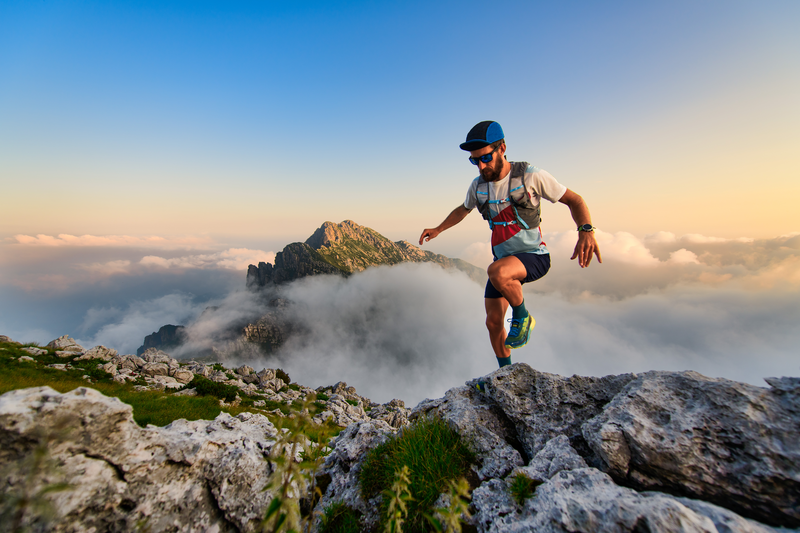
95% of researchers rate our articles as excellent or good
Learn more about the work of our research integrity team to safeguard the quality of each article we publish.
Find out more
EDITORIAL article
Front. Cell Dev. Biol. , 29 January 2025
Sec. Stem Cell Research
Volume 13 - 2025 | https://doi.org/10.3389/fcell.2025.1556403
This article is part of the Research Topic Induced Pluripotent Stem Cells (iPSCs) for Skeletal Muscle Diseases View all 6 articles
Editorial on the Research Topic
Induced pluripotent stem cells (iPSCs) for skeletal muscle diseases
Skeletal muscle is a highly regenerative tissue in the vertebrate body. This skeletal muscle regenerative capacity is mediated by muscle satellite cells (MuSCs), a stem cell population within skeletal muscle (Seale et al., 2001; Asakura et al., 2002). While MuSCs display some non-muscle differentiation potential, such as adipocytes, osteocytes, and fibroblasts in vitro or in some diseased environments (Asakura et al., 2001), their primary differentiation fate in normal muscle regeneration is skeletal muscle cells. MuSCs usually display a mitotically quiescent state and reside on the myofibers. However, during muscle regeneration, MuSCs are activated, exit the quiescent state, and give rise to myogenic precursor cells. After several rounds of cell proliferation, these myogenic precursor cells exit the cell cycle and fuse with each other to contribute to the newly formed myofibers. MuSC-derived myogenic precursor cells or myoblasts expanded ex vivo can be utilized for myoblast-mediated cell therapy for skeletal muscle diseases, including Duchenne muscular dystrophy (DMD) when injected into damaged myofibers via integration into myofibers (Peault et al., 2007; Perie et al., 2014; Skuk and Tremblay, 2015; Negroni et al., 2016). However, there are significant limitations to treatment in humans. The number of MuSCs and myoblasts available from human biopsies is limited. In addition, low cell viability and low contribution of transplanted cells have hindered their practical use in patients (Peault et al., 2007; Skuk and Tremblay, 2015; Negroni et al., 2016).
Human induced pluripotent stem cells (hiPSCs) are a type of pluripotent stem cells originally derived from adult somatic cells. These cells are genetically initialized to an embryonic stem cell (ESC)-like pluripotent state by expressing genes and factors that are essential for maintaining the characteristic properties of pluripotent stem cells. hiPSCs can be generated from human somatic cells from various tissues (Takahashi and Yamanaka, 2006; Takahashi et al., 2007; Chan et al., 2018). They are capable of self-renewal and can give rise to any cell type, including skeletal muscle cells and MuSCs, both in vitro and in vivo. Therefore, hiPSC-derived myogenic cells are a source of myogenic precursor cells that repair injured or diseased or aged skeletal muscle in patients after transplantation (Caron et al., 2023). In addition, the ability to capture the genetic diversity of different inherent muscle diseases and age-related muscle atrophy in an accessible culture system makes hiPSCs an attractive model source for generating myogenic cells for drug screening (Caron et al., 2023).
This Research Topic aims to highlight the progress of research on disease modeling using iPSCs and iPSC-based cell therapy in the field of skeletal muscle diseases. In particular, collecting technical tips for successful induction protocols of iPSC-derived skeletal muscle (Chal et al., 2016; Kodaka et al., 2017; Zhao et al., 2024) suitable for regenerative medicine and in vitro iPSC-based disease modeling is critical for understanding the molecular and cellular mechanisms of disease pathogenesis as well as drug screening.
Five primary research or review manuscripts were published on this Research Topic. In the first manuscript, Hamer and Rossi summarized the protocols to improve the generation, purification, and maturation of iPSC-derived myogenic cells for novel therapeutic applications. Fibro/adipogenic progenitors (FAPs) residing in skeletal muscle play essential roles in skeletal muscle homeostasis and regeneration via secreted molecules and remodeling of extracellular matrix (Molina et al., 2021). In diseased environments, FAPs give rise to adipogenic, osteogenic, or fibroblastic cells that significantly modulate normal muscle function. The second manuscript, by Zhao and Ikeya, summarized the current progress of the developmental origins and functions of FAPs as stromal cells of the muscle connective tissue (MCT) and the FAP-mediated heterotopic ossification causing the rare genetic disorder fibrodysplasia ossificans progressive (FOP) (Nakajima and Ikeya, 2019). In addition, this manuscript provides novel insights into the heterotopic ossification from patient-derived iPSCs. The third manuscript, by Xie et al., uncovered the molecular mechanism by which leukemia inhibitory factor (LIF) promotes the self-renewal and proliferation of MuSCs. Treatment with LIF also enhances the engraftment capability of MuSCs following injection into the skeletal muscle of DMD model mdx mice, suggesting the beneficial effect of LIF for hiPSC-derived MuSC transplantation therapy. The mutations of superoxide dismutase 1 (SOD1) gene have been known as a genetic cause of familial amyotrophic lateral sclerosis (ALS), a progressive neuromuscular disease without cure (Mead et al., 2023). In the fourth manuscript, Couturier et al. reported that hiPSC-derived myogenic cells carrying the SOD1 D90A mutation display a reduction of several myogenic markers, including modulation of nuctic acetylcholine receptor (nAChR) cluster expression and the function, causing the morphological alteration of sarcomeres in myotubes. Therefore, the authors introduced the novel hiPSC-derived neuromuscular model for ALS (Lynch et al., 2019; Zhou et al., 2023). As humans age, changes in the composition of blood circulating factors are thought to contribute to the decline in muscle mass and strength. In the fifth manuscript, Tey et al. reported that supplementation with serum from aged rats significantly reduced cell proliferation, myotube formation, and myofiber maturation, significantly increased cell death, and induced transcriptomic changes in both ESC- and iPSC-derived myogenic cells, providing therapeutic applications to prevent age-related muscle weakness.
This series of five articles highlights the high quality of manuscripts published in the Research Topic of the Stem Cell Research section of Frontiers in Cell and Developmental Biology and reveals a research field that is moving forward at a remarkable pace.
HS: Writing–original draft, Writing–review and editing. MS: Writing–original draft, Writing–review and editing. AA: Writing–original draft, Writing–review and editing.
The author(s) declare that no financial support was received for the research, authorship, and/or publication of this article.
We thank the laboratory members for their helpful comments.
The authors declare that the research was conducted in the absence of any commercial or financial relationships that could be construed as a potential conflict of interest.
The author(s) declared that they were an editorial board member of Frontiers, at the time of submission. This had no impact on the peer review process and the final decision.
The author(s) declare that no Generative AI was used in the creation of this manuscript.
All claims expressed in this article are solely those of the authors and do not necessarily represent those of their affiliated organizations, or those of the publisher, the editors and the reviewers. Any product that may be evaluated in this article, or claim that may be made by its manufacturer, is not guaranteed or endorsed by the publisher.
Asakura, A., Komaki, M., and Rudnicki, M. (2001). Muscle satellite cells are multipotential stem cells that exhibit myogenic, osteogenic, and adipogenic differentiation. Differentiation 68 (4-5), 245–253. doi:10.1046/j.1432-0436.2001.680412.x
Asakura, A., Seale, P., Girgis-Gabardo, A., and Rudnicki, M. A. (2002). Myogenic specification of side population cells in skeletal muscle. J. Cell. Biol. 159 (1), 123–134. doi:10.1083/jcb.200202092
Caron, L., Testa, S., and Magdinier, F. J. (2023). Induced pluripotent stem cells for modeling physiological and pathological striated muscle complexity. Neuromuscul. Dis. 10 (5), 761–776. doi:10.3233/JND-230076
Chal, J., Al Tanoury, Z., Hestin, M., Gobert, B., Aivio, S., Hick, A., et al. (2016). Generation of human muscle fibers and satellite-like cells from human pluripotent stem cells in vitro. Nat. Protoc. 11 (10), 1833–1850. doi:10.1038/nprot.2016.110
Chan, S. S., Arpke, R. W., Filareto, A., Xie, N., Pappas, M. P., Penaloza, J. S., et al. (2018). Skeletal muscle stem cells from PSC-derived Teratomas have functional regenerative capacity. Cell. Stem Cell. 23 (1), 74–85.e6. doi:10.1016/j.stem.2018.06.010
Kodaka, Y., Rabu, G., and Asakura, A. (2017). Skeletal muscle cell induction from pluripotent stem cells. Stem Cells Int. 2017, 1376151. doi:10.1155/2017/1376151
Lynch, E., Semrad, T., Belsito, V. S., FitzGibbons, C., Reilly, M., Hayakawa, K., et al. (2019). C9ORF72-related cellular pathology in skeletal myocytes derived from ALS-patient induced pluripotent stem cells. Dis. Model. Mech. 12 (8), dmm039552. doi:10.1242/dmm.039552
Mead, R. J., Shan, N., Reiser, H. J., Marshall, F., and Shaw, P. J. (2023). Amyotrophic lateral sclerosis:a neurodegenerative disorder poised for successful therapeutic translation. Nat. Rev. Drug Discov. 22 (3), 185–212. doi:10.1038/s41573-022-00612-2
Molina, T., Fabre, P., and Dumont, N. A. (2021). Fibro-adipogenic progenitors in skeletal muscle homeostasis, regeneration and diseases. Open Biol. 11 (12), 210110. doi:10.1098/rsob.210110
Nakajima, T., and Ikeya, M. (2019). Insights into the biology of fibrodysplasia ossificans progressiva using patient-derived induced pluripotent stem cells. Regen. Ther. 11, 25–30. doi:10.1016/j.reth.2019.04.004
Negroni, E., Bigot, A., Butler-Browne, G. S., Trollet, C., and Mouly, V. (2016). Cellular therapies for muscular dystrophies: frustrations and clinical successes. Hum. Gene Ther. 27 (2), 117–126. doi:10.1089/hum.2015.139
Peault, B., Rudnicki, M., Torrente, Y., Cossu, G., Tremblay, J. P., Partridge, T., et al. (2007). Stem and progenitor cells in skeletal muscle development, maintenance, and therapy. Mol. Ther. 15 (5), 867–877. doi:10.1038/mt.sj.6300145
Perie, S., Trollet, C., Mouly, V., Vanneaux, V., Mamchaoui, K., Bouazza, B., et al. (2014). Autologous myoblast transplantation for oculopharyngeal muscular dystrophy: a phase I/IIa clinical study. Mol. Ther. 22 (1), 219–225. doi:10.1038/mt.2013.155
Seale, P., Asakura, A., and Rudnicki, M. A. (2001). The potential of muscle stem cells. Dev. Cell. 1 (3), 333–342. doi:10.1016/S1534-5807(01)00049-1
Skuk, D., and Tremblay, J. P. (2015). Cell therapy in muscular dystrophies: many promises in mice and dogs, few facts in patients. Expert Opin. Biol. Ther. 15 (9), 1307–1319. doi:10.1517/14712598.2015.1057564
Takahashi, K., Tanabe, K., Ohnuki, M., Narita, M., Ichisaka, T., Tomoda, K., et al. (2007). Induction of pluripotent stem cells from adult human fibroblasts by defined factors. Cell. 131 (5), 861–872. doi:10.1016/j.cell.2007.11.019
Takahashi, K., and Yamanaka, S. (2006). Induction of pluripotent stem cells from mouse embryonic and adult fibroblast cultures by defined factors. Cell. 126 (4), 663–676. doi:10.1016/j.cell.2006.07.024
Zhao, M., Taniguchi, Y., Shimono, C., Jonouchi, T., Cheng, Y., Shimizu, Y., et al. (2024). Heparan sulfate chain-conjugated laminin-E8 fragments advance paraxial mesodermal differentiation followed by high myogenic induction from hiPSCs. Adv. Sci. Weinh. 11 (26), e2308306. doi:10.1002/advs.202308306
Keywords: skeletal muscle, muscle stem cell, muscular dystrophgies, aging, IPSC, neuromuscular diseases, amyotrophic lateral sclerosis (ALS), satellite cell
Citation: Sakurai H, Suzuki M and Asakura A (2025) Editorial: Induced pluripotent stem cells (iPSCs) for skeletal muscle diseases. Front. Cell Dev. Biol. 13:1556403. doi: 10.3389/fcell.2025.1556403
Received: 06 January 2025; Accepted: 15 January 2025;
Published: 29 January 2025.
Edited and reviewed by:
Valerie Kouskoff, The University of Manchester, United KingdomCopyright © 2025 Sakurai, Suzuki and Asakura. This is an open-access article distributed under the terms of the Creative Commons Attribution License (CC BY). The use, distribution or reproduction in other forums is permitted, provided the original author(s) and the copyright owner(s) are credited and that the original publication in this journal is cited, in accordance with accepted academic practice. No use, distribution or reproduction is permitted which does not comply with these terms.
*Correspondence: Hidetoshi Sakurai, hsakurai@cira.kyoto-u.ac.jp; Masatoshi Suzuki, masatoshi.suzuki@wisc.edu; Atsushi Asakura, asakura@umn.edu
Disclaimer: All claims expressed in this article are solely those of the authors and do not necessarily represent those of their affiliated organizations, or those of the publisher, the editors and the reviewers. Any product that may be evaluated in this article or claim that may be made by its manufacturer is not guaranteed or endorsed by the publisher.
Research integrity at Frontiers
Learn more about the work of our research integrity team to safeguard the quality of each article we publish.