- Department of Chemistry, Faculty of Science, Hokkaido University, Sapporo, Japan
We achieved an integration of a lithiation of aryl bromides, a zincation of thus-generated aryllithiums, and a Negishi coupling of the arylzinc with an aryl halide in one flow. Taking advantages of flow microreactors, biaryls bearing a wide range of functional groups, especially biaryls bearing multiple electrophilic-functionalities were synthesized.
Introduction
As awarded the Nobel Prize in Chemistry in 2010, palladium-catalyzed cross-couplings, particularly coupling of aryl metals with aryl halides, are ubiquitous in our society. A wide range of molecules have been obtained through cross-coupling in the pharmaceutical, agrochemical, and material-chemistry fields (Nicolaou et al., 2005; Corbet and Mignani, 2006; Magano and Dunetz, 2011, respectively). Current social development has accelerated the demand for novel and high-performance organic molecules. Thus, a rapid process that allows chemists in R&D to prepare libraries of candidate molecules quickly and manufacturers to promptly supply their customers with sufficient products for cross-coupling reactions is urgently required.
We focused on the coupling process referred to as the Murahashi coupling (Murahashi et al., 1979; Giannerini et al., 2013) using aryl lithium as the aryl metal. Because of the low electronegativity of lithium (Pauling scale: 0.98, c.f. 1.3 for Mg and 2.0 for B), Murahashi coupling was assumed to be a rapid synthetic method. We demonstrated continuous-flow Murahashi-coupling (Nagaki et al., 2010; Nagaki et al., 2012b) that enables the syntheses of biaryls and biheteroaryls in high yields within 90 s under mild conditions (temperature for coupling: 50 °C). However, because of the high reactivity of aryl lithium, the functional groups were limited and no electrophilic functionalities were utilized for both aryl lithium and its coupling partner (aryl halide). This resulted in lower product diversity and overshadowed the utility of this process.
To achieve rapid and functional group-tolerant cross-coupling, we applied the strategy of flash chemistry (Yoshida, 2008; Colella et al., 2020; Nagaki et al., 2021; Takumi, et al., 2022) and reaction integration (Figure 1) using flow reactors. The use of flow reactors, especially micro-meter-sized flow reactors, has several benefits, such as an efficient heat transfer, a rapid mixing, a precise control of the reaction time and a direct use of transient unstable species, in the organic synthesis field (Heinz et al., 2021; Mazzarella et al., 2021; Ashikari, et al., 2022; Miyamura and Kobayashi, 2022; Nogami et al., 2022). Taking the advantage of the flow microreactors, highly-selective chemical reactions have been achieved (Ashikari et al., 2019; Sugisawa et al., 2020; Ashikari et al., 2021). Based on this idea, we envisaged that aryl lithiums bearing electrophilic functionalities are generated by functional-group-tolerant halogen–lithium exchange reactions and, before they decompose, are converted to aryl zincs. Reaction integration (Nagaki, 2021) allows the generated aryl zincs to be subsequently engaged in Negishi coupling in one flow. The modest electronegativity of zinc (1.7) causes adequate reactivity, resulting in functional group tolerance. Herein, we report the flow microsynthesis of functionalized biaryls by integrating lithiation, zincation, and coupling reactions mediated by functionalized aryl zinc. We applied this strategy to metal-selective cross-coupling reactions and demonstrated the synthesis of biaryls bearing metal substituents. Moreover, by utilizing flash chemistry thoroughly, we demonstrated room-temperature coupling of aryl halides.
Materials and methods
Materials
Chemicals were purchased from Kanto Chemical, FUJIFILM Wako Pure Chemical, Tokyo Chemical Industry, and Sigma–Aldrich, and were used without further purification unless otherwise stated.
Flow synthesis
T-shaped stainless steel (SUS304) micromixers with inner diameters of 500 and 250 μm were manufactured by Sanko Seiki. Stainless steel (SUS316) flow reactors with inner diameters of 1000 and 2,170 μm were purchased from GL Sciences. PTFE tubes with inner diameters of 1000 and 1588 μm were purchased from ISIS. Syringe pumps (Harvard Model PHD 2000 or PHD ULTRA) equipped with gastight syringes (purchased from SGE) were used to introduce the reaction solutions. Product yields were determined through GC analyses (Shimadzu GC-2014) calibrated using internal standards with commercial samples.
Results and discussion
Initially, we studied the feasibility, rapidity and the flexibility of this concept. Using conditions similar to those described in a previous publication (Nagaki et al., 2010), we attempted the flow Murahashi coupling of 4-anisyl lithium with arylbromides bearing electrophilic functional groups in a flow microreactor (Scheme 1). After the halogen–lithium exchange reaction of 4-bromoanisole (1a) with n-butyllithium (0°C, 2.4 s), aryl lithium was reacted with aryl bromides (2a–c) and 5 mol% palladium catalyst (PEPPSI-IPr) at 50 °C for 89 s. As anticipated, the presence of electrophilic groups led to very low yields (less than 3%) if compared to system where such functions are not present (Nagaki et al., 2010). GC-MS analyses indicated that the crude mixture of the reaction using 4-bromobenzaldehyde (2a) contained 4-methoxybenzhydrol, which can be generated by a nucleophilic attack of the aryl lithium on the formyl group of 2a. These results demonstrate that aryl lithiums tend to react with electrophilic functional groups before initiating the Murahashi coupling; therefore, we investigated the integration strategy. After lithiation, aryl lithium was reacted with 0.5 equivalent amount of zinc chloride to be converted to aryl zinc, which was subsequently reacted under similar coupling conditions. The corresponding biaryls (3aa–ac) were obtained in high yields. Notably, this series of transformations ended within 92 s owing to the fast generation of aryl lithium and the sufficient reactivity of aryl zinc.
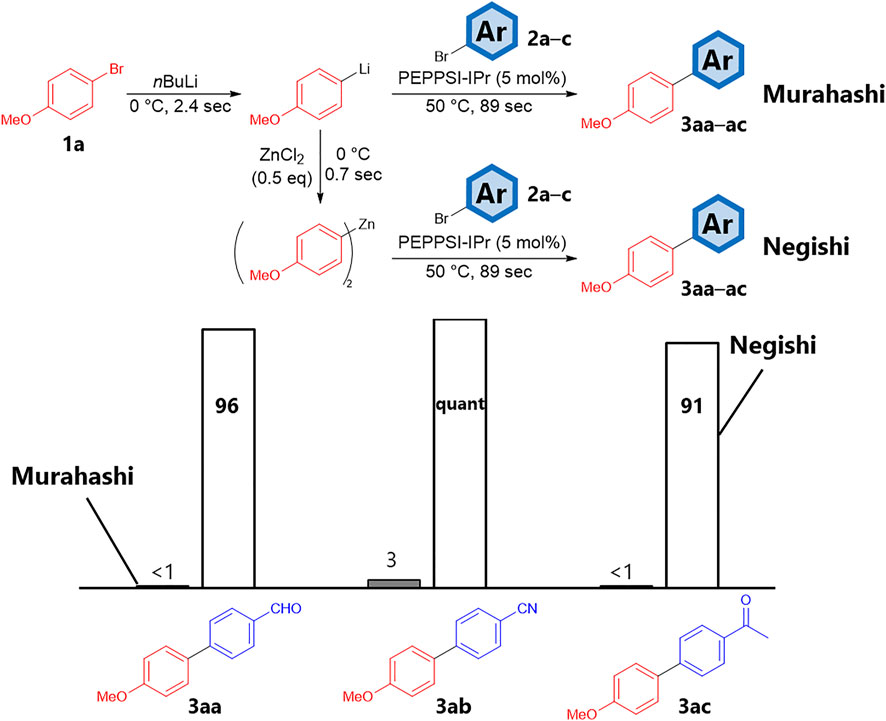
SCHEME 1. Reaction yields of Murahashi (left) and Negishi (right) coupling using aryl halides bearing electrophilic functionalities (2a–2c) in flow microreactor. Bars are the reaction yields determined by GC.
Once the feasibility of the flow integration of lithiation, zincation, and coupling was confirmed, the coupling of different aryl zinc and aryl halides bearing electrophilic functional groups was investigated. As summarized in Table 1, aryl halides bearing electrophilic functional groups were introduced into micromixer M1 (internal diameter: 500 μm) at 0°C, where a solution of n-butyllithium was also introduced. After 2.4 s, the resulting solution was introduced into micromixer M2 (internal diameter: 500 μm) and mixed with a solution of zinc chloride to generate the functionalized aryl zinc. Thereafter, aryl zinc was mixed with another aryl halide bearing electrophilic functionalities and PEPPSI-IPr or Pd(PPh3)4 in micromixer M3. The resulting solution was passed through a tube reactor and heated to 50°C to promote the cross-coupling reaction, and to increase the reaction conversion we utilized longer tube reactor to prolong the reaction time. From the aryl halides bearing a cyano group (1b and 1c, entries 1–5) and an ester moiety (1d, entry 6), the corresponding biaryls were obtained in good to high yields. Interestingly, when using aryl iodides (2f and 2g) as coupling partners, bromo-substituted aryl zincs, which were generated from the selective mono-lithiation of dibromobenzene 1e based on the previously reported conditions (Nagaki et al., 2007; Usutani et al., 2007), selectively reacted at the iodo groups of 2f and 2g to generate bromo-substituted biaryls 3ef and 3eg (entries 7 and 8), respectively. Notably, because the aryl lithiums were generated by the halogen–lithium exchange reaction, the position of the substituents (ortho- and para-position) could be controlled by changing the starting materials (for instance, entry 1 vs 2), which deprotonative lithiation such as directed ortho-lithiation (Snieckus, 1990) seldom achieved.
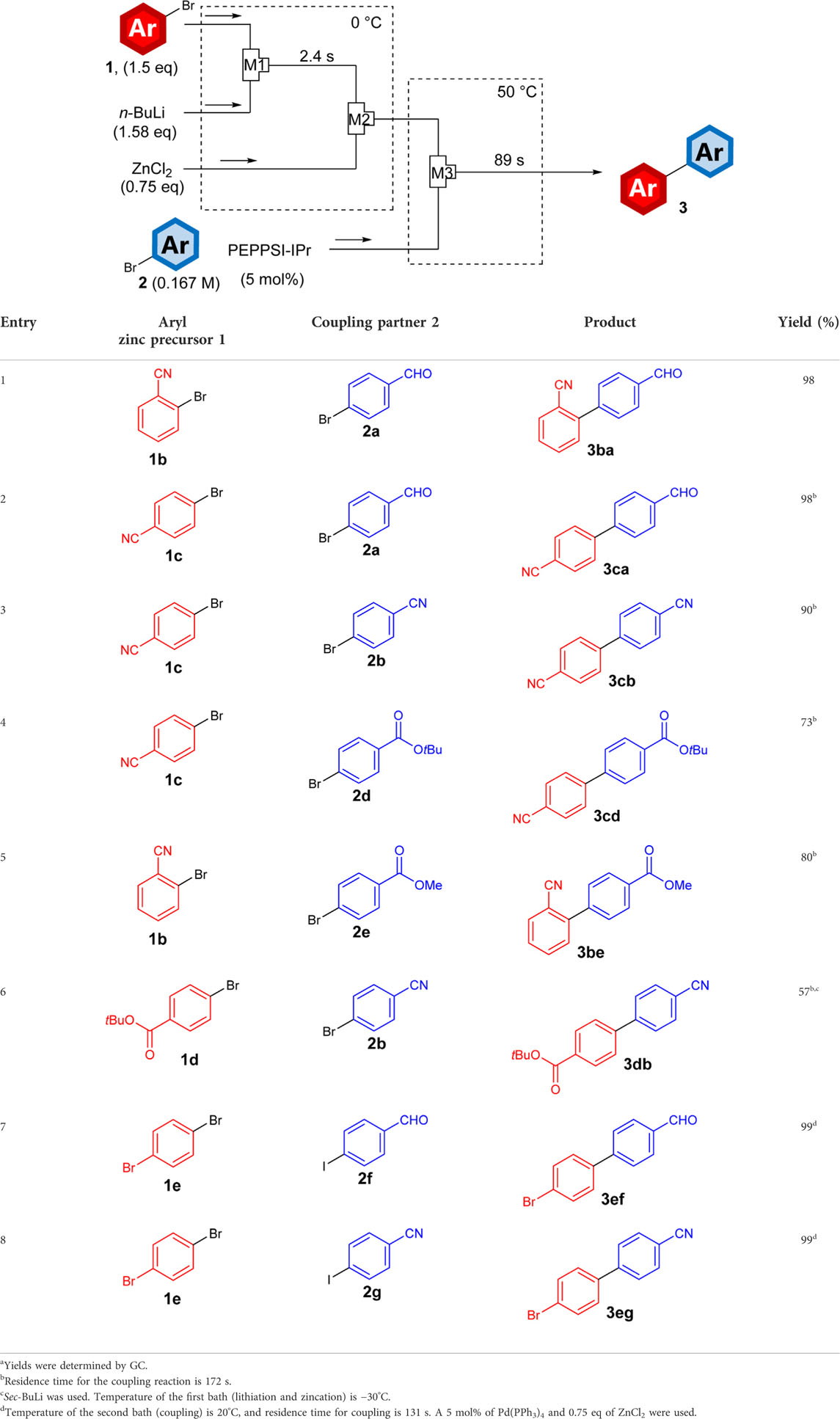
TABLE 1. Integrated lithiation-zincation-coupling process of substituted aryl zinc precursors 1 with substituted coupling partner 2 in flowmicro reactor.a
The success of the halogen-selective cross-coupling of this process prompted us to attempt metal-selective cross-coupling to generate metal-substituted biaryls. Based on our previous efforts to synthesize bimettalic arenes, which are arenes bearing multiple metal substituents and their cross-coupling reactions (Ashikari et al., 2020), we designed the flow system shown in Figure 2. In the flow reactors, aryl zincs were generated and subsequently reacted with aryl halides bearing boryl groups catalyzed by PEPPSI-IPr or Pd(PPh3)4. Using 4-bromobenzonitrile (1c) as the precursor of aryl zinc and 4-bromophenylboronic acid pinacol ester as the coupling partner, the corresponding biphenyl bearing the boryl group (3ch) was obtained in high yields. This Negishi-selectivity is derived from that the Suzuki coupling basically requires basic conditions and this flow reaction was carried out in the absence of a base. Because the coupling product 3ch still has a metal substituent (the boryl group), further transformations including Suzuki–Miyaura coupling are achievable. When the position of the cyano group is varied, the corresponding biphenyl with ortho- (3bh) and meta-substitution (3fh) can be obtained in high yields, demonstrating the advantage of site-selective lithiation enabled by the halogen–lithium exchange. Notably, from the bromo-substituted aryl zinc and an aryl iodide bearing a boryl group, the corresponding biphenyl bearing the bromo and boryl groups (3eh) was obtained in a high yield. This is an excellent example of halogen- (Br vs I) and metal-selective (Zn vs B) cross-couplings.
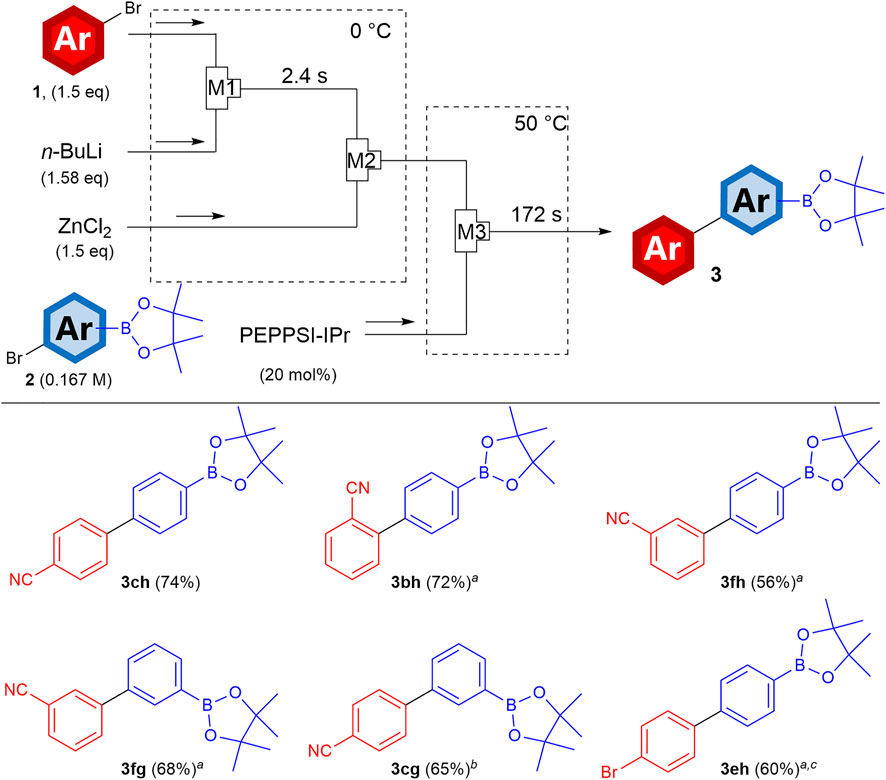
FIGURE 2. Schematic for metal-selective cross-coupling in flow microreactor. Yields were determined by GC. aAdditional stirring in batch for 30 min bAdditional stirring in batch for 12 h at 50°C, c Aryl iodide was used as the coupling partner, and Pd(PPh3)4 was used as the catalyst. 0.75 eq of ZnCl2 was used. For the coupling, the temperature was 20°C and the residence time was 131 s.
To prevent side reactions, lithiation and metalation steps for synthesizing aryl metal species were performed at cryogenic temperatures such as −78°C. However, the coupling reaction usually requires high temperatures such as the boiling points of the solvents. Thus, this series of transformations requires both low and high temperatures, leading to a waste of energy. Because the demand for products synthesized through cross-coupling is increasing, the energy loss of this process is also increasing. To save energy, in the context of Sustainable Development Goals, we investigated the integrated lithiation-zincation-coupling process at room temperature as a demonstration for the greener process.
With the flowmicro reactor dipped in a water bath as depicted in Figure 3, the cyano-substituted aryl bromide (1b) was mixed with n-butyllithium at 24°C, and 0.014 s later, zinc chloride was added to the mixture. After the addition of coupling partner 2f and palladium catalyst (Pd(PPh3)4, 5 mol% to the aryl iodide), the resulting mixture was passed through a tube reactor in a water bath (24 °C) until the reaction was quenched (residence time: 131 s). Under this full-room-temperature condition, 2f was fully consumed and the desired product, which is a key intermediate (Goossen and Melzer, 2007; Nagaki et al., 2016) of valsartan (Diovan, Novartis: US $773 million sales in 2021 (Novartis, 2022)), the angiotensin-II-receptor antagonist, was obtained in a quantitative yield.
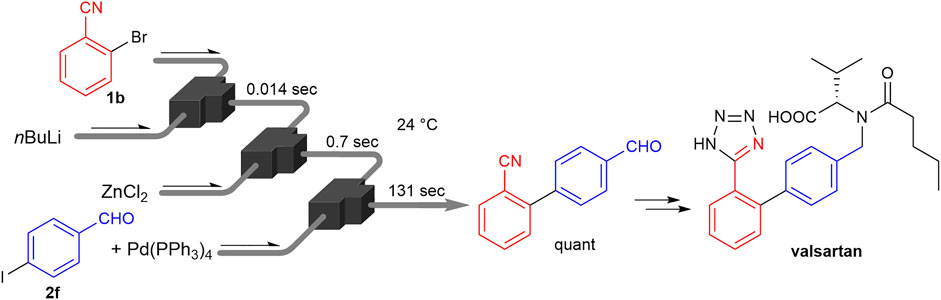
FIGURE 3. Full-room-temperature process of integrated lithiation-metalation-coupling for synthesizing the key intermediate of valsartan.
Conclusion
In conclusion, we achieved the integration of the halogen–lithium exchange reaction, zincation, and Negishi coupling in a flow microreactor. Using this methodology, a range of biphenyls with multiple electrophilic functionalities were synthesized within several minutes. The use of the zincyl group enables the boryl-group tolerant coupling, which flow Suzuki coupling (Shu et al., 2011; Nagaki et al., 2012a; Takahashi et al., 2020) cannot achieve. Although Knochel (Becker et al., 2015; Becker and Knochel, 2015) and Buchwald (Roesner and Buchwald, 2016) reported numerous examples of flow Negishi coupling, the proposed method has a higher flow rate of the substrate solution (typically 4 ml/min whereas those of the previous reports are 2 ml/min or less) and shorter reaction times, allowing higher productivity. Moreover, since this present method utilizes the halogen–lithium exchange reaction, site-selective lithiation can be achieved, which provides biaryls with varying substitution patterns.
Another aspect of the proposed method is flash chemistry in which intermediates with extremely short lifetimes can be utilized. The integrated lithiation-metalation-coupling process was achieved in a single pass at room temperature by controlling the reaction time of the lithiation step at 14 ms. Because no heating or cooling is required, the cross-coupling of aryl halides saves energy. We assert that this environmentally benign synthetic method will contribute to save energy and satisfy the increasing demand for cross-coupling products by supplying fruitful organic molecules.
Data availability statement
The original contributions presented in the study are included in the article/Supplementary Material, further inquiries can be directed to the corresponding author.
Author contributions
YA and AN contributed to conception and design of the study. YA and KG operated the investigation. YA wrote the first draft of the manuscript. AN supervised the project. All authors revised the manuscript, approved the manuscript to be published, and agree to be accountable for all aspects of the work in ensuring that questions related to the accuracy or integrity of any part of the work are appropriately investigated and resolved.
Funding
This work was supported by the Kanto Chemical Award in Synthetic Organic Chemistry, Japan, and the Kyoto Technoscience Center. This work was also supported by JSPS KAKENHI Grant Numbers, JP20K15276 (Grant-in-Aid for Early-Career Scientists), JP20KK0121 (Fostering Joint International Research (B)), JP21H01936 (Grant-in-Aid for Scientific Research (B)), JP21H01706 (Grant-in-Aid for Scientific Research (B)), JP19K22186 (Grant-in-Aid for Challenging Exploratory Research), and JP21H05080 (Grant-in-Aid for Transformative Research Areas (B)). This work was also partially supported by AMED (JP21ak0101156), the Core Research for Evolutional Science and Technology (CREST, JPMJCR18R1), New Energy and Industrial Technology Development Organization (NEDO, P19004), the Japan Keirin Autorace Foundation, and the Ogasawara Foundation for the Promotion of Science & Engineering.
Conflict of interest
The authors declare that the research was conducted in the absence of any commercial or financial relationships that could be construed as a potential conflict of interest.
Publisher’s note
All claims expressed in this article are solely those of the authors and do not necessarily represent those of their affiliated organizations, or those of the publisher, the editors and the reviewers. Any product that may be evaluated in this article, or claim that may be made by its manufacturer, is not guaranteed or endorsed by the publisher.
Supplementary material
The Supplementary Material for this article can be found online at: https://www.frontiersin.org/articles/10.3389/fceng.2022.964767/full#supplementary-material
References
Ashikari, Y., Kawaguchi, T., Mandai, K., Aizawa, Y., and Nagaki, A. (2020). A synthetic approach to dimetalated arenes using flow microreactors and the switchable application to chemoselective cross-coupling reactions. J. Am. Chem. Soc. 142, 17039–17047. doi:10.1021/jacs.0c06370
Ashikari, Y., Saito, K., Nokami, T., Yoshida, J., and Nagaki, A. (2019). Oxo-thiolation of cationically polymerizable alkenes using flow microreactors. Chem. Eur. J. 25, 15239–15243. doi:10.1002/chem.201903426
Ashikari, Y., Tamaki, T., Kawaguchi, T., Furusawa, M., Yonekura, Y., Ishikawa, S., et al. (2021). Switchable chemoselectivity of reactive intermediates formation and their direct use in a flow microreactor. Chem. Eur. J. 27, 16107–16111. doi:10.1002/chem.202103183
Ashikari, Y., Tamaki, T., Takahashi, Y., Yao, Y., Atobe, M., Nagaki, A., et al. (2022). Investigation of parameter control for electrocatalytic semihydrogenation in a proton-exchange membrane reactor utilizing bayesian optimization. Front. Chem. Eng. 3, 819752. doi:10.3389/fceng.2021.819752
Becker, M. R., Ganiek, M. A., and Knochel, P. (2015). Practical and economic lithiations of functionalized arenes and heteroarenes using Cy2NLi in the presence of Mg, Zn or La halides in a continuous flow. Chem. Sci. 6, 6649–6653. doi:10.1039/c5sc02558c
Becker, M. R., and Knochel, P. (2015). Practical continuous-flow trapping metalations of functionalized arenes and heteroarenes using TMPLi in the presence of Mg, Zn, Cu, or La halides. Angew. Chem. Int. Ed. 54, 12501–12505. doi:10.1002/anie.201502393
Colella, M., Tota, A., Takahashi, Y., Higuma, R., Ishikawa, S., Degennaro, L., et al. (20202020). Fluoro-substituted methyllithium chemistry: External quenching method using flow microreactors. Angew. Chem. Int. Ed. 59, 10924–10928. doi:10.1002/anie.202003831
Corbet, J.-P., and Mignani, G. (2006). Selected patented cross-coupling reaction technologies. Chem. Rev. 106, 2651–2710. doi:10.1021/cr0505268
Giannerini, M., Fañanás-Mastral, M., and Feringa, B. L. (2013). Direct catalytic cross-coupling of organolithium compounds. Nat. Chem. 5, 667–672. doi:10.1038/nchem.1678
Goossen, L. J., and Melzer, B. (2007). Synthesis of valsartan via decarboxylative biaryl coupling. J. Org. Chem. 72, 7473–7476. doi:10.1021/jo701391q
Heinz, B., Djukanovic, D., Filipponi, P., Martin, B., Karaghiosoff, K., Knochel, P., et al. (2021). Regioselective difunctionalization of pyridines via 3, 4-pyridynes. Chem. Sci. 12, 6143–6147. doi:10.1039/d1sc01208h
Magano, J., and Dunetz, J. R. (2011). Large-scale Applications of transition metal-catalyzed couplings for the synthesis of pharmaceuticals. Chem. Rev. 111, 2177–2250. doi:10.1021/cr100346g
Mazzarella, D., Pulcinella, A., Bovy, L., Broersma, R., and Noël, T. (2021). Rapid and direct photocatalytic C(sp3)‒H acylation and arylation in flow. Angew. Chem. Int. Ed. 60, 21277–21282. doi:10.1002/anie.202108987
Miyamura, H., and Kobayashi, S. (2022). Reaction rate acceleration of cooperative catalytic systems: Metal nanoparticles and lewis acids in arene hydrogenation. Angew. Chem. Int. Ed. Engl. 61, e202201203. doi:10.1002/anie.202201203
Murahashi, S., Yamamura, M., Yanagisawa, K., Mita, N., and Kondo, K. (1979). Stereoselective synthesis of alkenes and alkenyl sulfides from alkenyl halides using palladium and ruthenium catalysts. J. Org. Chem. 44, 2408–2417. doi:10.1021/jo01328a016
Nagaki, A., Ashikari, Y., Takumi, M., and Tamaki, T. (2021). Flash chemistry makes impossible organolithium chemistry possible. Chem. Lett. 50, 485–492. doi:10.1246/cl.200837
Nagaki, A., Hirose, K., Tonomura, O., Taniguchi, S., Taga, T., Hasebe, S., et al. (2016). Design of a numbering-up system of monolithic microreactors and its application to synthesis of a key intermediate of valsartan. Org. Process Res. Dev. 20, 687–691. doi:10.1021/acs.oprd.5b00414
Nagaki, A., Kenmoku, A., Moriwaki, Y., Hayashi, A., and Yoshida, J. (2010). Cross-coupling in a flow microreactor: Space integration of lithiation and Murahashi coupling. Angew. Chem. Int. Ed. 49, 7543–7547. doi:10.1002/anie.201002763
Nagaki, A., Moriwaki, Y., Haraki, S., Kenmoku, A., Hayashi, A., and Yoshida, J. (2012b). Cross-coupling of aryllithiums with aryl and vinyl halides in flow microreactors. Chem. Asian J. 7, 1061–1068. doi:10.1002/asia.201101019
Nagaki, A., Moriwaki, Y., and Yoshida, J. (2012a). Flow synthesis of arylboronic esters bearing electrophilic functional groups and space integration with suzuki–miyaura coupling without intentionally added base. Chem. Commun. 48, 11211. doi:10.1039/c2cc36197c
Nagaki, A. (2021). “Multiple organolithium reactions based on space integration,” in Middle molecular strategy: Flow synthesis to functional molecules. Editors K. Fukase, and T. Doi (Singapore: Gateway, SGP, Springer), 309–320.
Nagaki, A., Tomida, Y., Usutani, H., Kim, H., Takabayashi, N., Nokami, T., et al. (2007). Integrated micro flow synthesis based on sequential Br-Li exchange reactions of p-m-and o-dibromobenzenes. Chem. Asian J. 2, 1513–1523. doi:10.1002/asia.200700231
Nicolaou, K. C., Bulger, P. G., and Sarlah, D. (2005). Palladium catalyzed cross-coupling reactions in total synthesis. Angew. Chem. Int. Ed. 44, 4442–4489. doi:10.1002/anie.200500368
Nogami, S., Shida, N., Iguchi, S., Nagasawa, K., Inoue, H., Yamanaka, I., et al. (2022). Mechanistic insights into the electrocatalytic hydrogenation of alkynes on Pt–Pd electrocatalysts in a proton-exchange membrane reactor. ACS Catal. 12, 5430–5440. doi:10.1021/acscatal.2c01594
Novartis (2022). Product sales. Available at: https://www.novartis.com/investors/financial-data/product-sales (Accessed: May 3, 2022).
Roesner, S., and Buchwald, S. L. (2016). Continuous-flow synthesis of biaryls by Negishi cross-coupling of fluoro- and trifluoromethyl-substituted (Hetero)arenes. Angew. Chem. Int. Ed. 55, 10463–10467. doi:10.1002/anie.201605584
Shu, W., Pellegatti, L., Oberli, M. A., and Buchwald, S. L. (2011). Continuous-flow synthesis of biaryls enabled by multistep solid-handling in a lithiation/borylation/suzuki–miyaura cross-coupling sequence. Angew. Chem. Int. Ed. 50, 10665–10669. doi:10.1002/anie.201105223
Snieckus, V. (1990). Directed ortho metalation. Tertiary amide and O-carbamate directors in synthetic strategies for polysubstituted aromatics. Chem. Rev. 90, 879–933. doi:10.1021/cr00104a001
Sugisawa, N., Nakamura, H., and Fuse, S. (2020). Micro-flow synthesis of β-amino acid derivatives via a rapid dual activation approach. Chem. Commun. 56, 4527–4530. doi:10.1039/d0cc01403f
Takahashi, Y., Ashikari, Y., Takumi, M., Shimizu, Y., Jiang, Y., Higuma, R., et al. (2020). Synthesis of biaryls having a piperidylmethyl group based on space integration of lithiation, borylation and suzuki-miyaura coupling. Eur. J. Org. Chem. 5, 618–622. doi:10.1002/ejoc.201901729
Takumi, M., Sakaue, H., and Nagaki, A. (2022). Flash electrochemical approach to carbocations. Angew. Chem. Int. Ed. Engl. 61, e202116177. doi:10.1002/anie.202116177
Usutani, H., Tomida, T., Nagaki, A., Okamoto, H., Nokami, T., Yoshida, J., et al. (2007). Generation and reactions of o-bromophenyllithium without benzyne formation using a microreactor. J. Am. Chem. Soc. 129, 3046–3047. doi:10.1021/ja068330s
Keywords: flow microreactor, negishi coupling, reaction integration, functional group-tolerance, biaryl synthesis
Citation: Ashikari Y, Guan K and Nagaki A (2022) Flash functional group-tolerant biaryl-synthesis based on integration of lithiation, zincation and negishi coupling in flow. Front. Chem. Eng. 4:964767. doi: 10.3389/fceng.2022.964767
Received: 09 June 2022; Accepted: 01 July 2022;
Published: 22 July 2022.
Edited by:
Volker Hessel, University of Adelaide, AustraliaReviewed by:
Julio Pastre, State University of Campinas, BrazilJulien Estager, Centre of Technological Resources in Chemistry (CERTECH), Belgium
Copyright © 2022 Ashikari, Guan and Nagaki. This is an open-access article distributed under the terms of the Creative Commons Attribution License (CC BY). The use, distribution or reproduction in other forums is permitted, provided the original author(s) and the copyright owner(s) are credited and that the original publication in this journal is cited, in accordance with accepted academic practice. No use, distribution or reproduction is permitted which does not comply with these terms.
*Correspondence: Aiichiro Nagaki, nagaki@sci.hokudai.ac.jp