- 1Department of Rehabilitation Medicine, The Second Affiliated Hospital, Nanchang University, Nanchang, Jiangxi, China
- 2Department of Gastrointestinal Surgery, The Second Affiliated Hospital, Nanchang University, Nanchang, Jiangxi, China
- 3Department of Physical Examination, The Second Affiliated Hospital, Nanchang University, Nanchang, Jiangxi, China
Damage to the nervous system can lead to functional impairment, including sensory and motor functions. Importantly, neuropathic pain (NPP) can be induced after nerve injury, which seriously affects the quality of life of patients. Therefore, the repair of nerve damage and the treatment of pain are particularly important. However, the current treatment of NPP is very weak, which promotes researchers to find new methods and directions for treatment. Recently, cell transplantation technology has received great attention and has become a hot spot for the treatment of nerve injury and pain. Olfactory ensheathing cells (OECs) are a kind of glial cells with the characteristics of lifelong survival in the nervous system and continuous division and renewal. They also secrete a variety of neurotrophic factors, bridge the fibers at both ends of the injured nerve, change the local injury microenvironment, and promote axon regeneration and other biological functions. Different studies have revealed that the transplantation of OECs can repair damaged nerves and exert analgesic effect. Some progress has been made in the effect of OECs transplantation in inhibiting NPP. Therefore, in this paper, we provided a comprehensive overview of the biology of OECs, described the possible pathogenesis of NPP. Moreover, we discussed on the therapeutic effect of OECs transplantation on central nervous system injury and NPP, and prospected some possible problems of OECs transplantation as pain treatment. To provide some valuable information for the treatment of pain by OECs transplantation in the future.
1 Introduction
Nervous system encounters noxious stimuli (such as inflammation, trauma, mechanical and immune), which can lead to different degrees of nerve damage and induce neuropathic pain (Sato et al., 2014; Philpott et al., 2017; Lovaglio et al., 2019). NPP is a kind of chronic pain, which can persist with a variety of diseases. It has the same manifestation with other types of chronic pain (cancer pain and inflammatory pain), mainly expressed as abnormal pain, hyperalgesia and spontaneous pain (Gierthmühlen and Baron, 2016; Finnerup et al., 2021). Although these pains can exist individually or simultaneously, there are certain differences in the expression and degree of pain due to individual differences. So, the diagnosis and treatment of NPP lack a unified standard, which brings great difficulties in treatment. The injury of central spinal cord and peripheral nerve is the key to induce pain. In addition, some other diseases, such as ischemic injury (Lin et al., 2018), tumor (Gül et al., 2020), inflammatory stimulation (Li et al., 2021a), can stimulate or damage nerves and induce pain in different degrees (Cohen and Mao, 2014). In view of this, it is particularly important to find treatments for pain relief. Currently, great progress has been made in the treatment of NPP, such as drug therapy (Lee et al., 2018a) and physiotherapy (Finnerup et al., 2015), but the effect is still not ideal, especially in drug therapy, which is not only short-term, but also has certain dependence and side effects. Therefore, exploring new and promising treatment methods is on the agenda.
In recent years, with the continuous research and exploration of nerve injury and pain treatment, the concept of cell transplantation has been introduced (Sullivan et al., 2016). Researchers have tried to transplant some special types of cells or intravenously into the host (such as spinal cord injury, sciatic nerve injury, and trigeminal nerve injury) to relieve pain and have achieved good progress (Chen et al., 2013; Ito et al., 2020). Indeed, cell transplantation technology has achieved remarkable results in the treatment of nerve injury. Such as neural stem/progenitor cells (Lee et al., 2021a), olfactory ensheathing cells (Guerout et al., 2014), adipose stem cells (Li et al., 2018), mesenchymal cells (Cofano et al., 2019) and Schwann cells (Li et al., 2020a) in the central nervous system (CNS) and peripheral nervous system (PNS) have neurorestorative effects (Assinck et al., 2017; Kubiak et al., 2020). For example, transplantation of mesenchymal stem cell (MSCs)-derived exosomes into locally injured nerve tissue can effectively reduce inflammation and oxidation, and significantly restore injured nerves (Li et al., 2020b). Accordingly, these cells with functional properties are gradually being used in the treatment of pain, including inflammation-induced pain and cancer-induced pain (Wei et al., 2019; Zhang et al., 2020a). These cells can continuously secrete neuroactive nutritional factors for analgesia (such as neurotrophin Y, brain-derived neurofactor, b-endorphin, enkephalin and vascular growth factor). It has a good effect on long-term analgesia (Chen et al., 2013). Indeed, these living cells transplanted into the body can play a role in alleviating hyperalgesia or lowering pain threshold in the host (Lindvall, 2015; He et al., 2019).
OECs are glial cells that have been studied more in recent years. They can exist for life in the central and peripheral nervous system, constantly renew and survive, secrete a variety of neurotrophic factors, reduce local inflammatory response (such as the release of TNF-a, IL-1β, and IL-18), change the local surrounding microenvironment, repair damaged nerves and reduce inflammatory reaction (Reshamwala et al., 2019; Gilmour et al., 2020). It is worth mentioning that in the treatment of other diseases [such as retinopathy (Yu et al., 2022), dorsal root injury (Minkelyte et al., 2021) and Parkinson’s disease (Weng et al., 2020)], OECs also have a good effect. Correspondingly, OECs have also achieved certain recognition and achievements in the treatment of NPP. Indeed, OECs transplantation contributes to pain-relieving effects (Zheng et al., 2017). Studies have shown that OECs transplantation significantly increases NF200 expression, reduces GFAP expression, improves sensory function after spinal cord injury, reduces P2X4R overexpression, and relieves NPP (Zheng et al., 2017). These findings identify an important contribution of OECs transplantation in the treatment of nerve injury and pain. Therefore, in this paper, we explored the efficacy of OECs on nerve injury repair and the treatment of NPP, and OECs are expected to become a new method and technology for the treatment of pain in the future.
2 Biological characteristics of OECs
Regeneration and repair of nervous system injury is a complex process involving multiple steps and factors. On the one hand, the key to nerve injury repair lies in the survival and function of neurons. On the other hand, damaged axons have the function of bridging the damaged site or broken end (Jessen and Mirsky, 2016; Min et al., 2021). Importantly, axonal regeneration requires remyelination and reconstitution of functional synapses, delivery of neurotrophic factors, improved inflammation, and modulation of immune responses, which are critical for nerve injury repair and regeneration (Otto, 2021). Different studies have found that transplanted cells, such as neural stem cells, have functional properties to accomplish these processes (Hu et al., 2019; Shao et al., 2019). Recently, a large number of studies have revealed a kind of glial cells-OECs, which can promote axonal regeneration and nerve tissue reconstruction, improve functional characteristics, and have received extensive attention in the field of tissue engineering and injury repair (Wright et al., 2018; Collins et al., 2019).
The role of OECs in nerve injury repair and regeneration has been confirmed by more and more studies. The biological characteristics and mechanisms of OECs in repairing damaged nerves and inhibiting pain have been recognized and affirmed, mainly in the following aspects (Table 1):
a OECs can survive and renew for life in the central nervous system, ensuring the number and vitality (Roet and Verhaagen, 2014; Li et al., 2021b).
b Abundant sources of OECs: In animal model studies, OECs are derived from the olfactory bulb tissue and olfactory mucosa (the most superficial layer and the lamina propria), which belong to the central nervous system and peripheral nervous system respectively (Guerout et al., 2014). The in vitro culture survival rate and purity are as high as 95% or more, which is also the key factor for experimental and clinical in vitro culture of OECs (Dombrowski et al., 2006; Delaviz et al., 2008; Radtke et al., 2009; Roet and Verhaagen, 2014).
c Heterogeneity of OECs: Primary OECs are easier to obtain from embryonic animals and early postnatal animals, but it is more difficult to obtain purified OECs due to the abundance of non-OECs in the embryonic and early postnatal olfactory bulbs and olfactory mucosa. Conversely, OECs can be readily purified and cultured from adult olfactory bulbs and olfactory mucosa. Moreover, OECs are obtained from different parts, and there are also certain differences in their effects on nerve damage repair. An important factor is that in vitro culturing of OECs (e.g., culture conditions and media) can affect OECs (Paviot et al., 2011; Honoré et al., 2012). In addition, OECs derived from the olfactory mucosa are closely related to neurons and fibroblasts, while OECs derived from the olfactory bulb are closely related to fibroblasts and astrocytes (Lakatos et al., 2000; Jani and Raisman, 2004; Windus et al., 2010). Compared with olfactory bulb-derived OECs, olfactory mucosa-derived OECs can promote nerve regeneration more (Li et al., 2020c). This means that OECs from different sources may have completely different ability to promote nerve regeneration and the efficacy after transplantation. Therefore, there is a certain heterogeneity in OECs from different sources.
d OECs have the function of secreting various neurotrophic factors, such as neurotrophin Y, brain-derived neurotrophic factor and VEGF: Transplantation of OECs has a strong potential to provide an adaptive microenvironment for damaged nerves, one of which is characterized by the secretion of trophic factors and cellular matrix, improving the microenvironment for axon regeneration and extension (Nazareth et al., 2020). Indeed, OECs can secrete platelet-derived growth factor, neuropeptide Y, glial-derived connexin, S100, ciliary neurotrophic factor (CNTF), these nutrients provide a nutritional basis for nerve regeneration and repair (Ye and Houle, 1997; Ahuja et al., 2020). Importantly, OECs can produce NT-3, and BDNF which effectively promote neuronal survival and axonal sprouting, even extending longer distances within areas of CNS injury (Ma et al., 2010; Gu et al., 2017).
e OECs secrete cell adhesion molecules: In addition to the production of trophic factors, another important feature of OECs is that OECs can produce a series of extracellular matrix proteins, which have been confirmed by different studies to promote neuronal survival, axon regeneration and extension, especially the function of axon guidance (Liu et al., 2010). Indeed, an important mechanism for OECs to promote nerve injury repair is through the secretion of adhesion molecules [such as L1-neural cell adhesion molecule (L1-NCAM) and neural cell adhesion molecule 1 (NCAM1)] (Witheford et al., 2013; Tang et al., 2017; Guo et al., 2020).
f OECs promote axon regeneration and inhibit glial scarring and cavitation (DeLucia et al., 2003; Khankan et al., 2015): Studies have shown that the transplantation of OECs into the injured part of the T8 spinal cord in rats can increase the axonal growth of the neurospinal tract, the blue pulp and the corticospinal tract at the stump of the spinal cord. The expression of GFAP and NG2 is down-regulated in the spinal cord segments around the injury, suggesting that there is a more suitable environment for axon regeneration (López-Vales et al., 2006). OECs treatment can increase the number of regenerated neurons, improve the morphology of nerve fibers, increase the number of myelinated nerve fibers, nerve fiber diameter and myelin sheath thickness (Gu et al., 2019).
g Another interesting feature is the phagocytic activity of OECs (Nazareth et al., 2021): In the past, it was thought that OECs and astrocytes had some similar characteristics, but later it was further found that OECs and microglia also had common characteristics, which means that OECs have the function of phagocytosis of bacteria (Vincent et al., 2007; Panni et al., 2013; Nazareth et al., 2015). OECs or Schwann cells (SCs) are thought to aid regeneration by removing necrotic cells (necrotic bodies, NBS) as well as myelin debris. Interesting finding is that OECs have higher phagocytosis and transport capacity than stem cells (Nazareth et al., 2020). OECs degrade neurocalcitonin, an inhibitor of axonal regeneration, by secreting matrix metalloproteinase-2, which promotes nerve regeneration (Yui et al., 2014). Effective removal of neurodegenerative and apoptotic nerve tissue fragments and cell products are essential for creating an environment that allows damaged neurons to regenerate. The phagocytic activity of OECs can make a substantial contribution to the growth of neurons in this harsh environment, which depends on the phenotypic changes of OECs (enhanced activation and phagocytosis), and this enhanced phagocytic activity greatly promotes the growth of neurons under hostile culture conditions (Hao et al., 2017).
h OECs have the function of “bridge” and remyelination formation ability (Franklin, 2003): Purified OECs express PSA-E-NCAM (polysialo neural cell adhesion molecule) and tenascin, which promote axonal elongation and remyelination ability (Higginson and Barnett, 2011).
i OECs can reduce the local inflammatory response of nerve injury (inhibit the pro-inflammatory effect of astrocytes), reduce the release of inflammatory factors (such as TNF-a), improve the microenvironment around local inflammation, and have a better role in repairing damaged nerves (Su et al., 2009; Chuah et al., 2011). The study found that OECs secreted αB-crystallin (CryAB), an anti-inflammatory protein that coordinated the immune response between cells, and also inhibited the production of neurotoxins by astrocytes, created an environment for nerve growth (Saglam et al., 2021).
j Migration ability of OECs (Santos-Silva et al., 2019): The lipid rafts of OECs are rich in cholesterol, sphingomyelin, phosphatidylcholine, caveolin-1, Flotillin-1, ganglioside Gm1 and gangliosides recognized by 9murine O-acetyl GD3, A2B5-recognized gangliosides, CNPase, α-Actinin and β-1-integrin (Campos et al., 2021). Analysis of the OECs actin cytoskeleton reveals stress fibers, membrane spinous processes, folded membranes and lamellar fat deposits during cell migration and the distribution of α-Actinin in membrane projections (Campos et al., 2021). For the first time, α-actin and Flotillin-1 were found in OECs lipid rafts, suggesting that membrane lipid rafts together with β-1-integrin and gangliosides play a role in the migration of OECs (Campos et al., 2021). OECs have migratory roles in both physiological and pathological conditions of the nervous system, such as inflammation, hypoxia, aging, neurodegenerative diseases, head trauma, brain tumors, and infections (Grassi et al., 2020).
3 The role of OECs in treatment of nerve injury
It is understood that OECs are present in the olfactory bulb and olfactory mucosa, and exist and renew for life in the central and peripheral nervous system. Therefore, the role of OECs in the repair of central injury and peripheral nerve injury has been widely studied and recognized (Duan and Lu, 2015; Khankan et al., 2016). Indeed, OECs transplantation exerts its biological characteristics to improve the microenvironment at the injured nerve site and promote nerve repair. Nerve damage can lead to permanent loss of motor and sensory function, yet current treatments are limited and some are ineffective, inspiring researchers to explore new and promising treatment methods. Fortunately, OECs transplantation has emerged as a possible therapy for CNS or PNS injury or other neurological disorders in animal models (Goulart et al., 2016; Wang et al., 2021a; Lee et al., 2021b). This is because transplanting OECs into the damaged site of the damaged nerve can create better survival conditions for the damaged neurons.
Spinal cord injury is a common site in the central nervous system, and can lead to sensory and motor dysfunction after (Muniswami and Tharion, 2018; Voronova et al., 2018). OECs transplantation can promote axonal regeneration and myelination in spinal cord injury, and can restore part of sensory and motor functions, which all lie in the biological function of OECs (Reshamwala et al., 2019) (Figure 1). The transplantation of OECs to the injured spinal cord can limit the activation and penetration of immune cells, protect axons and neurons, reduce the secretion of inhibitory molecules in the lesion core, and exert neuroprotective and immunomodulatory effects (Khankan et al., 2016). It is worth mentioning that the transplantation of OECs has the effect of promoting the regeneration of the injured site of the blood vessels (Beiersdorfer et al., 2019; Beiersdorfer et al., 2020; Wang et al., 2022). For example, OECs can promote the increase of vascular endothelial growth factor-A and platelet-derived growth factor-AA, effectively promoting the proliferation, migration and formation of vascular-like structures of vascular endothelial cells (Wang et al., 2022).
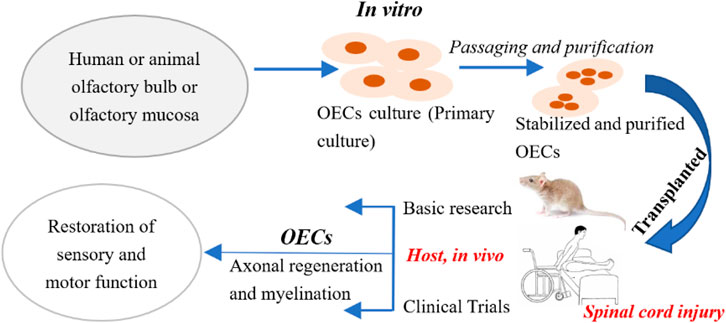
FIGURE 1. Procedure for OECs transplantation of the treatment for spinal cord injury. Step 1: Take olfactory bulb tissue or olfactory mucosa from animals or humans; Step 2: Pay attention to aseptic operation throughout the process, and in vitro primary culture OECs; Step 3: Purify and identify the cultured OECs to obtain stable OECs; Step 4: Transplant the stably cultured OECs into the host; Step 5: Check the host’s sensory and functional recovery after OECs transplantation.
Another important role is that OECs can inhibit the activation of microglial and reduce neuroinflammation (Zhang et al., 2019a; Guo et al., 2020). After OECs transplantation, the expression of interleukin-1 receptor antagonist (IL-1ra) was up-regulated, and the expression of many chemokines, including proinflammatory chemokine IL-1α and IL-1β was down-regulated, which inhibited the activation of microglia (Zhang et al., 2021). Other studies found that the combination of OECs and minocycline reduced cavitation and astrogliosis, further reduced levels of IL-1β, TNF-α, caspase-3 and oxidative stress (Pourkhodadad et al., 2019).
Moreover, scarring caused by astrocytes at the edge of spinal cord injury can hinder axonal regeneration (Deng et al., 2011; Hara et al., 2017; Okada et al., 2018). OECs can integrate and migrate with astrocytes at the site of spinal cord injury, providing a bridge for axons to penetrate scars and grow to the lesion core (Witheford et al., 2013; Khankan et al., 2015). Studies have been found that the combined transplantation of OECs and spinal cord decellularized scaffolds can better promote axonal regeneration, improve the proliferation and distribution of astrocytes at the injury site, and restore some functions (Yu et al., 2021). Another important finding is that OECs can associate with other cells (such as adipose stem cells, neural stem cells and mesenchymal stem cells) (Wang et al., 2010; Yazdani et al., 2012; Gomes et al., 2018), biomaterials (such as albumin scaffolds and spinal cord cell scaffolds) (Ferrero-Gutierrez et al., 2013; Yu et al., 2021) and physical therapy methods (such as exercise training and stimulation) (Thornton et al., 2018; Prager et al., 2021) play a better role in promoting the repair of spinal cord injury. It has been found that the combined transplantation of nerve stem cells (NSCs) and OECs has a synergistic effect on spinal cord injury and promotes nerve regeneration and functional reconstruction (Ao et al., 2007). They also found that OECs could bridge between nerve injuries while performing axon elongation and promoting myelination (Ao et al., 2007). Other studies have further found that co-transplantation of OECs and NSCs can promote the proliferation and differentiation of neural stem cells in vitro. Interestingly, OECs can improve the survival rate of NSCs in vivo and inhibit the programmed necrosis of NSCs after co-transplantation, thus enhancing the therapeutic effect of spinal cord injury (Wang et al., 2021b).
Successful results in animal models show that OECs transplantation is safe and reliable for the treatment of spinal cord injury. Correspondingly, some clinical trials of OECs transplantation for the treatment of spinal cord injury have also been carried out, and certain results have been achieved (Tabakow et al., 2014; Gomes et al., 2016; Gómez et al., 2018). Researchers transplanted human fetal OECs into the upper and lower ends of spinal cord injuries in 300 patients by intraspinal injection, it was found that 300 patients with complete SCI (ASIA grade A) and incomplete SCI (ASIA grade D), rapidly improved some neurological functions (Huang et al., 2006). Wu et al used fetal OECs in a clinical trial in patients with spinal cord injury and tested the safety of six patients with chest injuries and the efficacy of five patients with neck injuries. They found that OECs showed a good safety, with improvements in sensation and spasticity, but the treatment effect was suboptimal (Wu et al., 2012). Although the OECs obtained from the fetus have a certain effect in the treatment of spinal cord injury, some researchers believe that whether the cells obtained from the fetus are pure OECs is still controversial (Hummel et al., 2007). The investigators transplanted autologous OECs into the site of six patients with complete thoracic paralysis and followed up for up to 3 years. They did not find any tumorigenic cells, syringomyelia or other adverse radiological phenomena (Mackay-Sim et al., 2008). Strangely, most patients showed no significant functional changes, but in one patient, both tactile and acupuncture sensitivity were found to improve (Mackay-Sim et al., 2008). They believe that it is feasible and safe to transplant autologous OECs into the injured spinal cord.
Taken together, OECs show a good repair effect in basic experiments, although OECs transplantation also show a certain therapeutic effect in clinical trials, but the results of these clinical trials are not satisfactory. Whether OECs are effective in promoting neural repair after spinal cord injury remains unknown. Therefore, more research data are needed to support the application of OECs in clinical treatment. At present, there are some obstacles to the use of OECs in clinical trials, such as difficulties in obtaining autologous cells, adverse reactions, correct transplantation methods, cell usage and sources, identification and purification of human OECs, etc. Therefore, the use of OECs for the treatment of clinical patients in the future requires a large number of experiments to provide a solid foundation.
4 Pathological mechanism of neuropathic pain
NPP is a symptom caused by direct or indirect damage to the nervous system, resulting in dysfunction or transient disturbance (Bouhassira, 2019). It is worth noting that pain can still exist or persist even after the elimination of nociceptive stimulation, which may be related to the transmission of neurological function and sensory information and the degree of nerve repair, as well as individual differences and psychological influences. Most of the pain is spontaneous pain and hyperalgesia (Meacham et al., 2017; Macone and Otis, 2018). Although the pathological mechanism of NPP is complex, which involves the participation of many factors. However, with the research on the pathological mechanism of NPP, some widespread recognitions have been obtained, which are reflected in the following aspects (Table 2):
a. Nociceptor sensitization and nociceptive fiber afferents (Sun et al., 2018; Simonetti and Kuner, 2020): Nerve damage can lead to decreased nerve fibers and demyelination of axons in the damaged area. It was found that the intracellular calcium and hyperexcitability of sensory neurons increased in the area of nerve injury, and the synaptic plasticity between afferent C fibers and dorsal horn neurons increased (Liu et al., 2015; Yang et al., 2017).
b. Microglia activation: Microglia are resident immune cells in the nervous system, widely distributed in the central nervous system, and are essential for maintaining the stability of the immune microenvironment of the nervous system (Voet et al., 2019). Although, under physiological conditions, microglia are in a quiescent state and lack immune and phagocytic functions. However, they still have a certain ability to migrate and play a certain protective role in the maintenance of the microenvironment in the brain and the stability of the nervous system function (Rajendran and Paolicelli, 2018). Indeed, microglia can migrate to the injury site and promptly remove cell metabolites and apoptotic cell debris, which is very important to ensure the relative stability of the brain environment and maintain the normal function of the central nervous system (Stadelmann et al., 2019; Abe et al., 2020). Moreover, activation of microglia also facilitates myelin formation and axonal regeneration after nerve injury (Pinto et al., 2021). Other studies reveal that efficient remyelination requires mediation of pro-inflammatory microglial death from proliferative to pro-regenerative states (Lloyd et al., 2019). This study implies that microglial cell death or impaired regeneration may underlie dysregulated microglial activation in neurological diseases. Additionally, microglial activation can induce pain by increasing neuroinflammatory responses. While inhibiting the activation of microglia prevents persistent stimulation of neuroinflammation and reduces pain (Inoue and Tsuda, 2018; Yi et al., 2021a; Navia-Pelaez et al., 2021). Studies have shown that 17β-estradiol inhibits the activation of microglia and astrocyte, reduces the expression of IL-1β, IL-6, iNOS and COX-2, and alleviates NPP caused by spinal cord injury (Lee et al., 2018b). Importantly, microglia can communicate with other glial cells and neurons, enhance the transmission of nociceptive information and increase pain perception (Jin et al., 2019; Zhou et al., 2019; Yi et al., 2021b) (Figure 2).
c. Abnormal neuronal activity (St John Smith, 2018; Vilela et al., 2021; Wercberger et al., 2021): Neurons stimulated by noxious factors can lead to abnormal discharge and enhanced synaptic plasticity, enhancing sensory information afferents (Terayama et al., 2015; Yang et al., 2021). In animal models of NPP, parabrachial neurons exhibit enhanced spontaneous and evoked activity and significantly increase afterdischarge responses (Raver et al., 2020). It is worth mentioning that the mutual communication between microglia and dorsal horn neurons also promotes the progression of pain. Indeed, activated microglia help spinal dorsal horn neurons to aggregate nociceptive input to induce NPP (Yamamoto et al., 2015; Terayama et al., 2018). Studies have shown that nerve injury is involved in P2Y12 receptor-dependent GTP-RhoA/ROCK2 signaling, increases the activation of microglia, and increases excitatory synaptic transmission in dorsal horn neurons (Yu et al., 2019), suggest that crosstalk between microglia and neurons is ultimately involved in the performance of nociceptive hyperalgesia. In addition, recent studies have found that ion channels, such as calcium channels, on nociceptor neurons help regulate nociceptive synaptic transmission (Chen et al., 2009; Ritter et al., 2015). For example, rapidly inactivating Kv3.4 potassium current dysfunction in neurons of dorsal root ganglion (DRG) is associated with persistent pain. (Zemel et al., 2017).
d. Neurotransmitter release (eg GABA) (Fan et al., 2022): Kv3.4 channel is a regulator of nerve cell excitability, which can change presynaptic membrane potential, affect glutamatergic fiber transmission, and generate pain afferents (Muqeem et al., 2018). For example, O-desmethyltramadol (M1) inhibits the quantum release of L-glutamate from nerve terminals by activating μ-opioid receptors, but not norepinephrine and serotonin receptors, inhibiting nociceptive information transmission (Koga et al., 2019). These primary afferents activate inhibitory interneurons of the spinal cord, which release (e.g., GABA and enkephalin) as transmitters to regulate pain inputs (Otsuka and Yanagisawa, 1990). Nociceptive receptor neurons release neuropeptides and neurotransmitters from nerve endings and regulate vascular, congenital and adaptive immune cell responses. These afferent fibers release neurotransmitters in the dorsal root ganglion and dorsal horn of the spinal cord and activate microglia as local immune cells. Activated microglia produce pro-inflammatory cytokines, chemokines and neuropeptides, which interact with secondary neurons to amplify the hypersensitivity of secondary neurons and trigger central sensitization (Vergne-Salle and Bertin, 2021).
e. Immune cell infiltration (such as macrophages, and T lymphocytes) (Durante et al., 2021; Micheli et al., 2021): Marked expansion and proliferation of macrophages around injured sensory neurons, involved in pain progression. Depletion of DRG macrophages reduces nerve injury-induced mechanical hypersensitivity and DRG macrophage expansion, prevents nerve injury-induced activation and proliferation of microglia, and reduces hyperalgesia (Yu et al., 2020). Agtr2 macrophages are the main immune cells that invade the site of nerve injury. Interestingly, neuropathic mechanical and cold hyperalgesia are mitigated by peripheral macrophage depletion (Shepherd et al., 2018a). Other studies show that angiotensin II triggers peripheral macrophage-sensory neuron redox crosstalk to trigger pain (Shepherd et al., 2018b).
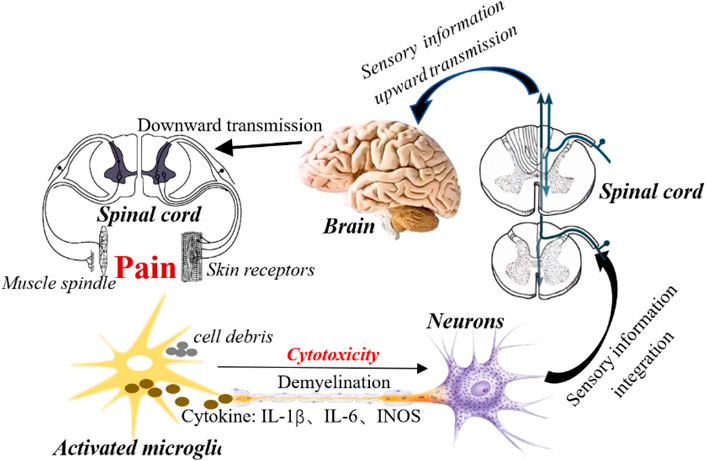
FIGURE 2. Activation of microglial is involved in the development of NPP. Activation of microglia increases the neuroinflammatory response around nerve injury by releasing a variety of cytotoxic factors (such as TNF-a, IL-1β, etc.). In addition, microglia activation transmits nociceptive information to neurons, enhances neuronal synaptic plasticity, enhances sensory information transmission, and stimulated neurons upload sensory information to the spinal cord center. Subsequently, the spinal cord center integrates sensory information to the higher center (brain) through the ascending conduction tract, and then the brain sends out instructions after receiving the information, and passes through the spinal cord through the descending conduction tract to the peripheral receptors (skin and muscle spindles), resulting in pain.
Taken together, the pathological mechanisms of NPP have received some consistent recognition, involving sensory neuron activity, microglia activation, inflammatory cell infiltration and pro-inflammatory factor release, enhanced neurotransmission, and central sensitization. This also means that inhibiting the activation of microglia, reducing the infiltration of inflammatory cells, protecting neurons, and improving the microenvironment of the nerve injury area may be potential mechanisms for the treatment of NPP. However, given that the pathological mechanism of NPP is complex and involves many factors, it is necessary to continuously explore and study the detailed mechanism of NPP.
5 The application of OECs in the treatment of NPP
The treatment of NPP is still a difficult problem to overcome at present. Usually, drugs are used to suppress pain or some physical rehabilitation therapy is used to relieve pain, but the effect is still not ideal, which also brings new challenges to researchers. Therefore, it is increasingly important to explore and find new treatments. Fortunately, in recent years, cell transplantation technology has entered people’s field of vision, and it has been well applied in the repair of nerve damage, and has also achieved satisfactory results. Although the effect in clinical trials is not very satisfactory, the exact role of cell transplantation in repairing damaged nerves and restoring function has been confirmed by different studies. Indeed, transplanting functional cells (such as neural stem cells and Schwann cells) into nerve injury has a good effect of suppressing pain (Zhang et al., 2018a; Du et al., 2019; Wang et al., 2021c). For example, Sertoli cells transplantation significantly improved the recovery of motor function and pain relief, which was related to the decrease of cavity, the expression level of TRPC6 and Caspase3 and the number of activated microglia after transplantation (Rahimi et al., 2022). In view of the unique biological characteristics of OECs, they have become candidate cells for the treatment of nerve injury. Correspondingly, it also exerts a better analgesic effect in pain treatment (Nakhjavan-Shahraki et al., 2018). Transplantation of OECs into spinal cord injury of rat found that the expression of NF200 was significantly increased, the expression of GFAP was decreased, and the sensory nerve function was improved (Zheng et al., 2017).
OECs play a role in pain relief by mediating the expression of some pain-related molecules. P2X purine receptors belong to ATP-dependent ion channel receptor, which is abundantly expressed in nervous system. For example, P2X2/3 receptor is expressed in sensory neurons, P2X4 receptor is expressed in microglia, while P2X7 receptor is expressed in neurons and microglia, and expressed in immune cells (Zhang, 2021). These purine receptors are highly expressed in pain and are closely related to the development of pain. An important mechanism of cell therapy is to exert the pharmacological characteristics of analgesia by reducing the expression of these P2X purine receptors. Transplantation of Schwann cells into sciatic nerve injury reduced the expression level of P2X2/3 receptor in the dorsal root ganglia and suppressed pain (Zhang et al., 2018b). OECs transplantation can relieve pain by down-regulating the expression of P2X purine receptors, which is also a well-studied pathological mechanism. OECs transplantation increased the expression of NF200, decreased the expression of GFAP, and inhibited the overexpression of P2X4 receptor, which plays an important role in NPP induced by spinal cord injury (Zheng et al., 2017). Our previous studies also found that OECs transplantation could significantly reduce the expression of P2X4 receptor in spinal cord and P2X2/3 receptor in dorsal root ganglion, and relieve NPP in rats (Zhao et al., 2015; Zhang et al., 2019b). Trigeminal neuralgia (TN) is a common facial nerve pain. OECs was transplanted into the ligation of infraorbital nerve in rats, the facial mechanical pain threshold increased significantly, which was related to the decreased expression of P2X7 receptor in trigeminal ganglion (Lu et al., 2022). In view of the fact that allogeneic cell transplantation will cause immune rejection, and OECs transplantation is likely to lead to the attack of inflammatory factors, resulting in a decrease in the survival rate of cells transplanted into the host, and the effect of pain relief is reduced. Therefore, later, we used the microencapsulation technology. Microcapsule is a lipid translucent membrane with good immune isolation, macromolecular substances are not easy to pass through, and it has the function of protecting transplanted cells (Olabisi, 2015; Mao et al., 2019; Santos et al., 2021). We transplanted microencapsulated OECs to the site of sciatic nerve injury and found significantly decreased the expression of P2X7 receptor in spinal cord and relieved neuropathic pain in rats. Interestingly, the effect of microencapsulated OECs on down-regulating the expression of P2X7 receptor and relieving pain was stronger than that of OECs alone (Zhang et al., 2019c). This also means that protecting the host OECs and reducing immune rejection may have a better biological function of pain relief, and a new model of cell therapy is proposed.
Under physiological conditions, the expression of NGF in primary sensory neurons was very low, but its expression level increased significantly after nerve injury. The study confirmed that NGF induced the transfer of TRPV1 to the membrane of neurons (Lawrence et al., 2021). However, these neurotrophic activities lead to axonal terminal budding and help to increase local pain sensitivity (Ro et al., 1999). In spinal cord injury, the transplanted neural stem cells restored the sensory function of rats with spinal cord injury, while OECs caused hyperalgesia. However, co-transplantation could promote the survival of neural stem cells and reverse the hyperalgesia induced by OECs. The mechanism may be related to the down-regulation of NGF (Luo et al., 2013). Brain-derived neurotrophic factor (BDNF) is an important member of the NGF family, and its high expression is involved in pain (such as neuropathic pain and trigeminal neuralgia). Studies have shown that transplantation of OECs into the hemi-transected spinal cord can lead to hyperalgesia, possibly due to upregulation of brain-derived neurotrophic factor (BDNF) (Lang et al., 2013). There are differences in the possible causes of different contradictions, the parts and causes of inducing pain, the dose and time of OECs transplantation, and the transplantation methods. In addition, an important point may be related to the regulation of the expression of pain-related molecules by OECs transplantation.
Another characteristic of OECs in nerve injury and pain relief is that activated OECs can effectively promote the proliferation and migration of vascular endothelial cells and the formation of vascular-like structures (Wang et al., 2022). After transplantation of OECs after photochemical injury of spinal cord in rats, the BBB score, motor and somatosensory evoked potential amplitudes were significantly increased, the expression of COX-2 and VEGF of astrocytes in spinal cord tissue increased, and the vascular density increased at the site of spinal cord injury (López-Vales et al., 2004).
The beneficial effect of OECs on pain is attributed to the ability of OECs to cross the boundary of PNS-CNS. The growth factors, cell adhesion molecules and extracellular matrix proteins they produce promote and guide axonal growth, and their ability to remyelinate axons. OECs are thought to help axon regeneration by removing necrotic cells (necrotic bodies) and myelin fragments (Wu et al., 2011). It was found that Schwann cells could produce a small amount of pro-inflammatory cytokine TNF-α, while OECs did not produce detectable TNF-α and engulfed myelin fragments. This also means that OECs have higher phagocytosis and transport capacity than Schwann cells, produce a lower number of pro-inflammatory cytokines, and play a better role in the repair of injured nerves (Nazareth et al., 2020). All these create the basic conditions for the reconstruction of nerve function and pain relief. Other studies have found that 1 week after C7 and C8 dorsal root injury, transplantation of OECs into the dorsal horn effectively alleviated the neuropathological disorders associated with dorsal root injury, including spontaneous pain behavior, tactile hypersensitivity and thermal hyperalgesia (Wu et al., 2011). However, interestingly, delayed OECs transplantation did not improve the sensory control of complex, goal-oriented skillful stretching and ladder walking, and no significant effect of transplanted OECs on injury-induced central recombination and afferent sprouting was found (Wu et al., 2011). This phenomenon may be explained by the antinociceptive effect mediated by OECs transplantation by altering other mechanisms such as inflammation and astrocyte proliferation.
In view of the feasibility of OECs transplantation in the treatment of pain. Later, researchers co-transplanted OECs with other functional cells (such as neural stem cells) or bioscaffolds to relieve pain (Luo et al., 2013; Zhang et al., 2020b). OECs combined with neural stem cells were transplanted into spinal cord injury of rats. It was found that the BBB score of rats increased significantly. And the BBB score increased rapidly at 2 weeks, while the BBB score increased slightly at 6 weeks. The recovery of limb function was better than that of single cell transplantation, and the neat arrangement of spinal cord cells was close to normal (Guo et al., 2015). Moreover, they also found that OECs combined with NSCs can better induce neural stem cells to differentiate into neurons (Guo et al., 2015). The study showed that compared with SCs transplantation, the axonal regeneration of sciatic nerve stump after OECs transplantation was 25% less, but the axonal regeneration of sciatic nerve stump after SCs/OECs combined transplantation was 28% more, which significantly restored the motor and sensory function of rats (You et al., 2011). We later combined OECs with chitosan biomaterials and found that the combined transplantation was more effective in relieving pain than single-cell transplantation (Zhang et al., 2020c).
OECs have made some achievements in the treatment of nerve injury in clinical patients, and their contribution to repairing damaged nerves and restoring some functions is also affirmed. However, there are few reports on the use of OECs in the treatment of patients with NPP clinical. Transplantation of fetal OECs into 17 patients with intractable chronic pain with spinal cord injury aged 6-309 months (mean 102.2 months). The degree of pain before and after 0.5-88 months was compared with the International Society of Neurorepair Spinal Cord injury rating scale (IANRSCIFRS). 0 represents extremely uncontrollable pain, one point represents severe pain that needs and responds to anesthetics, two points represents mild pain that responds to common painkillers, and three points represents no pain. The results showed that pain improved by an average of 1.2 points after OECs transplantation (Chen et al., 2020). Another report is: a 72-year-old stroke patient with right limb sensory and functional impairment and pain for 8 years, 4-month fetal-derived OECs were transplanted into the patient’s brain (cell volume 1x1010/L-1). The results showed that the patient had no adverse reactions such as fever, abnormal platelets and other complications after OECs transplantation (Liu et al., 2008). The pain of the right limb was reduced by 70% on the first day after the operation, 80% on the second day after the operation, and 90% on the third day after the operation. The muscle tension was reduced, and the function of the right limb recovered. The neurological function score increased significantly, and the patient was discharged from the hospital after 3 weeks (Lang et al., 2013). Although there are few reports in clinical trials, but OECs transplantation has a certain effect on pain relief and promotes functional recovery.
Although some data obtained from basic research support the feasibility of OECs for the treatment of pain, this is not enough to support extensive clinical development, which may require overcoming some difficulties. For example, there is still a lack of basic research data to support clinical development, the source and purity of OECs, the selection of cell transplantation time, the transplantation method and the control of the number of transplanted cells, immune rejection and the survival rate of cell transplantation, etc. Therefore, it is limited in clinical trials. However, the therapeutic effect of OECs transplantation on pain and nerve injury has been affirmed, so many problems need to be overcome in the future clinical treatment of OECs. Non-etheless, OECs hold promise as a promising new technology and method for promoting functional repair and pain relief.
6 Conclusion
Nerve injury is accompanied by sensory and functional disorders, leading to pain, which is a common symptom of most diseases. However, the treatment strategies for NPP are limited. Therefore, it is particularly important to find new treatment methods. Fortunately, the role of OECs in nerve injury repair has been affirmed and recognized by a large number of studies. Transplantation of OECs can promote regeneration of injured nerves and restore some body functions. Indeed, OECs transplantation can relieve pain, and exert analgesic effect for a longer period of time. The mechanism by which OECs exert their analgesic effect lies in their unique biological characteristics. Therefore, OECs are expected to be a promising method for the treatment of NPP and bringing new hope to patients in the future.
Author contributions
J-pL: completed this first draft and revision. J-lW: the revision and mechanism diagram is completed. B-eH: literature search. F-lZ: literature Retrieval and revision. C-lW: table making. JS: review and revision. W-jZ: project design, fund acquisition and final revision.
Funding
These studies were supported by grants from the Youth Science Foundation of Jiangxi Province (20224BAB216030). Project of Education Department of Jiangxi Province (GJJ2200238). National Natural incubation Project of the second affiliated Hospital of Nanchang University (2022YNFY12006).
Conflict of interest
The authors declare that the research was conducted in the absence of any commercial or financial relationships that could be construed as a potential conflict of interest.
Publisher’s note
All claims expressed in this article are solely those of the authors and do not necessarily represent those of their affiliated organizations, or those of the publisher, the editors and the reviewers. Any product that may be evaluated in this article, or claim that may be made by its manufacturer, is not guaranteed or endorsed by the publisher.
Abbreviations
Neuropathic pain, NPP; Olfactory ensheathing cells, OECs; nerve stem cells, NSCs; dorsal root ganglion, DRG; entral nervous system, CNS; peripheral nervous system, PNS; spinal cord injury, SCI; interleukin-1β, IL-1β; NALP3, NACHT, LRR and PYD domains-containing protein 3; Tumor necrosis factor- α, TNF-α; interleukin-18, IL-18; Nitric oxide synthase, NOS; brain-derived neurotrophic factor, BDNF.
References
Abe, N., Nishihara, T., Yorozuya, T., and Tanaka, J. (2020). Microglia and macrophages in the pathological central and peripheral nervous systems. Cells 9 (9), 2132. doi:10.3390/cells9092132
Ahuja, C. S., Mothe, A., Khazaei, M., Badhiwala, J. H., Gilbert, E. A., van der Kooy, D., et al. (2020). The leading edge: Emerging neuroprotective and neuroregenerative cell-based therapies for spinal cord injury. Stem Cells Transl. Med. 9 (12), 1509–1530. doi:10.1002/sctm.19-0135
Ao, Q., Wang, A. J., Chen, G. Q., Wang, S. J., Zuo, H. C., and Zhang, X. F. (2007). Combined transplantation of neural stem cells and olfactory ensheathing cells for the repair of spinal cord injuries. Med. Hypotheses 69 (6), 1234–1237. doi:10.1016/j.mehy.2007.04.011
Assinck, P., Duncan, G. J., Hilton, B. J., Plemel, J. R., and Tetzlaff, W. (2017). Cell transplantation therapy for spinal cord injury. Nat. Neurosci. 20 (5), 637–647. doi:10.1038/nn.4541
Beiersdorfer, A., Scheller, A., Kirchhoff, F., and Lohr, C. (2019). Panglial gap junctions between astrocytes and olfactory ensheathing cells mediate transmission of Ca2+ transients and neurovascular coupling. Glia 67 (7), 1385–1400. doi:10.1002/glia.23613
Beiersdorfer, A., Wolburg, H., Grawe, J., Scheller, A., Kirchhoff, F., and Lohr, C. (2020). Sublamina-specific organization of the blood brain barrier in the mouse olfactory nerve layer. Glia 68 (3), 631–645. doi:10.1002/glia.23744
Bouhassira, D. (2019). Neuropathic pain: Definition, assessment and epidemiology. Rev. Neurol. Paris. 175 (1-2), 16–25. doi:10.1016/j.neurol.2018.09.016
Campos, F. S. O., Piña-Rodrigues, F. M., Reis, A., Atella, G. C., Mermelstein, C. S., Allodi, S., et al. (2021). Lipid rafts from olfactory ensheathing cells: Molecular composition and possible roles. Cell. Mol. Neurobiol. 41 (3), 525–536. doi:10.1007/s10571-020-00869-4
Chen, S. R., Cai, Y. Q., and Pan, H. L. (2009). Plasticity and emerging role of BKCa channels in nociceptive control in neuropathic pain. J. Neurochem. 110 (1), 352–362. doi:10.1111/j.1471-4159.2009.06138.x
Chen, L., Huang, H., Sharma, H. S., Zuo, H., and Sanberg, P. R. (2013). Cell transplantation as a pain therapy targets both analgesia and neural repair. Cell. Transpl. 22, 11–19. doi:10.3727/096368913X672091
Chen, L., Huang, H., Jiang, Z., Zhang, F., Liu, Y., Xi, H., et al. (2020). Preliminary result of olfactory ensheathing cell transplantation in intractable neuropathic pain following spinal cord injury: 17 cases report. Chin. J. Rehabil. Theory Pract. 16 (2), 146–148.
Chuah, M. I., Hale, D. M., and West, A. K. (2011). Interaction of olfactory ensheathing cells with other cell types in vitro and after transplantation: Glial scars and inflammation. Exp. Neurol. 229 (1), 46–53. doi:10.1016/j.expneurol.2010.08.012
Cofano, F., Boido, M., Monticelli, M., Zenga, F., Ducati, A., Vercelli, A., et al. (2019). Mesenchymal stem cells for spinal cord injury: Current options, limitations, and future of cell therapy. Int. J. Mol. Sci. 20 (11), 2698. doi:10.3390/ijms20112698
Cohen, S. P., and Mao, J. (2014). Neuropathic pain: Mechanisms and their clinical implications. BMJ 348, f7656. doi:10.1136/bmj.f7656
Collins, A., Ibrahim, A., Li, D., Olushanu, M., and Li, Y. (2019). Reconstruction of the damaged dorsal root entry zone by transplantation of olfactory ensheathing cells. Cell. Transpl. 28 (9-10), 1212–1219. doi:10.1177/0963689719855938
Delaviz, H., Joghataie, M. T., Mehdizadeh, M., Bakhtiyari, M., Nobakht, M., and Khoei, S. (2008). Transplantation of olfactory mucosa improve functional recovery and axonal regeneration following sciatic nerve repair in rats. Iran. Biomed. J. 12 (4), 197–202.
DeLucia, T. A., Conners, J. J., Brown, T. J., Cronin, C. M., Khan, T., and Jones, K. J. (2003). Use of a cell line to investigate olfactory ensheathing cell-enhanced axonal regeneration. Anat. Rec. B New Anat. 271 (1), 61–70. doi:10.1002/ar.b.10014
Deng, L. X., Hu, J., Liu, N., Wang, X., Smith, G. M., Wen, X., et al. (2011). GDNF modifies reactive astrogliosis allowing robust axonal regeneration through Schwann cell-seeded guidance channels after spinal cord injury. Exp. Neurol. 229 (2), 238–250. doi:10.1016/j.expneurol.2011.02.001
Dombrowski, M. A., Sasaki, M., Lankford, K. L., Kocsis, J. D., and Radtke, C. (2006). Myelination and nodal formation of regenerated peripheral nerve fibers following transplantation of acutely prepared olfactory ensheathing cells. Brain Res. 1125 (1), 1–8. doi:10.1016/j.brainres.2006.09.089
Du, X. J., Chen, Y. X., Zheng, Z. C., Wang, N., Wang, X. Y., and Kong, F. E. (2019). Neural stem cell transplantation inhibits glial cell proliferation and P2X receptor-mediated neuropathic pain in spinal cord injury rats. Neural Regen. Res. 14 (5), 876–885. doi:10.4103/1673-5374.249236
Duan, D., and Lu, M. (2015). Olfactory mucosa: A rich source of cell therapy for central nervous system repair. Rev. Neurosci. 26 (3), 281–293. doi:10.1515/revneuro-2014-0065
Durante, M., Squillace, S., Lauro, F., Giancotti, L. A., Coppi, E., Cherchi, F., et al. (2021). Adenosine A3 agonists reverse neuropathic pain via T cell-mediated production of IL-10. J. Clin. Invest. 131 (7), e139299. doi:10.1172/JCI139299
Fan, W., Sullivan, S. J., and Sdrulla, A. D. (2022). Dorsal column and root stimulation at Aβ-fiber intensity activate superficial dorsal horn glutamatergic and GABAergic populations. Mol. Pain 18, 79559. doi:10.1177/17448069221079559
Ferrero-Gutierrez, A., Menendez-Menendez, Y., Alvarez-Viejo, M., Meana, A., and Otero, J. (2013). New serum-derived albumin scaffold seeded with adipose-derived stem cells and olfactory ensheathing cells used to treat spinal cord injured rats. Histol. Histopathol. 28 (1), 89–100. doi:10.14670/HH-28.89
Finnerup, N. B., Attal, N., Haroutounian, S., McNicol, E., Baron, R., Dworkin, R. H., et al. (2015). Pharmacotherapy for neuropathic pain in adults: A systematic review and meta-analysis. Lancet Neurol. 14 (2), 162–173. doi:10.1016/S1474-4422(14)70251-0
Finnerup, N. B., Kuner, R., and Jensen, T. S. (2021). Neuropathic pain: From mechanisms to treatment. Physiol. Rev. 101 (1), 259–301. doi:10.1152/physrev.00045.2019
Franklin, R. J. (2003). Remyelination by transplanted olfactory ensheathing cells. Anat. Rec. B New Anat. 271 (1), 71–76. doi:10.1002/ar.b.10013
Gierthmühlen, J., and Baron, R. (2016). Neuropathic pain. Semin. Neurol. 36 (5), 462–468. doi:10.1055/s-0036-1584950
Gilmour, A. D., Reshamwala, R., Wright, A. A., Ekberg, J. A. K., and St John, J. A. (2020). Optimizing olfactory ensheathing cell transplantation for spinal cord injury repair. J. Neurotrauma 37 (5), 817–829. doi:10.1089/neu.2019.6939
Gomes, E. D., Mendes, S. S., Leite-Almeida, H., Gimble, J. M., Tam, R. Y., Shoichet, M. S., et al. (2016). Combination of a peptide-modified gellan gum hydrogel with cell therapy in a lumbar spinal cord injury animal model. Biomaterials 105, 38–51. doi:10.1016/j.biomaterials.2016.07.019
Gomes, E. D., Mendes, S. S., Assunção-Silva, R. C., Teixeira, F. G., Pires, A. O., Anjo, S. I., et al. (2018). Co-transplantation of adipose tissue-derived stromal cells and olfactory ensheathing cells for spinal cord injury repair. Stem Cells 36 (5), 696–708. doi:10.1002/stem.2785
Gómez, R. M., Sánchez, M. Y., Portela-Lomba, M., Ghotme, K., Barreto, G. E., Sierra, J., et al. (2018). Cell therapy for spinal cord injury with olfactory ensheathing glia cells (OECs). Glia 66 (7), 1267–1301. doi:10.1002/glia.23282
Goulart, C. O., Ângelo Durço, D. F., de Carvalho, L. A., Oliveira, J. T., Alves, L., Cavalcante, L. A., et al. (2016). Olfactory ensheathing glia cell therapy and tubular conduit enhance nerve regeneration after mouse sciatic nerve transection. Brain Res. 1650, 243–251. doi:10.1016/j.brainres.2016.09.021
Grassi, S., Giussani, P., Mauri, L., Prioni, S., Sonnino, S., and Prinetti, A. (2020). Lipid rafts and neurodegeneration: Structural and functional roles in physiologic aging and neurodegenerative diseases. J. Lipid Res. 61 (5), 636–654. doi:10.1194/jlr.TR119000427
Gu, M., Gao, Z., Li, X., Guo, L., Lu, T., Li, Y., et al. (2017). Conditioned medium of olfactory ensheathing cells promotes the functional recovery and axonal regeneration after contusive spinal cord injury. Brain Res. 1654, 43–54. doi:10.1016/j.brainres.2016.10.023
Gu, J., Xu, H., Xu, Y. P., Liu, H. H., Lang, J. T., Chen, X. P., et al. (2019). Olfactory ensheathing cells promote nerve regeneration and functional recovery after facial nerve defects. Neural Regen. Res. 14 (1), 124–131. doi:10.4103/1673-5374.243717
Guerout, N., Paviot, A., Bon-Mardion, N., Honoré, A., Obongo, R., Duclos, C., et al. (2014). Transplantation of olfactory ensheathing cells to evaluate functional recovery after peripheral nerve injury. J. Vis. Exp. 84, e50590. doi:10.3791/50590
Gül, Ş. K., Tepetam, H., and Gül, H. L. (2020). Duloxetine and pregabalin in neuropathic pain of lung cancer patients. Brain Behav. 10 (3), e01527. doi:10.1002/brb3.1527
Guo, S. G., Wang, C. J., Wang, Y. X., and Qu, C. Q. (2015). Transplantation of hyperthermic preconditioning olfactory ensheathing cells combined with neural stem cells in the treatment of central nerve injury. J. Biol. Regul. Homeost. Agents 29 (3), 677–682.
Guo, J., Cao, G., Yang, G., Zhang, Y., Wang, Y., Song, W., et al. (2020). Transplantation of activated olfactory ensheathing cells by curcumin strengthens regeneration and recovery of function after spinal cord injury in rats. Cytotherapy 22 (6), 301–312. doi:10.1016/j.jcyt.2020.03.002
Hao, D. J., Liu, C., Zhang, L., Chen, B., Zhang, Q., Zhang, R., et al. (2017). Lipopolysaccharide and curcumin Co-stimulation potentiates olfactory ensheathing cell phagocytosis via enhancing their activation. Neurotherapeutics 14 (2), 502–518. doi:10.1007/s13311-016-0485-8
Hara, M., Kobayakawa, K., Ohkawa, Y., Kumamaru, H., Yokota, K., Saito, T., et al. (2017). Interaction of reactive astrocytes with type I collagen induces astrocytic scar formation through the integrin-N-cadherin pathway after spinal cord injury. Nat. Med. 23 (7), 818–828. doi:10.1038/nm.4354
He, Y., Sun, D., Dai, C., Xie, J., Li, Y., Dai, J., et al. (2019). Olfactory ensheathing cells grafted into the retina of RCS rats suppress inflammation by down-regulating the JAK/STAT pathway. Front. Cell. Neurosci. 13, 341. doi:10.3389/fncel.2019.00341
Higginson, J. R., and Barnett, S. C. (2011). The culture of olfactory ensheathing cells (OECs)-a distinct glial cell type. Exp. Neurol. 229 (1), 2–9. doi:10.1016/j.expneurol.2010.08.020
Honoré, A., Le Corre, S., Derambure, C., Normand, R., Duclos, C., Boyer, O., et al. (2012). Isolation, characterization, and genetic profiling of subpopulations of olfactory ensheathing cells from the olfactory bulb. Glia 60 (3), 404–413. doi:10.1002/glia.22274
Hu, X., Zhou, X., Li, Y., Jin, Q., Tang, W., Chen, Q., et al. (2019). Application of stem cells and chitosan in the repair of spinal cord injury. Int. J. Dev. Neurosci. 76, 80–85. doi:10.1016/j.ijdevneu.2019.07.005
Huang, H., Wang, H., Chen, L., Gu, Z., Zhang, J., Zhang, F., et al. (2006). Influence factors for functional improvement after olfactory ensheathing cell transplantation for chronic spinal cord injury. Zhongguo Xiu Fu Chong Jian Wai Ke Za Zhi 20 (4), 434–438.
Hummel, T., Kobal, G., Gudziol, H., and Mackay-Sim, A. (2007). Normative data for the "sniffin' sticks" including tests of odor identification, odor discrimination, and olfactory thresholds: An upgrade based on a group of more than 3,000 subjects. Eur. Arch. Otorhinolaryngol. 264 (3), 237–243. doi:10.1007/s00405-006-0173-0
Inoue, K., and Tsuda, M. (2018). Microglia in neuropathic pain: Cellular and molecular mechanisms and therapeutic potential. Nat. Rev. Neurosci. 19 (3), 138–152. doi:10.1038/nrn.2018.2
Ito, T., Toriumi, T., Otake, K., Okuwa, Y., Tanaka, S., Arai, Y., et al. (2020). Performance of Schwann cell transplantation into extracted socket after inferior alveolar nerve injury in a novel rat model. J. Oral Sci. 62 (4), 402–409. doi:10.2334/josnusd.19-0487
Jani, H. R., and Raisman, G. (2004). Ensheathing cell cultures from the olfactory bulb and mucosa. Glia 47 (2), 130–137. doi:10.1002/glia.20038
Jessen, K. R., and Mirsky, R. (2016). The repair Schwann cell and its function in regenerating nerves. J. Physiol. 594 (13), 3521–3531. doi:10.1113/JP270874
Jin, X., Liu, M. Y., Zhang, D. F., Zhong, X., Du, K., Qian, P., et al. (2019). Baicalin mitigates cognitive impairment and protects neurons from microglia-mediated neuroinflammation via suppressing NLRP3 inflammasomes and TLR4/NF-κB signaling pathway. CNS Neurosci. Ther. 25 (5), 575–590. doi:10.1111/cns.13086
Khankan, R. R., Wanner, I. B., and Phelps, P. E. (2015). Olfactory ensheathing cell-neurite alignment enhances neurite outgrowth in scar-like cultures. Exp. Neurol. 269, 93–101. doi:10.1016/j.expneurol.2015.03.025
Khankan, R. R., Griffis, K. G., Haggerty-Skeans, J. R., Zhong, H., Roy, R. R., Edgerton, V. R., et al. (2016). Olfactory ensheathing cell transplantation after a complete spinal cord transection mediates neuroprotective and immunomodulatory mechanisms to facilitate regeneration. J. Neurosci. 36 (23), 6269–6286. doi:10.1523/JNEUROSCI.0085-16.2016
Koga, A., Fujita, T., Piao, L. H., Nakatsuka, T., and Kumamoto, E. (2019). Inhibition by O-desmethyltramadol of glutamatergic excitatory transmission in adult rat spinal substantia gelatinosa neurons. Mol. Pain 15, 824243. doi:10.1177/1744806918824243
Kubiak, C. A., Grochmal, J., Kung, T. A., Cederna, P. S., Midha, R., and Kemp, S. W. P. (2020). Stem-cell-based therapies to enhance peripheral nerve regeneration. Muscle Nerve 61 (4), 449–459. doi:10.1002/mus.26760
Lakatos, A., Franklin, R. J., and Barnett, S. C. (2000). Olfactory ensheathing cells and Schwann cells differ in their in vitro interactions with astrocytes. Glia 32 (3), 214–225. doi:10.1002/1098-1136(200012)32:3<214::aid-glia20>3.0.co;2-7
Lang, B. C., Zhang, Z., Lv, L. Y., Liu, J., Wang, T. Y., Yang, L. H., et al. (2013). OECs transplantation results in neuropathic pain associated with BDNF regulating ERK activity in rats following cord hemisection. BMC Neurosci. 14, 80. doi:10.1186/1471-2202-14-80
Lawrence, G. W., Zurawski, T. H., and Dolly, J. O. (2021). Ca2+Signalling induced by NGF identifies a subset of capsaicin-excitable neurons displaying enhanced chemo-nociception in dorsal root ganglion explants from adult pirt-GCaMP3 mouse. Int. J. Mol. Sci. 22 (5), 2589. doi:10.3390/ijms22052589
Lee, G., Grovey, B., Furnish, T., and Wallace, M. (2018). Medical cannabis for neuropathic pain. Curr. Pain Headache Rep. 22 (1), 8. doi:10.1007/s11916-018-0658-8
Lee, J. Y., Choi, H. Y., Ju, B. G., and Yune, T. Y. (2018). Estrogen alleviates neuropathic pain induced after spinal cord injury by inhibiting microglia and astrocyte activation. Biochim. Biophys. Acta Mol. Basis Dis. 1864 (7), 2472–2480. doi:10.1016/j.bbadis.2018.04.006
Lee, H. L., Yeum, C. E., Lee, H., Oh, J., Kim, J. T., Lee, W. J., et al. (2021). Peripheral nerve-derived stem cell spheroids induce functional recovery and repair after spinal cord injury in rodents. Int. J. Mol. Sci. 22 (8), 4141. doi:10.3390/ijms22084141
Lee, J. Y., Kim, Y. H., Kim, B. Y., Jang, D. H., Choi, S. W., Joen, S. H., et al. (2021). Peripheral nerve regeneration using a nerve conduit with olfactory ensheathing cells in a rat model. Tissue Eng. Regen. Med. 18 (3), 453–465. doi:10.1007/s13770-020-00326-9
Li, X., Zhao, S., and Wang, L. (2018). Therapeutic effect of adipose-derived stem cell transplantation on optic nerve injury in rats. Mol. Med. Rep. 17 (2), 2529–2534. doi:10.3892/mmr.2017.8103
Li, R., Li, D., Wu, C., Ye, L., Wu, Y., Yuan, Y., et al. (2020). Nerve growth factor activates autophagy in Schwann cells to enhance myelin debris clearance and to expedite nerve regeneration. Theranostics 10 (4), 1649–1677. doi:10.7150/thno.40919
Li, L., Zhang, Y., Mu, J., Chen, J., Zhang, C., Cao, H., et al. (2020). Transplantation of human mesenchymal stem-cell-derived exosomes immobilized in an adhesive hydrogel for effective treatment of spinal cord injury. Nano Lett. 20 (6), 4298–4305. doi:10.1021/acs.nanolett.0c00929
Li, M., Zhu, Q., and Liu, J. (2020). Olfactory ensheathing cells in facial nerve regeneration. Braz J. Otorhinolaryngol. 86 (5), 525–533. doi:10.1016/j.bjorl.2018.07.006
Li, Z., Zhu, J., and Wang, Y. (2021). ADAR3 alleviated inflammation and pyroptosis of neuropathic pain by targeting NLRP3 in chronic constriction injury mice. Gene 805, 145909. doi:10.1016/j.gene.2021.145909
Li, J. X., Gao, Z. C., He, X. J., Li, J., and Zhao, H. (2021). [Advances in olfactory ensheathing cells for the treatment of spinal cord injury]. Zhongguo Gu Shang 34 (8), 785–790. Chinese. doi:10.12200/j.issn.1003-0034.2021.08.018
Lin, Y., Chen, Z., Tang, J., Cao, P., and Shi, R. (2018). Acrolein contributes to the neuropathic pain and neuron damage after ischemic-reperfusion spinal cord injury. Neuroscience 384, 120–130. doi:10.1016/j.neuroscience.2018.05.029
Lindvall, O. (2015). Treatment of Parkinson's disease using cell transplantation. Philos. Trans. R. Soc. Lond B Biol. Sci. 370, 20140370. doi:10.1098/rstb.2014.0370
Liu, R. W., Chen, L., Huang, H. Y., Zhang, F., Xiao, J., and Zhang, X. D. (2008). Fetal olfactory ensheathing cell transplantation for post-stroke pain in one case. Zhongguo Zuzhi Gongcheng Yanjiu yu Linchuang Kangfu 2 (25), 4945–4947.
Liu, Y., Teng, X., Yang, X., Song, Q., Lu, R., Xiong, J., et al. (2010). Shotgun proteomics and network analysis between plasma membrane and extracellular matrix proteins from rat olfactory ensheathing cells. Cell. Transpl. 19 (2), 133–146. doi:10.3727/096368910X492607
Liu, S., Liu, Y. P., Huang, Z. J., Zhang, Y. K., Song, A. A., Ma, P. C., et al. (2015). Wnt/Ryk signaling contributes to neuropathic pain by regulating sensory neuron excitability and spinal synaptic plasticity in rats. Pain 156 (12), 2572–2584. doi:10.1097/j.pain.0000000000000366
Lloyd, A. F., Davies, C. L., Holloway, R. K., Labrak, Y., Ireland, G., Carradori, D., et al. (2019). Central nervous system regeneration is driven by microglia necroptosis and repopulation. Nat. Neurosci. 22 (7), 1046–1052. doi:10.1038/s41593-019-0418-z
López-Vales, R., García-Alías, G., Forés, J., Navarro, X., and Verdú, E. (2004). Increased expression of cyclo-oxygenase 2 and vascular endothelial growth factor in lesioned spinal cord by transplanted olfactory ensheathing cells. J. Neurotrauma 21 (8), 1031–1043. doi:10.1089/0897715041651105
López-Vales, R., Forés, J., Verdú, E., and Navarro, X. (2006). Acute and delayed transplantation of olfactory ensheathing cells promote partial recovery after complete transection of the spinal cord. Neurobiol. Dis. 21 (1), 57–68. doi:10.1016/j.nbd.2005.06.011
Lovaglio, A. C., Socolovsky, M., Di Masi, G., and Bonilla, G. (2019). Treatment of neuropathic pain after peripheral nerve and brachial plexus traumatic injury. Neurol. India 67, S32–S37. doi:10.4103/0028-3886.250699
Lu, J., Yang, B., Liao, J., Chen, B., Lu, M., Zhang, W., et al. (2022). Olfactory ensheathing cells alleviate facial pain in rats with trigeminal neuralgia by inhibiting the expression of P2X7 receptor. Brain Sci. 12 (6), 706. doi:10.3390/brainsci12060706
Luo, Y., Zou, Y., Yang, L., Liu, J., Liu, S., Liu, J., et al. (2013). Transplantation of NSCs with OECs alleviates neuropathic pain associated with NGF downregulation in rats following spinal cord injury. Neurosci. Lett. 549, 103–108. doi:10.1016/j.neulet.2013.06.005
Ma, Y. H., Zhang, Y., Cao, L., Su, J. C., Wang, Z. W., Xu, A. B., et al. (2010). Effect of neurotrophin-3 genetically modified olfactory ensheathing cells transplantation on spinal cord injury. Cell. Transpl. 19 (2), 167–177. doi:10.3727/096368910X492634
Mackay-Sim, A., Féron, F., Cochrane, J., Bassingthwaighte, L., Bayliss, C., Davies, W., et al. (2008). Autologous olfactory ensheathing cell transplantation in human paraplegia: A 3-year clinical trial. Brain 131 (9), 2376–2386. doi:10.1093/brain/awn173
Macone, A., and Otis, J. A. D. (2018). Neuropathic pain. Semin. Neurol. 38 (6), 644–653. doi:10.1055/s-0038-1673679
Mao, A. S., Özkale, B., Shah, N. J., Vining, K. H., Descombes, T., Zhang, L., et al. (2019). Programmable microencapsulation for enhanced mesenchymal stem cell persistence and immunomodulation. Proc. Natl. Acad. Sci. U. S. A. 116 (31), 15392–15397. doi:10.1073/pnas.1819415116
Meacham, K., Shepherd, A., Mohapatra, D. P., and Haroutounian, S. (2017). Neuropathic pain: Central vs. Peripheral mechanisms. Curr. Pain Headache Rep. 21 (6), 28. doi:10.1007/s11916-017-0629-5
Micheli, L., Durante, M., Lucarini, E., Sgambellone, S., Lucarini, L., Di Cesare Mannelli, L., et al. (2021). The histamine H4Receptor participates in the anti-neuropathic effect of the adenosine A3 receptor agonist IB-meca: Role of CD4+ T cells. Biomolecules 11 (10), 1447. doi:10.3390/biom11101447
Min, Q., Parkinson, D. B., and Dun, X. P. (2021). Migrating Schwann cells direct axon regeneration within the peripheral nerve bridge. Glia 69 (2), 235–254. doi:10.1002/glia.23892
Minkelyte, K., Collins, A., Liadi, M., Ibrahim, A., Li, D., and Li, Y. (2021). High-yield mucosal olfactory ensheathing cells restore loss of function in rat dorsal root injury. Cells 10 (5), 1186. doi:10.3390/cells10051186
Muniswami, D. M., and Tharion, G. (2018). Functional recovery following the transplantation of olfactory ensheathing cells in rat spinal cord injury model. Asian Spine J. 12 (6), 998–1009. doi:10.31616/asj.2018.12.6.998
Muqeem, T., Ghosh, B., Pinto, V., Lepore, A. C., and Covarrubias, M. (2018). Regulation of nociceptive glutamatergic signaling by presynaptic Kv3.4 channels in the rat spinal dorsal horn. J. Neurosci. 38 (15), 3729–3740. doi:10.1523/JNEUROSCI.3212-17.2018
Nakhjavan-Shahraki, B., Yousefifard, M., Rahimi-Movaghar, V., Baikpour, M., Nasirinezhad, F., Safari, S., et al. (2018). Transplantation of olfactory ensheathing cells on functional recovery and neuropathic pain after spinal cord injury; systematic review and meta-analysis. Sci. Rep. 8 (1), 325. doi:10.1038/s41598-017-18754-4
Navia-Pelaez, J. M., Choi, S. H., Dos Santos Aggum Capettini, L., Xia, Y., Gonen, A., Agatisa-Boyle, C., et al. (2021). Normalization of cholesterol metabolism in spinal microglia alleviates neuropathic pain. J. Exp. Med. 218 (7), e20202059. doi:10.1084/jem.20202059
Nazareth, L., Tello Velasquez, J., Lineburg, K. E., Chehrehasa, F., St John, J. A., and Ekberg, J. A. (2015). Differing phagocytic capacities of accessory and main olfactory ensheathing cells and the implication for olfactory glia transplantation therapies. Mol. Cell. Neurosci. 65, 92–101. doi:10.1016/j.mcn.2015.03.005
Nazareth, L., Shelper, T. B., Chacko, A., Basu, S., Delbaz, A., Lee, J. Y. P., et al. (2020). Key differences between olfactory ensheathing cells and Schwann cells regarding phagocytosis of necrotic cells: Implications for transplantation therapies. Sci. Rep. 10 (1), 18936. doi:10.1038/s41598-020-75850-8
Nazareth, L., St John, J., Murtaza, M., and Ekberg, J. (2021). Phagocytosis by peripheral glia: Importance for nervous system functions and implications in injury and disease. Front. Cell. Dev. Biol. 9, 660259. doi:10.3389/fcell.2021.660259
Okada, S., Hara, M., Kobayakawa, K., Matsumoto, Y., and Nakashima, Y. (2018). Astrocyte reactivity and astrogliosis after spinal cord injury. Neurosci. Res. 126, 39–43. doi:10.1016/j.neures.2017.10.004
Olabisi, R. M. (2015). Cell microencapsulation with synthetic polymers. J. Biomed. Mater Res. A 103 (2), 846–859. doi:10.1002/jbm.a.35205
Otsuka, M., and Yanagisawa, M. (1990). Pain and neurotransmitters. Cell. Mol. Neurobiol. 10 (3), 293–302. doi:10.1007/BF00711176
Otto, G. (2021). Myelin loss in AD. Nat. Rev. Neurosci. 22 (8), 456–457. doi:10.1038/s41583-021-00492-2
Panni, P., Ferguson, I. A., Beacham, I., Mackay-Sim, A., Ekberg, J. A., and St John, J. A. (2013). Phagocytosis of bacteria by olfactory ensheathing cells and Schwann cells. Neurosci. Lett. 539, 65–70. doi:10.1016/j.neulet.2013.01.052
Paviot, A., Guérout, N., Bon-Mardion, N., Duclos, C., Jean, L., Boyer, O., et al. (2011). Efficiency of laryngeal motor nerve repair is greater with bulbar than with mucosal olfactory ensheathing cells. Neurobiol. Dis. 41 (3), 688–694. doi:10.1016/j.nbd.2010.12.004
Philpott, H. T., O'Brien, M., and McDougall, J. J. (2017). Attenuation of early phase inflammation by cannabidiol prevents pain and nerve damage in rat osteoarthritis. Pain 158 (12), 2442–2451. doi:10.1097/j.pain.0000000000001052
Pinto, M. V., Santos, F. M. F., Barros, C., Ribeiro, A. R., Pischel, U., Gois, P. M. P., et al. (2021). BASHY dye platform enables the fluorescence bioimaging of myelin debris phagocytosis by microglia during demyelination. Cells 10 (11), 3163. doi:10.3390/cells10113163
Pourkhodadad, S., Oryan, S. H., Kaka, G., and Sadraie, S. H. (2019). Neuroprotective effects of combined treatment with minocycline and olfactory ensheathing cells transplantation against inflammation and oxidative stress after spinal cord injury. Cell. J. 21 (2), 220–228. doi:10.22074/cellj.2019.6126
Prager, J., Ito, D., Carwardine, D. R., Jiju, P., Chari, D. M., Granger, N., et al. (2021). Delivery of chondroitinase by canine mucosal olfactory ensheathing cells alongside rehabilitation enhances recovery after spinal cord injury. Exp. Neurol. 340, 113660. doi:10.1016/j.expneurol.2021.113660
Radtke, C., Aizer, A. A., Agulian, S. K., Lankford, K. L., Vogt, P. M., and Kocsis, J. D. (2009). Transplantation of olfactory ensheathing cells enhances peripheral nerve regeneration after microsurgical nerve repair. Brain Res. 1254, 10–17. doi:10.1016/j.brainres.2008.11.036
Rahimi, B., Aliaghaei, A., Ramezani, F., Behroozi, Z., and Nasirinezhad, F. (2022). Sertoli cell transplantation attenuates microglial activation and inhibits TRPC6 expression in neuropathic pain induced by spinal cord injury. Physiol. Behav. 251, 113807. doi:10.1016/j.physbeh.2022.113807
Rajendran, L., and Paolicelli, R. C. (2018). Microglia-mediated synapse loss in alzheimer's disease. J. Neurosci. 38 (12), 2911–2919. doi:10.1523/JNEUROSCI.1136-17.2017
Raver, C., Uddin, O., Ji, Y., Li, Y., Cramer, N., Jenne, C., et al. (2020). An amygdalo-parabrachial pathway regulates pain perception and chronic pain. J. Neurosci. 40 (17), 3424–3442. doi:10.1523/JNEUROSCI.0075-20.2020
Reshamwala, R., Shah, M., St John, J., and Ekberg, J. (2019). Survival and integration of transplanted olfactory ensheathing cells are crucial for spinal cord injury repair: Insights from the last 10 Years of animal model studies. Cell. Transpl. 28 (1), 132S–159S. doi:10.1177/0963689719883823
Ritter, D. M., Zemel, B. M., Hala, T. J., O'Leary, M. E., Lepore, A. C., and Covarrubias, M. (2015). Dysregulation of Kv3.4 channels in dorsal root ganglia following spinal cord injury. J. Neurosci. 35 (3), 1260–1273. doi:10.1523/JNEUROSCI.1594-14.2015
Ro, L. S., Chen, S. T., Tang, L. M., and Jacobs, J. M. (1999). Effect of NGF and anti-NGF on neuropathic pain in rats following chronic constriction injury of the sciatic nerve. Pain 79 (2-3), 265–274. doi:10.1016/s0304-3959(98)00164-x
Roet, K. C., and Verhaagen, J. (2014). Understanding the neural repair-promoting properties of olfactory ensheathing cells. Exp. Neurol. 261, 594–609. doi:10.1016/j.expneurol.2014.05.007
Saglam, A., Calof, A. L., and Wray, S. (2021). Novel factor in olfactory ensheathing cell-astrocyte crosstalk: Anti-inflammatory protein α-crystallin B. Glia 69 (4), 1022–1036. doi:10.1002/glia.23946
Santos, A. P., Chevallier, S. S., de Haan, B., de Vos, P., and Poncelet, D. (2021). Impact of electrostatic potential on microcapsule-formation and physicochemical analysis of surface structure: Implications for therapeutic cell-microencapsulation. J. Biomater. Appl. 36 (4), 638–647. doi:10.1177/0885328221988979
Santos-Silva, A., Piña-Rodrigues, F. M., Mermelstein, C. D. S., Allodi, S., Barradas, P. C., and Cavalcante, L. A. (2019). A role for gangliosides and β1-integrin in the motility of olfactory ensheathing glia. J. Anat. 235 (5), 977–983. doi:10.1111/joa.13057
Sato, K. L., Johanek, L. M., Sanada, L. S., and Sluka, K. A. (2014). Spinal cord stimulation reduces mechanical hyperalgesia and glial cell activation in animals with neuropathic pain. Anesth. Analg. 118 (2), 464–472. doi:10.1213/ANE.0000000000000047
Shao, A., Tu, S., Lu, J., and Zhang, J. (2019). Crosstalk between stem cell and spinal cord injury: Pathophysiology and treatment strategies. Stem Cell. Res. Ther. 10 (1), 238. doi:10.1186/s13287-019-1357-z
Shepherd, A. J., Mickle, A. D., Golden, J. P., Mack, M. R., Halabi, C. M., de Kloet, A. D., et al. (2018). Macrophage angiotensin II type 2 receptor triggers neuropathic pain. Proc. Natl. Acad. Sci. U. S. A. 115 (34), E8057–E8066. doi:10.1073/pnas.1721815115
Shepherd, A. J., Copits, B. A., Mickle, A. D., Karlsson, P., Kadunganattil, S., Haroutounian, S., et al. (2018). Angiotensin II triggers peripheral macrophage-to-sensory neuron redox crosstalk to elicit pain. J. Neurosci. 38 (32), 7032–7057. doi:10.1523/JNEUROSCI.3542-17.2018
Simonetti, M., and Kuner, R. (2020). Spinal Wnt5a plays a key role in spinal dendritic spine remodeling in neuropathic and inflammatory pain models and in the proalgesic effects of peripheral Wnt3a. J. Neurosci. 40 (35), 6664–6677. doi:10.1523/JNEUROSCI.2942-19.2020
St John Smith, E. (2018). Advances in understanding nociception and neuropathic pain. J. Neurol. 265 (2), 231–238. doi:10.1007/s00415-017-8641-6
Stadelmann, C., Timmler, S., Barrantes-Freer, A., and Simons, M. (2019). Myelin in the central nervous system: Structure, function, and pathology. Physiol. Rev. 99 (3), 1381–1431. doi:10.1152/physrev.00031.2018
Su, Z., Yuan, Y., Chen, J., Cao, L., Zhu, Y., Gao, L., et al. (2009). Reactive astrocytes in glial scar attract olfactory ensheathing cells migration by secreted TNF-alpha in spinal cord lesion of rat. PLoS One 4 (12), e8141. doi:10.1371/journal.pone.0008141
Sullivan, R., Dailey, T., Duncan, K., Abel, N., and Borlongan, C. V. (2016). Peripheral nerve injury: Stem cell therapy and peripheral nerve transfer. Int. J. Mol. Sci. 17 (12), 2101. doi:10.3390/ijms17122101
Sun, J., Li, N., Duan, G., Liu, Y., Guo, S., Wang, C., et al. (2018). Increased Nav1.7 expression in the dorsal root ganglion contributes to pain hypersensitivity after plantar incision in rats. Mol. Pain 14, 8782323. doi:10.1177/1744806918782323
Tabakow, P., Raisman, G., Fortuna, W., Czyz, M., Huber, J., Li, D., et al. (2014). Functional regeneration of supraspinal connections in a patient with transected spinal cord following transplantation of bulbar olfactory ensheathing cells with peripheral nerve bridging. Cell. Transpl. 23 (12), 1631–1655. doi:10.3727/096368914X685131
Tang, Y. Y., Guo, W. X., Lu, Z. F., Cheng, M. H., Shen, Y. X., and Zhang, Y. Z. (2017). Ginsenoside Rg1 promotes the migration of olfactory ensheathing cells via the PI3K/akt pathway to repair rat spinal cord injury. Biol. Pharm. Bull. 40 (10), 1630–1637. doi:10.1248/bpb.b16-00896
Terayama, R., Yamamoto, Y., Kishimoto, N., Maruhama, K., Mizutani, M., Iida, S., et al. (2015). Peripheral nerve injury activates convergent nociceptive input to dorsal horn neurons from neighboring intact nerve. Exp. Brain Res. 233 (4), 1201–1212. doi:10.1007/s00221-015-4203-2
Terayama, R., Tabata, M., Maruhama, K., and Iida, S. (2018). A3 adenosine receptor agonist attenuates neuropathic pain by suppressing activation of microglia and convergence of nociceptive inputs in the spinal dorsal horn. Exp. Brain Res. 236 (12), 3203–3213. doi:10.1007/s00221-018-5377-1
Thornton, M. A., Mehta, M. D., Morad, T. T., Ingraham, K. L., Khankan, R. R., Griffis, K. G., et al. (2018). Evidence of axon connectivity across a spinal cord transection in rats treated with epidural stimulation and motor training combined with olfactory ensheathing cell transplantation. Exp. Neurol. 309, 119–133. doi:10.1016/j.expneurol.2018.07.015
Vergne-Salle, P., and Bertin, P. (2021). Chronic pain and neuroinflammation. Jt. Bone Spine 88 (6), 105222. doi:10.1016/j.jbspin.2021.105222
Vilela, F. C., Vieira, J. S., Vitor-Vieira, F., Kalil-Cutti, B., da Silva, J. R. T., Giusti-Paiva, A., et al. (2021). Maternal separation increases pain sensitivity by reducing the activity of serotonergic neurons in the dorsal raphe nucleus and noradrenergic neurons in locus coeruleus. Neurosci. Lett. 748, 135734. doi:10.1016/j.neulet.2021.135734
Vincent, A. J., Choi-Lundberg, D. L., Harris, J. A., West, A. K., and Chuah, M. I. (2007). Bacteria and PAMPs activate nuclear factor kappaB and Gro production in a subset of olfactory ensheathing cells and astrocytes but not in Schwann cells. Glia 55 (9), 905–916. doi:10.1002/glia.20512
Voet, S., Prinz, M., and van Loo, G. (2019). Microglia in central nervous system inflammation and multiple sclerosis pathology. Trends Mol. Med. 25 (2), 112–123. doi:10.1016/j.molmed.2018.11.005
Voronova, A. D., Valikhov, M. P., Stepanova, O. V., Mel'nikov, P. A., Chadin, A. V., Sidoruk, K. N., et al. (2018). Survival and migration of rat olfactory ensheathing cells after transplantation into posttraumatic cysts in the spinal cord. Bull. Exp. Biol. Med. 166 (1), 118–123. doi:10.1007/s10517-018-4299-z
Wang, G., Ao, Q., Gong, K., Zuo, H., Gong, Y., and Zhang, X. (2010). Synergistic effect of neural stem cells and olfactory ensheathing cells on repair of adult rat spinal cord injury. Cell. Transpl. 19 (10), 1325–1337. doi:10.3727/096368910X505855
Wang, G. Y., Cheng, Z. J., Yuan, P. W., Li, H. P., and He, X. J. (2021). Olfactory ensheathing cell transplantation alters the expression of chondroitin sulfate proteoglycans and promotes axonal regeneration after spinal cord injury. Neural Regen. Res. 16 (8), 1638–1644. doi:10.4103/1673-5374.301023
Wang, X., Kuang, N., Chen, Y., Liu, G., Wang, N., Kong, F., et al. (2021). Transplantation of olfactory ensheathing cells promotes the therapeutic effect of neural stem cells on spinal cord injury by inhibiting necrioptosis. Aging (Albany NY) 13 (6), 9056–9070. doi:10.18632/aging.202758
Wang, L., Gu, S., Gan, J., Tian, Y., Zhang, F., Zhao, H., et al. (2021). Neural stem cells overexpressing nerve growth factor improve functional recovery in rats following spinal cord injury via modulating microenvironment and enhancing endogenous neurogenesis. Front. Cell. Neurosci. 15, 773375. doi:10.3389/fncel.2021.773375
Wang, X., Jiang, C., Zhang, Y., Chen, Z., Fan, H., Zhang, Y., et al. (2022). The promoting effects of activated olfactory ensheathing cells on angiogenesis after spinal cord injury through the PI3K/Akt pathway. Cell. Biosci. 12 (1), 23. doi:10.1186/s13578-022-00765-y
Wei, Z., Fei, Y., Su, W., and Chen, G. (2019). Emerging role of Schwann cells in neuropathic pain: Receptors, glial mediators and myelination. Front. Cell. Neurosci. 13, 116. doi:10.3389/fncel.2019.00116
Weng, S. J., Chen, C. F., Huang, Y. S., Chiu, C. H., Wu, S. C., Lin, C. Y., et al. (2020). Olfactory ensheathing cells improve the survival of porcine neural xenografts in a Parkinsonian rat model. Xenotransplantation 27 (2), e12569. doi:10.1111/xen.12569
Wercberger, R., Braz, J. M., Weinrich, J. A., and Basbaum, A. I. (2021). Pain and itch processing by subpopulations of molecularly diverse spinal and trigeminal projection neurons. Proc. Natl. Acad. Sci. U. S. A. 118 (28), e2105732118. doi:10.1073/pnas.2105732118
Windus, L. C., Lineburg, K. E., Scott, S. E., Claxton, C., Mackay-Sim, A., Key, B., et al. (2010). Lamellipodia mediate the heterogeneity of central olfactory ensheathing cell interactions. Cell. Mol. Life Sci. 67 (10), 1735–1750. doi:10.1007/s00018-010-0280-3
Witheford, M., Westendorf, K., and Roskams, A. J. (2013). Olfactory ensheathing cells promote corticospinal axonal outgrowth by a L1 CAM-dependent mechanism. Glia 61 (11), 1873–1889. doi:10.1002/glia.22564
Wright, A. A., Todorovic, M., Tello-Velasquez, J., Rayfield, A. J., St John, J. A., and Ekberg, J. A. (2018). Enhancing the therapeutic potential of olfactory ensheathing cells in spinal cord repair using neurotrophins. Cell. Transpl. 27 (6), 867–878. doi:10.1177/0963689718759472
Wu, A., Lauschke, J. L., Gorrie, C. A., Cameron, N., Hayward, I., Mackay-Sim, A., et al. (2011). Delayed olfactory ensheathing cell transplants reduce nociception after dorsal root injury. Exp. Neurol. 229 (1), 143–157. doi:10.1016/j.expneurol.2010.07.006
Wu, J., Sun, T., Ye, C., Yao, J., Zhu, B., and He, H. (2012). Clinical observation of fetal olfactory ensheathing glia transplantation (OEGT) in patients with complete chronic spinal cord injury. Cell. Transpl. 21 (1), S33–S37. doi:10.3727/096368912X633743
Yamamoto, Y., Terayama, R., Kishimoto, N., Maruhama, K., Mizutani, M., Iida, S., et al. (2015). Activated microglia contribute to convergent nociceptive inputs to spinal dorsal horn neurons and the development of neuropathic pain. Neurochem. Res. 40 (5), 1000–1012. doi:10.1007/s11064-015-1555-8
Yang, Q. O., Yang, W. J., Li, J., Liu, F. T., Yuan, H., and Ou Yang, Y. P. (2017). Ryk receptors on unmyelinated nerve fibers mediate excitatory synaptic transmission and CCL2 release during neuropathic pain induced by peripheral nerve injury. Mol. Pain 13, 7709372. doi:10.1177/1744806917709372
Yang, H., de Jong, J. W., Cerniauskas, I., Peck, J. R., Lim, B. K., Gong, H., et al. (2021). Pain modulates dopamine neurons via a spinal-parabrachial-mesencephalic circuit. Nat. Neurosci. 24 (10), 1402–1413. doi:10.1038/s41593-021-00903-8
Yazdani, S. O., Pedram, M., Hafizi, M., Kabiri, M., Soleimani, M., Dehghan, M. M., et al. (2012). A comparison between neurally induced bone marrow derived mesenchymal stem cells and olfactory ensheathing glial cells to repair spinal cord injuries in rat. Tissue Cell. 44 (4), 205–213. doi:10.1016/j.tice.2012.03.003
Ye, J. H., and Houle, J. D. (1997). Treatment of the chronically injured spinal cord with neurotrophic factors can promote axonal regeneration from supraspinal neurons. Exp. Neurol. 143 (1), 70–81. doi:10.1006/exnr.1996.6353
Yi, M. H., Liu, Y. U., Liu, K., Chen, T., Bosco, D. B., Zheng, J., et al. (2021). Chemogenetic manipulation of microglia inhibits neuroinflammation and neuropathic pain in mice. Brain Behav. Immun. 92, 78–89. doi:10.1016/j.bbi.2020.11.030
Yi, M. H., Liu, Y. U., Umpierre, A. D., Chen, T., Ying, Y., Zheng, J., et al. (2021). Optogenetic activation of spinal microglia triggers chronic pain in mice. PLoS Biol. 19 (3), e3001154. doi:10.1371/journal.pbio.3001154
You, H., Wei, L., Liu, Y., Oudega, M., Jiao, S. S., Feng, S. N., et al. (2011). Olfactory ensheathing cells enhance Schwann cell-mediated anatomical and functional repair after sciatic nerve injury in adult rats. Exp. Neurol. 229 (1), 158–167. doi:10.1016/j.expneurol.2010.08.034
Yu, T., Zhang, X., Shi, H., Tian, J., Sun, L., Hu, X., et al. (2019). P2Y12 regulates microglia activation and excitatory synaptic transmission in spinal lamina II neurons during neuropathic pain in rodents. Cell. Death Dis. 10 (3), 165. doi:10.1038/s41419-019-1425-4
Yu, X., Liu, H., Hamel, K. A., Morvan, M. G., Yu, S., Leff, J., et al. (2020). Dorsal root ganglion macrophages contribute to both the initiation and persistence of neuropathic pain. Nat. Commun. 11 (1), 264. doi:10.1038/s41467-019-13839-2
Yu, F., Li, P., Du, S., Lui, K. W., Lin, Y., Chen, L., et al. (2021). Olfactory ensheathing cells seeded decellularized scaffold promotes axonal regeneration in spinal cord injury rats. J. Biomed. Mater Res. A 109 (5), 779–787. doi:10.1002/jbm.a.37066
Yu, Y., Li, L., Lin, S., and Hu, J. (2022). Update of application of olfactory ensheathing cells and stem cells/exosomes in the treatment of retinal disorders. Stem Cell. Res. Ther. 13 (1), 11. doi:10.1186/s13287-021-02685-z
Yui, S., Fujita, N., Chung, C. S., Morita, M., and Nishimura, R. (2014). Olfactory ensheathing cells (OECs) degrade neurocan in injured spinal cord by secreting matrix metalloproteinase-2 in a rat contusion model. Jpn. J. Vet. Res. 62 (4), 151–162.
Zemel, B. M., Muqeem, T., Brown, E. V., Goulão, M., Urban, M. W., Tymanskyj, S. R., et al. (2017). Calcineurin dysregulation underlies spinal cord injury-induced K+Channel dysfunction in DRG neurons. J. Neurosci. 37 (34), 8256–8272. doi:10.1523/JNEUROSCI.0434-17.2017
Zhang, Y. L., Chen, D. J., Yang, B. L., Liu, T. T., Li, J. J., Wang, X. Q., et al. (2018). Microencapsulated Schwann cell transplantation inhibits P2X3 receptor expression in dorsal root ganglia and neuropathic pain. Neural Regen. Res. 13 (11), 1961–1967. doi:10.4103/1673-5374.238715
Zhang, Y. L., Liu, Y. G., Chen, D. J., Yang, B. L., Liu, T. T., Li, J. J., et al. (2018). Microencapsulated Schwann cell transplantation inhibits P2X2/3 receptors overexpression in a sciatic nerve injury rat model with neuropathic pain. Neurosci. Lett. 676, 51–57. doi:10.1016/j.neulet.2018.03.063
Zhang, L., Zhuang, X., Chen, Y., and Xia, H. (2019). Intravenous transplantation of olfactory bulb ensheathing cells for a spinal cord hemisection injury rat model. Cell. Transpl. 28 (12), 1585–1602. doi:10.1177/0963689719883842
Zhang, W., Liu, Y., Sun, Y., and Liu, Z. (2019). Microencapsulated olfactory ensheathing cell transplantation reduces P2X4 receptor overexpression and inhibits neuropathic pain in rats. Brain Res. 1724, 146465. doi:10.1016/j.brainres.2019.146465
Zhang, W., Liu, Y., Sun, Y., and Liu, Z. (2019). Effects of microencapsulated olfactory ensheathing cell transplantation on neuropathic pain and P2X7 receptor expression in the L4-5 spinal cord segment. Neurosci. Lett. 701, 48–53. doi:10.1016/j.neulet.2019.02.013
Zhang, W. J., Zhu, J. F., and Zhu, Z. M. (2020). Transplantation of microencapsulated neural stem cells inhibits neuropathic pain mediated by P2X7 receptor overexpression. Biochem. Biophys. Res. Commun. 533 (4), 1219–1225. doi:10.1016/j.bbrc.2020.09.112
Zhang, W. J., Luo, H. L., Zhu, J. F., Hu, C. G., and Zhu, Z. M. (2020). Transplantation of olfactory ensheathing cells combined with chitosan down-regulates the expression of P2X7 receptor in the spinal cord and inhibits neuropathic pain. Brain Res. 1748, 147058. doi:10.1016/j.brainres.2020.147058
Zhang, W. J., Luo, H. L., and Zhu, Z. M. (2020). Effect of olfactory ensheathing cells combined with chitosan on inhibition of P2×4 receptor over-expression-mediated neuropathic pain. Neurosci. Lett. 722, 134859. doi:10.1016/j.neulet.2020.134859
Zhang, L., Zhuang, X., Kotitalo, P., Keller, T., Krzyczmonik, A., Haaparanta-Solin, M., et al. (2021). Intravenous transplantation of olfactory ensheathing cells reduces neuroinflammation after spinal cord injury via interleukin-1 receptor antagonist. Theranostics 11 (3), 1147–1161. doi:10.7150/thno.52197
Zhang, W. J. (2021). Effect of P2X purinergic receptors in tumor progression and as a potential target for anti-tumor therapy. Purinergic Signal 17 (1), 151–162. doi:10.1007/s11302-020-09761-8
Zhao, H., Yang, B. L., Liu, Z. X., Yu, Q., Zhang, W. J., Yuan, K., et al. (2015). Microencapsulation improves inhibitory effects of transplanted olfactory ensheathing cells on pain after sciatic nerve injury. Neural Regen. Res. 10 (8), 1332–1337. doi:10.4103/1673-5374.162769
Zheng, Z., Du, X., Zhang, K., Wang, X., Chen, Y., Kuang, N., et al. (2017). Olfactory ensheathing cell transplantation inhibits P2X4 receptor overexpression in spinal cord injury rats with neuropathic pain. Neurosci. Lett. 651, 171–176. doi:10.1016/j.neulet.2017.04.060
Keywords: OECs, nerve injury, neuropathic pain, treatment, cell transplantation
Citation: Liu J-p, Wang J-l, Hu B-e, Zou F-l, Wu C-l, Shen J and Zhang W-j (2023) Olfactory ensheathing cells and neuropathic pain. Front. Cell Dev. Biol. 11:1147242. doi: 10.3389/fcell.2023.1147242
Received: 18 January 2023; Accepted: 27 March 2023;
Published: 05 April 2023.
Edited by:
Govindan Dayanithi, Centre National de la Recherche Scientifique (CNRS), FranceReviewed by:
Luis R. Hernandez-Miranda, Charité University Medicine Berlin, GermanyRonak Reshamwala, Griffith University, Australia
Copyright © 2023 Liu, Wang, Hu, Zou, Wu, Shen and Zhang. This is an open-access article distributed under the terms of the Creative Commons Attribution License (CC BY). The use, distribution or reproduction in other forums is permitted, provided the original author(s) and the copyright owner(s) are credited and that the original publication in this journal is cited, in accordance with accepted academic practice. No use, distribution or reproduction is permitted which does not comply with these terms.
*Correspondence: Jie Shen, NTI1OTU0NDIwQHFxLmNvbQ==; Wen-jun Zhang, NzA3Mzg0NzMxQHFxLmNvbQ==
†These authors have contributed equally to this work