-known anticancer drug cisplatin and the starting ligand bzimpy. Mentioned Mn inorganic compounds exhibited potential cytotoxic activity, especially towards MCF-7 and A549 cell lines, and luckily it was inactive towards the normal cells. Mechanistic studies on A549 cells performed for complex 7 indicated that it induced G0/G1 arrest leading to significant intracellular ROS levels increased. This phenomenon caused both mitochondrial dysfunction and double-strand DNA breaks. The up-regulated Bax and down-regulated Bcl-2 expression levels, caspase-3/7 activation, and reduced Fas expression indicated that complex 7 induced ROS-dependent mitochondria-mediated intrinsic apoptosis in A549 cells (Icsel, et al., 2020). Nevertheless, the authors cannot omit the strong impact of compound 7 on DNA damage, which can play an important role in the final action mode.
Next Mn compound possessing potential anticancer activity was Mn2 (μ-O,O′-4-Mebz)2 (bpy)2 (μ2-H2O) (4-Mebz)2] (Figure 1; 8) (Bhattacharyya, et al., 2019) (where bpy=2,2′-bipyridine, 4-Mebz=4-methyl benzoate) obtained and characterized by M.K. Bhattacharyya and coworkers. Interestingly, the action mode of this complex was not linked to ROS production. The antiproliferative activity of complex (8) has been studied in T-cell lymphoma cell line by (i) MTT assay, (ii) apoptosis assay, and (iii) molecular docking simulation. Complex 8 exhibited satisfying cytotoxicity leading to apoptotic cell death with unimportant activity (∼5–10%) in normal cells. It is worth mentioning that Mn(II) complex interacted with overexpressed cancer target proteins with a higher binding affinity comparable with the affinity of reference inhibitors (Bhattacharyya, et al., 2019). One of the biological characteristics of cancer cells is the expression or overexpression of their specific groups of proteins or receptors (Fath, et al., 2022). Each of them plays a key role at specific stages of cancer growth and progression. Overexpressed proteins are involved, for example, in DNA repair, signaling and metabolic pathways, apoptosis, and more cellular processes. This leads to cancer initiation, progression, angiogenesis and could promote the invasion of tumor cells into ambient tissues (Pessoa, et al., 2022). Therefore, targeting the interactions between tumor proteins and new chemotherapeutic agents has become an attractive approach in cancer treatment (Welsh et al., 2003; Fath, et al., 2022; Pessoa, et al., 2022).
The first example of Mn complex that reached clinical trials was synthesized by I. Batinić-Haberle and coworkers (Batinić-Haberle, et al., 2002; Florido, et al., 2019). Manganese (III) porphyrin (Figure 2; 9) is mimicking behavior of superoxide dismutase (SOD) and it has a significant impact on anticancer effects in combination treatment with chemo- and radiotherapy. Despite the ongoing clinical trials, unfortunately, scientists still do not know the effect of 9 on metastasis, which is crucial for further cancer treatments. The co-treatment of cancerous cells with non-toxic concentrations of complex 9 and anticancer drug doxorubicin altered intracellular ROS, and significantly increased the concentration of H2O2.
While 9 alone did not influence cell migration, the mixture of doxorubicin and compound 9 led to a reduction in collective cell migration and chemotaxis. In addition, compound 9 reduced the doxorubicin-induced increase in random migration of human breast cancer cells (MDAMB-231). Treatment with either compound 9 or doxorubicin led to the decrease of MDAMB231 cells. It is worth mentioning that the authors obtained various results, some of them dependent on cell line and migration type, however, the cytotoxic effect induced by 9 in doxorubicin-treated cells was always favorable (Florido, et al., 2019). Interesting results have been obtained by A.T. Gordon and coworkers. They synthesized Mn complex [Mn (theo)2(H2O)4] (Figure 2; 10) (Gordon, et al., 2022), which possessed limited activity compared to compound 9. The anticancer activity of this Mn complex was evaluated against several cell lines, representing breast-, lung-, colorectal-, and pancreatic cancer, as well as glioblastoma and neuroblastoma. However, the authors did not notice significant anticancer activity of this complex 10. Nevertheless, preliminary analysis of the action mode of the Mn complex and cell morphology studies indicates the absence of necrotic cell death. Interestingly, this type of compound caused paraptosis of the cell leading to the formation of cytoplasmic vacuoles. Due to those processes and decreased binding of Hoechst 33342 to the nuclei, the authors speculate that complex 10 may affect cellular DNA function of the human cancer cells (neuroblastoma, SY-SH5Y; breast adenocarcinoma, MDA-MB-231; pancreatic, MIA PaCa-2) (Gordon, et al., 2022). Paraptosis is reported to be induced via several mechanisms, e.g.,: (i) the expression of insulin growth factor 1 receptor (IGF1R), (ii) proteasome inhibition and ER stress, (iii) reactive oxygen species (ROS) production, (iv) Ca2+ influx into mitochondria or (v) opening of the ion channel, etc. (Hanson, et al., 2023). The authors concluded, taking into account the above facts, that Mn compound (10) is taking part in direct or indirect ROS production. A series of binuclear manganese–oxo-complexes (Figure 2; 11-15) (Kurz, 2009) was prepared by P. Kurz and coworkers. The authors proved that all manganese compounds were able to generate molecular oxygen in an aqueous solution (Welch et al., 2009).
Summing up, presented here Mn complexes exhibited brilliant or moderate anticancer properties towards different cancer cells. Mostly their mode of action was checked in the direction of the overproduction of ROS and/or destruction of the DNA helix, which is one of the known pathways leading to the induction of apoptosis by metal complexes. However, there are also many other mechanisms of action of transition metal compounds on cancer cells described in the literature, such as DNA binding and damage, mitochondrial dysfunction, endoplasmic reticulum stress, enzyme and protein inhibition, and cell cycle arrest (Ndagi et al., 2017, Abdolmaleki et al., 2024) Additionally, it is also puzzling why the scientist did not investigate other cell death pathways. As is well known, ROS molecules regulate not only pyroptosis, apoptosis, and necrosis, but can also cause ferroptosis (Yu et al., 2021). Ferroptosis is a new type of cell death that is considered as a more desirable and immunogenic process than apoptosis. This mechanism is iron-dependent, and oxidative cell death is caused by reactive oxygen species in the Fenton reaction and subsequent lipid peroxidation (Kuang et al., 2020; Tang et al., 2020). Moreover, the combination of several mechanisms sometimes allows to achieve the anticancer effect of transition metal complexes. That’s why more investigation should be done by scientists to grasp the molecular mechanisms and biological targets of newly synthesized complexes with more promising anticancer potential.
Antimicrobial resistance (AMR) is becoming one of the leading reasons of death in the world. In 2019, there were an estimated 5 million deaths associated with AMR, of which 1.3 million were directly attributable to resistant infections. This number is expected to reach 10 million deaths per year worldwide by 2050, or even sooner due to the widespread antibiotics’ over-prescription to patients with COVID-19 over the last 3 years. Unfortunately, conventional therapy based on organic chemistry has failed and in 2022, there were only forty-five traditional antibiotics in clinical development (Frei, et al., 2023). That is why new approaches leading to the next generations of antibiotics discovery are urgently needed. However, increasing bacterial resistance to well-known antibiotics (O’Connell, et al., 2013) and extremely limited treatment possibilities for tropical diseases (Renslo and Mckerrow, 2006; Nagle, et al., 2014) led to the exploration of metal complexes as a future antibacterial drug (Sanchez-Delgado, et al., 2004). The inorganic compounds studied for potential antibacterial activity are very often based on relatively weak metal–ligand interactions and what is more their reactivity in biological media is not enough characterized. Nevertheless, there are some exceptions, especially organometallic compounds, among which has been found ferroquine, an organometallic antimalarial drug candidate in advanced clinical trials (Biot and Dive, 2010; Biot, et al., 2011; Biot, et al., 2012; Navarro, et al., 2012; Patra, et al., 2012; Patra, et al., 2013; Lewandowski, et al., 2015). Particularly, numerous organometallic gold compounds (Glisic and Djuran, 2014) as well as ruthenium (II) ones (Gambino and Otero, 2012) received important attention due to facile structural variation important for structure–activity relationship studies (Demoro, et al., 2012; Arancibia, et al., 2014; Cipriani, et al., 2014). There are still other metal–ligand architectures that have been not studied enough, especially those with Mn ions. That is why, in this paper, we wanted to know more about Mn compound possessing antibacterial activity.
A. A. Osowole with coworkers use the ketoamine, 2-(1,3-benzothiazol-2-ylamino)naphthalene-1,4-dio-ne (HL) to synthesize Mn(II) complex (Osowole, et al., 2016) (Figure 3; 16) which was evaluated in vitro against K. oxytoca, P. aeruginosa, E. coli, B. cereus, and S. aureus. The research revealed that the Mn compound (16) showed broad-spectrum moderate antibacterial activities against the selected bacteria strains (with MIC 8.0–25.0 mM). Interestingly, molecular docking studies showed good molecular interactions between compound 16 and all the bacterial receptors which were also consistent with the observed antibacterial effects, e.g., the manganese complex with the lowest activity exhibited the poorest binding affinities across the investigated enzymes (Osowole, et al., 2016). Another group of compounds has been obtained and characterized by E.A. Ermakova and coworkers. They synthesized mixed-ligand manganese (II) complexes (Ermakova, et al., 2021), [Mn (dmphen)2L2] (Figure 3; 19 17), [Mn2 (dmbipy)2L2(OAc)2] (Figure 3; 18) and [Mn3 (bipy)2L4(OAc)2] (Figure 3; 17 19), where L—5-phenyltetrazolate anion, bipy—2,2′ -bipyridine, dmbipy—2,2′ -bi-4-picoline, dmphen—4,7-dimethyl-1,10-phenanthroline. The antimicrobial effects of complexes 18 and 19, as well as starting ligands, were investigated towards E. coli, S. aureus, P. italicum and C. steinii. The antimicrobial activity study revealed that the manganese inorganic compounds show noticeable protistocidal properties, but no bacteriostatic activity (except [Mn3 (bipy)2L4(OAc)2] (17)) and fungistatic activity. Protistocidal properties of [Mn (dmphen)2L2] (19) have been comparable to medicinal chloroquine. A study of antioxidant properties of examined complexes 17, 18, and 19 showed that all compounds possess radical scavenging activity, comparable to antioxidant properties of ascorbic acid, which was measured using ABTS•+ radical (Ermakova, et al., 2021).
The novel 2D manganese polymeric complex (Figure 3; 20) (Ashouri, et al., 2020) with diclofenac, a non-steroidal anti-inflammatory drug, was effectively synthesized by F. Ashouri and coworkers. The in vitro antibacterial activity of the manganese complex 20 was screened towards four bacterial strains including S. aureus, B. subtilis, E. coli, and P. aeruginosa. The study revealed that the obtained polymeric complex acts as antibacterial active inorganic polymers towards both Gram-positive and Gram-negative bacteria. Manganese polymer exhibited good inhibitory activity compared to substrates (Ashouri, et al., 2020).
Two new manganese (II) complexes (Ahmed, et al., 2023) were synthesized in the reaction of manganese (II) nitrate tetrahydrate with bisaroylhydrazone derivative in acetonitrile at room temperature by R.K. Ahmed and coworkers: [MnII(L1)2(NO3)2] (Figure 3; 21) and [MnII(L2)2(NO3)2] (Figure 3; 22). Interestingly, their structure–activity relationship and binding energies for the most suitable conformation, as well as the bond between Mn(II) complexes and enzymes, have been calculated by time-dependent DFT (TD-DFT). Additionally, in vitro enzyme inhibition and antimicrobial activity were investigated. The authors found that inorganic compound 21 showed a competitive inhibition for α-glucosidase enzyme, while 22 inhibited in a non-competitive way. The antimicrobial activity study of ligands, 21, and 22 towards a few selected pathogenic bacteria (B. cereus, S. aureus, E. coli, and P. aeruginosa) exhibited good activity. The brilliant in vitro inhibition potency of studied manganese compounds suggests their potential use in treating urease and α-glucosidase-associated problems (Ahmed, et al., 2023). This investigation suggests that these newly discovered molecules may be promising drug candidates for further structural optimization and even better antibacterial properties.
Novel Schiff base octaazamacrocyclic complexes of Mn(II) (Figure 3; 23-25) (Mamta et al., 2023) were obtained by A. Chaudhary and coworkers using macrocyclic ligands derived from Schiffbase ligand and dicarboxylic acids. The antimicrobial activity of the Mn-synthesized compounds 23, 24, and 25 were evaluated towards bacterial (E. coli, B. subtilis) and fungal strains (C. albicans, F. oxysporum). The results showed that the macrocyclic Mn(II) complexes have more antimicrobial potential when compared with parent macrocyclic ligands. Interestingly, complex 23 has been described as an excellent antimicrobial agent for studied microorganisms. Based on these phenomena, manganese may be an interesting subject for more research in the investigation of possible metal-based compounds with antimicrobial properties (Mamta et al., 2023). Manganese complexes with macrocyclic appear to possess interesting antibacterial properties, which is why a series of binuclear manganese (III) complexes (Figure 3; 26 31) (Archana and Sreedaran, 2023) were synthesized by B. Archana and coworkers from the macrocyclic ligands 1,8-[bis(3-formyl- 2-hydroxy-5-methyl)benzyl]-1,4,8,11-tetraazacyclotetradecane and 1,8-[bis(3-formyl-2-hydroxy-5-bromo) benzyl]-1,4,8,11-tetraazacyclotetradecane by a Schiff base condensation with the appropriate aliphatic or aromatic diamines, MnCl2⋅4H2O and triethylamine. Antimicrobial effects studied by the cup plate method showed much better results obtained for aromatic manganese complexes than aliphatic ones. The authors concluded that even small variations in the ligand framework, such as changing the length of the carbon chain and the nature of the para substituent of the phenoxide to the phenyl ring, can significantly affect the properties and applications of the manganese complexes. Additionally, some DNA binding activity experiments have been performed which suggest that the manganese complexes can bind to DNA via the intercalation processes. The enhanced DNA binding affinity of complexes 28 and 30 is due to the presence of the electron-donating -CH3 group, leading to a hydrophobic interaction with the hydrophobic DNA surface. On the other hand, the weakest DNA binding affinity has been attributed to complexes 26 and 29 possessing electron-withdrawing Br atoms. The DNA cleavage experiments were in good agreement with the binding studies (begins at a low concentration of 25 μM and reaches a maximum cleavage in concentration of 100 μM) (Archana and Sreedaran, 2023).
The interaction of Mn2+ with α-diimine such as 1,10-phenanthroline (Figure 4; 32), 2,2′ -bipyridine (Figure 4; 33) or 2,2′ -bipyridylamine (Figure 4; 34), lead to the formation of five manganese (II) complexes of the formula [Mn (X-salo)2 (α-diimine)] (Figure 4; 32-34) (Ntanatsidis, et al., 2022). The antibacterial activity of such type of manganese complexes was tested in vitro towards S. aureus and X. campestris bacterial strains and was found moderate. The best antimicrobial effects among all described Mn inorganic compounds were found for complex 32 (MIC = 50 μg/mL, 93 μM). Additionally, the ability of the 32, 33, and 34 to scavenge DPPH, hydroxyl, and ABTS radicals was checked in the context of their antioxidant properties. Unfortunately, the DPPH-scavenging ability of the Mn complexes was low. On the other hand, the manganese complexes exhibited quite interesting and strong affinity to hydroxyl and ABTS radicals which led authors to the conclusion that such Mn compounds are potent scavengers especially compounds 33 and 34—the most active towards hydroxyl radicals. Formulated conclusion by the authors clearly shows that such manganese (II) complexes exhibiting the ability of radical scavenging may be attributed to the biological occurrence of manganese in enzymes such as Mn–SOD and catalase whose biological role is to scavenge reactive oxygen species in nature (Ntanatsidis, et al., 2022).
An interesting idea had A. Barmpa and coworkers who synthesized and characterized the manganese (II) complexes (Figure 4; 35 and 36) (Barmpa, et al., 2018) with the quinolone antimicrobial agent flumequine (Hflmq) in the absence or presence of the N,N-donor heterocyclic ligands 1,10-phenanthroline (phen, 35) and 2,2-bipyridylamine (bipyam, 36). The antimicrobial activity of the Mn inorganic compounds showed that the Mn(II)–flumequine complexes 35 and 36 revealed similar or slightly better activity towards the four bacterial strains compared to the free flumequine drug. The interaction of the complexes with two serum albumins human (HAS) and bovine (BSA) demonstrates that complexes 35 and 36 can bind to both albumins with relatively high binding constants. Furthermore, the authors proved that 35 and 36 can bind tightly to ctDNA in an intercalative mode allowing them to compete with strong intercalator EB (ethidium bromide) (Barmpa, et al., 2018). A novel manganese (II) complex (Figure 4; 37) (Firuzabadi and Asadi, 2021) of the quinolone antibacterial agent gemifloxacin (gem) was synthesized and characterized by F.D. Firuzabadi and coworkers. The antimicrobial properties of the Mn inorganic compound were tested on four different microorganisms (E. coli, S. aureose, P. aeruginosa, E. faecalis) and the results revealed that Mn–gem complex exhibited much higher biological efficiency than the parent quinolone antibiotic. The theoretical studies of the interaction of 37 with DNA led to the conclusion that this Mn complex exhibited much higher binding affinity to DNA, presumably by partial intercalation and/or groove binding, than the parent antibiotic Gemifloxacin (Firuzabadi and Asadi, 2021). J.-l. Tian and coworkers used a different quinolone antibiotic: enrofloxacin (EFX), a third-generation fluoroquinolone drug, to synthesize the Mn complex (Figure 4; 38) (Tian, et al., 2023). In vitro antibacterial activity test showed that complex 38 exhibits strong growth inhibition of many pathogenic bacterial strains in turn to parent antibiotic enrofloxacin. Interestingly, for two Gram-negative bacterial strains, E. coli and S. typhi, 38 showed higher bactericidal effects than the antibacterial drug itself (Tian, et al., 2023).
M.L. Lawrence with coworkers synthesized and characterized a new large group of mixed Fe-Mn compounds CORMs (Figure 4; 39-43) (Lawrence, et al., 2020). The authors proved that all mixed Mn-Fe complexes possess efficient inhibitory properties for the growth of both Gram-positive and Gram-negative bacterial strains. In addition, these mixed manganese-iron inorganic compounds show exceptionally good activity towards Gram-negative bacteria, which was the biggest finding especially since therapeutics for these types of bacteria are difficult to find. Presented results also demonstrate that current-generation CORMs display moderate antibiotic properties against both prokaryotic and eukaryotic cells (Lawrence, et al., 2020).
In the literature, we can find much more information about manganese inorganic compounds possessing antibacterial activity than about anticancer Mn compounds. Mostly, it was discovered that the best antibacterial activity was for Mn complexes with macrocyclic ligands. The most hypothesized mode of action of antimicrobial activity relates to DNA interactions. Most of the compounds exhibited antioxidant properties. However, the study on antibacterial or even bactericidal mechanism of action for Mn complexes should be more investigated. Besides the examination of MIC and MBC (minimal bactericidal concentration) values, in the literature, there are not a lot of experiments on bacteria itself, just isolated biomolecules to determine the type of interactions. Furthermore, scientists that specialize in the examination of the antibacterial activity of metal-based complexes should find more precise answers, if studied compounds are effective in overcoming the processes due to bacteria resistance, like biofilm production, bypass, efflux pump expression, or inactivation of the enzymes (Schillaci et al., 2017; Bailey et al., 2019).
The worldwide number of invasive candidiasis has significantly increased in recent decades, being the fourth and sixth leading cause of various blood infections in the United States of America and Europe, respectively (Richardson and Lass-Flori, 2008; Caggiano, et al., 2015; Sanguinetti, et al., 2015). On the other hand, infections caused by non-albicans Candida species, are becoming increasingly more common in hospitals. Unfortunately, it was found that such non-albicans Candida species are more resistant to the various classes of antifungal drugs becoming serious a clinical challenge (Abu-Elteen and Hamad, 2012; Ramos, et al., 2015). This trend is also being observed for fungal infections caused by other uncommon species (Candida haemulonii, Candida duobushaemulonii and C. haemulonii) (Almeida, et al., 2012; Cendejas-Bueno, et al., 2012; Muro, et al., 2012).
Manganese (II) complexes (Figure 5; 45) (Ali and iqbal, 2017; Kongot, et al., 2020) derived from methimazole, azomethinobenzene sulphonamide-thiadiazole Schiff base, aminopyrimidine-isatin Schiff base have exhibited exceptional antifungal activity. The synthesized Mn compound 45 induced a significant inhibition (93%) of a widespread fungal pathogen, Cryptococcus neoformans. The manganese complex 45 also was found to induce the uptake of glucose (83%) by insulin resistance cells (Kongot, et al., 2020).
Five mononuclear Mn(II) complexes (Kani, et al., 2016), Mn (phen)2 (ba) (H2O)](ClO4) (CH3OH) (Figure 5; 46), [Mn (phen)3](ClO4)2(H2CO3)2 (Fig. ;47), [Mn (phen)2(ClO4)2] (Figure 5; 48), [Mn (bipy)3](ClO4)2) (Figure 5; 49), [Mn (bipy)2(ClO4)2] (Figure 5; 50) and, where bipy = 2,2′-bipyridine, phen = 1,10-phenanthroline, and ba = benzoic acid were prepared by I. Kani and coworkers. The authors proved that the presence and number of phen ligands improved the antifungal activity of Mn compounds more than those complexes with the bipy ligand. Inorganic compounds 48 and 46, which contain more basic phen ligands, disproportionate H2O2 much faster than complexes 49 and 50, with a lower number of bipy ligands. The in vitro antifungal (anticandidal) activities of the Mn(II) complexes were remarkably higher than the reference drug ketoconazole (Kani, et al., 2016).
There are still not many Mn inorganic compounds in the literature possessing antifungal properties. Even though we were able to find a few examples, authors still do not study the mode of antifungal action. Clearly, Mn compounds in a fight with fungus is a very new idea, worth further exploration.
Manganese inorganic compounds are still not enough discovered, analyzed, or described by scientists. In the literature, we can find a limited number of examples of Mn compounds possessing biological activities. In the case of Mn complexes with anticancer activity, described here, these compounds were found to exhibit brilliant or moderate anticancer properties. Mostly their mode of action was based on ROS production leading to apoptotic cancer cell death even though some of them were able to cause paraptotic cell death. However, for some Mn compounds strong interaction with DNA can still be observed leading even to this acid cleavage. Dominant numbers of structures of such complexes are dedicated to antibacterial therapies. The most hypothesized mode of action of antimicrobial activity relates to DNA interactions, however, there is not much information about the precise action mode. In the case of Mn compound with antifungal activities, there are only a few examples without precise explanation of their activity mode of action.
Our review showed that the most common ligands used to obtain Mn compounds are -N (especially polyamines and phenanthroline derivatives), -O, and sometimes -S donor ligands. Many described here structures of the Mn compounds possess phenanthroline derivatives because they stabilize the Mn(II) oxidation state. However, since it is well-known that phenanthroline or phenanthroline derivatives are genotoxic and can be responsible for the high toxicity of resulting compounds, there is a need to prepare new Mn compounds with different types of N-, S-, or even P- donor ligands. It was demonstrated that Mn2+ is the most common manganese valence state, which shows the best stability compared with Mn3+ ions and Mn4+ ions. In literature, we can find complexes exhibiting biological properties mostly with Mn(II) or Mn(III) ions. Researchers should also focus on understanding the action mode of Mn complexes–it will help, in the future, to design structures with high activity against cancer, bacteria, or fungus and low toxicity towards normal cell lines.
Summing up, the Mn inorganic compounds are still not enough explored group in terms of their biological use. However, the examples we found in the literature suggest that manganese compounds can be an extremely attractive alternative to currently well-known metal-based drugs.
SK: Conceptualization, Funding acquisition, Supervision, Visualization, Writing–original draft, Writing–review and editing. DW: Writing–original draft. MS: Writing–original draft. JS: Writing–original draft. UK: Conceptualization, Funding acquisition, Supervision, Visualization, Writing–original draft, Writing–review and editing.
The author(s) declare financial support was received for the research, authorship, and/or publication of this article. The authors gratefully acknowledge financial support from the Polish National Science Centre (Grant 2020/37/N/ST4/02698) and the program “Excellence initiative–research university” for years 2020–2026, University of Wrocław (No. BPIDUB.17.2023). We also extend a word of thanks to Rimon Mikhail for language editing of our manuscript.
The authors declare that the research was conducted in the absence of any commercial or financial relationships that could be construed as a potential conflict of interest.
All claims expressed in this article are solely those of the authors and do not necessarily represent those of their affiliated organizations, or those of the publisher, the editors and the reviewers. Any product that may be evaluated in this article, or claim that may be made by its manufacturer, is not guaranteed or endorsed by the publisher.
Abdolmaleki, S., Aliabadi, A., Aliabadi, A., and Khaksar, S. (2024). Riding the metal wave: a review of the latest developments in metal-based anticancer agents. Coordination Chemistry Reviews 501, 215579. doi:10.1016/j.ccr.2023.215579
Abu-Elteen, K. H., and Hamad, M. A. (2012). Changing epidemiology of classical and emerging human fungal infections: a review. Jordan Biol. Sci. 5, 215–230.
Ahmed, R. K., OmarAli, A.-A. B., Al-Karawi, A. J. M., Marah, S., Jaafar, M. I., Dege, N., et al. (2023). Designing of eight-coordinate manganese(II) complexes as bio-active materials: synthesis, X-ray crystal structures, spectroscopic, DFT, and molecular docking studies. Polyhedron 244, 116606. doi:10.1016/j.poly.2023.116606
Alejandro, S., Höller, S., Meier, B., and Peiter, E. (2020). Manganese in plants: from acquisition to subcellular allocation. Front. Plant Sci. 11, 300. doi:10.3389/fpls.2020.00300
Ali, B., and Iqbal, M. A. (2017). Coordination complexes of manganese and their biomedical applications. ChemistrySelect 2, 1586–1604. doi:10.1002/slct.201601909
Allardyce, C. S., and Dyson, P. J. (2016). Metal-based drugs that break the rules. Dalton Trans. 45, 3201–3209. doi:10.1039/C5DT03919C
Ansari, K. I., Grant, J. D., Kasiri, S., Woldemariam, G., Shrestha, B., and Mandal, S. S. (2009a). Manganese(III)-salens induce tumor selective apoptosis in human cells. J. Inorg. Biochem. 103, 818–826. doi:10.1016/j.jinorgbio.2009.02.004
Ansari, K. I., Kasiri, S., Grant, J. D., and Mandal, S. S. (2009b). Apoptosis and anti-tumour activities of manganese(III)-salen and -salphen complexes. Dalton Trans. 40, 8525–8531. doi:10.1039/B905276C
Arancibia, R., Klahn, A. H., Lapier, M., Maya, J. D., Ibanez, A., Garland, M. T., et al. (2014). Synthesis, characterization and in vitro anti-Trypanosoma cruzi and anti-Mycobacterium tuberculosis evaluations of cyrhetrenyl and ferrocenyl thiosemicarbazones. J. Organomet. Chem. 755, 1–6. doi:10.1016/j.jorganchem.2013.12.049
Archana, B., and Sreedaran, S. (2023). Synthesis, characterization, DNA binding and cleavage studies, in-vitro antimicrobial, cytotoxicity assay of new manganese(III) complexes of N-functionalized macrocyclic cyclam based Schiff base ligands. Polyhedron 231, 116269. doi:10.1016/j.poly.2022.116269
Ashouri, F., Faraji, A. R., Molaeian, S., Fall, M. A., and Butcher, R. J. (2020). The novel cobalt and manganese polymeric complex with the non-steroidal anti-inflammatory drug diclofenac: synthesis, characterization and antibacterial studies. J. Mol. Struct. 1204, 127483. doi:10.1016/j.molstruc.2019.127483
Bae, Y. J., and Kim, M.-H. (2008). Manganese supplementation improves mineral density of the spine and femur and serum osteocalcin in rats. Biol. Trace Elem. Res. 124 (1), 28–34. doi:10.1007/s12011-008-8119-6
Bailey, J., Cass, J., Gasper, J., Ngo, N. -D., Wiggins, P., and Manoil, C. (2019). Essential gene deletions producing gigantic bacteria. PLoS Genet. 15, 1008195. doi:10.1371/journal.pgen.1008195
Bailey, N. A., Fenton, D. E., Franklin, M. V., and Hall, M. (1980). „Ternary complexes of copper(II) with mixed acetylacetonate and nitrogen-containing ligands. J. Chem. Soc. Dalton Trans. 6, 984. doi:10.1039/DT9800000984
Barmpa, A., Frousiou, O., Kalogiannis, S., Perdih, F., Turel, I., and Psomas, G. (2018). Manganese(II) complexes of the quinolone family member flumequine: structure, antimicrobial activity and affinity for albumins and calf-thymus DNA. Polyhedron 145, 166–175. doi:10.1016/j.poly.2018.02.006
Batinić-Haberle, I., Spasojević, I., Stevens, R. D., Hambright, P., and Fridovich, I. (2002). Manganese(iii) meso-tetrakis(ortho-N-alkylpyridyl)porphyrins. Synthesis, char-acterization, and catalysis of O2˙- dismutation. J. Chem. Soc. Dalton Trans. 2689, 2689. doi:10.1039/b201057g
Bhattacharyya, M. K., Gogoi, A., Chetry, S., Dutta, D., Verma, A. K., Sarma, B., et al. (2019). Antiproliferative evaluation and supramolecular association in Mn(II) and Zn(II) bipyridine complexes: combined experimental and theoretical studies. J. Inorg. Biochem. 200, 110803. doi:10.1016/j.jinorgbio.2019.110803
Biava, H., Palopoli, C., Duhayon, C., Tuchagues, J.-P., and Signorella, S. (2009). Synthesis, structure, and catalase-like activity of dimanganese(III) complexes of 1,5-Bis[(2-hydroxy-5-X-benzyl)(2-pyridylmethyl)amino]pentan-3-ol (X = H, Br, OCH3). Inorg. Chem. 48, 3205–3214. doi:10.1021/ic8019793
Biju, A., and Rajasekharan, M. (2011). Structure, magnetic properties, catalase activity and DFT studies of [Mn2(μ-RCOO)2(μ-OR)2]2+ type dinuclear manganese(III,III) complexes. Inorganica Chim. Acta 372 (1), 275–280. doi:10.1016/j.ica.2011.03.004
Biot, C., Castro, W., Botte, C. Y., and Navarro, M. (2012). The therapeutic potential of metal-based antimalarial agents: implications for the mechanism of action. Dalton Trans. 41, 6335–6349. doi:10.1039/c2dt12247b
Biot, C., and Dive, D. (2010). Bioorganometallic chemistry and malaria. Top. Organomet. Chem. 32, 155–193. doi:10.1007/978-3-642-13185-1_7
Biot, C., Nosten, F., Fraisse, L., Ter-Minassian, D., Khalife, J., Dive, D., et al. (2011). The antimalarial ferroquine: from bench to clinic. Parasite 18, 207–214. doi:10.1051/parasite/2011183207
Caggiano, G., Coretti, C., Bartolomeo, N., Lovero, G., De Giglio, O., and Montagna, M. T. (2015). Candida bloodstream infections in Italy: changing epidemiology during 16 Years of surveillance. BioMed Res. Int. 2015, 1–9. doi:10.1155/2015/256580
Cendejas-Bueno, E., Kolecka, A., Alastruey-Izquierdo, A., Theelen, B., Groenewald, M., Kostrzewa, M., et al. (2012). Reclassification of the Candida haemulonii complex as Candida haemulonii (C. haemulonii group I), C. duobushaemulonii sp. nov. (C. haemulonii group II), and C. haemulonii var. vulnera var. nov.: three multiresistant human pathogenic yeasts. J. Clin. Microbiol. 50, 3641–3651. doi:10.1128/JCM.02248-12
Chen, X., Tang, L.-J., Sun, Y.-N., Qiu, P.-H., and Liang, G. (2010). Syntheses, characterization and antitumor activities of transition metal complexes with isoflavone. J. Inorg. Biochem. 104, 379–384. doi:10.1016/j.jinorgbio.2009.11.008
Cipriani, M., Toloza, J., Bradford, L., Putzu, E., Vieites, M., Curbelo, E., et al. (2014). Effect of the metal ion on the anti T. Cruzi activity and mechanism of action of 5-nitrofuryl-containing thiosemicarbazone metal complexes. Eur. J. Inorg. Chem. 2014, 4677–4689. doi:10.1002/ejic.201402614
Dasari, S., and Tchounwou, P. B. (2014). Cisplatin in cancer therapy: molecular mechanisms of action. Eur. J. Pharmacol. 740, 364–378. doi:10.1016/j.ejphar.2014.07.025
de Almeida, J. N., Motta, A. L., Rossi, F., Abdala, E., Pierrotti, L. C., Kono, A. S. G., et al. (2012). First report of a clinical isolate of Candida haemulonii in Brazil. Clinics 67, 1229–1231. doi:10.6061/clinics/2012(10)18
Demoro, B., Sarniguet, C., Sanchez-Delgado, R., Rossi, M., Liebowitz, D., Caruso, F., et al. (2012). New organoruthenium complexes with bioactive thiosemicarbazones as co-ligands: potential anti-trypanosomal agents. Dalton Trans. 41, 1534–1543. doi:10.1039/C1DT11519G
Ding, B., Shao, S., Jiang, F., Dang, P., Sun, C., Huang, S., et al. (2019). MnO2-Disguised upconversion hybrid nanocomposite: an ideal architecture for tumor microenvironment-triggered UCL/MR bioimaging and enhanced chemodynamic therapy. Chem. Mater. 31 (7), 2651–2660. doi:10.1021/acs.chemmater.9b00893
Dominguez Muro, M., Motta, F. d. A., Burger, M., Melo, A. S. d. A., and Dalla-Costa, L. M. (2012). Echinocandin resistance in two Candida haemulonii isolates from pediatric patients. J. Clin. Microbiol. 50, 3783–3785. doi:10.1128/JCM.01136-12
Dyson, P. J., and Sava, G. (2006). Metal-based antitumour drugs in the post genomic era. Dalton Trans. 16, 1929–1933. doi:10.1039/b601840h
Emsley, J. (2001). „Nature’s building blocks: an A-Z guide to the elements - manganese”. Oxford University Press.
Enikeeva, K. R., Shamsieva, A. V., Kasimov, A. I., Fayzullin, R. R., Litvinov, I. A., Khrizanforova, V. V., et al. (2023). Binuclear manganese(II) complexes based on pyridyl-containing dialkylphosphine oxides. Inorganica Chim. Acta 558, 121741. doi:10.1016/j.ica.2023.121741
Ermakova, E. A., Eremina, J. A., Smirnova, K. S., Klyushova, L. S., Kal’nyi, D. B., Sukhikh, T. S., et al. (2021). Mixed-ligand manganese(II) complexes with 5-phenyltetrazole and polypyridine derivatives: synthesis, crystal structures and biological activity. Results Chem. 3, 100239. doi:10.1016/j.rechem.2021.100239
Eskandari, A., and Suntharalingam, K. (2019). A reactive oxygen species-generating, cancer stem cell-potent manganese(ii) complex and its encapsulation into polymeric nanoparticles. Chem. Sci. 10 (33), 7792–7800. doi:10.1039/c9sc01275c
Farghadani, R., Rajarajeswaran, J., Mohd Hashim, N. B., Abdulla, M. A., and Muniandy, S. (2017). A novel β-diiminato manganeseIII complex as the promising anticancer agent induces G0/G1 cell cycle arrest and triggers apoptosis via mitochondrial-dependent pathways in MCF-7 and MDA-MB-231 human breast cancer cells. RSC Adv. 7, 24387–24398. doi:10.1039/C7RA02478A
Fath, M. H., Babakhaniyan, K., Zokaei, M., Yaghoubian, A., Akbari, S., Khorsandi, M., et al. (2022). Anti-cancer peptide-based therapeutic strategies in solid tumors. Cell. Mol. Biol. Lett. 9, 27–33. doi:10.1186/s11658-022-00332-w
Firuzabadi, F. D., and Asadi, Z. (2021). Experimental and computational studies of the interaction of gemifloxacin and manganese (II) gemifloxacin complex with DNA. J. Mol. Struct. 1224, 129248. doi:10.1016/j.molstruc.2020.129248
Florido, A., Saraiva, N., Cerqueira, S., Almeida, N., Parsons, M., Batinic-Haberle, I., et al. (2019). The manganese(III) porphyrin MnTnHex-2-PyP5+ modulates intracellular ROS and breast cancer cell migration: impact on doxorubicin-treated cells. Redox Biol. 20, 367–378. doi:10.1016/j.redox.2018.10.016
Frei, A., Verderosa, A. D., Elliott, A. G., Zuegg, J., and Blaskovich, M. A. T. (2023). Metals to combat antimicrobial resistance. Nat. Rev. Chem. 7, 202–224. doi:10.1038/s41570-023-00463-4
Fukuda, Y., and Sone, K. (1970). Formation and absorption spectra of mixed copper(II) chelates containing acetylacetone and 2,2′-bipyridine or 1,10-phenanthroline. Bull. Chem. Soc. Jpn. 43 (2), 556–558. doi:10.1246/bcsj.43.556
Gambino, D., and Otero, L. (2012). Perspectives on what ruthenium-based compounds could offer in the development of potential antiparasitic drugs. Inorganica Chim. Acta 393, 103–114. doi:10.1016/j.ica.2012.05.028
Ghosh, R. D., Banerjee, K., Das, S., Ganguly, A., Chakraborty, P., Sarkar, A., et al. (2013). A novel manganese complex, Mn-(II) N-(2-hydroxy acetophenone) glycinate overcomes multidrug-resistance in cancer. Eur. J. Pharm. Sci. 49, 737–747. doi:10.1016/j.ejps.2013.05.002
Glisic, B. D., and Djuran, M. I. (2014). Gold complexes as antimicrobial agents: an overview of different biological activities in relation to the oxidation state of the gold ion and the ligand structure. Dalton Trans. 43, 5950–5969. doi:10.1039/c4dt00022f
Gordon, A. T., Abosede, O. O., Ntsimango, S., Hosten, E. C., Myeza, N., Eyk, A. v., et al. (2022). Synthesis and anticancer evaluation of copper(II)- and manganese(II)- theophylline mixed ligand complexes. Polyhedron 214, 115649. doi:10.1016/j.poly.2022.115649
Hanson, S., Dharan, A., Jinsha, P. V., Pal, S., Nair, B. G., Kar, R., et al. (2023). Corrigendum: paraptosis: a unique cell death mode for targeting cancer. Front. Pharmacol. 14, 1274076. doi:10.3389/fphar.2023.1274076
Hao, Y., Zheng, C., Wang, L., Zhang, J., Niu, X., Song, Q., et al. (2017). Tumor acidity-activatable manganese phosphate nanoplatform for amplification of photodynamic cancer therapy and magnetic resonance imaging. Acta Biomater. 62, 293–305. doi:10.1016/j.actbio.2017.08.028
Haque, S., Tripathy, S., and Ranjan, P. C. (2021). Manganese-based advanced nanoparticles for biomedical applications: future opportunity and challenges. Nanoscale 39, 16405–16426. doi:10.1039/D1NR04964J
Horning, K. J., Caito, S. W., Tipps, K. G., Bowman, A. B., and Aschner, M. (2015). Manganese is essential for neuronal health. Annu. Rev. Nutr. 35, 71–108. doi:10.1146/annurev-nutr-071714-034419
Icsel, C., Yilmaz, V. T., Aydinlik, S., and Aygun, M. (2020). New manganese(II), iron(II), cobalt(II), nickel(II) and copper(II) saccharinate complexes of 2,6-bis(2-benzimidazolyl)pyridine as potential anticancer agents. Eur. J. Med. Chem. 202, 112535. doi:10.1016/j.ejmech.2020.112535
Jakupec, M. A., Galanski, M. S., Arion, V. B., Hartinger, C. G., and Keppler, B. K. (2008). Antitumour metal compounds: more than theme and variations. Dalton Trans. 2, 183–194. doi:10.1039/b712656p
Kalaiselvan, C. R., Laha, S. S., Somvanshi, S. B., Tabish, T. A., Thorat, N. D., and Sahu, N. K. (2022). Manganese ferrite (MnFe2O4) nanostructures for cancer theranostics. Coord. Chem. Rev. 473, 214809. doi:10.1016/j.ccr.2022.214809
Kani, I., Atlier, O., and Guven, K. (2016). Mn(II) complexes with bipyridine, phenanthroline and benzoic acid: biological and catalase-like activity. J. Chem. Sci. 128, 523–536. doi:10.1007/s12039-016-1050-z
Kellet, A., O’Connor, M., McCann, M., Howe, O., Casey, A., McCarron, P., et al. (2011). Water-soluble bis(1,10-phenanthroline) ocatnedioate Cu2+ and Mn2+ complexes with unprecedented nano and picomolar in vitro ctytotoxicity: promising leads for chemotherapeutic drug development. Med. Chem. Commun. 2, 579–584. doi:10.1039/C0MD00266F
Kongot, M., Reddy, D. S., Singh, V., Patel, R., Singhal, N. K., and Kumar, A. (2020). A manganese (II) complex tethered with S-benzyldithiocarbazate Schiff base: synthesis, characterization, in-vitro therapeutic activity and protein interaction studies. Spectrochimica Acta Part A Mol. Biomol. Spectrosc. 231, 118123. doi:10.1016/j.saa.2020.118123
Kuang, F., Liu, J., Tang, D., and Kang, R. (2020). Oxidative damage and antioxidant defense in ferroptosis. Front. Cell Dev. Biol. 8, 586578. doi:10.3389/fcell.2020.586578
Kumar Singh, A., Kumar, A., Singh, H., Sonawane, P., Pathak, P., Grishina, M., et al. (2023). Metal complexes in cancer treatment: journey so far. Chem. Biodivers. 20 (4), e202300061. doi:10.1002/cbdv.202300061
Kurz, P. (2009). Oxygen evolving reactions catalysed by manganese–oxo-complexes adsorbed on clays. Dalton Trans. 6103, 6103–6108. doi:10.1039/B904532E
Lawrence, M. L., Shell, S. M., and Beckford, F. A. (2020). Binuclear manganese-iron complexes containing ferrocenyl thiosemicarbazones: biological activity and carbon monoxide-releasing properties. Inorganica Chim. Acta 507, 119548. doi:10.1016/j.ica.2020.119548
Lewandowski, E. M., Skiba, J., Torelli, N. J., Rajnisz, A., Solecka, J., Kowalski, K., et al. (2015). Antibacterial properties and atomic resolution X-ray complex crystal structure of a ruthenocene conjugated β-lactam antibiotic. Chem. Commun. 51, 6186–6189. doi:10.1039/C5CC00904A
Lin, L. S., Song, J., Song, L., Ke, K., Liu, Y., Zhou, Z., et al. (2018). Simultaneous fenton-like ion delivery and glutathione depletion by MnO2 - based nanoagent to enhance chemodynamic therapy. Angew. Chem. Int. Ed. 57 (18), 4902–4906. doi:10.1002/anie.201712027
Liu, J., Bu, W., Pan, L., and Shi, J. (2013). NIR-triggered anticancer drug delivery by upconverting nanoparticles with integrated azobenzene-modified mesoporous silica. Angew. Chem. 125, 4471–4475. doi:10.1002/ange.201300183
Liu, J., Guo, W., Li, J., Li, X., Geng, J., Chen, Q., et al. (2015). Tumor-targeting novel manganese complex induces ROS-mediated apoptotic and autophagic cancer cell death. Int. J. Mol. Med. 35, 607–616. doi:10.3892/ijmm.2015.2073
Madalan, A. M., Kravtsov, V. C., Pajic, D., Zadro, K., Simonov, Y. A., Stanica, N., et al. (2004). „Chemistry at the apical position of square-pyramidal copper(II) complexes: synthesis, crystal structures, and magnetic properties of mononuclear Cu(II), and heteronuclear Cu(II)–Hg(II) and Cu(II)–Co(II) complexes containing [Cu(AA)(BB)]+ moieties (AA=acetylacetonate, salicylaldehydate; BB=1,10-phenanthroline, Me2bipy=4,4′-dimethyl-2,2′-bipyridine). Inorganica Chim. Acta 357, 4151–4164. doi:10.1016/j.ica.2004.06.010
Mamta, , Subhash, , Pinki, , and Chaudhary, A. (2023). In vitro cytotoxicity and antimicrobial evaluation of novel 24–28 membered Schiff base octaazamacrocyclic complexes of manganese(II): synthesis, characterization, DFT and molecular docking studies. J. Mol. Struct. 1275, 134667. doi:10.1016/j.molstruc.2022.134667
Nagle, A. S., Khare, S., Kumar, A. B., Supek, F., Buchynskyy, A., Mathison, C. J. N., et al. (2014). Recent developments in drug discovery for leishmaniasis and human african trypanosomiasis. Chem. Rev. 114, 11305–11347. doi:10.1021/cr500365f
Navarro, M., Castro, W., and Biot, C. (2012). Bioorganometallic compounds with antimalarial targets: inhibiting hemozoin formation. Organometallics 31, 5715–5727. doi:10.1021/om300296n
Ndagi, U., Mhlongo, N., and Soliman, M. E. (2017). Metal complexes in cancer therapy - an update from drug design perspective. Drug Des. Dev. Ther. 11, 599–616. doi:10.2147/DDDT.S119488
Ntanatsidis, S., Perontsis, S., Konstantopoulou, S., Kalogiannis, S., Hatzidimitriou, A. G., Papadopoulos, A. N., et al. (2022). Manganese(II) complexes of substituted salicylaldehydes and α-diimines: synthesis, characterization and biological activity. J. Inorg. Biochem. 227, 111693. doi:10.1016/j.jinorgbio.2021.111693
O’Connell, K. M. G., Hodgkinson, J. T., Sore, H. F., Welch, M., Salmond, G. P. C., and Spring, D. R. (2013). Combating multidrug-resistant bacteria: current strategies for the discovery of novel antibacterials. Angew. Chem. Int. Ed. 52 (41), 10706–10733. doi:10.1002/anie.201209979
Osowole, A. A., Ekennia, A. C., Olubiyi, O. O., and Olagunju, M. (2016). Synthesis, spectral, thermal, antibacterial and molecular docking studies of some metal(II) complexes of 2-(1,3-benzothiazol-2-ylamino)naphthalene-1,4-dione. Res. Chem. Intermed. 43, 2565–2585. doi:10.1007/s11164-016-2780-8
Patra, M., Gasser, G., and Metzler-Nolte, N. (2012). Small organometallic compounds as antibacterial agents. Dalton Trans. 41, 6350–6358. doi:10.1039/c2dt12460b
Patra, M., Ingram, K., Pierroz, V., Ferrari, S., Spingler, B., Gasser, R. B., et al. (2013). [(η6-Praziquantel)Cr(CO)3] derivatives with remarkable in vitro anti-schistosomal activity. Chem. – A Eur. J. 19, 2232–2235. doi:10.1002/chem.201204291
Paulovicova, A., El-Ayaan, U., and Fukuda, Y. (2001). Synthesis, characterization and X-ray crystal structures of two five-coordinate ternary copper(II) complexes containing acetylacetonate with 1,10-phenanthroline and 2,9-dimethyl phenanthroline. Inorganica Chim. Acta 321, 56–62. doi:10.1016/S0020-1693(01)00514-X
Pessoa, J., Martins, M., Casimiro, S., Perez-Plasencia, C., and Shoshan-Barmatz, V. (2022). Editorial: altered expression of proteins in cancer: function and potential therapeutic targets. Front. Oncol. 12, 949139. doi:10.3389/fonc.2022.949139
Piras, C. C., Mahon, C. S., Genever, P. G., Smith, D. K., Ma, Z., Yang, X., et al. (2022). Shaping and patterning supramolecular Materials─Stem cell-compatible dual-network hybrid gels loaded with silver nanoparticles. ACS Biomaterials Sci. Eng. 8 (5), 1829–1840. doi:10.1021/acsbiomaterials.1c01560
Prudhomme, M. (2013). „Advances Anticancer Agents Med. Chemistry”, 2. doi:10.2174/97816080549611130201
Qiu-Yun, C., Dong-Fang, Z., Juan, H., Wen-Jie, G., and Jing, G. (2010). Synthesis, anticancer activities, interaction with DNA and mitochondria of manganese complexes. J. Inorg. Biochem. 104, 1141–1147. doi:10.1016/j.jinorgbio.2010.06.012
Ramos, L. S., Figueiredo-Carvalho, M. H. G., Barbedo, L. S., Ziccardi, M., Chaves, A., Zancopé-Oliveira, R. M., et al. (2015). Candida haemulonii complex: species identification and antifungal susceptibility profiles of clinical isolates from Brazil. J. Antimicrob. Chemother. 70, 111–115. doi:10.1093/jac/dku321
Reddig, N., Pursche, D., Kloskowski, M., Slinn, C., Baldeau, S. M., and Rompel, A. (2004). Tuning the catalase activity of dinuclear manganese complexes by utilizing different substituted tripodal ligands. Eur. J. Inorg. Chem. 2004, 879–887. doi:10.1002/ejic.200300157
Renslo, A. R., and McKerrow, J. H. (2006). Drug discovery and development for neglected parasitic diseases. Nat. Chem. Biol. 2, 701–710. doi:10.1038/nchembio837
Richardson, M., and Lass-Florl, C. (2008). Changing epidemiology of systemic fungal infections. Clin. Microbiol. Infect. 14, 5–24. doi:10.1111/j.1469-0691.2008.01978.x
Sánchez-Delgado, R. A., Anzellotti, A., and Suarez, L. (2004). Metal complexes as chemotherapeutic agents against tropical diseases: malaria, trypanosomiasis, and leishmaniasis. Metal Ions Biol. Syst. 41, 379–419. doi:10.1201/9780203913703.ch12
Sanguinetti, M., Posteraro, B., and Lass-Florl, C. (2015). Antifungal drug resistance among Candida species: mechanisms and clinical impact. Mycoses 58, 2–13. doi:10.1111/myc.12330
Schillaci, D., Spanò, V., Parrino, B., Carbone, A., Montalbano, A., Barraja, P., et al. (2017). Pharmaceutical approaches to target antibiotic resistance mechanisms. J. Med Chem. 60, 8268–8297. doi:10.1021/acs.jmedchem.7b00215
Schmidt, S. B., and Husted, S. (2019). The biochemical properties of manganese in plants. Plants 8 (10), 381. doi:10.3390/plants8100381
Signorella, S., and Hureau, C. (2012). Bioinspired functional mimics of the manganese catalases. Coord. Chem. Rev. 256, 1229–1245. doi:10.1016/j.ccr.2012.02.003
Signorella, S., Rompel, A., Buldt-Karentzopoulos, K., Krebs, B., Pecoraro, V. L., and Tuchagues, J. P. (2007). Reevaluation of the kinetics of polynuclear mimics for manganese catalases. Inorg. Chem. 46, 10864–10868. doi:10.1021/ic070180e’
Slator, C., Molphy, Z., McKee, V., and Kellett, A. (2017). Triggering autophagic cell death with a di-manganese(II) developmental therapeutic. Redox Biol. 12, 150–161. doi:10.1016/j.redox.2017.01.024
Tang, D., Chen, X., Kang, R., and Kroemer, G. (2020). Ferroptosis: molecular mechanisms and health implications. Cell Res. 31, 107–125. doi:10.1038/s41422-020-00441-1
Tian, J.-l., Yan, H.-t., Hu, Q., Liu, R.-x., Liu, L.-m., and Liu, Y.-c. (2023). The manganese(II) and cobalt(II) complexes of enrofloxacin showed comparable antibacterial activity: synthesis, crystal structure and antibacterial activity. J. Mol. Struct. 1289, 135842. doi:10.1016/j.molstruc.2023.135842
Triller, M. U., Hsieh, W.-Y., Pecoraro, V. L., Rompel, A., and Krebs, B. (2002). Preparation of highly efficient manganese catalase mimics. Inorg. Chem. 41, 5544–5554. doi:10.1021/ic025897a
Vazquez-Fernandez, M. A., Fernandez-Garcia, M. I., Gonzalez-Riopedre, G., Maneiro, M., and Rodriguez-Douton, M. J. (2011). Self-assembled biomimetic catalysts: studies of the catalase and peroxidase activities of Mn(III)-Schiff base complexes. J. Coord. Chem. 64, 3843–3858. doi:10.1080/00958972.2011.633164
Welch, G. C., Prietto, R., Dureen, M. A., Lough, A. J., Labeodan, O. A., Holtrichter-Rossman, T., et al. (2009). Reactions of phosphines with electron deficient boranes. Dalton Trans. 6103, 6108. doi:10.1039/B814486A
Welsh, J. B., Sapinoso, L. M., Kern, S. G., Brown, D. A., Liu, T., Bauskin, A. R., et al. (2003). Large-scale delineation of secreted protein biomarkers overexpressed in cancer tissue and serum. Proc. Natl. Acad. Sci. 100 (6), 3410–3415. doi:10.1073/pnas.0530278100
Wu, A. J., Penner-Hahn, J. E., and Pecoraro, V. L. (2004). Structural, spectroscopic and reactivity models for the manganese catalases. Chem. Rev. 104 (2), 903–938. doi:10.1021/cr020627v
Yu, Y., Yan, Y., Niu, F., et al. (2021). Ferroptosis: a cell death connecting oxidative stress, inflammation and cardiovascular diseases. Cell Death Discov. 7, 193. doi:10.1038/s41420-021-00579-w
Zhang, Z., He, R., Yan, K., Guo, Q.-n., Lu, Y.-g., Wang, X.-x., et al. (2009). Synthesis and in vitro and in vivo evaluation of manganese(III) porphyrin-dextran as a novel MRI contrast agent. Bioorg. Med. Chem. Lett. 19 (23), 6675–6678. doi:10.1016/j.bmcl.2009.10.003
Zhao, P., Liu, M.-C., Zheng, M., Jin, S.-f., Tang, D.-T., Chen, J., et al. (2016). G-quadruplex DNA interactions, docking and cell photocytotoxicity research of porphyrin dyes. Dyes Pigments 128, 41–48. doi:10.1016/j.dyepig.2016.01.009
Zheng, R., Guo, J., Cai, X., Bin, L., Lu, C., Singh, A., et al. (2022). Manganese complexes and manganese-based metal-organic frameworks as contrast agents in MRI and chemotherapeutics agents: applications and prospects. Colloids Surfaces B Biointerfaces 213, 112432. doi:10.1016/j.colsurfb.2022.112432
Keywords: manganese, coordination compounds, cancer, bacterial, fungus
Citation: Kozieł S, Wojtala D, Szmitka M, Sawka J and Komarnicka UK (2024) Can Mn coordination compounds be good candidates for medical applications?. Front. Chem. Biol 3:1337372. doi: 10.3389/fchbi.2024.1337372
Received: 12 November 2023; Accepted: 16 January 2024;
Published: 31 January 2024.
Edited by:
Sylvia Mary Draper, Trinity College Dublin, IrelandReviewed by:
Andrea Erxleben, University of Galway, IrelandCopyright © 2024 Kozieł, Wojtala, Szmitka, Sawka and Komarnicka. This is an open-access article distributed under the terms of the Creative Commons Attribution License (CC BY). The use, distribution or reproduction in other forums is permitted, provided the original author(s) and the copyright owner(s) are credited and that the original publication in this journal is cited, in accordance with accepted academic practice. No use, distribution or reproduction is permitted which does not comply with these terms.
*Correspondence: Sandra Kozieł, c2FuZHJhLmtvemllbEB1d3IuZWR1LnBs; Urszula K. Komarnicka, dXJzenVsYS5rb21hcm5pY2thMkB1d3IuZWR1LnBs
Disclaimer: All claims expressed in this article are solely those of the authors and do not necessarily represent those of their affiliated organizations, or those of the publisher, the editors and the reviewers. Any product that may be evaluated in this article or claim that may be made by its manufacturer is not guaranteed or endorsed by the publisher.
Research integrity at Frontiers
Learn more about the work of our research integrity team to safeguard the quality of each article we publish.