- Key Laboratory of Physical Fitness and Exercise Rehabilitation of Hunan Province, Hunan Normal University, Changsha, China
Exercise is one of the most effective treatments for the diseases of aging. In recent years, a growing number of researchers have used Drosophila melanogaster to study the broad benefits of regular exercise in aging individuals. With the widespread use of Drosophila exercise models and the upgrading of the Drosophila exercise apparatus, we should carefully examine the differential contribution of regular exercise in the aging process to facilitate more detailed quantitative measurements and assessment of the exercise phenotype. In this paper, we review some of the resources available for Drosophila exercise models. The focus is on the impact of regular exercise or exercise adaptation in the aging process in Drosophila and highlights the great potential and current challenges faced by this model in the field of anti-aging research.
1 Introduction
Exercise is a serious challenge to systemic homeostasis and it causes a wide range of effects in a variety of cells, tissues and organs (Hawley et al., 2014). Indeed, a single exercise session is sufficient to produce acute changes at the transcriptional level (Williams et al., 1996), (Pilegaard et al., 2003). Multiple repetitions of exercise can produce exercise adaptation and more lasting effects on protein function (McGee and Hargreaves, 2020). Planned regular exercise can delay the development of chronic metabolic diseases, including cardiovascular diseases (CVDs), type 2 diabetes (T2D), insulin resistance and obesity (Hawley and Krook, 2016), (Fiuza-Luces et al., 2018), (Umpierre et al., 2011), (Goodyear and Kahn, 1998), (Castaño et al., 2020), (Houghton et al., 2017).
Aging is a major risk factor for CVDs, T2D and neurodegenerative diseases (Paneni et al., 2017), (Laiteerapong et al., 2019), (Hou et al., 2019). Epidemiological studies have shown that the increase in human lifespan has led to a high incidence of aging-related diseases, which places a huge burden on the world health care system (Partridge et al., 2018). Therefore, healthy aging will become one of the most important goals to be addressed today. Aging is determined by complex interactions between biology, environment, and society, which are beyond the control of the individual (Myint and Welch, 2012). But, lifestyle interventions can help to maintain health, such as increasing exercise as well as controlling diet (Partridge et al., 2018). It is well known that lifespan has heritable properties and therefore has a genetic basis. This was shown in studies in Drosophila, where differences in lifespan can be almost twofold across genetic backgrounds and these differences are heritable, supporting the model of genetic determination of lifespan (Campisi et al., 2019). Drosophila has powerful genetic tools and short lifespan characteristics, which make it an ideal model organism for studying lifespan and aging (Helfand and Rogina, 2003), (Makarova et al., 2015). In addition, Drosophila models have many notable achievements in age-related diseases, such as CVDs, sarcopenia and neurodegenerative diseases (Diop and Bodmer, 2015), (Hunt et al., 2021), (Feany and Bender, 2000), (Lu and Vogel, 2009).
Although exercise is an economical and effective treatment for age-related diseases (Li et al., 2020a), (Fiuza-Luces et al., 2018), (Batsis and Villareal, 2018), there are still many limitations in human and animal studies due to life cycle limitations (Blice-Baum et al., 2019). In recent years, Drosophila exercise models with short lifespan and mature genetic tools have become the optimal choice for researchers (Li et al., 2020b), (Kim et al., 2020), (Wen et al., 2016).
2 Development of a Drosophila exercise model for cardiovascular aging research
CVDs are the leading cause of death worldwide, with an estimated 17.9 million deaths from CVDs in 2019, accounting for 32% of global deaths (WHO (World Health Organization), 2017). Aging is a major risk factor for CVDs, including atherosclerosis, hypertension, myocardial infarction, and stroke (Paneni et al., 2017), (North and Sinclair, 2012). Exercise therapy is an economical and effective therapy to reduce mortality and risk of heart disease (Goenka and Lee, 2017). These studies are equally applicable in flies. In aged Drosophila, exercise enhances cardiac function and improves cardiomyocyte ultrastructure and heart failure (Piazza et al., 2009), (Li et al., 2020b). Nicotinamide adenine dinucleotide (NAD+) is a central metabolite associated with atherosclerosis, ischemic, diabetic, arrhythmogenic, hypertrophic or dilated cardiomyopathy, and different forms of heart failure (Abdellatif et al., 2021). Recently, several studies using Drosophila models have shown that NAD + supplementation improves mitochondrial mass, delays accelerated aging and extends lifespan through DCT-1 and ULK-1 (Fang et al., 2019). Consistently, mice prolong lifespan by supplementation with the NAD + precursor nicotinamide riboside (NR) (Zhang et al., 2016). In addition, high expression of NAD + synthase protein positively affected cardiac function in aging flies, including increased cardiac output and reduced heart failure (Wen et al., 2016). Similarly, NAD + precursor treatment also improved cardiac function in aged MDX mice with cardiomyopathy and improved mitochondrial and cardiac function in a mouse model of iron deficiency heart failure (Ryu et al., 2016), (Xu et al., 2015). During physical exercise, cellular energy requirements change all the time, including NAD + and NADH concentrations (White and Schenk, 2012). In mice, swimming increased NAD + levels in muscle (Cantó et al., 2010). In rats, endurance exercise resulted in a sustained increase in NAD + levels in the gastrocnemius muscle of young and aging rats (Koltai et al., 2010). A recent study showed that exercise increased cardiac NAD + levels and PGC-1α activity to improve lipotoxic cardiomyopathy in aged flies, which was associated with NAD+/dSIR2/PGC-1α pathway activation (Wen et al., 2019a). Drosophila dSir2, a homolog of mammalian Sir2, encodes deacetylase activity that prolongs flies lifespan (Rosenberg and Parkhurst, 2002), (Griswold et al., 2008). In addition, exercise activates the cardiac dSir2/Foxo/SOD and dSir2/Foxo/bmm pathways and reduces the occurrence of diastolic dysfunction as well as enhances cardiac contractility (Wen et al., 2019b). Exercise not only improves CVDs in aging Drosophila, but also resists the stress on the heart caused by a high-fat, high-sugar and high-salt diet. For example, lipid levels in the heart are significantly increased in dFatphet mutants, and exercise rescues myocardial lipid content and cardiac function (Sujkowski et al., 2012). Long-term exercise resists high salt-induced premature cardiac failure by blocking CG2196 (salt)/TOR/oxidative stress and activating dFOXO/PGC-1α (Wen et al., 2021a). Electrical pacing produced a significantly increased rate of heart failure when flies were exposed to a high sucrose diet (Bazzell et al., 2013). Aging is an important cause of arrhythmias (Chadda et al., 2018). In aged flies, early physical exercise improves arrhythmias, mainly by reducing the incidence of fibrillation and increasing the occurrence of bradycardia (Zheng et al., 2017). These facts show a great similarity, and humans compared to rats. For humans, exercise training is important for the prevention and treatment of CVDs (Lavie et al., 2015). For example, persistent regular exercise provides benefits in a variety of diseases, including atherosclerosis, atrial fibrillation, heart failure, and cardiac lipotoxic injury (Rognmo et al., 2012), (Risom et al., 2017), (Cattadori et al., 2018), (Schrauwen-Hinderling et al., 2010). In mice, the same beneficial effects of exercise on the heart have been reported (Fiuza-Luces et al., 2018), (Harris et al., 2020), (Vujic et al., 2018), (Börzsei et al., 2021), (Cheedipudi et al., 2020). In conclusion, this illustrates the significance of the Drosophila exercise model for the study of cardiac function.
3 Development of Drosophila exercise models in circadian rhythm studies
Aging leads to a weakening of circadian rhythms, such as the sleep/wake cycle. These rhythms are generated by biological clocks, which are based on cell-autonomous negative feedback loops involving clock genes that display molecular oscillations in approximately 24-h cycles. Clock genes are conserved from Drosophila to humans, and their oscillatory activity coordinates rhythms at the molecular, physiological and behavioral levels (Rakshit et al., 2013). Drosophila exhibits a sleep-like state that is regulated by both circadian rhythms and homeostasis (Shaw et al., 2000). It has been reported that knockdown of dATF-2 in pacemaker neurons decreases sleep duration, while ectopic expression of dATF-2 increases sleep duration (Shimizu et al., 2008). However, the degree of dATF-2 phosphorylation can be enhanced by forced exercise of the dp38 pathway (Shimizu et al., 2008). This suggests that dATF-2 is the regulator that links sleep to exercise. Furthermore, both chronic hypoxia and exercise improved sleep quality and climbing ability and extended maximum lifespan in aged flies, but exercise was insensitive to improvements in circadian rest/activity rhythms (Li et al., 2020b), (Zheng et al., 2017). Neuropeptide F (NPF) positive clock neurons have been reported to be critical for the control of nocturnal activity in Drosophila (Hermann et al., 2012). Interestingly, Drosophila exercise is similar to humans in maintaining and improving circadian rhythms (Rakshit et al., 2013), (Gabriel and Zierath, 2019). However, in Drosophila, the link between regular exercise and NPF remains poorly understood. Another study showed that exercise increased the duration of nighttime sleep by decreasing nocturnal activity, while also increasing the number of second deep sleeps and the intensity of daytime activity (Li et al., 2020b). Therefore, it will be interesting to study the effect of exercise and NPF on circadian rhythms. In contrast, one study reported that regular exercise did not improve circadian rhythms or lifespan in wild-type flies, but the clock mutant per 01 significantly reduced climbing ability with or without exercise, suggesting a role for some specific clock genes in maintaining health as part of healthy aging (Rakshit et al., 2013). The paradoxes that lead to the results may be due to differences in model building, including exercise devices, protocols and detection means. As an excellent model of circadian biology and aging, Drosophila is well suited to be combined with exercise to explore the molecular pathways between exercise and circadian rhythms. However, we must carefully consider the experimental errors caused by different exercise devices and protocols, otherwise revealing the intrinsic connection between exercise and circadian rhythms will become difficult.
4 Development of Drosophila exercise models in obesity-related diseases
Obesity is a global epidemic that is associated with aging and diet (Santos and Sinha, 2021). Obesity increases the risk of many health problems, including T2D, metabolic syndrome, CVDs, and cancer, and therefore leads to higher mortality (Aune et al., 2016), (Global BMI Mortality Collaboration Di Angelantonio et al., 2016). Physical exercise prevents obesity, reduces visceral fat and maintains body weight (Oppert et al., 2021), (Swift et al., 2018), (Villareal et al., 2017). Drosophila has become an excellent model for metabolic and diet-related diseases due to its powerful genetic tools and stable reproducible phenotype (Birse et al., 2010), (Musselman and Kühnlein, 2018). Although exercise is believed to mitigate the damage caused by obesity, it remains controversial (Waters et al., 2013). Therefore, the Drosophila exercise model serves as a bridge to reveal the intrinsic relation between exercise and obesity. Drosophila need only be fed a diet containing 30% coconut oil for 5 days to exhibit a phenotype similar to that of the mammalian metabolic syndrome, including increased glucose levels and decreased insulin-like peptide 2 (Dilp2) levels (Birse et al., 2010). The TOR pathway is associated with nutrient-sensing signaling in flies (Luong et al., 2006). Reducing the function of the TOR pathway may accelerate lipolytic metabolism and may also reduce lipid anabolism or storage (Birse et al., 2010). These results are similar to those in humans and rodents in that elevated TG levels induced by high-fat diets are associated with disruptions in lipid and glucose homeostasis, and mitochondrial function, which may lead to lipid accumulation and lipotoxic damage (Ouwens et al., 2005), (Unger, 2003), (Schaffer, 2003). Recent studies have found that regular exercise reduces aging-induced increases in cardiac triglycerides, which may be associated with activation of the cardiac dSir2 pathway (Wen et al., 2019b). Regular exercise is also able to increase antioxidant defense and control the production of RS required for cellular metabolic regulation, improving adiposity and glycemia (Dahleh et al., 2021). In addition, exercise may also reduce high-fat diet-induced whole-body hypertriglyceride levels by decreasing the expression of apoLpp (Ding et al., 2021). It is well known that high-fat diet-induced obesity induces cardiac lipid accumulation and leads to the development of lipotoxic cardiomyopathy. A study showed that lipotoxic cardiomyopathy can be reversed by exercise activation of the Nmnat/NAD+/SIR2 pathway (Wen et al., 2021b). These results have similarities with some studies in humans and mammals, such as exercise improving dyslipidemia and insulin resistance by reducing apolipoprotein B in patients with T2D (Alam et al., 2004), and in aged rats, exercise training promoting SIRT1 activity and improving antioxidant defenses in heart and adipose tissue (Ferrara et al., 2008). Another recent study showed that exercise and cold stimulation were able to alter the expression levels of the brown fat and beige fat markers ucp1, serca2b, β3-adrenergic receptor, prdm16, ampk, and camk, and reduce lipid accumulation (Huang et al., 2022). Although the above studies are not sufficient to prove whether exercise has an effect on lipid browning in flies, they provide indirect evidence, which suggests that exercise holds great potential in the regulation of lipid metabolism in flies.
5 Drosophila exercise model in skeletal muscle aging
In humans, the mortality and pathogenesis of many age-related diseases are related to the functional status, metabolic demands and mass of skeletal muscle, suggesting that skeletal muscle is a key regulator of whole-body aging (Anker et al., 1997) (Metter et al., 2002) (Nair, 2005) (Ruiz et al., 2008). In Drosophila melanogaster, the organization and metabolism of skeletal muscle fibers is similar to that of mammals (Piccirillo et al., 2014). But, muscles undergo more drastic age-related degeneration, which may be due to the lack of satellite stem cells and the limited muscle repair capacity of this organism (Grotewiel et al., 2005). A major difference between Drosophila and mammalian muscles is the lack of muscle stem cells. This feature makes Drosophila muscle, excellent models for identifying the mechanisms by which assembled sarcomeres are maintained and repaired without the confounding influence of regeneration as found in mammalian muscle (Christian and Benian, 2020). In addition, Drosophila muscle function can be analyzed by measuring their ability to fly and climb (Gargano et al., 2005). Due to these properties, flies are emerging as a useful model organism to study muscle aging together with mammalian models.
Skeletal muscle aging is a risk factor for the development of several age-related diseases, such as sarcopenia, metabolic syndrome, cancer, Alzheimer’s disease, and Parkinson’s disease (Christian and Benian, 2020), (Ruiz et al., 2011), (Demontis et al., 2013a). Exercise and muscle function are important predictors of age-related mortality in humans (Anker et al., 1997), (Metter et al., 2002), (Demontis et al., 2013b). For example, exercise protects transgenic mice with Alzheimer’s disease and Parkinson’s disease from neurodegeneration (Zigmond et al., 2009). Endurance exercise rescues mitochondrial defects and premature aging in mice defective in proofreading-exonuclease activity of mitochondrial DNA polymerase γ (Safdar et al., 2011). Another study showed that Sestrins are necessary and sufficient for beneficial adaptations to muscle function and metabolism in Drosophila and mice (Kim et al., 2020). Knockdown of Sestrins reduced endurance and flight in exercise-adapted flies (Sujkowski and Wessells, 2021). Similarly, knockdown of Sestrins in exercise mice impeded endurance and metabolic benefits (Sujkowski and Wessells, 2021). Drosophila muscle-specific dSesn expression replicates similar improvements in aging mobility by exercise and mediates changes in lysosomal activity in a variety of tissues, and both adaptations are dependent on TORC2-Akt activity and PGC1α (Sujkowski and Wessells, 2021). In addition, Drosophila muscle can play an important role in delaying aging (Rai et al., 2021). The first finding was that adult muscle-specific overexpression of dFOXO prolongs lifespan in Drosophila (Demontis and Perrimon, 2010). Although these findings underscore the fundamental role of muscle in regulating systemic aging, the molecular mechanisms involved in this inter-tissue communication are largely unknown.
Exercise not only produces beneficial effects in one’s own muscles but also has the potential to trigger beneficial effects in other tissues. Examples include increased energy expenditure and clearance of ectopic lipid stores (Hawley et al., 2014), improved insulin sensitivity and lower circulating insulin levels (Hawley et al., 2014), and increased secretion of exercise-regulated myocytokines, including irisin (Whitham et al., 2018) and extracellular vesicles (Arnold et al., 2011). Myokines can act on distant tissues such as adipose tissue, liver, pancreatic β-cells and endothelium (Pedersen and Febbraio, 2012). Insulin-like growth factor-1 (IGF-1) is an actin produced by muscles in response to exercise (Pedersen and Febbraio, 2012), (Hede et al., 2012). In Drosophila, ImpL2 is a member of the immunoglobulin superfamily, similar to mammalian IGFBP7, which binds to Dilps and inhibits insulin signaling and promotes mitochondrial autophagy (Owusu-Ansah et al., 2013). Mild muscle mitochondrial damage preserves mitochondrial function, inhibits age-dependent degeneration of muscle function and structure, and prolongs lifespan (Copeland et al., 2009), (Kirchman et al., 1999), (Dillin et al., 2002), (Liu et al., 2005). Although muscle-derived insulin-like growth factor binding protein is not detected in the circulation, it induces muscle hypertrophy after exercise in an autocrine/paracrine manner (Vinciguerra et al., 2010), (Silverman et al., 1995). It is well known that physical exercise counteracts the deleterious effects of secondary aging by preventing the decline in mitochondrial respiration, attenuating the loss of muscle mass associated with aging, and enhancing insulin sensitivity (Cartee et al., 2016). Although the Drosophila exercise model is not well studied in the field of skeletal muscle aging, its evolutionarily conserved myokines and short lifespan characteristics make it an excellent model for studying the role in intertissue communication.
6 Different genetic backgrounds of Drosophila exercise models
A growing number of studies have used Drosophila exercise to mimic phenotypes similar to those of humans, including increased endurance, improved age-related decreases in mobility and cardiac function, improved lipid metabolism, and increased lifespan (Wen et al., 2016), (Ding et al., 2021), (Lowman et al., 2018), (Piazza et al., 2009). Drosophila exercise is a complex multifactorial response and it has different exercise performance in different genetic backgrounds, including climbing speed and endurance (Damschroder et al., 2020). In addition, age, diet and gender are also factors that influence exercise performance. For example, climbing speed, endurance and flight performance decrease with age (Damschroder et al., 2020), (Sujkowski et al., 2019). The effect of diet on endurance is dramatic and affects acute endurance and adaptation to chronic exercise training, with diet composition having a greater effect than calorie content (Bazzell et al., 2013). In addition, the effect of gender on exercise is equally important; when looking at the distribution of activity levels during the same 2-h exercise session, there is a strong correlation between gender and exercise, with females experiencing an early burst of activity and males maintaining activity levels throughout the exercise session (Sujkowski et al., 2020). The mechanisms that lead to genotypic variation in exercise capacity are important to uncover the genetic pathways of exercise, and we should take advantage of cross-species genetics to better explore the interactions between exercise and aging-related diseases.
7 Drosophila exercise device
More than 40 years ago, scientists discovered that Drosophila exhibit an inherent behavior of crawling against gravity when at the bottom of a vial, a behavior commonly referred to as negative geotaxis (Miquel et al., 1976). Negative geotaxis in Drosophila requires Johnston’s organ, a mechanosensory structure located in the tentacles that also detects near-field sounds (Kamikouchi et al., 2009), (Sun et al., 2009). To date, five Drosophila exercise devices have been described. A decade ago, a first generation locomotion device, the Power Tower, was developed based on the negative tropism of Drosophila (Piazza et al., 2009). The Power Tower device lifts up flies fixed to a platform by a motor and then lets the platform fall freely with gravity, causing the flies to fall to the bottom of the bottle (Figure 1A). Due to their negative geostasis response, the flies crawl upward until the motor makes them fall to the bottom of the bottle once again. The TreadWheel device stimulates flies to crawl upward in a fully rotating manner and avoids some physical shocks during locomotion (Mendez et al., 2016), (Katzenberger et al., 2013) (Figure 1B). The Swing Boat device allows the tube to be rotated alternately 30° to each side and also allows the collection of data during locomotion in combination with the Drosophila Monitoring System (DAMSystem) (Berlandi et al., 2017) (Figure 1C). REQS is an upgraded version of the TreadWheel, similar to the Swing Boat, allowing the combination of a DAMSystem to quantify the level of movement of flies (Watanabe and Riddle, 2018) (Figure 1D). In addition, the Key Laboratory of Physical and Exercise Rehabilitation of Hunan Province also developed a Drosophila exercise device in an experiment in which a motor was controlled to drive the flip of the vial on the platform (Figure 1E). The difference is that each rotation of the device is 180 and this stimulates the flies to actively walk upwards inside the vial (Zheng et al., 2015). This device is called “Flip Bottle” because it keeps turning the bottle during its operation. All in all, the other four devices are mainly rotational in design compared to the Power Tower device. The “rotational” approach allows for greater avoidance of physical damage during the exercise of flies. Although the upgrade of the exercise device reduces the possibility of physical damage, there are still some questions about the efficiency of flies’ exercise in the vial and how to accurately determine the intensity of exercise. Various research protocols currently use motor rotation speed and time as key factors in determining exercise intensity (Katzenberger et al., 2013), (Berlandi et al., 2017), (Watanabe and Riddle, 2018). In short, Power Tower triggers Drosophila exercise through mechanical vibration, while the other four trigger exercise through rotation, including full rotation for TreadWheel and REQS, alternating 30 per side for Swing Boat, and alternating 180° per side for Flip Bottle. In addition, Swing Boat and REQS incorporate the DAMSystem, which makes them more objective in monitoring the intensity of exercise. However, in previous studies it was found that flies exhibited a passive tendency to climb after exercising for a period of time (usually after 20–30 min) (Watanabe and Riddle, 2017). This means that flies stay in a certain location in the vial and their range of movement decreases dramatically, which makes it more difficult to determine the intensity of exercise. It is well known that regular exercise can bring great benefits for healthy aging, and the emergence of Drosophila exercise models will further reveal the relationship between exercise and aging. Therefore, precise quantification of exercise intensity and the development of more advanced Drosophila exercise devices will become highly relevant in the future.
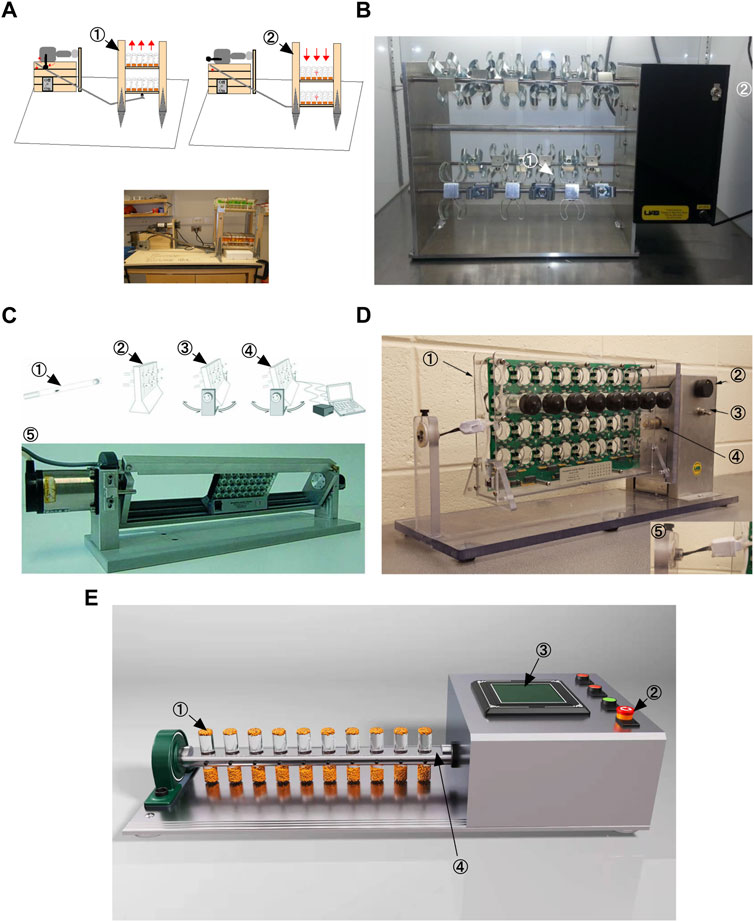
FIGURE 1. Different Drosophila exercise devices. (A) Power Tower. This is adapted from Piazza et al., 2009. (B) TreadWheel. This is adapted from Mendez et al., 2016. (C) Swing Boat. This is adapted from Berlandi et al., 2017. (D) REQS. This is adapted from Watanabe and Riddle, 2018 (E) Flip Bottle.
8 Summary
Drosophila and other animal models are widely used to study the relationship between exercise and aging, such as mice and zebrafish (Murphy et al., 2021), (van Praag et al., 2005). But because of the complexity of these systems, we need simpler model organisms to overcome these challenges. Drosophila has the obvious advantage of being a first-line model for testing hundreds of potential longevity enhancers in multicellular organisms and can be easily adapted to mammalian models. Drosophila exercise produces physiological characteristics similar to those of humans (Figure 2). As described in this review, the Drosophila exercise model provides some new insights into cardiac aging, abnormal lipid metabolism, circadian rhythm disorders, and skeletal muscle aging. In addition to this, exercise regulates a variety of neurological disorders, including neuroendocrine, neurotransmitter, neuroinsulin signaling, antioxidant and anti-inflammatory responses, and cell survival and death pathways (Viru, 1992), (Meeusen and De Meirleir, 1995), (Lovatel et al., 2013), (Monteiro-Junior et al., 2015), (Kurgan et al., 2019), (Serra et al., 2019), (Kang et al., 2013). Surprisingly, however, Drosophila exercise models have only been reported in studies of octopamine and its receptors, a powerful neuromodulator that affects sensory and cognitive functions in insects (Zheng et al., 2015), (Sujkowski et al., 2017), (Farooqui, 2007). Many neuronal genes and neuronal transcriptional regulators were reported in a recent study to be affected by the exercise of the genus Drosophila (Watanabe and Riddle, 2021). Therefore, in the future, more studies will apply Drosophila exercise models to explore neurological disorders.
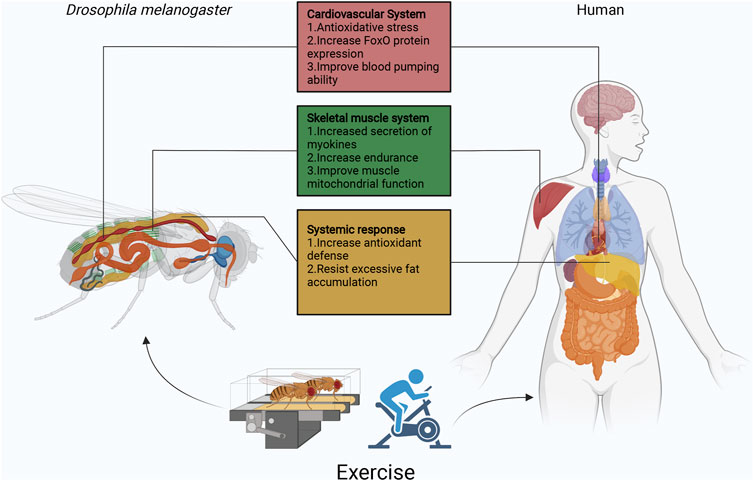
FIGURE 2. Overview of the function of Drosophila exercise in different organs. Currently, Drosophila exercise models have made some achievements in cardiovascular, skeletal muscle, and fat body. Exercise activates the cardiomyocyte dSir2/FoxO/SOD and dSir2/FoxO/bmm pathways to delay cardiac aging. In Sestrins, Sesn1 is mainly expressed in muscle, where it is involved in the metabolic response to exercise and is associated with TORC2-Akt activity and PGC1α. In addition, skeletal muscle secretes myokines (ImpL2) that inhibit insulin signaling and promote mitochondrial autophagy. Exercise though apoLpp to regulate abnormal lipid metabolism, it also activates Nmnat/NAD+/dSir2 to resist lipotoxicity. In exception to the heart, fat body and skeletal muscles, Drosophila has other systems similar to those of humans, such as the central nervous system represented by the Drosophila and human brain, the digestive system represented by the Drosophila and human intestine, the respiratory system represented by the Drosophila thorax and human lungs, and the reproductive system represented by the Drosophila and human ovaries/testes. The effects of exercise on aging individuals are complex, but the use of simple Drosophila exercise models will be exciting for exploring the role of exercise in different biological processes, while facing various difficulties and challenges.
Drosophila exercise models have made remarkable achievements in this decade or so, but there are still some limitations awaiting the development of future methods and tools. Specifically, Drosophila exercise models are currently used primarily to study endurance exercise, but human exercise types also include exercises to improve strength, flexibility, and balance. In addition to increasing endurance, human exercise needs often include improving muscle strength or muscle tone, altering body composition, or increasing flexibility. Therefore, new methods and tools are needed to match Drosophila exercise to human exercise models. Although the Drosophila model has a limited redundancy of conserved pathways, it is still of great value for such studies. Therefore, it is important to further investigate the molecular mechanisms behind the physiological changes in exercise and aging. Furthermore, elucidating the role of exercise-related genes in Drosophila aging would provide evidence for a potential role of their human counterparts in aging (eg Table 1). Drosophila exercise models could provide therapeutic targets for exercise treatment of aging-related diseases. Of course these exercise-related genes need to be validated by extensive experiments before they hold promise as new therapeutic approaches.
Author contributions
MD and HL: Conceptualization, writing–original draft; LZ: Funding acquisition, Supervision, writing–review & editing.
Funding
This research was funded by The Key scientific research project of Hunan Education Department was funded, grant number 19A328, and the National Natural Science Foundation of China, grant number 32071175.
Acknowledgments
We thank Ying Guo, Qiufang Li and Shiyi He for discussions on this manuscript.
Conflict of interest
The authors declare that the research was conducted in the absence of any commercial or financial relationships that could be construed as a potential conflict of interest.
Publisher’s note
All claims expressed in this article are solely those of the authors and do not necessarily represent those of their affiliated organizations, or those of the publisher, the editors and the reviewers. Any product that may be evaluated in this article, or claim that may be made by its manufacturer, is not guaranteed or endorsed by the publisher.
References
Abdellatif, M., Sedej, S., and Kroemer, G. (2021). NAD+ metabolism in cardiac health, aging, and disease. Circulation 144 (22), 1795–1817. doi:10.1161/CIRCULATIONAHA.121.056589
Alam, S., Stolinski, M., Pentecost, C., Boroujerdi, M. A., Jones, R. H., Sonksen, P. H., et al. (2004). The effect of a six-month exercise program on very low-density lipoprotein apolipoprotein B secretion in type 2 diabetes. J. Clin. Endocrinol. Metab. 89 (2), 688–694. doi:10.1210/jc.2003-031036
Anker, S. D., Ponikowski, P., Varney, S., Chua, T. P., Clark, A. L., Webb-Peploe, K. M., et al. (1997). Wasting as independent risk factor for mortality in chronic heart failure. Lancet 349 (9058), 1050–1053. doi:10.1016/S0140-6736(96)07015-8
Arnold, A. S., Egger, A., and Handschin, C. (2011). PGC-1α and myokines in the aging muscle - a mini-review. Gerontology 57 (1), 37–43. doi:10.1159/000281883
Aune, D., Sen, A., Prasad, M., Norat, T., Janszky, I., Tonstad, S., et al. (2016). BMI and all cause mortality: Systematic review and non-linear dose-response meta-analysis of 230 cohort studies with 3.74 million deaths among 30.3 million participants. BMJ 353, i2156. PMID: 27146380; PMCID: PMC4856854. doi:10.1136/bmj.i2156
Batsis, J. A., and Villareal, D. T. (2018). Sarcopenic obesity in older adults: Aetiology, epidemiology and treatment strategies. Nat. Rev. Endocrinol. 14 (9), 513–537. PMID: 30065268; PMCID: PMC6241236. doi:10.1038/s41574-018-0062-9
Bazzell, B., Ginzberg, S., Healy, L., and Wessells, R. J. (2013). Dietary composition regulates Drosophila mobility and cardiac physiology. J. Exp. Biol. 216 (5), 859–868. Epub 2012 Nov 15. PMID: 23155082; PMCID: PMC3571989. doi:10.1242/jeb.078758
Berlandi, J., Lin, F. J., Ambrée, O., Rieger, D., Paulus, W., and Jeibmann, A. (2017). Swing Boat: Inducing and recording locomotor activity in a Drosophila melanogaster model of Alzheimer's disease. Front. Behav. Neurosci. 11, 159. PMID: 28912696; PMCID: PMC5582087. doi:10.3389/fnbeh.2017.00159
Birse, R. T., Choi, J., Reardon, K., Rodriguez, J., Graham, S., Diop, S., et al. (2010). High-fat-diet-induced obesity and heart dysfunction are regulated by the TOR pathway in Drosophila. Cell Metab. 12 (5), 533–544. PMID: 21035763; PMCID: PMC3026640. doi:10.1016/j.cmet.2010.09.014
Blice-Baum, A. C., Guida, M. C., Hartley, P. S., Adams, P. D., Bodmer, R., and Cammarato, A. (2019). As time flies by: Investigating cardiac aging in the short-lived Drosophila model. Biochim. Biophys. Acta Mol. Basis Dis. 1865 (7), 1831–1844. Epub 2018 Nov 27. PMID: 30496794; PMCID: PMC6527462. doi:10.1016/j.bbadis.2018.11.010
Börzsei, D., Priksz, D., Szabó, R., Bombicz, M., Karácsonyi, Z., Puskás, L. G., et al. (2021). Exercise-mitigated sex-based differences in aging: From genetic alterations to heart performance. Am. J. Physiol. Heart Circ. Physiol. 320 (2), H854–H866. Epub 2020 Dec 18. PMID: 33337964. doi:10.1152/ajpheart.00643.2020
Campisi, J., Kapahi, P., Lithgow, G. J., Melov, S., Newman, J. C., and Verdin, E. (2019). From discoveries in ageing research to therapeutics for healthy ageing. Nature 571 (7764), 183–192. Epub 2019 Jul 10. PMID: 31292558; PMCID: PMC7205183. doi:10.1038/s41586-019-1365-2
Cantó, C., Jiang, L. Q., Deshmukh, A. S., Mataki, C., Coste, A., Lagouge, M., et al. (2010). Interdependence of AMPK and SIRT1 for metabolic adaptation to fasting and exercise in skeletal muscle. Cell Metab. 11 (3), 213–219. PMID: 20197054; PMCID: PMC3616265. doi:10.1016/j.cmet.2010.02.006
Cartee, G. D., Hepple, R. T., Bamman, M. M., and Zierath, J. R. (2016). Exercise promotes healthy aging of skeletal muscle. Cell Metab. 23 (6), 1034–1047. PMID: 27304505; PMCID: PMC5045036. doi:10.1016/j.cmet.2016.05.007
Castaño, C., Mirasierra, M., Vallejo, M., Novials, A., and Párrizas, M. (2020). Delivery of muscle-derived exosomal miRNAs induced by HIIT improves insulin sensitivity through down-regulation of hepatic FoxO1 in mice. Proc. Natl. Acad. Sci. U. S. A. 117 (48), 30335–30343. Epub 2020 Nov 16. PMID: 33199621; PMCID: PMC7720135. doi:10.1073/pnas.2016112117
Cattadori, G., Segurini, C., Picozzi, A., Padeletti, L., and Anzà, C. (2018). Exercise and heart failure: An update. Esc. Heart Fail. 5 (2), 222–232. Epub 2017 Dec 13. PMID: 29235244; PMCID: PMC5880674. doi:10.1002/ehf2.12225
Chadda, K. R., Ajijola, O. A., Vaseghi, M., Shivkumar, K., Huang, C. L., and Jeevaratnam, K. (2018). Ageing, the autonomic nervous system and arrhythmia: From brain to heart. Ageing Res. Rev. 48, 40–50. Epub 2018 Oct 6. PMID: 30300712. doi:10.1016/j.arr.2018.09.005
Cheedipudi, S. M., Hu, J., Fan, S., Yuan, P., Karmouch, J., Czernuszewicz, G., et al. (2020). Exercise restores dysregulated gene expression in a mouse model of arrhythmogenic cardiomyopathy. Cardiovasc Res. 116 (6), 1199–1213. PMID: 31350552; PMCID: PMC7177479. doi:10.1093/cvr/cvz199
Christian, C. J., and Benian, G. M. (2020). Animal models of sarcopenia. Aging Cell 19 (10), e13223. Epub 2020 Aug 28. PMID: 32857472; PMCID: PMC7576270. doi:10.1111/acel.13223
Copeland, J. M., Cho, J., Lo, T., Hur, J. H., Bahadorani, S., Arabyan, T., et al. (2009). Extension of Drosophila life span by RNAi of the mitochondrial respiratory chain. Curr. Biol. 19 (19), 1591–1598. Epub 2009 Sep 10. PMID: 19747824. doi:10.1016/j.cub.2009.08.016
Dahleh, M. M. M., Araujo, S. M., Bortolotto, V. C., Pinheiro, F. C., Poetini, M. R., Musachio, E. A. S., et al. (2021). Exercise associated with γ-oryzanol supplementation suppresses oxidative stress and prevents changes in locomotion in Drosophila melanogaster. Free Radic. Res. 55 (2), 198–209. Epub 2021 May 18. PMID: 33655816. doi:10.1080/10715762.2021.1895992
Damschroder, D., Richardson, K., Cobb, T., and Wessells, R. (2020). The effects of genetic background on exercise performance in Drosophila. Fly. (Austin) 14 (1-4), 80–92. PMID: 33100141; PMCID: PMC7714460. doi:10.1080/19336934.2020.1835329
Demontis, F., and Perrimon, N. (2010). FOXO/4E-BP signaling in Drosophila muscles regulates organism-wide proteostasis during aging. Cell 143 (5), 813–825. PMID: 21111239; PMCID: PMC3066043. doi:10.1016/j.cell.2010.10.007
Demontis, F., Piccirillo, R., Goldberg, A. L., and Perrimon, N. (2013). Mechanisms of skeletal muscle aging: Insights from Drosophila and mammalian models. Dis. Model. Mech. 6 (6), 1339–1352. Epub 2013 Oct 2. PMID: 24092876; PMCID: PMC3820258. doi:10.1242/dmm.012559
Demontis, F., Piccirillo, R., Goldberg, A. L., and Perrimon, N. (2013). The influence of skeletal muscle on systemic aging and lifespan. Aging Cell 12 (6), 943–949. Epub 2013 Jul 17. PMID: 23802635; PMCID: PMC3838468. doi:10.1111/acel.12126
Dillin, A., Hsu, A. L., Arantes-Oliveira, N., Lehrer-Graiwer, J., Hsin, H., Fraser, A. G., et al. (2002). Rates of behavior and aging specified by mitochondrial function during development. Science 298 (5602), 2398–2401. Epub 2002 Dec 5. PMID: 12471266. doi:10.1126/science.1077780
Ding, M., Zheng, L., Li, Q. F., Wang, W. L., Peng, W. D., and Zhou, M. (2021). Exercise-training regulates apolipoprotein B in Drosophila to improve HFD-mediated cardiac function damage and low exercise capacity. Front. Physiol. 12, 650959. PMID: 34305631; PMCID: PMC8294119. doi:10.3389/fphys.2021.650959
Diop, S. B., and Bodmer, R. (2015). Gaining insights into diabetic cardiomyopathy from Drosophila. Trends Endocrinol. Metab. 26 (11), 618–627. Epub 2015 Oct 16. PMID: 26482877; PMCID: PMC4638170. doi:10.1016/j.tem.2015.09.009
Ebanks, B., Ingram, T. L., Katyal, G., Ingram, J. R., Moisoi, N., and Chakrabarti, L. (2021). The dysregulated Pink1- Drosophila mitochondrial proteome is partially corrected with exercise. Aging (Albany NY) 13 (11), 14709–14728. Epub 2021 Jun 1. PMID: 34074800; PMCID: PMC8221352. doi:10.18632/aging.203128
Fang, E. F., Hou, Y., Lautrup, S., Jensen, M. B., Yang, B., SenGupta, T., et al. (2019). NAD+ augmentation restores mitophagy and limits accelerated aging in Werner syndrome. Nat. Commun. 10 (1), 5284. PMID: 31754102; PMCID: PMC6872719. doi:10.1038/s41467-019-13172-8
Farooqui, T. (2007). Octopamine-mediated neuromodulation of insect senses. Neurochem. Res. 32 (9), 1511–1529. Epub 2007 May 5. PMID: 17484052. doi:10.1007/s11064-007-9344-7
Feany, M. B., and Bender, W. W. (2000). A Drosophila model of Parkinson's disease. Nature 404 (6776), 394–398. doi:10.1038/35006074
Ferrara, N., Rinaldi, B., Corbi, G., Conti, V., Stiuso, P., Boccuti, S., et al. (2008). Exercise training promotes SIRT1 activity in aged rats. Rejuvenation Res. 11 (1), 139–150. doi:10.1089/rej.2007.0576
Fiuza-Luces, C., Santos-Lozano, A., Joyner, M., Carrera-Bastos, P., Picazo, O., Zugaza, J. L., et al. (2018). Exercise benefits in cardiovascular disease: Beyond attenuation of traditional risk factors. Nat. Rev. Cardiol. 15 (12), 731–743. doi:10.1038/s41569-018-0065-1
Gabriel, B. M., and Zierath, J. R. (2019). Circadian rhythms and exercise - re-setting the clock in metabolic disease. Nat. Rev. Endocrinol. 15 (4), 197–206. PMID: 30655625. doi:10.1038/s41574-018-0150-x
Gargano, J. W., Martin, I., Bhandari, P., and Grotewiel, M. S. (2005). Rapid iterative negative geotaxis (RING): A new method for assessing age-related locomotor decline in Drosophila. Exp. Gerontol. 40 (5), 386–395. Epub 2005 Mar 19. PMID: 15919590. doi:10.1016/j.exger.2005.02.005
Global BMI Mortality Collaboration Di Angelantonio, E., Bhupathiraju, S., Wormser, D., Gao, P., Kaptoge, S., Berrington de Gonzalez, A., et al. (2016). Body-mass index and all-cause mortality: Individual-participant-data meta-analysis of 239 prospective studies in four continents. Lancet 388 (10046), 776–786. Epub 2016 Jul 13. PMID: 27423262; PMCID: PMC4995441. doi:10.1016/S0140-6736(16)30175-1
Goenka, S., and Lee, I. M. (2017). Physical activity lowers mortality and heart disease risks. Lancet 390 (10113), 2609–2610. Epub 2017 Sep 21. PMID: 28943265. doi:10.1016/S0140-6736(17)32104-9
Goodyear, L. J., and Kahn, B. B. (1998). Exercise, glucose transport, and insulin sensitivity. Annu. Rev. Med. 49, 235–261. PMID: 9509261. doi:10.1146/annurev.med.49.1.235
Griswold, A. J., Chang, K. T., Runko, A. P., Knight, M. A., and Min, K. T. (2008). Sir2 mediates apoptosis through JNK-dependent pathways in Drosophila. Proc. Natl. Acad. Sci. U. S. A. 105 (25), 8673–8678. Epub 2008 Jun 17. PMID: 18562277; PMCID: PMC2438412. doi:10.1073/pnas.0803837105
Grotewiel, M. S., Martin, I., Bhandari, P., and Cook-Wiens, E. (2005). Functional senescence in Drosophila melanogaster. Ageing Res. Rev. 4 (3), 372–397. PMID: 16024299. doi:10.1016/j.arr.2005.04.001
Harris, J. E., Pinckard, K. M., Wright, K. R., Baer, L. A., Arts, P. J., Abay, E., et al. (2020). Exercise-induced 3'-sialyllactose in breast milk is a critical mediator to improve metabolic health and cardiac function in mouse offspring. Nat. Metab. 2 (8), 678–687. Epub 2020 Jun 29. PMID: 32694823; PMCID: PMC7438265. doi:10.1038/s42255-020-0223-8
Hawley, J. A., Hargreaves, M., Joyner, M. J., and Zierath, J. R. (2014). Integrative biology of exercise. Cell 159 (4), 738–749. doi:10.1016/j.cell.2014.10.029
Hawley, J. A., and Krook, A. (2016). Metabolism: One step forward for exercise. Nat. Rev. Endocrinol. 12 (1), 7–8. Epub 2015 Nov 27. PMID: 26610411. doi:10.1038/nrendo.2015.201
Hede, M. S., Salimova, E., Piszczek, A., Perlas, E., Winn, N., Nastasi, T., et al. (2012). E-peptides control bioavailability of IGF-1. PLoS One 7 (12), e51152. Epub 2012 Dec 10. PMID: 23251442; PMCID: PMC3519493. doi:10.1371/journal.pone.0051152
Helfand, S. L., and Rogina, B. (2003). Genetics of aging in the fruit fly, Drosophila melanogaster. Annu. Rev. Genet. 37, 329–348. PMID: 14616064. doi:10.1146/annurev.genet.37.040103.095211
Hermann, C., Yoshii, T., Dusik, V., and Helfrich-Förster, C. (2012). Neuropeptide F immunoreactive clock neurons modify evening locomotor activity and free-running period in Drosophila melanogaster. J. Comp. Neurol. 520 (5), 970–987. PMID: 21826659. doi:10.1002/cne.22742
Hou, Y., Dan, X., Babbar, M., Wei, Y., Hasselbalch, S. G., Croteau, D. L., et al. (2019). Ageing as a risk factor for neurodegenerative disease. Nat. Rev. Neurol. 15 (10), 565–581. Epub 2019 Sep 9. PMID: 31501588. doi:10.1038/s41582-019-0244-7
Houghton, D., Thoma, C., Hallsworth, K., Cassidy, S., Hardy, T., Burt, A. D., et al. (2017). Exercise reduces liver lipids and visceral adiposity in patients with nonalcoholic steatohepatitis in a randomized controlled trial. Clin. Gastroenterol. Hepatol. 15 (1), 96–102. e3. Epub 2016 Aug 10. PMID: 27521509; PMCID: PMC5196006. doi:10.1016/j.cgh.2016.07.031
Huang, T., Jian, X., Liu, J., Zheng, L., Li, F. Q., Meng, D., et al. (2022). Exercise and/or cold exposure alters the gene expression profile in the fat body and changes the heart function in Drosophila. Front. Endocrinol. (Lausanne). 13, 790414. PMID: 35418948; PMCID: PMC8995477. doi:10.3389/fendo.2022.790414
Hunt, L. C., Schadeberg, B., Stover, J., Haugen, B., Pagala, V., Wang, Y. D., et al. (2021). Antagonistic control of myofiber size and muscle protein quality control by the ubiquitin ligase UBR4 during aging. Nat. Commun. 12 (1), 1418. PMID: 33658508; PMCID: PMC7930053. doi:10.1038/s41467-021-21738-8
Kamikouchi, A., Inagaki, H. K., Effertz, T., Hendrich, O., Fiala, A., Göpfert, M. C., et al. (2009). The neural basis of Drosophila gravity-sensing and hearing. Nature 458 (7235), 165–171. doi:10.1038/nature07810
Kang, E. B., Kwon, I. S., Koo, J. H., Kim, E. J., Kim, C. H., Lee, J., et al. (2013). Treadmill exercise represses neuronal cell death and inflammation during Aβ-induced ER stress by regulating unfolded protein response in aged presenilin 2 mutant mice. Apoptosis 18 (11), 1332–1347. doi:10.1007/s10495-013-0884-9
Katzenberger, R. J., Loewen, C. A., Wassarman, D. R., Petersen, A. J., Ganetzky, B., and Wassarman, D. A. (2013). A Drosophila model of closed head traumatic brain injury. Proc. Natl. Acad. Sci. U. S. A. 110 (44), E4152–E4159. Epub 2013 Oct 14. PMID: 24127584; PMCID: PMC3816429. doi:10.1073/pnas.1316895110
Kim, M., Sujkowski, A., Namkoong, S., Gu, B., Cobb, T., Kim, B., et al. (2020). Sestrins are evolutionarily conserved mediators of exercise benefits. Nat. Commun. 11 (1), 190. PMID: 31929512; PMCID: PMC6955242. doi:10.1038/s41467-019-13442-5
Kirchman, P. A., Kim, S., Lai, C. Y., and Jazwinski, S. M. (1999). Interorganelle signaling is a determinant of longevity in Saccharomyces cerevisiae. Genetics 152 (1), 179–190. PMID: 10224252; PMCID: PMC1460582. doi:10.1093/genetics/152.1.179
Koltai, E., Szabo, Z., Atalay, M., Boldogh, I., Naito, H., Goto, S., et al. (2010). Exercise alters SIRT1, SIRT6, NAD and NAMPT levels in skeletal muscle of aged rats. Mech. Ageing Dev. 131 (1), 21–28. Epub 2009 Nov 12. PMID: 19913571; PMCID: PMC2872991. doi:10.1016/j.mad.2009.11.002
Kurgan, N., Noaman, N., Pergande, M. R., Cologna, S. M., Coorssen, J. R., and Klentrou, P. (2019). Changes to the human serum proteome in response to high intensity interval exercise: A sequential top-down proteomic analysis. Front. Physiol. 10, 362. PMID: 31001142; PMCID: PMC6454028. doi:10.3389/fphys.2019.00362
Laiteerapong, N., Ham, S. A., Gao, Y., Moffet, H. H., Liu, J. Y., Huang, E. S., et al. (2019). The legacy effect in type 2 diabetes: Impact of early glycemic control on future complications (the diabetes & aging study). Diabetes Care 42 (3), 416–426. Epub 2018 Aug 13. PMID: 30104301; PMCID: PMC6385699. doi:10.2337/dc17-1144
Lavie, C. J., Arena, R., Swift, D. L., Johannsen, N. M., Sui, X., Lee, D. C., et al. (2015). Exercise and the cardiovascular system: Clinical science and cardiovascular outcomes. Circ. Res. 117 (2), 207–219. PMID: 26139859; PMCID: PMC4493772. doi:10.1161/CIRCRESAHA.117.305205
Li, H., Hastings, M. H., Rhee, J., Trager, L. E., Roh, J. D., and Rosenzweig, A. (2020). Targeting age-related pathways in heart failure. Circ. Res. 126 (4), 533–551. Epub 2020 Feb 13. PMID: 32078451; PMCID: PMC7041880. doi:10.1161/CIRCRESAHA.119.315889
Li, Q. F., Wang, H., Zheng, L., Yang, F., Li, H. Z., Li, J. X., et al. (2020). Effects of modest hypoxia and exercise on cardiac function, sleep-activity, negative geotaxis behavior of aged female Drosophila. Front. Physiol. 10, 1610. PMID: 32038290; PMCID: PMC6985434. doi:10.3389/fphys.2019.01610
Liu, X., Jiang, N., Hughes, B., Bigras, E., Shoubridge, E., and Hekimi, S. (2005). Evolutionary conservation of the clk-1-dependent mechanism of longevity: Loss of mclk1 increases cellular fitness and lifespan in mice. Genes Dev. 19 (20), 2424–2434. Epub 2005 Sep 29. PMID: 16195414; PMCID: PMC1257397. doi:10.1101/gad.1352905
Lovatel, G. A., Elsner, V. R., Bertoldi, K., Vanzella, C., Moysés Fdos, S., Vizuete, A., et al. (2013). Treadmill exercise induces age-related changes in aversive memory, neuroinflammatory and epigenetic processes in the rat hippocampus. Neurobiol. Learn. Mem. 101, 94–102. Epub 2013 Jan 26. PMID: 23357282. doi:10.1016/j.nlm.2013.01.007
Lowman, K. E., Wyatt, B. J., Cunneely, O. P., and Reed, L. K. (2018). The TreadWheel: Interval training protocol for gently induced exercise in Drosophila melanogaster. J. Vis. Exp. 27 (135), 57788. PMID: 29939171; PMCID: PMC6101642. doi:10.3791/57788
Lu, B., and Vogel, H. (2009). Drosophila models of neurodegenerative diseases. Annu. Rev. Pathol. 4, 315–342. PMID: 18842101; PMCID: PMC3045805. doi:10.1146/annurev.pathol.3.121806.151529
Luong, N., Davies, C. R., Wessells, R. J., Graham, S. M., King, M. T., Veech, R., et al. (2006). Activated FOXO-mediated insulin resistance is blocked by reduction of TOR activity. Cell Metab. 4 (2), 133–142. PMID: 16890541. doi:10.1016/j.cmet.2006.05.013
Makarova, K. S., Wolf, Y. I., Alkhnbashi, O. S., Costa, F., Shah, S. A., Saunders, S. J., et al. (2015). An updated evolutionary classification of CRISPR-Cas systems. Nat. Rev. Microbiol. 13 (11), 722–736. Epub 2015 Sep. PMID: 26411297; PMCID: PMC5426118. doi:10.1038/nrmicro3569
McGee, S. L., and Hargreaves, M. (2020). Exercise adaptations: Molecular mechanisms and potential targets for therapeutic benefit. Nat. Rev. Endocrinol. 16 (9), 495–505. Epub 2020 Jul 6. PMID: 32632275. doi:10.1038/s41574-020-0377-1
Meeusen, R., and De Meirleir, K. (1995). Exercise and brain neurotransmission. Sports Med. 20 (3), 160–188. PMID: 8571000. doi:10.2165/00007256-199520030-00004
Mendez, S., Watanabe, L., Hill, R., Owens, M., Moraczewski, J., Rowe, G. C., et al. (2016). The TreadWheel: A novel apparatus to measure genetic variation in response to gently induced exercise for Drosophila. PLoS One 11 (10), e0164706. PMID: 27736996; PMCID: PMC5063428. doi:10.1371/journal.pone.0164706
Metter, E. J., Talbot, L. A., Schrager, M., and Conwit, R. (2002). Skeletal muscle strength as a predictor of all-cause mortality in healthy men. J. Gerontol. A Biol. Sci. Med. Sci. 57 (10), B359–B365. PMID: 12242311. doi:10.1093/gerona/57.10.b359
Miquel, J., Lundgren, P. R., Bensch, K. G., and Atlan, H. (1976). Effects of temperature on the life span, vitality and fine structure of Drosophila melanogaster. Mech. Ageing Dev. 5 (5), 347–370. PMID: 823384. doi:10.1016/0047-6374(76)90034-8
Monteiro-Junior, R. S., Cevada, T., Oliveira, B. R., Lattari, E., Portugal, E. M., Carvalho, A., et al. (2015). We need to move more: Neurobiological hypotheses of physical exercise as a treatment for Parkinson's disease. Med. Hypotheses 85 (5), 537–541. Epub 2015 Jul 17. PMID: 26209418. doi:10.1016/j.mehy.2015.07.011
Murphy, L. B., Santos-Ledo, A., Dhanaseelan, T., Eley, L., Burns, D., Henderson, D. J., et al. (2021). Exercise, programmed cell death and exhaustion of cardiomyocyte proliferation in aging zebrafish. Dis. Model Mech. 14 (7), dmm049013. Epub 2021 Jul 22. PMID: 34296752; PMCID: PMC8319546. doi:10.1242/dmm.049013
Musselman, L. P., and Kühnlein, R. P. (2018). Drosophila as a model to study obesity and metabolic disease. J. Exp. Biol. 221 (1), jeb163881. doi:10.1242/jeb.163881
Myint, P. K., and Welch, A. A. (2012). Healthier ageing. BMJ 344, e1214. PMID: 22411918. doi:10.1136/bmj.e1214
North, B. J., and Sinclair, D. A. (2012). The intersection between aging and cardiovascular disease. Circ. Res. 110 (8), 1097–1108. PMID: 22499900; PMCID: PMC3366686. doi:10.1161/CIRCRESAHA.111.246876
Oppert, J. M., Bellicha, A., and Ciangura, C. (2021). Physical activity in management of persons with obesity. Eur. J. Intern. Med. 93, 8–12. Epub 2021 May 21. PMID: 34024703. doi:10.1016/j.ejim.2021.04.028
Ouwens, D. M., Boer, C., Fodor, M., de Galan, P., Heine, R. J., Maassen, J. A., et al. (2005). Cardiac dysfunction induced by high-fat diet is associated with altered myocardial insulin signalling in rats. Diabetologia 48 (6), 1229–1237. Epub 2005 Apr 30. PMID: 15864533. doi:10.1007/s00125-005-1755-x
Owusu-Ansah, E., Song, W., and Perrimon, N. (2013). Muscle mitohormesis promotes longevity via systemic repression of insulin signaling. Cell 155 (3), 699–712. Epub 2013 Oct 24. PMID: 24243023; PMCID: PMC3856681. doi:10.1016/j.cell.2013.09.021
Paneni, F., Diaz Cañestro, C., Libby, P., Lüscher, T. F., and Camici, G. G. (2017). The aging cardiovascular system: Understanding it at the cellular and clinical levels. J. Am. Coll. Cardiol. 69 (15), 1952–1967. PMID: 28408026. doi:10.1016/j.jacc.2017.01.064
Partridge, L., Deelen, J., and Slagboom, P. E. (2018). Facing up to the global challenges of ageing. Nature 561 (7721), 45–56. Epub 2018 Sep 5. PMID: 30185958. doi:10.1038/s41586-018-0457-8
Pedersen, B. K., and Febbraio, M. A. (2012). Muscles, exercise and obesity: Skeletal muscle as a secretory organ. Nat. Rev. Endocrinol. 8 (8), 457–465. doi:10.1038/nrendo.2012.49
Piazza, N., Gosangi, B., Devilla, S., Arking, R., and Wessells, R. (2009). Exercise-training in young Drosophila melanogaster reduces age-related decline in mobility and cardiac performance. PLoS One 4 (6), e5886. PMID: 19517023; PMCID: PMC2691613. doi:10.1371/journal.pone.0005886
Piccirillo, R., Demontis, F., Perrimon, N., and Goldberg, A. L. (2014). Mechanisms of muscle growth and atrophy in mammals and Drosophila. Dev. Dyn. 243 (2), 201–215. Epub 2013 Oct 24. PMID: 24038488; PMCID: PMC3980484. doi:10.1002/dvdy.24036
Pilegaard, H., Saltin, B., and Neufer, P. D. (2003). Exercise induces transient transcriptional activation of the PGC-1alpha gene in human skeletal muscle. J. Physiol. 546 (3), 851–858. PMID: 12563009; PMCID: PMC2342594. doi:10.1113/jphysiol.2002.034850
Rai, M., Coleman, Z., Curley, M., Nityanandam, A., Platt, A., Robles-Murguia, M., et al. (2021). Proteasome stress in skeletal muscle mounts a long-range protective response that delays retinal and brain aging. Cell Metab. 33 (6), 1137–1154. e9. Epub 2021 Mar 26. PMID: 33773104; PMCID: PMC8172468. doi:10.1016/j.cmet.2021.03.005
Rakshit, K., Wambua, R., Giebultowicz, T. M., and Giebultowicz, J. M. (2013). Effects of exercise on circadian rhythms and mobility in aging Drosophila melanogaster. Exp. Gerontol. 48 (11), 1260–1265. Epub 2013 Aug 2. PMID: 23916842; PMCID: PMC3798010. doi:10.1016/j.exger.2013.07.013
Risom, S. S., Zwisler, A. D., Johansen, P. P., Sibilitz, K. L., Lindschou, J., Gluud, C., et al. (2017). Exercise-based cardiac rehabilitation for adults with atrial fibrillation. Cochrane Database Syst. Rev. 2 (2), CD011197. pub2. PMID: 28181684; PMCID: PMC6464537. doi:10.1002/14651858.CD011197
Rognmo, Ø., Moholdt, T., Bakken, H., Hole, T., Mølstad, P., Myhr, N. E., et al. (2012). Cardiovascular risk of high- versus moderate-intensity aerobic exercise in coronary heart disease patients. Circulation 126 (12), 1436–1440. Epub 2012 Aug 9. PMID: 22879367. doi:10.1161/CIRCULATIONAHA.112.123117
Rosenberg, M. I., and Parkhurst, S. M. (2002). Drosophila Sir2 is required for heterochromatic silencing and by euchromatic Hairy/E(Spl) bHLH repressors in segmentation and sex determination. Cell 109 (4), 447–458. PMID: 12086602. doi:10.1016/s0092-8674(02)00732-8
Ruiz, J. R., Morán, M., Arenas, J., and Lucia, A. (2011). Strenuous endurance exercise improves life expectancy: it's in our genes. Br. J. Sports Med. 45 (3), 159–161. Epub 2010 Sep 27. PMID: 20876590. doi:10.1136/bjsm.2010.075085
Ruiz, J. R., Sui, X., Lobelo, F., Morrow, J. R., Jackson, A. W., Sjöström, M., et al. (2008). Association between muscular strength and mortality in men: Prospective cohort study. BMJ 337 (7661), a439. PMID: 18595904; PMCID: PMC2453303. doi:10.1136/bmj.a439
Ryu, D., Zhang, H., Ropelle, E. R., Sorrentino, V., Mázala, D. A., Mouchiroud, L., et al. (2016). NAD+ repletion improves muscle function in muscular dystrophy and counters global PARylation. Sci. Transl. Med. 8 (361), 361ra139. PMID: 27798264; PMCID: PMC5535761. doi:10.1126/scitranslmed.aaf5504
Safdar, A., Bourgeois, J. M., Ogborn, D. I., Little, J. P., Hettinga, B. P., Akhtar, M., et al. (2011). Endurance exercise rescues progeroid aging and induces systemic mitochondrial rejuvenation in mtDNA mutator mice. Proc. Natl. Acad. Sci. U. S. A. 108 (10), 4135–4140. Epub 2011 Feb 22. PMID: 21368114; PMCID: PMC3053975. doi:10.1073/pnas.1019581108
Santos, A. L., and Sinha, S. (2021). Obesity and aging: Molecular mechanisms and therapeutic approaches. Ageing Res. Rev. 67, 101268. Epub 2021 Feb 5. PMID: 33556548. doi:10.1016/j.arr.2021.101268
Schaffer, J. E. (2003). Lipotoxicity: When tissues overeat. Curr. Opin. Lipidol. 14 (3), 281–287. PMID: 12840659. doi:10.1097/00041433-200306000-00008
Schrauwen-Hinderling, V. B., Hesselink, M. K., Meex, R., van der Made, S., Schär, M., Lamb, H., et al. (2010). Improved ejection fraction after exercise training in obesity is accompanied by reduced cardiac lipid content. J. Clin. Endocrinol. Metab. 95 (4), 1932–1938. Epub 2010 Feb 19. PMID: 20173015. doi:10.1210/jc.2009-2076
Serra, F. T., Carvalho, A. D., Araujo, B. H. S., Torres, L. B., Cardoso, F. D. S., Henrique, J. S., et al. (2019). Early exercise induces long-lasting morphological changes in cortical and hippocampal neurons throughout of a sedentary period of rats. Sci. Rep. 9 (1), 13684. PMID: 31548605; PMCID: PMC6757043. doi:10.1038/s41598-019-50218-9
Shaw, P. J., Cirelli, C., Greenspan, R. J., and Tononi, G. (2000). Correlates of sleep and waking in Drosophila melanogaster. Science 287 (5459), 1834–1837. PMID: 10710313. doi:10.1126/science.287.5459.1834
Shimizu, H., Shimoda, M., Yamaguchi, T., Seong, K. H., Okamura, T., and Ishii, S. (2008). Drosophila ATF-2 regulates sleep and locomotor activity in pacemaker neurons. Mol. Cell. Biol. 28 (20), 6278–6289. Epub 2008 Aug 11. PMID: 18694958; PMCID: PMC2577423. doi:10.1128/MCB.02242-07
Silverman, L. A., Cheng, Z. Q., Hsiao, D., and Rosenthal, S. M. (1995). Skeletal muscle cell-derived insulin-like growth factor (IGF) binding proteins inhibit IGF-I-induced myogenesis in rat L6E9 cells. Endocrinology 136 (2), 720–726. doi:10.1210/endo.136.2.7530651
Sujkowski, A., Gretzinger, A., Soave, N., Todi, S. V., and Wessells, R. (2020). Alpha- and beta-adrenergic octopamine receptors in muscle and heart are required for Drosophila exercise adaptations. PLoS Genet. 16 (6), e1008778. PMID: 32579604; PMCID: PMC7351206. doi:10.1371/journal.pgen.1008778
Sujkowski, A., Ramesh, D., Brockmann, A., and Wessells, R. (2017). Octopamine drives endurance exercise adaptations in Drosophila. Cell Rep. 21 (7), 1809–1823. PMID: 29141215; PMCID: PMC5693351. doi:10.1016/j.celrep.2017.10.065
Sujkowski, A., Richardson, K., Prifti, M. V., Wessells, R. J., and Todi, S. V. (2022). Endurance exercise ameliorates phenotypes in Drosophila models of spinocerebellar ataxias. Elife 11, e75389. PMID: 35170431; PMCID: PMC8871352. doi:10.7554/eLife.75389
Sujkowski, A., Saunders, S., Tinkerhess, M., Piazza, N., Jennens, J., Healy, L., et al. (2012). dFatp regulates nutrient distribution and long-term physiology in Drosophila. Aging Cell 11 (6), 921–932. Epub 2012 Aug 27. PMID: 22809097; PMCID: PMC3533766. doi:10.1111/j.1474-9726.2012.00864.x
Sujkowski, A., Spierer, A. N., Rajagopalan, T., Bazzell, B., Safdar, M., Imsirovic, D., et al. (2019). Mito-nuclear interactions modify Drosophila exercise performance. Mitochondrion 47, 188–205. Epub 2018 Nov 6. PMID: 30408593; PMCID: PMC7035791. doi:10.1016/j.mito.2018.11.005
Sujkowski, A., and Wessells, R. (2021). Exercise and sestrin mediate speed and lysosomal activity in Drosophila by partially overlapping mechanisms. Cells 10 (9), 2479. PMID: 34572128; PMCID: PMC8466685. doi:10.3390/cells10092479
Sun, Y., Liu, L., Ben-Shahar, Y., Jacobs, J. S., Eberl, D. F., and Welsh, M. J. (2009). TRPA channels distinguish gravity sensing from hearing in Johnston's organ. Proc. Natl. Acad. Sci. U. S. A. 106 (32), 13606–13611. Epub 2009 Jul 28. PMID: 19666538; PMCID: PMC2717111. doi:10.1073/pnas.0906377106
Swift, D. L., McGee, J. E., Earnest, C. P., Carlisle, E., Nygard, M., and Johannsen, N. M. (2018). The effects of exercise and physical activity on weight loss and maintenance. Prog. Cardiovasc. Dis. 61 (2), 206–213. Epub 2018 Jul 9. PMID: 30003901. doi:10.1016/j.pcad.2018.07.014
Tinkerhess, M. J., Healy, L., Morgan, M., Sujkowski, A., Matthys, E., Zheng, L., et al. (2012). The Drosophila PGC-1α homolog spargel modulates the physiological effects of endurance exercise. PLoS One 7 (2), e31633. Epub 2012 Feb 13. PMID: 22348115; PMCID: PMC3278454. doi:10.1371/journal.pone.0031633
Umpierre, D., Ribeiro, P. A., Kramer, C. K., Leitão, C. B., Zucatti, A. T., Azevedo, M. J., et al. (2011). Physical activity advice only or structured exercise training and association with HbA1c levels in type 2 diabetes: A systematic review and meta-analysis. JAMA 305 (17), 1790–1799. doi:10.1001/jama.2011.576
Unger, R. H. (2003). Minireview: Weapons of lean body mass destruction: The role of ectopic lipids in the metabolic syndrome. Endocrinology 144 (12), 5159–5165. Epub 2003 Sep 4. PMID: 12960011. doi:10.1210/en.2003-0870
van Praag, H., Shubert, T., Zhao, C., and Gage, F. H. (2005). Exercise enhances learning and hippocampal neurogenesis in aged mice. J. Neurosci. 25 (38), 8680–8685. PMID: 16177036; PMCID: PMC1360197. doi:10.1523/JNEUROSCI.1731-05.2005
Villareal, D. T., Aguirre, L., Gurney, A. B., Waters, D. L., Sinacore, D. R., Colombo, E., et al. (2017). Aerobic or resistance exercise, or both, in dieting obese older adults. N. Engl. J. Med. 376 (20), 1943–1955. PMID: 28514618; PMCID: PMC5552187. doi:10.1056/NEJMoa1616338
Vinciguerra, M., Musaro, A., and Rosenthal, N. (2010). Regulation of muscle atrophy in aging and disease. Adv. Exp. Med. Biol. 694, 211–233. PMID: 20886766. doi:10.1007/978-1-4419-7002-2_15
Viru, A. (1992). Plasma hormones and physical exercise. Int. J. Sports Med. 13 (3), 201–209. doi:10.1055/s-2007-1021254
Vujic, A., Lerchenmüller, C., Wu, T. D., Guillermier, C., Rabolli, C. P., Gonzalez, E., et al. (2018). Exercise induces new cardiomyocyte generation in the adult mammalian heart. Nat. Commun. 9 (1), 1659. PMID: 29695718; PMCID: PMC5916892. doi:10.1038/s41467-018-04083-1
Watanabe, L. P., and Riddle, N. C. (2017). Characterization of the rotating exercise quantification system (REQS), a novel Drosophila exercise quantification apparatus. PLoS One 12 (10), e0185090. PMID: 29016615; PMCID: PMC5634558. doi:10.1371/journal.pone.0185090
Watanabe, L. P., and Riddle, N. C. (2021). GWAS reveal a role for the central nervous system in regulating weight and weight change in response to exercise. Sci. Rep. 11 (1), 5144. PMID: 33664357; PMCID: PMC7933348. doi:10.1038/s41598-021-84534-w
Watanabe, L. P., and Riddle, N. C. (2018). Measuring exercise levels in Drosophila melanogaster using the rotating exercise quantification system (REQS). J. Vis. Exp. 8 (136), 57788. PMID: 29889199; PMCID: PMC6101427. doi:10.3791/57751
Waters, D. L., Ward, A. L., and Villareal, D. T. (2013). Weight loss in obese adults 65years and older: A review of the controversy. Exp. Gerontol. 48 (10), 1054–1061. Epub 2013 Feb 10. PMID: 23403042; PMCID: PMC3714333. doi:10.1016/j.exger.2013.02.005
Wen, D. T., Wang, W. Q., Hou, W. Q., Cai, S. X., and Zhai, S. S. (2020). Endurance exercise protects aging Drosophila from high-salt diet (HSD)-induced climbing capacity decline and lifespan decrease by enhancing antioxidant capacity. Biol. Open 9 (5), bio045260. PMID: 32414766; PMCID: PMC7272356. doi:10.1242/bio.045260
Wen, D. T., Zheng, L., Li, J. X., Cheng, D., Liu, Y., Lu, K., et al. (2019). Endurance exercise resistance to lipotoxic cardiomyopathy is associated with cardiac NAD+/dSIR2/PGC-1α pathway activation in old Drosophila. Biol. Open 8 (10), bio044719. PMID: 31624074; PMCID: PMC6826281. doi:10.1242/bio.044719
Wen, D. T., Zheng, L., Li, J. X., Lu, K., and Hou, W. Q. (2019). The activation of cardiac dSir2-related pathways mediates physical exercise resistance to heart aging in old Drosophila. Aging (Albany NY) 11 (17), 7274–7293. Epub 2019 Sep 10. PMID: 31503544; PMCID: PMC6756900. doi:10.18632/aging.102261
Wen, D. T., Zheng, L., Lu, K., and Hou, W. Q. (2021). Activation of cardiac Nmnat/NAD+/SIR2 pathways mediates endurance exercise resistance to lipotoxic cardiomyopathy in aging Drosophila. J. Exp. Biol. 224 (18), jeb242425. Epub 2021 Sep 15. PMID: 34495320. doi:10.1242/jeb.242425
Wen, D. T., Zheng, L., Lu, K., and Hou, W. Q. (2021). Physical exercise prevents age-related heart dysfunction induced by high-salt intake and heart salt-specific overexpression in Drosophila. Aging (Albany NY) 13 (15), 19542–19560. Epub 2021 Aug 12. PMID: 34383711; PMCID: PMC8386524. doi:10.18632/aging.203364
Wen, D. T., Zheng, L., Ni, L., Wang, H., Feng, Y., and Zhang, M. (2016). The expression of CG9940 affects the adaptation of cardiac function, mobility, and lifespan to exercise in aging Drosophila. Exp. Gerontol. 83, 6–14. Epub 2016 Jul 19. PMID: 27448710. doi:10.1016/j.exger.2016.07.006
White, A. T., and Schenk, S. (2012). NAD(+)/NADH and skeletal muscle mitochondrial adaptations to exercise. Am. J. Physiol. Endocrinol. Metab. 303 (3), E308–E321. Epub 2012 Mar 20. PMID: 22436696; PMCID: PMC3423123. doi:10.1152/ajpendo.00054.2012
Whitham, M., Parker, B. L., Friedrichsen, M., Hingst, J. R., Hjorth, M., Hughes, W. E., et al. (2018). Extracellular vesicles provide a means for tissue crosstalk during exercise. Cell Metab. 27 (1), 237–251. e4. PMID: 29320704. doi:10.1016/j.cmet.2017.12.001
WHO (World Health Organization) (2017). Cardiovascular diseases (CVDs). WHO. Available at: https://www.who.int/news-room/fact-sheets/detail/cardiovascular-diseases-(cvds).
Williams, R. S., and Neufer, P. D. (1996). “Regulation of gene expression in skeletal muscle by contractile activity,” in The handbook of physiology. Exercise: Regulation and integration of multiple systems. Editors L. B. Rowell, and J. T. Shepherd (New York, USA: Oxford University Press), 1124–1150.
Xu, W., Barrientos, T., Mao, L., Rockman, H. A., Sauve, A. A., and Andrews, N. C. (2015). Lethal cardiomyopathy in mice lacking transferrin receptor in the heart. Cell Rep. 13 (3), 533–545. Epub 2015 Oct 8. PMID: 26456827; PMCID: PMC4618069. doi:10.1016/j.celrep.2015.09.023
Zhang, H., Ryu, D., Wu, Y., Gariani, K., Wang, X., Luan, P., et al. (2016). NAD⁺ repletion improves mitochondrial and stem cell function and enhances life span in mice. Science 352 (6292), 1436–1443. Epub 2016 Apr 28. PMID: 27127236. doi:10.1126/science.aaf2693
Zheng, L., Feng, Y., Wen, D. T., Wang, H., and Wu, X. S. (2015). Fatiguing exercise initiated later in life reduces incidence of fibrillation and improves sleep quality in Drosophila. Age (Dordr). 37 (4), 9816. Epub 2015 Jul 24. PMID: 26206392; PMCID: PMC4512962. doi:10.1007/s11357-015-9816-7
Zheng, L., Li, Q. F., Ni, L., Wang, H., Ruan, X. C., and Wu, X. S. (2017). Lifetime regular exercise affects the incident of different arrhythmias and improves organismal health in aging female Drosophila melanogaster. Biogerontology 18 (1), 97–108. Epub 2016 Oct 27. PMID: 27787741. doi:10.1007/s10522-016-9665-5
Keywords: Drosophila, exercise, aging, obesity, cardiac aging, lipid metabolism
Citation: Ding M, Li H and Zheng L (2022) Drosophila exercise, an emerging model bridging the fields of exercise and aging in human. Front. Cell Dev. Biol. 10:966531. doi: 10.3389/fcell.2022.966531
Received: 11 June 2022; Accepted: 22 August 2022;
Published: 09 September 2022.
Edited by:
Adelino Sanchez Ramos da Silva, University of São Paulo, BrazilReviewed by:
Yifan Zhai, Shandong Academy of Agricultural Sciences, ChinaSubhash Rajpurohit, Ahmedabad University, India
Copyright © 2022 Ding, Li and Zheng. This is an open-access article distributed under the terms of the Creative Commons Attribution License (CC BY). The use, distribution or reproduction in other forums is permitted, provided the original author(s) and the copyright owner(s) are credited and that the original publication in this journal is cited, in accordance with accepted academic practice. No use, distribution or reproduction is permitted which does not comply with these terms.
*Correspondence: Lan Zheng, Lanzheng@hunnu.edu.cn