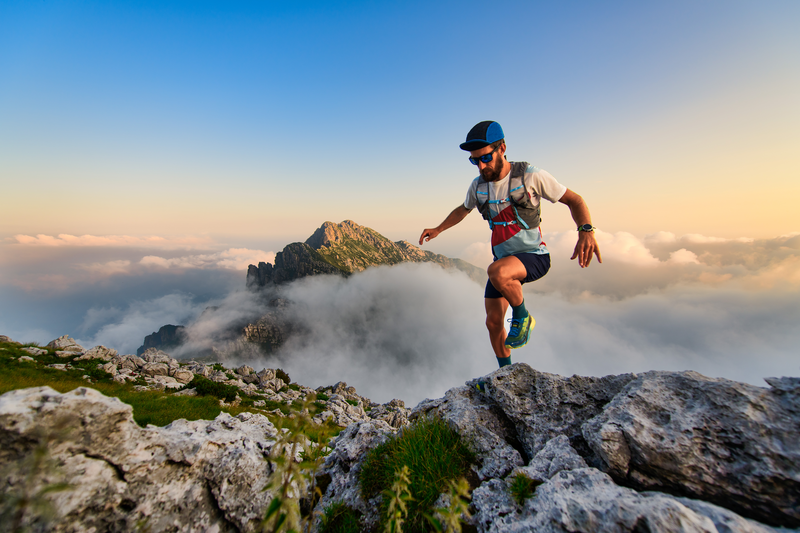
94% of researchers rate our articles as excellent or good
Learn more about the work of our research integrity team to safeguard the quality of each article we publish.
Find out more
ORIGINAL RESEARCH article
Front. Cell Dev. Biol. , 28 June 2023
Sec. Cancer Cell Biology
Volume 11 - 2023 | https://doi.org/10.3389/fcell.2023.1200197
Background: Cervical cancer (CC) remains one of the most common and deadly malignancies in women worldwide. FBXO5, a protein-coding gene, is highly expressed in a variety of primary tumors and promotes tumor progression, however, its role and prognostic value in CC remain largely unknown.
Methods: A key differential gene, FBXO5, was screened according to WGCNA based on immunohistochemical assays of clinical samples, multiple analyses of the Cancer Genome Atlas (TCGA) and Genotype-Tissue Expression (GTEx) databases, including survival analysis, tumor mutational burden, GO, KEGG, tumor immune infiltration, and chemotherapeutic drug sensitivity, to explore the expression and prognostic value of FBXO5 in CC. The migration and invasiveness of cervical cancer cells following FBXO5 knockdown and overexpression were examined using wound healing and transwell assays, and the viability of cancer cells was assessed using CCK8 and EdU assays.
Results: FBXO5 was discovered to be substantially expressed in CC tissues using data from our CC cohort and the TCGA database, and a survival analysis indicated FBXO5 as a predictive factor for poor overall survival in CC patients. In vitro, CC cells were more inclined to proliferate, migrate, and invade when FBXO5 was upregulated as opposed to when it was knocked down.
One of the most common female cancers in the world, cervical cancer can be surgically treated in early-stage patients and has limited efficacy in patients with intermediate to advanced disease, despite combined radiotherapy (Rahangdale et al., 2022; Ahmed et al., 2023). Death is primarily brought on by cancer’s metastasis and progression, giving patients a poor prognosis (Liontos et al., 2019; Coleman et al., 2021). Currently, the prognosis of cervical cancer is mainly assessed by squamous cell carcinoma antigen (SCC), however, this indicator does not change significantly in some patients with recurrence (Markovina et al., 2018; Shi et al., 2023). Finding additional prognostic indicators is, therefore, necessary to improve the prognosis of cervical cancer patients, as SCC alone is insufficient to determine the patient’s prognosis.
F-Box Protein 5 (FBXO5), also known as early mitotic inhibitory factor 1, is highly expressed in glioma, breast cancer, colorectal cancer, and hepatocellular carcinoma, and promotes tumor infiltration and metastasis (Zhao et al., 2013; Chao et al., 2014; Marzio et al., 2019; Wang et al., 2020). FBXO5 acts as a regulator of anaphase-promoting complex/cyclosome (APC/C) activity during the mitotic and meiotic cell cycles regulator, enters the cell cycle by upregulating the expression of FBXO5 mRNA, APC/C is responsible for the repeated elimination of mitotic cyclins to ensure cell division after each round of DNA replication. APC/C ubiquitin ligase destabilizes key regulators at predetermined points in the cycle (Di Fiore and Pines, 2007; Marzio et al., 2019). This leads to the conversion of FBXO5 from an APC/CCDH1 substrate to an inhibitor and the unidirectional inactivation of APC/CCDH1. FBXO5 also interacts with EVI5, which accumulates early in G1 and induces premature degradation of FBXO5, leading to premature activation of APC/C, cell cycle disruption, over-replication of cell centrosomes and ultimately induction of the Plk/SCF pathway via early degradation of FBXO5, thereby disrupting mitosis (Cappell et al., 2018).
A highly conserved lysosomal breakdown system called autophagy is linked to the in vivo upkeep of cellular homeostasis. On the one hand, activated autophagy prevents tumor growth by several methods, including reducing oxidative stress and inhibiting genomic damage. On the other hand, in the face of numerous stress stimuli such as a lack of oxygen, energy, or growth factors, autophagy gives cancer cells the nutrition and energy they need to sustain their fundamental survival and metabolism (Deegan et al., 2013; Dikic and Elazar, 2018). Improving cancer cells’ capacity to survive in a deprived and hypoxic environment during the phase of fast tumor multiplication, especially in the later stages of tumor development promotes tumorigenesis. According to earlier research, autophagy can increase tumor growth by impairing immunity (Amaravadi et al., 2019). Considering that autophagy involves many different cellular processes, the molecular mechanisms of tumor development are extremely complex, and autophagic turbulence is one of the possible causes of tumor formation and development. As autophagy is a sensitive and complex multi-step regulatory process with a high degree of susceptibility, autophagy can be disrupted at any stage, which may lead to the development of the entire pathology (Levine and Kroemer, 2008). A recent study found that silencing genes associated with HPV16 enhanced cellular autophagy and senescence, and also had a significant inhibitory effect on the oncogenic process of cervical cancer. The link between adenosine triphosphatase family protein 3A (ATAD3A), an anti-autophagy factor, and HPV infection has been demonstrated in cervical cancer, where ATAD3A silencing increased the number of autophagic vesicles and significantly reduced drug resistance in cervical cancer cells, further demonstrating the importance of autophagy in HPV-mediated cancer development (Lang et al., 2020). Given the complex role of autophagy in cells, it is important to study how autophagy affects specific types of disease so that lesions can be detected early and effective therapeutic strategies can be developed to curb the development of cervical cancer. Therefore, further study is required to discover whether FBXO5 can speed up the growth of cervical cancer by inducing autophagy (Cui et al., 2019).
In this study, we found that FBXO5 expression was elevated in cervical tissues and that FBXO5 overexpression stimulated autophagy, which in turn promoted the proliferation and migration of cervical cancer cells. According to these findings, FBXO5 may provide a therapeutic target for the treatment of cervical cancer.
FBXO5mRNA data from 306 tumors and 13 adjacent cervical tissues were downloaded from the Cancer Genome Atlas (TCGA) and the Gene Expression Volume Association database (https://gtexportal.org/home, GTEx). Single-cell analysis gene expression data from the Gene Expression Omnibus (GEO) database (http://www.ncbi.nlm.nih.gov/geo/) (GSE168652). For combinational analysis, the FPKM values were then changed to the million per kilobase (TPM) values for the transcripts. To lessen batch effects brought on by deviations outside the realm of biotechnology, Package R’s “SVA” uses the “ComBat” method (Johnson et al., 2007). Relevant clinical data files were downloaded, including tumor depth of infiltration, distant metastases, lymph node staging, survival time and survival status. The GeneCards database provided genes with an association with autophagy (https://www.genecards.org/).
The differentially expressed genes between the high and low FBXO5 groups were found using the R package “Limma” (adjusted p-value 0.01) (Ritchie et al., 2015). Molecular biological differences were taken into account when identifying differential genes in the high and low expression groups (|log2foldchange| > 0.5, adjusted p-value 0.05). For further investigation, their expression in all samples was isolated. To determine the biological functions and potential signaling pathways of FBXO5, the “clusterProfiler” package and the “org.Hs.e.g.db” package of R software were used to screen the relevant genes for GO and KEGG enrichment analysis was performed to explore the biological functions and signaling pathways (Kanehisa and Goto, 2000; Yu et al., 2012).
The ImmuneScore, StromalScore, and EstimateScore were obtained by running the “ESTIMATE” package of the R software (Yoshihara et al., 2013), representing the immune infiltration score, the stromal cell infiltration score, and the composite score, which is a combination of the stromal cell score and the immune cell score, respectively. The composite score is a combination of the stromal and immune cell scores. The “limma” package in the R software was used to classify the FBXO5 gene into high and low-expression groups and to determine whether the level of immune cell infiltration, stromal cell infiltration, and tumor purity differed between the high and low-expression groups, and the associated clinical features (Ritchie et al., 2015).
Somatic cell mutation information for cervical cancer was obtained from the TCGA database. Based on the median level of FBXO5, 306 tumor samples were divided into high and low-expression groups. The relationship between cervical cancer mutations and survival was analyzed by comparing the high and low groups using the “maftools” package (Mayakonda et al., 2018).
The human CC-related cell lines HeLa were purchased from Meisen (Zhejiang, China). Cells were cultured in Dulbecco’s modified Eagle medium (DMEM, Gibco, United States) supplemented with 10% fetal bovine serum (FBS, Gibco, Australia) at 37°C in an environment containing 5% CO2.
FBXO5 knockdown and overexpression lentivirus was purchased from genechem (Shanghai, China) and cells (6 × 10 4 cells/well) were inoculated into 6-well plates and incubated for 24 h after lentiviral infection. Twenty-4 hours after infection, cells were injected with puromycin (3 μg/mL) for 3 weeks to obtain a stably transfected cell line. Overexpression and knockdown efficiency of FBXO5 in CC cell lines was verified using qRT-PCR.
Cells were inoculated at 4×103 cells/well into 96-well plates, with 5 sub-wells per group. 10 μL CCK-8 (Bimake, Houston, Texas) was added to each well at 24, 48, 72, and 96 h respectively. After incubation at 37°C for 2 h, the absorbance values at 450 nm were measured by an enzyme marker.
EdU staining was used to determine the proliferation of cervical cancer cells. Cells were inoculated at 1×105 cells/well into 24-well plates and incubated with EdU (Beyotime, Shanghai, China) for 2 h. Cells were fixed in 4% paraformaldehyde for 10 min at room temperature.
For wound healing assays, the wound gap was recorded at 0, 12, or 24 h after scratching with a 0 μL pipette tip. Transwell assays were examined using an 8 mm well Transwell chamber (Corning, CA, United States).
Total RNA was isolated from the samples using the Promega Total RNA Extraction Kit (Shanghai, China). Using the Transcriptor First Strand cDNA Synthesis Kit (Shanghai, China), we reverse-transcribed total RNA after RNA extraction to create cDNA. SuperReal PreMix Plus from Tiangen Biotech (Beijing, China) was used to determine the expression levels of FBXO5. In Supplementary Table S1, the primer sequences are displayed.
Cells in the logarithmic growth phase were inoculated at 4×105/well in 6-well plates and left overnight. After wall mounting, cells were digested with trypsin. After centrifugation, the cells were fixed in 70% ethanol overnight, centrifuged at 2000 r/min for 5 min before use, 70% ethanol was discarded, washed once with pre-cooled PBS, suspended with 0.5 mL RNase and then bathed in water at 37°C for 30 min, and finally added PI staining for 30 min in water at 37°C, filtered through a 300 mesh sieve and tested on the machine.
The BCA protein concentration assay kit was used to assess the total protein content of CC cells. 20 μg of protein loading volume were electrophoretically separated on 10% SDS polyacrylamide gel, transferred to PVDF membranes, and sealed with TBST solution containing 5% BSA at room temperature. Following that, the membrane was incubated at 4°C for an overnight period with the diluted antibodies LC3, Beclin1, P62, and beta-actin (1:2000, proteintech, Wuhan, China) respectively. The samples were then developed using enhanced chemiluminescence after being re-incubated with goat anti-rabbit IgG antibody secondary antibody (1:5000) at room temperature for 1 h.
Tissue microarrays (HUteS136Su01) of CC specimens were obtained from Shanghai Outdo Biotech Company (http://www.superchip.com.cn/, Shanghai, China). To verify FBXO5 expression in CC. Immunohistochemistry was then performed using staining cycles as shown below. Sections were washed and deparaffinized with xylene, 100% ethanol, 95% ethanol, 80% ethanol, and phosphate buffer, and endogenous peroxidase activity was blocked with 3% hydrogen peroxide. Non-specific antigenic epitopes were blocked by incubation with 10% goat serum in PBS for 1 h at room temperature. Tissue sections were incubated overnight at 4°C with primary monoclonal rabbit anti-FBXO5 antibody at a dilution of 1:500 and then incubated for 1 h at room temperature with horseradish peroxidase-conjugated goat anti-rabbit secondary antibody. A total of 110 samples were collected by removing incomplete tissue sections and samples that lacked clinical information. Staining intensity score: unstained 0, light yellow 1, light brown 2, dark brown 3. The proportion of positive staining cells: less than 5% of positive cells count 0 points, 5% -25% of positive cells count 1 point, 25% -50% of positive cells count 2 points, and ≥50% of positive cells count 3 points. Each section was randomly selected to observe different representative areas, and a total of 5 fields of view were observed under high magnification. The total score was obtained by multiplying the staining intensity with the percentage of positive cells, and the average was taken. A total score greater than or equal to 3 is classified as a high expression, and a total score less than 3 is classified as a low expression.
The statistical analysis was performed using the R program (version 4.1.3). Statistical significance was defined as a two-tailed p-value of 0.05.
The genes associated with autophagy were correlated with the phenotypes using the WGCNA module, which demonstrated that the genes with extremely low expression were eliminated and the values satisfied the screening criterion at an ideal threshold of 10. The Pink module revealed the most significant link with “Tumor” (p = 6e-25, r = 0.54) and six out of the twelve modules had statistically significant differences (p < 0.05). With “Tumor” in the pink module (Figures 1A–C; Supplementary Figrues S1A, B). The “Pink” module’s PPI protein interaction network was built using the STRING online database, and the significant gene PDIA3 was obtained by excluding the autophagy-related genes previously demonstrated to be connected with cervical cancer patients’ prognosis (Figure 1D).
FIGURE 1. Identification of key modules of autophagy-related genes. (A) Gene clustering associated with autophagy-related genes. (B) Heatmap shows the relationship between gene modules and the normal/tumor type. Gene clustering associated with autophagy. (C) Correlation between the chosen module’s membership and gene importance. (D) Correlation between gene importance and the membership of the chosen module.
In cervical cancer tissues compared to normal tissues, FBXO5 expression was higher (p < 0.001) (Figure 2A). Based on median FBXO5 expression, patients were separated into high and low-expression groups, the high-expression group had worse survival rates (p = 0.017 Figure 2B). FBXO5 was more broadly distributed in malignant cells, according to a single-cell analysis of expression in the tumor microenvironment, which included immune cells, stromal cells, malignant cells, and functional cells (Figure 2C). Lymph node staging and morphology are used clinically to create a nomogram, which can be used to forecast a patient’s prognosis more specifically (Figures 2D, E). Except renal papillary cell carcinoma, pheochromocytoma, paraganglioma, and prostate adenocarcinoma, FBXO5 was expressed in a higher range of malignant tumors (Figure 2F). We explored the possible mechanisms of FBXO5 in cervical cancer through Gene Ontology (GO), Kyoto Encyclopedia of Genes and Genomes (KEGG) and Gene Set Enrichment Analysis (GESA). GO and KEGG revealed biological processes focused on signaling actions such as cell-cell adhesion via plasma-membrane adhesion molecules, synaptic membrane and regulation of endopeptidase activity (Figures 2G, H), and GESA enrichment in cell cycle and PPAR signaling pathways (Figure 2I).
FIGURE 2. Expression of FBXO5 in cancer (A) Expression of FBXO5 in cervical cancer and normal tissues. (B) Analysis of the relationship between FBXO5 and overall survival (OS) of cervical cancer patients in the TCGA dataset. (C) UMAP visualization of FBXO5 in significant cervical cancer cell subpopulations (GSE168652). (D) The nomogram was constructed using risk factors. (E) Calibration plot of a nomogram for predicting overall survival in CC patients at 1 year, 3 years, and 5 years. (F) Pan-cancer FBXO5 expression. (G) Bar graph of GO enrichment of FBXO5. (H) Bar graph of KEGG enrichment of FBXO5. (I) Enrichment of GESA pathway of FBXO5.
Immunohistochemistry was performed on 135 cervical tumors and matched para-cancer samples to further establish the degree of FBXO5 expression in cervical cancer (Figure 3D). In concordance with the TCGA findings, the comparison results showed that FBXO5 was strongly expressed in cancer tissues and differently expressed in paraneoplastic samples (Figure 3A). Immunohistochemistry was used to classify the patients into two groups: high expression (n = 54) and low expression (n = 56). The expression level of the FBXO5 protein linked with the patient’s pathological grade (p = 0.035) and N stage (p = 0.048), although clinical variables such as pathologic stage, age, and morphology did not (p > 0.05, Table 1), according to a correlation analysis of clinical data. Patients with high FBXO5 expression had shorter overall survival and progression-free survival (PFS) than those with low expression, with a statistically significant difference (p < 0.001, p = 0.001), using the Kaplan-Meier method for survival prognosis analysis of cervical cancer follow-up data (Figures 3B, C).
FIGURE 3. Expression of FBXO5 in our CC cohort (A) FBXO5 is differentially expressed in cervical cancer and normal tissue. (B) Analysis of the relationship between FBXO5 and overall survival (OS) of cervical cancer patients. (C) Analysis of the relationship between FBXO5 and progression-free survival (PFS) of cervical cancer patients. (D) FBXO5 protein expression in normal cervical tissue samples and CC tissue samples.
The overall mutations in the groups with low and high FBXO5 expression are shown in Supplementary Figures S1A, B. In addition, the majority of mutant genes communicate with one another (Supplementary Figures S1C, D). In both groups, the genes TTN, PIK3CA, KMT2C, and MUC16 were more frequently mutated. The mutation rate was higher in the FBXO5 high-expression patients, the PIK3CA gene was mutated in more patients in this group, and TTN was the most abundant mutated gene (Figures 4A–D). The prognosis of the high tumor mutational burden (TMB) score group was worse than that of the low score group, and the prognosis of patients in the high TMB score group combined with high FBXO5 expression was inferior to that of patients in the low TMB score group combined with low FBXO5 expression, according to a survival analysis using Kaplan-Meier curves (Figures 4E, F). The mutation analysis of FBXO5 in pan-cancer is shown in Figure 4G, with cervical cancer showing the majority of mutation and deep deletion.
FIGURE 4. Mutational analysis (A, C) FBXO5 high expression in patients with mutations. (B, D) FBXO5 low expression in patients with mutations. (E) The Kaplan-Meier curves for the groups with low and high TMB are shown. (F) The Kaplan-Meier analysis curves for the patients were divided into groups based on TMB and FBXO5 expression. (G) Analysis of the FBXO5 mutation in pancytopenia.
FBXO5 is associated with a variety of immune cells in cervical cancer, including Th2 cells, T helper cells, cytotoxic cells, dendritic cells, and neutrophils (Figure 5A). The FBXO5 high expression group had different ImmuneScore and ESTIMATEScore results from the low expression group (p < 0.01), and the high expression group performed lower on the tumor immune microenvironment (TME) score than the low expression group (Figure 5B). The relationship between FBXO5 and immunomodulators (chemokines, receptors, MHCs, immunostimulants, and checkpoints) was analyzed in order to further understand the impact of FBXO5 on tumor immunity (Figures 5C–G).
FIGURE 5. The relationship between immune infiltration and FBXO5. (A) Analysis of the correlation between FBXO5 and immune cells. (B) Differential analysis of tumor microenvironment scores between the FBXO5 high and low expression groups. (C–G) The relationship between FBXO5 and immunomodulators: immunostimulants (C), checkpoints (D), chemokines (E), receptors (F) and MHCs (G).
In order to evaluate the value of FBXO5 for chemotherapeutic effectiveness, we examined the difference in sensitivity to frequently used chemotherapeutic drugs in CC between the high and low expression groups. Patients with high FBXO5 expression were less responsive to Rapamycin and Pazopanib and more sensitive to (5Z)-7-Oxozeaenol, Cetuximab, Nutlin-3a, and Erlotinib (Figures 6A–F).
FIGURE 6. Differences in immunomodulatory drug sensitivity. (A–F) Differences in immunomodulatory drug sensitivity between the FBXO5 high and low expression groups. (5Z)-7-Oxozeaenol (A), Cetuximab (B), Nutlin-3a (C), Erlotinib (D), Rapamycin(E) and Pazopanib (F).
We transfected FBXO5 overexpression and knockdown virus in Hela and used qRT-PCR to assess the efficacy of FBXO5 transfection in order to better research the function of FBXO5 in cervical cancer (Supplementary Figures S1E, F; Table 1). As seen in the image, sh FBXO5 transfected cancer cells exhibited much less capacity to invade and migrate than the control (Figures 7A, B). Consistent with the transwell assays results, the ability of sh FBXO5-transfected cancer cells to migrate was inhibited in wound healing assays (Figures 7C, D). The CCK8 assay results demonstrated that FBXO5 knocked down cells drastically reduced the viability and proliferation of Hela cells (Figure 7E). The FBXO5 overexpression group had noticeably more cells in a proliferative state than the knockdown group, according to the EdU assay (Figure 7F). To assess the effect of FBXO5 on autophagy in cervical cancer, we examined the autophagy protein expression of the cells. According to the data, cells overexpressing FBXO5 had higher levels of Bcl-1 and LC3 and lower levels of P62 expression when compared to the control group (Figure 7G). While the proportion of G2/M + S phase cells increased in the FBXO5 group compared to the control group at a highly significant level, the number of G0/G1 phase cells was significantly lower in the transfected group than the control group (Figure 7H). In parallel, the opposing effect of FBXO5 gene knockdown on the cell cycle was confirmed (Figure 7I), demonstrating that the expression of FBXO5 would encourage cervical cancer cells to be halted in the G2/M + S phase.
FIGURE 7. FBXO5 promotes cervical cancer cells’ invasion and migration. (A, B) Transwell assays were used to evaluate the ability of HeLa cells to invade and migrate after FBXO5 was overexpressed (A) and knocked down (B) for 48 h (C, D) Wound healing assays were used to evaluate the ability of HeLa cells to invade and migrate after FBXO5 was overexpressed (E) and knocked down (D) for 72 h. (A) According to the CCK-8 assay, FBXO5 upregulation encouraged Hela growth while FBXO5 downregulation prevented it. (F) Percentage of Hela positive cells for EdU staining (×100). (G) Western blot was used to assess the levels of Bcl-1, LC3, and p62 protein expression in cells. (H) Detection of cell cycles in cervical cancer cells transfected with FBXO5 (+) virus. (I) Detection of cell cycles in cervical cancer cells transfected with sh FBXO5 virus.
One of the most prevalent cancers in women, cervical cancer poses a significant risk to one’s health in terms of incidence and mortality (Sung et al., 2021). Autophagy plays a significant part in the development of cervical cancer, just like it does in other tumors (Wu et al., 2019; You et al., 2019; Chen et al., 2022). Histones, which activate autophagy in cervical cancer cells, or chemicals, which support the body’s immune response by facilitating macrophage phagocytosis through autophagy, both increase cancer growth (Orfanelli et al., 2014; Yang et al., 2021; Sun et al., 2022). More and more autophagy-related genes have been found to affect the growth and development of CC (Mattoscio et al., 2018; Yang et al., 2021). We discovered FBXO5, a crucial cervical cancer gene, using the WGCNA method, and discovered for the first time that FBXO5 can affect the growth of cervical cancer by enhancing autophagy. We discovered that FBXO5 expression was elevated in cervical cancer and adversely linked with patient prognosis using public data and our own cohort analysis. Moreover, we discovered that a nomogram built from FBXO5 expression specifically predicted patient survival. Patients’ prognoses were also improved by higher TMB and FBXO5 expression. According to previous research, patients with a higher TMB have a stronger capacity to produce neoantigens and a larger tumor immunogenic response, which makes them more likely to respond favorably to immunotherapy (Galvano et al., 2021; Zheng et al., 2022). FBXO5 may be used as a prognostic indicator for tumor treatment in patients with CC by targeted therapy.
Many studies have previously found connections between the cell cycle and autophagy. For instance, the most important kinase in the cell cycle, CDK1, is phosphorylated to control the initiation protein ULK1/ATG13, which in turn promotes mitotic autophagy and cell cycle progression (Li et al., 2020).
The FBXO5 gene was first discovered in extracts from the African clawed toad as a cell cycle protein that prevents the anaphase promoting complex (APC) from being activated by binding to cytokinesis cycle proteins, accumulating cytokine B, and advancing mitosis into M phase (Min et al., 2013; Zhao et al., 2013) As a critical regulator of mitosis, FBXO5 prevents APC ubiquitination and encourages mitosis into the S phase. Aberrant FBXO5 expression affects chromosome stability and regular cell division (Margottin-Goguet et al., 2003). In contrast, polyploidy production and chromosome instability are significant contributors to carcinogenesis and significant markers of malignancies, while normal cell division and chromosome stability are key requirements for the maintenance of normal bodily function (Liu et al., 2013). Biological functional enrichment analysis results also suggest that FBXO5 is involved in cell cycle roles in patients with cervical cancer.
An analysis between FBXO5 and immune infiltration was used to determine whether FBXO5 can be used as a target for immunotherapy in patients with cervical cancer. The risk FBXO5 was negatively correlated with TME scores, indicating that patients with fewer side effects by targeting this molecule have a stronger anti-tumor immune response, a greater potential to benefit from immunotherapy, and a longer survival time (Ji et al., 2012; Sanmamed and Chen, 2018). Many patients with malignant tumors now have hope of survival thanks to tumor immunotherapy, which uses immunological checkpoints as its focal site of action and extends survival times for patients with advanced stages (Brower, 2015). As tumors develop, several components of the innate and adaptive immune system in the tumor microenvironment are immunosuppressive, contributing to immune evasion of the tumor and resistance to checkpoint inhibitors. Several immunological checkpoints were negatively connected with FBXO5, which was also highly correlated with its closely related T-cells (Fan et al., 2021; Gao et al., 2023). The effector T-cells encourage tumor cell death, and their release of lysis factors is essential for the development of anti-tumor immunity (Jiang et al., 2018). Immune checkpoints will mount an anti-cancer immune response at an early stage to avoid overaction in the early stages of cancer, but this allows cancer to escape the immune system (Li et al., 2023). By utilizing targeted therapy in the early stages and combining it with the appropriate chemotherapeutic agents, we can stimulate the body’s immune system and increase the number of patients who benefit from cervical cancer treatment options.
By comparing our cohort with data from three public databases, this study was the first to validate the expression levels of FBXO5 in CC and their effect on patient prognosis. As a result, it might develop into a stand-alone predictive risk factor and be used as a novel marker for the clinical diagnosis and prognosis of cervical cancer, supporting doctors in developing more patient-centered treatment regimens. In the future, additional long-term follow-up studies will be needed, along with the validation of our research utilizing a large number of normal samples and tumor samples. The results of this study also demand further investigation, such as animal and cell investigations, in order to more thoroughly validate the mechanism of FBXO5 promoting CC.
Cell cycle-related gene FBXO5 induces autophagy in cervical cancer cells and is substantially expressed in CC. It is also gradually linked to poor patient outcomes. Our research fills in the gap regarding FBXO5 in cervical cancer and points us that CC patients may be able to use it as a possible predictive biomarker.
Publicly available datasets were analyzed in this study. This data can be found here: https://portal.gdc.cancer.gov/ https://www.ncbi.nlm.nih.gov/geo/.
YS conceived, designed, and supervised the study. SJ analyzed the data, conducted experiments, and drafted the manuscript. JZ and ZC collected the data. QW, XC, and CZ arranged figures. YL revised the manuscript and carried out response to comments from reviewers. All authors contributed to the article and approved the submitted version.
This work was supported by the National Natural Science Foundation of China (81873045), Joint Funds for the Innovation of Science and Technology of Fujian Province (2021Y9209) and the High-level Talents Training Project of Fujian Cancer Hospital (2022YNG04).
We are grateful for the fund.
The authors declare that the research was conducted in the absence of any commercial or financial relationships that could be construed as a potential conflict of interest.
All claims expressed in this article are solely those of the authors and do not necessarily represent those of their affiliated organizations, or those of the publisher, the editors and the reviewers. Any product that may be evaluated in this article, or claim that may be made by its manufacturer, is not guaranteed or endorsed by the publisher.
The Supplementary Material for this article can be found online at: https://www.frontiersin.org/articles/10.3389/fcell.2023.1200197/full#supplementary-material
APC, anaphase promoting complex; APC/C, anaphase-promoting complex/cyclosome; CC, cervical cancer; FBXO5, F-Box Protein 5; GEO, The Gene Expression Omnibus; GO, Gene Ontology; GSEA, Gene Set Enrichment Analysis; GTEx, Genotype-Tissue Expression; K- M, Kaplan-Meier; KEGG, Kyoto Encyclopedia of Genes and Genomes; OS, overall survival; PI, propidium iodide; SCC, squamous cell carcinoma antigen; TCGA, The Tumor Genome Atlas; TMB, tumor mutational burden; TME, Tumor microenvironment.
Ahmed, W., Zaib, S., Ullah, S., Fatima, A., Zaib, Z., Haseeb Azam, M. A., et al. (2023). Role of human papillomavirus in various cancers: Epidemiology, screening and prevention. Mini Rev. Med. Chem. 23, 1079–1089. doi:10.2174/1389557523666230213140641
Amaravadi, R. K., Kimmelman, A. C., and Debnath, J. (2019). Targeting autophagy in cancer: Recent advances and future directions. Cancer Discov. 9 (9), 1167–1181. doi:10.1158/2159-8290.Cd-19-0292
Brower, V. (2015). Checkpoint blockade immunotherapy for cancer comes of age. J. Natl. Cancer Inst. 107 (3), djv069. doi:10.1093/jnci/djv069
Cappell, S. D., Mark, K. G., Garbett, D., Pack, L. R., Rape, M., and Meyer, T. (2018). EMI1 switches from being a substrate to an inhibitor of APC/C(CDH1) to start the cell cycle. Nature 558 (7709), 313–317. doi:10.1038/s41586-018-0199-7
Chao, T. C., Chen, K. J., Tang, M. C., Chan, L. C., Chen, P. M., Tzeng, C. H., et al. (2014). Thymosin beta-4 knockdown in IEC-6 normal intestinal epithelial cells induces DNA re-replication via downregulating Emi1. J. Cell Physiol. 229 (11), 1639–1646. doi:10.1002/jcp.24609
Chen, Y., Cui, Z., Wu, Q., Wang, H., Xia, H., and Sun, Y. (2022). Long non-coding RNA HOXA11-AS knockout inhibits proliferation and overcomes drug resistance in ovarian cancer. Bioengineered 13 (5), 13893–13905. doi:10.1080/21655979.2022.2086377
Coleman, R. L., Lorusso, D., Gennigens, C., González-Martín, A., Randall, L., Cibula, D., et al. (2021). Efficacy and safety of tisotumab vedotin in previously treated recurrent or metastatic cervical cancer (innovaTV 204/GOG-3023/ENGOT-cx6): A multicentre, open-label, single-arm, phase 2 study. Lancet Oncol. 22 (5), 609–619. doi:10.1016/s1470-2045(21)00056-5
Cui, B., Lin, H., Yu, J., Yu, J., and Hu, Z. (2019). Autophagy and the immune response. Adv. Exp. Med. Biol. 1206, 595–634. doi:10.1007/978-981-15-0602-4_27
Deegan, S., Saveljeva, S., Gorman, A. M., and Samali, A. (2013). Stress-induced self-cannibalism: On the regulation of autophagy by endoplasmic reticulum stress. Cell Mol. Life Sci. 70 (14), 2425–2441. doi:10.1007/s00018-012-1173-4
Di Fiore, B., and Pines, J. (2007). Emi1 is needed to couple DNA replication with mitosis but does not regulate activation of the mitotic APC/C. J. Cell Biol. 177 (3), 425–437. doi:10.1083/jcb.200611166
Dikic, I., and Elazar, Z. (2018). Mechanism and medical implications of mammalian autophagy. Nat. Rev. Mol. Cell Biol. 19 (6), 349–364. doi:10.1038/s41580-018-0003-4
Fan, Y., Geng, Y., Shen, L., and Zhang, Z. (2021). Advances on immune-related adverse events associated with immune checkpoint inhibitors. Front. Med. 15 (1), 33–42. doi:10.1007/s11684-019-0735-3
Galvano, A., Gristina, V., Malapelle, U., Pisapia, P., Pepe, F., Barraco, N., et al. (2021). The prognostic impact of tumor mutational burden (TMB) in the first-line management of advanced non-oncogene addicted non-small-cell lung cancer (NSCLC): A systematic review and meta-analysis of randomized controlled trials. ESMO Open 6 (3), 100124. doi:10.1016/j.esmoop.2021.100124
Gao, Z., Bai, Y., Lin, A., Jiang, A., Zhou, C., Cheng, Q., et al. (2023). Gamma delta T-cell-based immune checkpoint therapy: Attractive candidate for antitumor treatment. Mol. Cancer 22 (1), 31. doi:10.1186/s12943-023-01722-0
Ji, R. R., Chasalow, S. D., Wang, L., Hamid, O., Schmidt, H., Cogswell, J., et al. (2012). An immune-active tumor microenvironment favors clinical response to ipilimumab. Cancer Immunol. Immunother. 61 (7), 1019–1031. doi:10.1007/s00262-011-1172-6
Jiang, P., Gu, S., Pan, D., Fu, J., Sahu, A., Hu, X., et al. (2018). Signatures of T cell dysfunction and exclusion predict cancer immunotherapy response. Nat. Med. 24 (10), 1550–1558. doi:10.1038/s41591-018-0136-1
Johnson, W. E., Li, C., and Rabinovic, A. (2007). Adjusting batch effects in microarray expression data using empirical Bayes methods. Biostatistics 8 (1), 118–127. doi:10.1093/biostatistics/kxj037
Kanehisa, M., and Goto, S. (2000). Kegg: Kyoto encyclopedia of genes and genomes. Nucleic Acids Res. 28 (1), 27–30. doi:10.1093/nar/28.1.27
Lang, L., Loveless, R., and Teng, Y. (2020). Emerging links between control of mitochondrial protein ATAD3A and cancer. Int. J. Mol. Sci. 21 (21), 7917. doi:10.3390/ijms21217917
Levine, B., and Kroemer, G. (2008). Autophagy in the pathogenesis of disease. Cell 132 (1), 27–42. doi:10.1016/j.cell.2007.12.018
Li, G., Choi, J. E., Kryczek, I., Sun, Y., Liao, P., Li, S., et al. (2023). Intersection of immune and oncometabolic pathways drives cancer hyperprogression during immunotherapy. Cancer Cell 41 (2), 304–322.e7. doi:10.1016/j.ccell.2022.12.008
Li, Z., Tian, X., Ji, X., Wang, J., Chen, H., Wang, D., et al. (2020). ULK1-ATG13 and their mitotic phospho-regulation by CDK1 connect autophagy to cell cycle. PLoS Biol. 18 (6), e3000288. doi:10.1371/journal.pbio.3000288
Liontos, M., Kyriazoglou, A., Dimitriadis, I., Dimopoulos, M. A., and Bamias, A. (2019). Systemic therapy in cervical cancer: 30 years in review. Crit. Rev. Oncol. Hematol. 137, 9–17. doi:10.1016/j.critrevonc.2019.02.009
Liu, X., Wang, H., Ma, J., Xu, J., Sheng, C., Yang, S., et al. (2013). The expression and prognosis of Emi1 and Skp2 in breast carcinoma: Associated with PI3K/akt pathway and cell proliferation. Med. Oncol. 30 (4), 735. doi:10.1007/s12032-013-0735-0
Margottin-Goguet, F., Hsu, J. Y., Loktev, A., Hsieh, H. M., Reimann, J. D., and Jackson, P. K. (2003). Prophase destruction of Emi1 by the SCF(betaTrCP/Slimb) ubiquitin ligase activates the anaphase promoting complex to allow progression beyond prometaphase. Dev. Cell 4 (6), 813–826. doi:10.1016/s1534-5807(03)00153-9
Markovina, S., Wang, S., Henke, L. E., Luke, C. J., Pak, S. C., DeWees, T., et al. (2018). Serum squamous cell carcinoma antigen as an early indicator of response during therapy of cervical cancer. Br. J. Cancer 118 (1), 72–78. doi:10.1038/bjc.2017.390
Marzio, A., Puccini, J., Kwon, Y., Maverakis, N. K., Arbini, A., Sung, P., et al. (2019). The F-box domain-dependent activity of EMI1 regulates PARPi sensitivity in triple-negative breast cancers. Mol. Cell 73 (2), 224–237. doi:10.1016/j.molcel.2018.11.003
Mattoscio, D., Medda, A., and Chiocca, S. (2018). Human papilloma virus and autophagy. Int. J. Mol. Sci. 19 (6), 1775. doi:10.3390/ijms19061775
Mayakonda, A., Lin, D. C., Assenov, Y., Plass, C., and Koeffler, H. P. (2018). Maftools: Efficient and comprehensive analysis of somatic variants in cancer. Genome Res. 28 (11), 1747–1756. doi:10.1101/gr.239244.118
Min, K. W., Park, M. H., Hong, S. R., Lee, H., Kwon, S. Y., Hong, S. H., et al. (2013). Clear cell carcinomas of the ovary: A multi-institutional study of 129 cases in korea with prognostic significance of Emi1 and galectin-3. Int. J. Gynecol. Pathol. 32 (1), 3–14. doi:10.1097/PGP.0b013e31825554e9
Orfanelli, T., Jeong, J. M., Doulaveris, G., Holcomb, K., and Witkin, S. S. (2014). Involvement of autophagy in cervical, endometrial and ovarian cancer. Int. J. Cancer 135 (3), 519–528. doi:10.1002/ijc.28524
Rahangdale, L., Mungo, C., O'Connor, S., Chibwesha, C. J., and Brewer, N. T. (2022). Human papillomavirus vaccination and cervical cancer risk. Bmj 379, e070115. doi:10.1136/bmj-2022-070115
Ritchie, M. E., Phipson, B., Wu, D., Hu, Y., Law, C. W., Shi, W., et al. (2015). Limma powers differential expression analyses for RNA-sequencing and microarray studies. Nucleic Acids Res. 43 (7), e47. doi:10.1093/nar/gkv007
Sanmamed, M. F., and Chen, L. (2018). A paradigm shift in cancer immunotherapy: From enhancement to normalization. Cell 175 (2), 313–326. doi:10.1016/j.cell.2018.09.035
Shi, L., Liu, Y., Li, J., Kou, J., Ouyang, Y., Chen, F., et al. (2023). Establishment of a risk stratification model based on the combination of post-treatment serum squamous cell carcinoma antigen levels and FIGO stage of cervical cancer for treatment and surveillance decision-making. J. Cancer Res. Clin. Oncol. doi:10.1007/s00432-022-04558-1
Sun, X., Shu, Y., Ye, G., Wu, C., Xu, M., Gao, R., et al. (2022). Histone deacetylase inhibitors inhibit cervical cancer growth through Parkin acetylation-mediated mitophagy. Acta Pharm. Sin. B 12 (2), 838–852. doi:10.1016/j.apsb.2021.07.003
Sung, H., Ferlay, J., Siegel, R. L., Laversanne, M., Soerjomataram, I., Jemal, A., et al. (2021). Global cancer statistics 2020: GLOBOCAN estimates of incidence and mortality worldwide for 36 cancers in 185 countries. CA Cancer J. Clin. 71 (3), 209–249. doi:10.3322/caac.21660
Wang, M., Yang, C., Liu, X., Zheng, J., Xue, Y., Ruan, X., et al. (2020). An upstream open reading frame regulates vasculogenic mimicry of glioma via ZNRD1-AS1/miR-499a-5p/ELF1/EMI1 pathway. J. Cell Mol. Med. 24 (11), 6120–6136. doi:10.1111/jcmm.15217
Wu, H., Lu, X. X., Wang, J. R., Yang, T. Y., Li, X. M., He, X. S., et al. (2019). TRAF6 inhibits colorectal cancer metastasis through regulating selective autophagic CTNNB1/β-catenin degradation and is targeted for GSK3B/GSK3β-mediated phosphorylation and degradation. Autophagy 15 (9), 1506–1522. doi:10.1080/15548627.2019.1586250
Yang, S. L., Tan, H. X., Niu, T. T., Liu, Y. K., Gu, C. J., Li, D. J., et al. (2021). The IFN-γ-Ido1-kynureine pathway-induced autophagy in cervical cancer cell promotes phagocytosis of macrophage. Int. J. Biol. Sci. 17 (1), 339–352. doi:10.7150/ijbs.51241
Yoshihara, K., Shahmoradgoli, M., Martínez, E., Vegesna, R., Kim, H., Torres-Garcia, W., et al. (2013). Inferring tumour purity and stromal and immune cell admixture from expression data. Nat. Commun. 4, 2612. doi:10.1038/ncomms3612
You, Z., Xu, Y., Wan, W., Zhou, L., Li, J., Zhou, T., et al. (2019). TP53INP2 contributes to autophagosome formation by promoting LC3-ATG7 interaction. Autophagy 15 (8), 1309–1321. doi:10.1080/15548627.2019.1580510
Yu, G., Wang, L. G., Han, Y., and He, Q. Y. (2012). clusterProfiler: an R package for comparing biological themes among gene clusters. Omics 16 (5), 284–287. doi:10.1089/omi.2011.0118
Zhao, Y., Tang, Q., Ni, R., Huang, X., Wang, Y., Lu, C., et al. (2013). Early mitotic inhibitor-1, an anaphase-promoting complex/cyclosome inhibitor, can control tumor cell proliferation in hepatocellular carcinoma: Correlation with Skp2 stability and degradation of p27(kip1). Hum. Pathol. 44 (3), 365–373. doi:10.1016/j.humpath.2012.03.030
Zheng, J., Shao, M., Yang, W., Ren, J., Chen, X., and Yang, H. (2022). Benefits of combination therapy with immune checkpoint inhibitors and predictive role of tumour mutation burden in hepatocellular carcinoma: A systematic review and meta-analysis. Int. Immunopharmacol. 112, 109244. doi:10.1016/j.intimp.2022.109244
Keywords: WGCNA, autophagy, cervical cancer, prognosis, immunity
Citation: Jiang S, Zheng J, Cui Z, Li Y, Wu Q, Cai X, Zheng C and Sun Y (2023) FBXO5 acts as a novel prognostic biomarker for patients with cervical cancer. Front. Cell Dev. Biol. 11:1200197. doi: 10.3389/fcell.2023.1200197
Received: 04 April 2023; Accepted: 06 June 2023;
Published: 28 June 2023.
Edited by:
Anna M. Eiring, Texas Tech University Health Sciences Center El Paso, United StatesReviewed by:
Yiran Li, Tongji University, ChinaCopyright © 2023 Jiang, Zheng, Cui, Li, Wu, Cai, Zheng and Sun. This is an open-access article distributed under the terms of the Creative Commons Attribution License (CC BY). The use, distribution or reproduction in other forums is permitted, provided the original author(s) and the copyright owner(s) are credited and that the original publication in this journal is cited, in accordance with accepted academic practice. No use, distribution or reproduction is permitted which does not comply with these terms.
*Correspondence: Yang Sun, c3VueWFuZ0BmanpsaG9zcGl0YWwuY29t
Disclaimer: All claims expressed in this article are solely those of the authors and do not necessarily represent those of their affiliated organizations, or those of the publisher, the editors and the reviewers. Any product that may be evaluated in this article or claim that may be made by its manufacturer is not guaranteed or endorsed by the publisher.
Research integrity at Frontiers
Learn more about the work of our research integrity team to safeguard the quality of each article we publish.