- Institute of Biomedical Informatics, Bioinformatics Center, School of Basic Medical Sciences, Henan University, Kaifeng, China
Diabetic cardiomyopathy is an important complication of diabetes mellitus and the main cause of diabetes death. Diabetic cardiomyopathy is related with many factors, such as hyperglycemia, lipid accumulation, oxidative stress, myocarditis, and apoptosis. Hydrogen sulfide (H2S) is a newly discovered signal molecule, which plays an important role in many physiological and pathological processes. Recent studies have shown that H2S is involved in improving diabetic cardiomyopathy, but its mechanism has not been fully elucidated. This review summarizes the research on the roles and mechanisms of H2S in diabetic cardiomyopathy in recent years to provide the basis for in-depth research in the future.
Introduction
Diabetes has reached epidemic levels, and its prevalence has increased significantly in recent years. In the 1990s, the number of people with diabetes worldwide was ~135 million, and this figure could reach 300 million by 2025. Cardiomyopathy is an important complication of diabetes, and more than 50% of diabetic patients die of cardiomyopathy (Balakumar et al., 2016; Htay et al., 2019). Diabetic cardiomyopathy (DCM) is a disease that leads to myocardial dysfunction without major risk factors (including hypertension or coronary artery disease) (Jia et al., 2018b). DCM is characterized by various forms, including hypertrophy of cardiomyocytes, reduction of myofibrils, interstitial fibrosis, and myocardial microvascular lesions (Russo and Frangogiannis, 2016; Varma et al., 2018). It has been reported that hyperglycemia, lipid accumulation, oxidative stress, inflammation, calcium overload, mitochondrial dysfunction, myocarditis, and apoptosis might be related with the pathogenesis of DCM (Jia et al., 2018a; Dillmann, 2019). However, the mechanism of DCM has not been fully studied. Therefore, it is particularly important to explore the pathogenesis and treatments of diabetic cardiovascular diseases. It has been reported that hydrogen sulfide (H2S) has many biological functions (Sun H. J. et al., 2019) and is involved in many diseases (Wang et al., 2020a), including diabetes-related diseases (Qian et al., 2018). This review summarizes the research about the roles and mechanisms of H2S in DCM in recent years to provide the basis for in-depth research in the future.
Overview of DCM
The term diabetic cardiomyopathy was first used by Rubler in 1972. DCM increased the risk of heart failure in diabetes by four to five times. It is characterized by myocardial cell loss and myocardial fibrosis, ventricular systolic and/or diastolic dysfunction, without significant coronary atherosclerosis and hypertension (Poornima et al., 2006; Karbasforooshan and Karimi, 2017; Yao et al., 2018). In the early stage of DCM, the pathological alterations are mainly in the myocardial interstitium, including the formation of non-enzymatic advanced glycation end products (AGEs), impaired compliance, and ischemic intravascular diseases. Although the morphology of myocardial cells and small coronary arteries has been preserved anatomically, these changes still lead to impaired myocardial contractility. With the development of the disease, left ventricular (LV) hypertrophy is characterized by hypertrophy of myocardial cells, interstitial fibrosis and perivascular fibrosis, thickening of the capillary basement membrane, and formation of intracapillary microaneurysms (Felicio et al., 2016; Jia et al., 2018a). DCM occurs in both type 1 and type 2 diabetes mellitus (Sulaiman et al., 2010), affects nearly two-thirds of diabetic patients, and is the leading cause of diabetes-related morbidity and mortality (Boudina and Abel, 2007; Tillquist and Maddox, 2012). Although the strict control of blood glucose seems to play an important role in the prevention and treatment of DCM, there is an urgent need for new methods and specific therapeutic agents for DCM. Therefore, it is necessary to understand the pathogenesis of DCM (Acar et al., 2011). The molecular mechanism of DCM may be multifactorial, including but not limited to inflammation, cell death, nitroso stress, oxidative stress, impairment in calcium handling, increased AGEs, and mitochondrial dysfunction (Al Hroob et al., 2019). These pathologic alterations in cardiomyocytes are primarily triggered by many metabolic disorders including hyperglycemia, dyslipidemia, increased free fatty acid (FFA) release, and insulin resistance (Mahmoud, 2017). However, the pathogenesis of DCM remains to be elucidated.
Overview of H2S
For many years, H2S has been considered to be a toxic and odorous gas. However, since the 1990s, many studies have shown that H2S, along with NO and CO, belongs to the category of gasotransmitters (Wang et al., 2020b). Three “classic” H2S-producing enzymes have been identified: cystathionine-γ-lyase (CSE), cystathionine-β-synthase (CBS), and 3-mercaptopyruvate thiotransferase (3-MST) (Rose et al., 2017). The expression of H2S-producing enzyme is subcellular and tissue-specific. At the cellular level, CSE occurs strictly in the cytoplasm, while cysteine aminotransferase (CAT) is located in the mitochondria. In terms of tissue specificity, CSE is the most abundant in the cardiovascular system, while CBS is dominant in the nervous system and liver and is expressed in the heart (Kar et al., 2019; Shen et al., 2019). In the process of endogenous H2S production, CBS catalyzes the β-substitution reaction of homocysteine with serine to produce L-cystathionine. L-cystenine is produced by the elimination of α, γ-cysteine of L-cystathionine catalyzed by CSE. L-cystenine then produces H2S via β elimination reaction catalyzed by CSE/CBS. L-cystenine also produces 3-mercaptopyruvate (3-MP) by transferring its amines to α-ketoglutarate catalyzed by CAT. 3-MST catalyzes the sulfur of 3-MP to convert into H2S. In cardiomyocytes that mainly express CSE, H2S is produced with L-cysteine as substrate under the catalysis of CSE (Figure 1) (Behera et al., 2019). In addition, there are some other recognized or assumed sources of H2S, including D-amino acid oxylase (Han et al., 2020) and methionine oxidase (Pol et al., 2018). In biological systems, several non-enzymatic methods can also produce H2S (Yang et al., 2019). In T2DM patients with DCM, endogenous H2S production by CSE is inhibited in cardiomyocytes (Mard et al., 2016). H2S level in plasma is decreased, and the supplement of H2S can reduce the cardiomyopathy dysfunction induced by hyperglycemia (Kar et al., 2019).
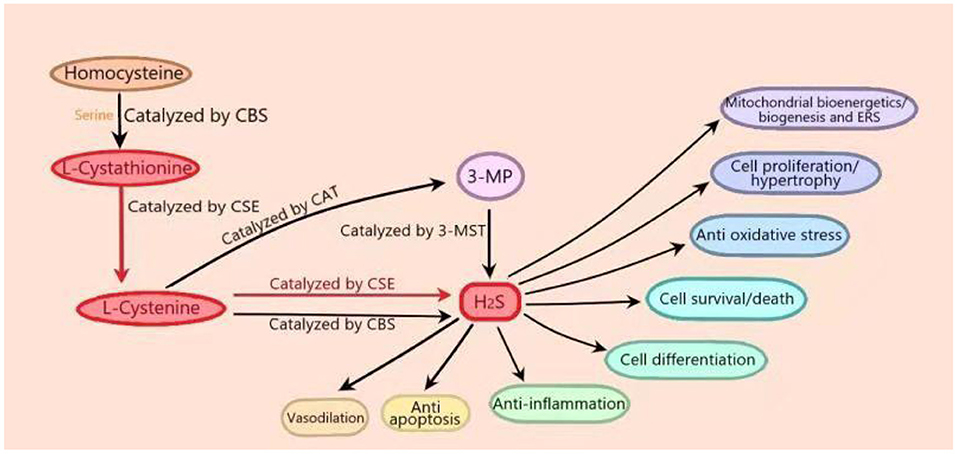
Figure 1. In vivo synthesis process and biological function of hydrogen sulfide (H2S). In the process of endogenous H2S production, firstly, cystathionine-β-synthase (CBS) catalyzes the β-substitution reaction of homocysteine with serine to produce L-cystathionine. L-cystenine is produced by the elimination of α, γ-cysteine of L-cystathionine catalyzed by cystathionine-γ-lyase (CSE). L-cystenine then produces hydrogen sulfide (H2S) via β elimination reaction catalyzed by CSE/CBS. L-cystenine also produces 3-mercaptopyruvate (3-MP) by transferring its amines to α-ketoglutarate catalyzed by cysteine aminotransferase (CAT). 3-Mercaptopyruvate thiotransferase (3-MST) catalyzes the sulfur of 3-MP to convert into H2S. In cardiomyocytes that mainly express CSE, H2S is produced with L-cysteine as substrate under the catalysis of CSE. (The part in the figure is marked in red.) H2S plays important roles in many physiological processes, including vasodilation, blood pressure reduction, anti-apoptosis, anti-inflammation, anti-oxidative stress, cell survival/death, cell differentiation, cell proliferation/hypertrophy, mitochondrial bioenergetics/biogenesis, and endoplasmic reticulum stress.
H2S can act as a signal molecule immediately after it is released, and it can also be stored as bound endosulfan, which can release H2S. At physiological pH, nearly two-thirds of H2S is in the form of hydrogen sulfide anion (HS–) (Guo et al., 2016). H2S plays important roles in many physiological processes, including vasodilation, blood pressure reduction (Greaney et al., 2017; Jin et al., 2017), anti-apoptosis (Li et al., 2019b), anti-inflammation (Zhao et al., 2019), anti-oxidative stress (Tocmo and Parkin, 2019), cell survival/death, cell differentiation, cell proliferation/hypertrophy, mitochondrial bioenergetics/biogenesis, and endoplasmic reticulum stress (ERS) (Figure 1) (Zhang D. et al., 2017). Recent studies have shown that H2S ameliorates diabetic complications including endothelial dysfunction (Li et al., 2019a), nephropathy (Karmin and Siow, 2018), retinopathy (Wang P. et al., 2019), and cardiovascular diseases (Citi et al., 2018). Research has reported that in diabetes, intraislet H2S could promote opening of the ATP dependent potassium channel, increase K+ efflux to lead to cell membrane hyperpolarization, and then close the L-type voltage-dependent calcium channel, thus inhibiting insulin secretion of pancreatic beta cells. On the contrary, much literature has shown that H2S can promote the release of insulin from β-cells. The reason for the above contradiction has not been fully studied (Szabo, 2012; Piragine and Calderone, 2020). In addition, H2S could also regulate the apoptosis of islet beta cells and increase ERS and apoptosis of pancreatic beta cells by inhibiting the extracellular signal regulated kinase (ERK) and activating p38 mitogen activated protein kinase (p38 MAPK) signal pathway (Yang et al., 2007). Other studies have shown that H2S could inhibit high glucose (HG)–induced apoptosis of pancreatic beta cells through the antioxidant, anti-inflammatory, or protein kinase B (Akt) signaling pathways (Taniguchi et al., 2011). However, the mechanism of H2S in diabetes is not fully understood.
The Role of H2S in DCM
Exogenous H2S Improves DCM by Inhibiting Oxidative Stress, Inflammation, and Apoptosis
One of the important pathophysiological factors of DCM is that persistent hyperglycemia induces oxidative stress and apoptosis to lead to cardiac fibrosis (Boudina and Abel, 2007). It has been reported that blocking the formation of reactive oxygen species (ROS) inhibited the expression of the fibrosis-related factor matrix metalloproteinase-2 (MMP-2) induced by HG (Yamagishi et al., 2001). Exogenous H2S can suppress oxidative stress by inhibiting ROS production (Xiao et al., 2018), which may be the mechanism of its anti-diabetic myocardial fibrosis. Nuclear factor kappa-B (NF-κB) is a ubiquitous inducible transcription factor. Activation of NF-κB can upregulate the expression of fibrosis-related factors, including transforming growth factor b1 (TGF-b1) and MMP-2, and increase Fas-ligand (Fas-L) expression to induce Fas-L–mediated apoptosis in the myocardium of diabetic rats, which leads to myocardial fibrosis (Darville and Eizirik, 2001; Chen et al., 2003; Hein et al., 2003). NaHS (a donor of H2S) treatment may play an anti-fibrogenic role by reducing HG-induced expression of NF-κB and the fibrogenic factor such as TGF-b1 and MMP-2 to improve diabetic myocardial fibrosis (El-Seweidy et al., 2011). Therefore, it can be inferred that H2S ameliorates DCM by inhibiting oxidative stress and apoptosis through suppressing the NF-κB pathway and ROS production.
Except for oxidative stress and apoptosis, inflammation is involved in the pathogenesis of DCM (Al-Rasheed et al., 2017; Zhao et al., 2017; Zou et al., 2019). A chronic, low-level state of systemic and sterile inflammation is an important feature of DCM (Sharma et al., 2018). The NLRP3 inflammasome is an important complex protein that mediates inflammation (Wang H. et al., 2019). Studies showed that suppressing NLRP3 notably improved DCM (Ye et al., 2017). H2S decreases HG-induced cell apoptosis, ROS production, and the expression of the NLRP3 inflammasome, Toll-like receptor 4 (TLR4), and NF-κB. NLRP3 gene silencing improves HG-induced cell apoptosis and inflammation, and the TLR4/NF-κB pathway mediates the activation of NLRP3 induced by HG in cardiac cells. These results indicate that H2S improves DCM by inhibiting HG-induced cardiomyocyte inflammation and apoptosis by suppressing the NLRP3 inflammasome through inhibiting the TLR4/NF-κB pathway (Huang et al., 2016). The NLRP3 inflammasome will be an important target for H2S to improve DCM. LV dysfunction, myocardial hypertrophy, and myocardial fibrosis are important pathological changes of DCM. H2S can improve LV function and inhibit myocardial hypertrophy and myocardial fibrosis to ameliorate DCM by suppressing inflammation, oxidative stress, and apoptosis induced by HG. Mechanism studies show that H2S suppresses HG-induced oxidative stress by activating the nuclear factor erythroid 2–related factor 2(Nrf2)/antioxidant response element (ARE) pathway, decreases HG-induced apoptosis through inhibiting the c-Jun N-terminal kinase (JNK)/p38 MAPK pathways, and activating the phosphatidylinositol 3-kinase (PI3K)/Akt pathway (Zhou et al., 2015). Another pathway, the AMPK/mTOR pathway, is also involved with the H2S protection of H9c2 cells against HG-induced apoptosis. GYY4137 (a donor of H2S) treatment improves HG-induced cell viability decrement. Moreover, both GYY4137 and AICAR (an AMPK activator) increase AMPK phosphorylation, decrease mammalian target of rapamycin (mTOR) phosphorylation, and ameliorate HG-induced cell viability decrement. Moreover, AraA (an AMPK inhibitor) attenuates the cardioprotection of GYY4137. Collectively, it can be inferred that exogenous H2S improves DCM through suppressing HG-induced apoptosis by activation of the AMPK/mTOR signal pathway (Wei et al., 2014).
Forkhead box protein O1 (FoxO1), which is a member of the FoxO family, plays a significant role in DCM through affecting oxidative stress, metabolism, inflammation, and apoptosis (Chistiakov et al., 2017). Exogenous H2S can ameliorate cardiac function, myocardial fibrosis, and hypertrophy in diabetic mice by inhibiting oxidative stress and apoptosis induced by HG. Mechanism studies show that H2S promotes the phosphorylation level of FoxO1 and suppresses FoxO1 nuclear translocation in cardiomyocytes via S-sulfhydration, which inhibits apoptosis and suppresses oxidative stress by increasing the expression of antioxidant enzymes. Therefore, it can be inferred that exogenous H2S ameliorates DCM via the FoxO1 pathway (Ye et al., 2018). FoxO1 may be an important target for H2S to improve DCM. The Wnt/β-catenin pathway also plays an important role in H2S protection of DCM. Zhang and Ye found that H2S could reduce the levels of ROS and malondialdehyde and increase the activities of superoxide dismutase, catalase, and glutathione peroxidase to attenuate HG-induced cardiomyocyte apoptosis and oxidative stress by suppressing the Wnt/β-catenin pathway, which need to be further studied, especially the relationship between the Wnt/β-catenin pathway and apoptosis (Zhang and Ye, 2019).
AGEs are proteins or lipids that become glycosylated after exposure to sugars. The receptor of advanced glycated product (RAGE) is a transmembrane receptor of the immunoglobulin superfamily, which can lead to oxidative stress, inflammation, and apoptosis. It has been found that H2S can inhibit oxidative stress, inflammation, and apoptosis induced by RAGE over-activation through inhibiting RAGE dimer formation and impairing its protein stability (Zhou et al., 2017). Whether RAGE mediates the protective effect of H2S on the diabetic myocardium is worth discussing. The signal transduction pathways involved in the above processes are summarized in Table 1.
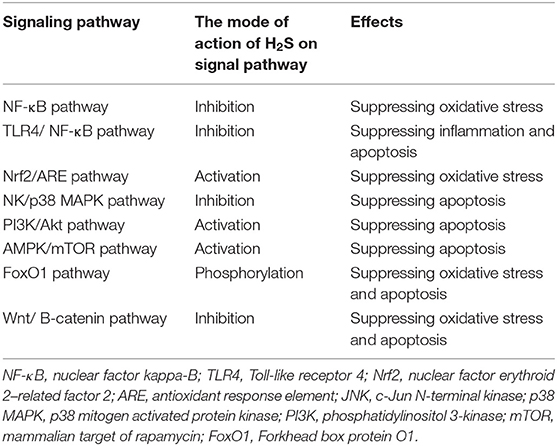
Table 1. The signaling pathways involved in exogenous hydrogen sulfide (H2S) improvements of diabetic cardiomyopathy by inhibiting oxidative stress, inflammation, and apoptosis.
Exogenous H2S Improves DCM by Regulating ERS
It has been reported that ERS can be induced in the diabetic heart and participate in the pathogenesis of DCM (Lian et al., 2017). Cardiac lipid toxicity refers to the direct toxic effect of excessive lipid deposition on myocardial cell function, which may be an important part of the phenotype of DCM. Both exogenous H2S and ERS inhibitors (4-PBA) can improve DCM by decreasing palmitic acid (PA)–induced myocardial injury. Similar results can be obtained in diabetic rats by using NaHS or 4-PBA. In addition, exogenous H2S inhibits ERS in diabetic rats or PA-induced AC 16 cardiac cells by decreasing the expressions of CHOP, GRP78, and caspase-12; therefore, it can be inferred that exogenous H2S can ameliorate DCM by inhibiting ERS (Guo et al., 2017). The relationship between ERS and apoptosis needs to be clarified, and how H2S regulates lipid metabolism through ERS deserves further study. It has been reported that ROS overproduction in DCM is often accompanied by ERS and that ROS/ERS-mediated apoptosis is involved in the pathogenesis and development of DCM. Exogenous H2S can improve the myocardial injury of diabetic rats, reduce the expression of ERS-related proteins, and improve the injury of H9c2 cells induced by HG. In HG-induced H9C2 cells, exogenous H2S notably reduced intracellular ROS levels, while an ROS scavenger decreases HG-induced apoptosis, indicating that ROS-induced cardiomyocyte apoptosis mediates the protective effect of exogenous H2S on the diabetic myocardium. HG can induce ROS and ERS, while an ROS scavenger can inhibit the expression of ERS-related proteins induced by HG, suggesting that HG increases ROS level to promote ERS. In addition, in an HG-induced H9C2 cell, HG induces Mfn-2 expression, and siRNA targeting Mfn-2 reduces ROS-induced apoptosis and HG-induced ERS, indicating that HG increases ROS/ERS-mediated apoptosis via Mfn-2. Based on the above, it can be inferred that exogenous H2S ameliorates DCM by decreasing ROS/ERS-mediated apoptosis through suppression of Mfn-2 expression (Yang et al., 2017). The relationship of ERS and Mfn-2 requires further study.
Exogenous H2S Improves DCM by Regulating Autophagy
Type 2 diabetes is characterized by protein misfolding and aggregation, which results in mitochondrial damage, ROS production, and apoptosis and leads to ubiquitin aggregates (Chiti and Dobson, 2006; Shang and Taylor, 2011; Sato et al., 2014). Ubiquitin aggregation, which also leads to apoptosis and ROS production, is mainly eliminated by autophagy (Zhang Y. et al., 2017; Grumati and Dikic, 2018). Autophagy plays a protective role in DCM by clearing misfolded protein and ubiquitin (Pei et al., 2018). In diabetic mice, exogenous H2S can ameliorate DCM by decreasing ROS production. Exogenous H2S also can promote the degradation of autophagosome content, decrease the expression of p62, and increase the expression of microtubule associated protein 1 light chain 3 II (LC3II), autophagy associated protein 7 (Atg7), and Beclin1, indicating that exogenous H2S promotes autophagy. Moreover, exogenous H2S increases the expression of kelch-like ECH related protein 1 (keap-1) by decreasing its ubiquitylation, and Keap-1 siRNA inhibits the effect of exogenous H2S on autophagy in the cardiomyocyte of diabetic rats, indicating that exogenous H2S promotes autophagy through keap-1. Further research shows that 1,4-dithiothreitol, an inhibitor of disulfide bonds, counteracts the effects of H2S on keap-1, ubiquitin aggregates clearance and ROS production in HG-induced H9C2 cells, and H2S can promote the formation of disulfide between two keap-1 molecules, which indicates that exogenous H2S suppresses Keap-1 ubiquitylation through promoting its disulfide formation. From the above results, it can be inferred that exogenous H2S improves DCM by promoting ubiquitin aggregation clearance through promoting autophagy via ubiquitylation of Keap-1, which contributes to ROS scavenging and provides a new mechanism for the antioxidation of H2S. In addition, exogenous H2S has no notable effect on Nrf2 nuclear translocation, indicating that its antioxidant effect is not related with the keap-1/Nrf2 signaling pathway (Wu et al., 2017). The mechanism of exogenous H2S acting as an antioxidant through autophagy needs further study.
Exogenous H2S Improves DCM by Improving Cardiac Mitochondrial Function
Recent evidence suggests that DCM is associated with metabolic abnormalities, more often with mitochondrial dysfunction. Sirtuin 3 (SIRT3) belongs to the nicotinamide adenine dinucleotide (NAD)–dependent deacetylase family and is the major mitochondrial deacetylase of lysine residues. In cardiac mitochondria of diabetic mice, cardiac mitochondrial respiratory capacities, ATP synthesis, and the expression and activity of SIRT3 were decreased, and exogenous H2S increased the expression and activity of SIRT3 by restoring the ratio of NAD+/NADH, and decreased the acetylation levels of the mitochondrial respiratory complex enzymes to improve cardiac mitochondrial dysfunction. SiRNA-mediated SIRT3 silencing increases the acetylation level of mitochondrial respiratory complexes, while exogenous H2S partially restores the acetylation level of these enzymes. Collectively, it can be inferred that exogenous H2S improves DCM by improving cardiac mitochondrial function through increasing the expression of SIRT3 to regulate the lysine acetylation of mitochondrial respiratory complexes (Sun Y. et al., 2019). The role of SIRT3 in H2S improving DCM remains to be further studied.
Exogenous H2S Improves DCM by Activating KATP Channels
The KATP channels are abundant in the myocardium (Nichols and Lederer, 1991). The opening of KATP channels can reduce the apoptosis induced by oxidative stress in cardiac cells to improve DCM (Akao et al., 2001). Liang et al. found that the expression levels of KATP channels were decreased by HG, which was abolished by exogenous H2S. HG or KATP channel blocker could induce in H9c2 cardiac cells considerable injuries, including reducing cell viability, increasing apoptosis, ROS generation, and cleaved caspase-3 expression, as well as the loss of MMP. However, exogenous H2S or KATP channel openers could reverse the changes. Collectively, it could be inferred that exogenous H2S could improve DCM by activating KATP channels. Moreover, an ROS scavenger could ameliorate the reduction in the expression levels of the KATP channel induced by HG, and KATP channel openers could decrease HG-induced ROS production, indicating that there is an interaction between KATP channels and ROS and that the interaction is involved in the above H2S improvement of DCM (Liang et al., 2016). The role of KATP channels in the improvement of DCM by H2S, especially the interaction between KATP channels and ROS, needs to be further studied. KATP channels will become an important target for H2S to improve DCM.
The Roles of Endogenous H2S in DCM
In addition to exogenous H2S, endogenous H2S can also improve DCM. El-Sayed et al. found that HG decreased CSE expression/activity, H2S, and serum adiponectin level and increased myocardial imidazoline I1 receptor expression, while moxonidine (imidazoline I1 receptor agonist) abolished the above effects of HG and improved the glycemic state; reversed myocardial hypertrophy, hypertension, and baroreflex dysfunction in streptozocin (STZ)-treated rats; and inhibited the expression of death associated protein kinase-3 (DAPK-3) to play cardiovascular protective effects in diabetic mice. Moreover, inhibition of CSE decreased endogenous H2S production and counteracted moxonidine protective effects, indicating that CSE-derived H2S might mediate the cardiovascular protective effects of moxonidine in diabetes (El-Sayed et al., 2016). So far, there are few studies on moxonidine and endogenous H2S, so how moxonidine promotes the production of CSE-derived H2S remains to be further explored.
Conclusion
Exogenous H2S can improve DCM by suppressing oxidative stress, inflammation, and apoptosis. Exogenous H2S improves DCM by inhibiting apoptosis through suppressing ERS or inhibiting ROS/ERS-mediated apoptosis through suppressing Mfn-2 expression. Exogenous H2S improves DCM by suppressing ubiquitylation of Keap-1 to promote autophagy for ubiquitin clearance. Exogenous H2S improves DCM by improving cardiac mitochondrial function through activating SIRT3. Exogenous H2S could improve DCM by activating KATP channels (Figure 2). It can be seen from the above that the anti-inflammatory, anti-apoptotic, and antioxidant effects of H2S have potential therapeutic value in DCM, but its mechanism has not been fully studied, especially the signal transduction pathways involved. Autophagy, the NLRP3 inflammasome, and ERS are the regulated targets of H2S and are involved in the process of improving DCM by H2S, so the interaction among the three in H2S improvement of DCM should be studied in the future. ERS and NLRP3 inflammasome crosstalk has been reported to play an important role in metabolic disorders (Ji et al., 2019), and H2S regulates the ERS/NLRP3 inflammasome in many diseases (Wang et al., 2020a,b), so whether H2S can regulate the ERS/NLRP3 inflammasome to improve diabetes deserves to be studied. In addition, the existing H2S releasers cannot fully meet the requirements of research and development of H2S related drugs, so the development of new H2S releasers is very important for the application of H2S related drugs in the treatment of clinical diseases.
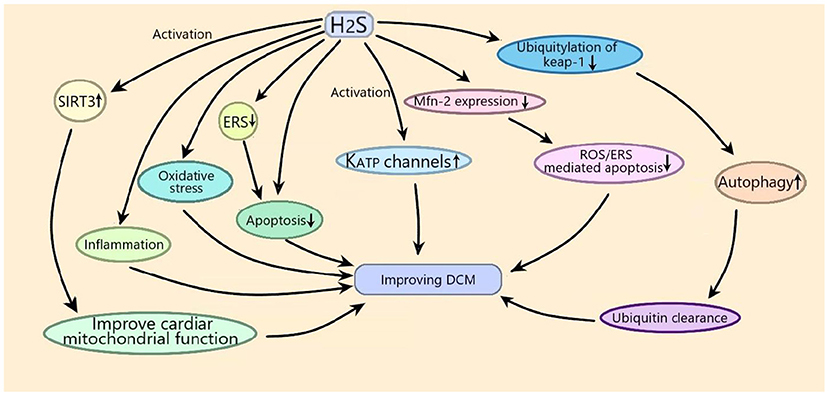
Figure 2. Schematic diagram of the mechanism of exogenous H2S improving diabetic cardiomyopathy (DCM). Exogenous H2S improves DCM by suppressing oxidative stress, inflammation, and apoptosis. Exogenous H2S improves DCM by inhibiting apoptosis through suppressing endoplasmic reticulum stress (ERS) or inhibiting reactive oxygen species (ROS)/ERS–mediated apoptosis through suppressing Mfn-2 expression. Exogenous H2S improves DCM by suppressing ubiquitylation of kelch-like ECH related protein 1 (Keap-1) to promote autophagy for ubiquitin clearance. Exogenous H2S improves DCM by improving cardiac mitochondrial function through activating sirtuin 3 (SIRT3). Exogenous H2S could improve DCM by activating KATP channels.
Diabetic states can inhibit endogenous H2S-producing enzyme CSE, while the activation of myocardial imidazoline I1 receptor with moxonidine can improve DCM by increasing endogenous H2S via CSE or increase CSE expression to inhibit DAPK-3 to ameliorate DCM (Figure 3). Myocardial imidazoline I1 receptor is an important target for the development of therapeutic drugs for DCM.
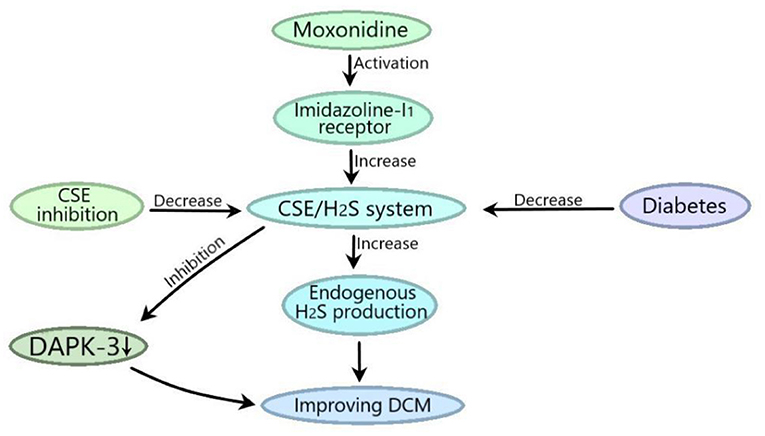
Figure 3. CSE-derived H2S mediates the moxonidine improvements of DCM. Diabetic state can inhibit the CSE/H2S system, and moxonidine can activate myocardial imidazoline I1 receptor to improve DCM by promoting the CSE/H2S system to increase endogenous H2S production or by promoting the CSE/H2S system to inhibit death related protein kinase 3 (DAPK-3).
In conclusion, with further research, H2S could provide new ways of treating DCM.
Author Contributions
HW: devised, writing, and funded with this review. XiaL: drawing. SZ: writing and funded with this review. XW: writing. All authors contributed to the article and approved the submitted version.
Funding
This work was supported by grants from key scientific and technological projects in Henan Province, China (Grant No. 202102310153), the National Natural Science Foundation of China (Grant No. 81903444), the China Postdoctoral Science Foundation (Grant No. 2018M640674), and the Key Science and Technology Program of Henan Province in China (Grant No. 192102310145).
Conflict of Interest
The authors declare that the research was conducted in the absence of any commercial or financial relationships that could be construed as a potential conflict of interest.
References
Acar, E., Ural, D., Bildirici, U., Sahin, T., and Yilmaz, I. (2011). Diabetic cardiomyopathy. Anatolian J. Cardiol. 11, 732–737. doi: 10.5152/akd.2011.196
Akao, M., Ohler, A., O'rourke, B., and Marban, E. (2001). Mitochondrial ATP-sensitive potassium channels inhibit apoptosis induced by oxidative stress in cardiac cells. Circ. Res. 88, 1267–1275. doi: 10.1161/hh1201.092094
Al Hroob, A. M., Abukhalil, M. H., Hussein, O. E., and Mahmoud, A. M. (2019). Pathophysiological mechanisms of diabetic cardiomyopathy and the therapeutic potential of epigallocatechin-3-gallate. Biomed. Pharmacotherapy 109, 2155–2172. doi: 10.1016/j.biopha.2018.11.086
Al-Rasheed, N. M., Al-Rasheed, N. M., Hasan, I. H., Al-Amin, M. A., Al-Ajmi, H. N., Mohamad, R. A., et al. (2017). Simvastatin ameliorates diabetic cardiomyopathy by attenuating oxidative stress and inflammation in rats. Oxid. Med. Cell. Longev. 2017, 1092015. doi: 10.1155/2017/1092015
Balakumar, P., Maung-U., K., and Jagadeesh, G. (2016). Prevalence and prevention of cardiovascular disease and diabetes mellitus. Pharmacol. Res. 113, 600–609. doi: 10.1016/j.phrs.2016.09.040
Behera, J., Tyagi, S. C., and Tyagi, N. (2019). Role of hydrogen sulfide in the musculoskeletal system. Bone 124, 33–39. doi: 10.1016/j.bone.2019.03.034
Boudina, S., and Abel, E. D. (2007). Diabetic cardiomyopathy revisited. Circulation 115, 3213–3223. doi: 10.1161/CIRCULATIONAHA.106.679597
Chen, S. L., Khan, Z. A., Cukiernik, M., and Chakrabarti, S. (2003). Differential activation of NF-κB and AP-1 in increased fibronectin synthesis in target organs of diabetic complications. Am. J. Physiol. Endocrinol. Metab. 284, E1089–E1097. doi: 10.1152/ajpendo.00540.2002
Chistiakov, D. A., Orekhov, A. N., and Bobryshev, Y. V. (2017). The impact of FOXO-1 to cardiac pathology in diabetes mellitus and diabetes-related metabolic abnormalities. Int. J. Cardiol. 245, 236–244. doi: 10.1016/j.ijcard.2017.07.096
Chiti, F., and Dobson, C. M. (2006). Protein misfolding, functional amyloid, and human disease. Annu. Rev. Biochem. 75, 333–366. doi: 10.1146/annurev.biochem.75.101304.123901
Citi, V., Piragine, E., Testai, L., Breschi, M. C., Calderone, V., and Martelli, A. (2018). The role of hydrogen sulfide and H2S-donors in myocardial protection against ischemia/reperfusion injury. Curr. Med. Chem. 25, 4380–4401. doi: 10.2174/0929867325666180212120504
Darville, M. I., and Eizirik, D. L. (2001). Cytokine induction of Fas gene expression in insulin-producing cells requires the transcription factors NF-κB and C/EBP. Diabetes 50, 1741–1748. doi: 10.2337/diabetes.50.8.1741
Dillmann, W. H. (2019). Diabetic cardiomyopathy what is it and can it be fixed? Circ. Res. 124, 1160–1162. doi: 10.1161/CIRCRESAHA.118.314665
El-Sayed, S. S., Zakaria, M. N. M., Abdel-Ghany, R. H., and Abdel-Rahman, A. A. (2016). Cystathionine-gamma lyase-derived hydrogen sulfide mediates the cardiovascular protective effects of moxonidine in diabetic rats. Eur. J. Pharmacol. 783, 73–84. doi: 10.1016/j.ejphar.2016.04.054
El-Seweidy, M. M., Sadik, N. A. H., and Shaker, O. G. (2011). Role of sulfurous mineral water and sodium hydrosulfide as potent inhibitors of fibrosis in the heart of diabetic rats. Arch. Biochem. Biophys. 506, 48–57. doi: 10.1016/j.abb.2010.10.014
Felicio, J. S., Koury, C. C., Carvalho, C. T., Abrahao Neto, J. F., Mileo, K. B., Arbage, T. P., et al. (2016). Present insights on cardiomyopathy in diabetic patients. Curr. Diabetes Rev. 12, 384–395. doi: 10.2174/1573399812666150914120529
Greaney, J. L., Kutz, J. L., Shank, S. W., Jandu, S., Santhanam, L., and Alexander, L. M. (2017). Impaired hydrogen sulfide-mediated vasodilation contributes to microvascular endothelial dysfunction in hypertensive adults. Hypertension 69, 902. doi: 10.1161/HYPERTENSIONAHA.116.08964
Grumati, P., and Dikic, I. (2018). Ubiquitin signaling and autophagy. J. Biol. Chem. 293, 5404–5413. doi: 10.1074/jbc.TM117.000117
Guo, F.-F., Yu, T.-C., Hong, J., and Fang, J.-Y. (2016). Emerging roles of hydrogen sulfide in inflammatory and neoplastic colonic diseases. Front. Physiol. 7:156. doi: 10.3389/fphys.2016.00156
Guo, R., Wu, Z., Jiang, J., Liu, C., Wu, B., Li, X., et al. (2017). New mechanism of lipotoxicity in diabetic cardiomyopathy: deficiency of endogenous H2S production and ER stress. Mech. Ageing Dev. 162, 46–52. doi: 10.1016/j.mad.2016.11.005
Han, M., Liu, D., Qiu, J., Yuan, H., Hu, Q., Xue, H., et al. (2020). Evaluation of H2S-producing enzymes in cerebrospinal fluid and its relationship with interleukin-6 and neurologic deficits in subarachnoid hemorrhage. Biomed. Pharmacotherapy 123, 109722. doi: 10.1016/j.biopha.2019.109722
Hein, S., Arnon, E., Kostin, S., Schonburg, M., Elsasser, A., Polyakova, V., et al. (2003). Progression from compensated hypertrophy to failure in the pressure-overloaded human heart - Structural deterioration and compensatory mechanisms. Circulation 107, 984–991. doi: 10.1161/01.CIR.0000051865.66123.B7
Htay, T., Soe, K., Lopez-Perez, A., Amy Hoanganh, D., Romagosa, M. A., and Aung, K. (2019). Mortality and cardiovascular disease in type 1 and type 2 diabetes. Curr. Cardiol. Rep. 21. doi: 10.1007/s11886-019-1133-9
Huang, Z., Zhuang, X., Xie, C., Hu, X., Dong, X., Guo, Y., et al. (2016). Exogenous hydrogen sulfide attenuates high glucose-induced cardiotoxicity by inhibiting NLRP3 inflammasome activation by suppressing TLR4/NF-κB pathway in H9c2 cells. Cell. Physiol. Biochem. 40, 1578–1590. doi: 10.1159/000453208
Ji, T., Han, Y., Yang, W., Xu, B., Sun, M., Jiang, S., et al. (2019). Endoplasmic reticulum stress and NLRP3 inflammasome: crosstalk in cardiovascular and metabolic disorders. J. Cell. Physiol. 234, 14773–14782. doi: 10.1002/jcp.28275
Jia, G., Hill, M. A., and Sowers, J. R. (2018a). Diabetic cardiomyopathy: an update of mechanisms contributing to this clinical entity. Circ. Res. 122, 624–638. doi: 10.1161/CIRCRESAHA.117.311586
Jia, G., Whaley-Connell, A., and Sowers, J. R. (2018b). Diabetic cardiomyopathy: a hyperglycaemia- and insulin-resistance-induced heart disease. Diabetologia 61, 21–28. doi: 10.1007/s00125-017-4390-4
Jin, S., Teng, X., Xiao, L., Xue, H., Guo, Q., Duan, X., et al. (2017). Hydrogen sulfide ameliorated L-NAME-induced hypertensive heart disease by the Akt/eNOS/NO pathway. Exp. Biol. Med. 242, 1831–1841. doi: 10.1177/1535370217732325
Kar, S., Kambis, T. N., and Mishra, P. K. (2019). Hydrogen sulfide-mediated regulation of cell death signaling ameliorates adverse cardiac remodeling and diabetic cardiomyopathy. Am. J. Physiol. Heart Circ. Physiol. 316, H1237–H1252. doi: 10.1152/ajpheart.00004.2019
Karbasforooshan, H., and Karimi, G. (2017). The role of SIRT1 in diabetic cardiomyopathy. Biomed. Pharmacotherapy 90, 386–392. doi: 10.1016/j.biopha.2017.03.056
Karmin, O., and Siow, Y. L. (2018). Metabolic imbalance of homocysteine and hydrogen sulfide in kidney disease. Curr. Med. Chem. 25, 367–377. doi: 10.2174/0929867324666170509145240
Li, J., Teng, X., Jin, S., Dong, J., Guo, Q., Tian, D., et al. (2019a). Hydrogen sulfide improves endothelial dysfunction by inhibiting the vicious cycle of NLRP3 inflammasome and oxidative stress in spontaneously hypertensive rats. J. Hypertens. 37, 1633–1643. doi: 10.1097/HJH.0000000000002101
Li, J., Yuan, Y.-Q., Zhang, L., Zhang, H., Zhang, S.-W., Zhang, Y., et al. (2019b). Exogenous hydrogen sulfide protects against high glucose-induced apoptosis and oxidative stress by inhibiting the STAT3/HIF-1 alpha pathway in H9c2 cardiomyocytes. Exp. Therap. Med. 18, 3948–3958. doi: 10.3892/etm.2019.8036
Lian, J., Chen, J., Yuan, Y., Chen, J., Sayed, M. D. M., Luo, L., et al. (2017). Cortex Mori Radicis extract attenuates myocardial damages in diabetic rats by regulating ERS. Biomed. Pharmacotherapy 90, 777–785. doi: 10.1016/j.biopha.2017.03.097
Liang, W., Chen, J., Mo, L., Ke, X., Zhang, W., Zheng, D., et al. (2016). ATP-sensitive K+ channels contribute to the protective effects of exogenous hydrogen sulfide against high glucose-induced injury in H9c2 cardiac cells. Int. J. Mol. Med. 37, 763–772. doi: 10.3892/ijmm.2016.2467
Mahmoud, A. M. (2017). Exercise amaliorates metabolic disturbances and oxidative stress in diabetic cardiomyopathy: possible underlying mecchanisms. Adv. Exp. Med. Biol. 999, 207–230. doi: 10.1007/978-981-10-4307-9_12
Mard, S. A., Ahmadi, I., Ahangarpour, A., Gharib-Naseri, M. K., and Badavi, M. (2016). Delayed gastric emptying in diabetic rats caused by decreased expression of cystathionine gamma lyase and H2S synthesis: in vitro and in vivo studies. Neurogastroenterol. Motil. 28, 1677–1689. doi: 10.1111/nmo.12867
Nichols, C. G., and Lederer, W. J. (1991). Adenosine triphosphate-sensitive potassium channels in the cardiovascular system. Am. J. Physiol. 261, H1675–1686. doi: 10.1152/ajpheart.1991.261.6.H1675
Pei, Z., Deng, Q., Babcock, S. A., He, E. Y., Ren, J., and Zhang, Y. (2018). Inhibition of advanced glycation endproduct (AGE) rescues against streptozotocin-induced diabetic cardiomyopathy: role of autophagy and ER stress. Toxicol. Lett. 284, 10–20. doi: 10.1016/j.toxlet.2017.11.018
Piragine, E., and Calderone, V. (2020). Pharmacological modulation of the hydrogen sulfide (H2S) system by dietary H2S-donors: a novel promising strategy in the prevention and treatment of type 2 diabetes mellitus. Phytotherapy Res. doi: 10.1002/ptr.6923
Pol, A., Renkema, G. H., Tangerman, A., Winkel, E. G., Engelke, U. F., De Brouwer, A. P. M., et al. (2018). Mutations in SELENBP1, encoding a novel human methanethiol oxidase, cause extraoral halitosis. Nat. Genet. 50, 120–129. doi: 10.1038/s41588-017-0006-7
Poornima, I. G., Parikh, P., and Shannon, R. P. (2006). Diabetic cardiomyopathy - the search for a unifying hypothesis. Circ. Res. 98, 596–605. doi: 10.1161/01.RES.0000207406.94146.c2
Qian, L.-L., Liu, X.-Y., Chai, Q., and Wang, R.-X. (2018). Hydrogen sulfide in diabetic complications: focus on molecular mechanisms. Endocr. Metab. Immune Disord. Drug Targets 18, 470–476. doi: 10.2174/1871530318666180426100532
Rose, P., Moore, P. K., and Zhu, Y. Z. (2017). H2S biosynthesis and catabolism: new insights from molecular studies. Cell. Mol. Life Sci. 74, 1391–1412. doi: 10.1007/s00018-016-2406-8
Russo, I., and Frangogiannis, N. G. (2016). Diabetes-associated cardiac fibrosis: cellular effectors, molecular mechanisms and therapeutic opportunities. J. Mol. Cell. Cardiol. 90, 84–93. doi: 10.1016/j.yjmcc.2015.12.011
Sato, A., Asano, T., Isono, M., Ito, K., and Asano, T. (2014). Panobinostat synergizes with bortezomib to induce endoplasmic reticulum stress and ubiquitinated protein accumulation in renal cancer cells. BMC Urol. 14:71. doi: 10.1186/1471-2490-14-71
Shang, F., and Taylor, A. (2011). Ubiquitin-proteasome pathway and cellular responses to oxidative stress. Free Radic. Biol. Med. 51, 5–16. doi: 10.1016/j.freeradbiomed.2011.03.031
Sharma, A., Tate, M., Mathew, G., Vince, J. E., Ritchie, R. H., and De Haan, J. B. (2018). Oxidative stress and NLRP3-inflammasome activity as significant drivers of diabetic cardiovascular complications: therapeutic implications. Front. Physiol. 9:114. doi: 10.3389/fphys.2018.00114
Shen, F., Zhao, C.-S., Shen, M.-F., Wang, Z., and Chen, G. (2019). The role of hydrogen sulfide in gastric mucosal damage. Med. Gas Res. 9, 88–92. doi: 10.4103/2045-9912.260650
Sulaiman, M., Matta, M. J., Sunderesan, N. R., Gupta, M. P., Periasamy, M., and Gupta, M. (2010). Resveratrol, an activator of SIRT1, upregulates sarcoplasmic calcium ATPase and improves cardiac function in diabetic cardiomyopathy. Am. J. Physiol. Heart Circ. Physiol. 298, H833–H843. doi: 10.1152/ajpheart.00418.2009
Sun, H.-J., Wu, Z.-Y., Cao, L., Zhu, M.-Y., Liu, T.-T., Guo, L., et al. (2019). Hydrogen sulfide: recent progression and perspectives for the treatment of diabetic nephropathy. Molecules 24, 2857. doi: 10.3390/molecules24152857
Sun, Y., Teng, Z., Sun, X., Zhang, L., Chen, J., Wang, B., et al. (2019). Exogenous H2S reduces the acetylation levels of mitochondrial respiratory enzymes via regulating the NAD(+)-SIRT3 pathway in cardiac tissues of db/db mice. Am. J. Physiol. Endocrinol. Metab. 317, E284–E297. doi: 10.1152/ajpendo.00326.2018
Szabo, C. (2012). Roles of hydrogen sulfide in the pathogenesis of diabetes mellitus and its complications. Antioxid. Redox Signal. 17, 68–80. doi: 10.1089/ars.2011.4451
Taniguchi, S., Kang, L., Kimura, T., and Niki, I. (2011). Hydrogen sulphide protects mouse pancreatic beta-cells from cell death induced by oxidative stress, but not by endoplasmic reticulum stress. Br. J. Pharmacol. 162, 1171–1178. doi: 10.1111/j.1476-5381.2010.01119.x
Tillquist, M. N., and Maddox, T. M. (2012). Update on diabetic cardiomyopathy: inches forward, miles to go. Curr. Diabetes Rep. 12, 305–313. doi: 10.1007/s11892-012-0274-7
Tocmo, R., and Parkin, K. (2019). S-1-propenylmercaptocysteine protects murine hepatocytes against oxidative stress via persulfidation of Keap1 and activation of Nrf2. Free Radic. Biol. Med. 143, 164–175. doi: 10.1016/j.freeradbiomed.2019.07.022
Varma, U., Koutsifeli, P., Benson, V. L., Mellor, K. M., and Delbridge, L. M. D. (2018). Molecular mechanisms of cardiac pathology in diabetes - Experimental insights. Biochim. Biophys. Acta Mol. Basis Dis. 1864, 1949–1959. doi: 10.1016/j.bbadis.2017.10.035
Wang, H., Shi, X., Qiu, M., Lv, S., and Liu, H. (2020a). Hydrogen sulfide plays an important protective role through influencing endoplasmic reticulum stress in diseases. Int. J. Biol. Sci. 16, 264–271. doi: 10.7150/ijbs.38143
Wang, H., Shi, X., Qiu, M., Lv, S., Zheng, H., Niu, B., et al. (2020b). Hydrogen sulfide plays an important role by influencing NLRP3 inflammasome. Int. J. Biol. Sci. 16, 2752–2760. doi: 10.7150/ijbs.47595
Wang, H., Zhong, P., and Sun, L. (2019). Exogenous hydrogen sulfide mitigates NLRP3 inflammasome-mediated inflammation through promoting autophagy via the AMPK-mTOR pathway. Biol. Open 8, bio043653. doi: 10.1242/bio.043653
Wang, P., Chen, F., Wang, W., and Zhang, X.-D. (2019). Hydrogen sulfide attenuates high glucose-induced human retinal pigment epithelial cell inflammation by inhibiting ROS formation and NLRP3 inflammasome activation. Mediat. Inflamm. 2019, 8908960. doi: 10.1155/2019/8908960
Wei, W.-B., Hu, X., Zhuang, X.-D., Liao, L.-Z., and Li, W.-D. (2014). GYY4137, a novel hydrogen sulfide-releasing molecule, likely protects against high glucose-induced cytotoxicity by activation of the AMPK/mTOR signal pathway in H9c2 cells. Mol. Cell. Biochem. 389, 249–256. doi: 10.1007/s11010-013-1946-6
Wu, J., Tian, Z., Sun, Y., Lu, C., Liu, N., Gao, Z., et al. (2017). Exogenous H2S facilitating ubiquitin aggregates clearance via autophagy attenuates type 2 diabetes-induced cardiomyopathy. Cell Death Dis. 8, e2992. doi: 10.1038/cddis.2017.380
Xiao, Q., Ying, J., Xiang, L., and Zhang, C. (2018). The biologic effect of hydrogen sulfide and its function in various diseases. Medicine 97, e13065. doi: 10.1097/MD.0000000000013065
Yamagishi, S., Edelstein, D., Du, X. L., and Brownlee, M. (2001). Hyperglycemia potentiates collagen-induced platelet activation through mitochondrial superoxide overproduction. Diabetes 50, 1491–1494. doi: 10.2337/diabetes.50.6.1491
Yang, F., Yu, X., Li, T., Wu, J., Zhao, Y., Liu, J., et al. (2017). Exogenous H2S regulates endoplasmic reticulum-mitochondria cross-talk to inhibit apoptotic pathways in STZ-induced type I diabetes. Am. J. Physiol. Endocrinol. Metab. 312, E190–E203. doi: 10.1152/ajpendo.00196.2016
Yang, G., Yang, W., Wu, L., and Wang, R. (2007). H2S, endoplasmic reticulum stress, and apoptosis of insulin-secreting beta cells. J. Biol. Chem. 282, 16567–16576. doi: 10.1074/jbc.M700605200
Yang, J., Minkler, P., Grove, D., Wang, R., Willard, B., Dweik, R., et al. (2019). Non-enzymatic hydrogen sulfide production from cysteine in blood is catalyzed by iron and vitamin B-6. Commun. Biol. 2, 19. doi: 10.1038/s42003-019-0431-5
Yao, Q., Ke, Z.-Q., Guo, S., Yang, X.-S., Zhang, F.-X., Liu, X.-F., et al. (2018). Curcumin protects against diabetic cardiomyopathy by promoting autophagy and alleviating apoptosis. J. Mol. Cell. Cardiol. 124, 26–34. doi: 10.1016/j.yjmcc.2018.10.004
Ye, P., Gu, Y., Zhu, Y.-R., Chao, Y.-L., Kong, X.-Q., Luo, J., et al. (2018). Exogenous hydrogen sulfide attenuates the development of diabetic cardiomyopathy via the FoxO1 pathway. J. Cell. Physiol. 233, 9786–9798. doi: 10.1002/jcp.26946
Ye, Y., Bajaj, M., Yang, H.-C., Perez-Polo, J. R., and Birnbaum, Y. (2017). SGLT-2 inhibition with dapagliflozin reduces the activation of the Nlrp3/ASC inflammasome and attenuates the development of diabetic cardiomyopathy in mice with type 2 diabetes. Further augmentation of the effects with saxagliptin, a DPP4 inhibitor. Cardiovasc. Drugs Therapy 31, 119–132. doi: 10.1007/s10557-017-6725-2
Zhang, D., Du, J., Tang, C., Huang, Y., and Jin, H. (2017). H2S-induced sulfhydration: biological function and detection methodology. Front. Pharmacol. 8:608. doi: 10.3389/fphar.2017.00608
Zhang, M., and Ye, M. (2019). Hydrogen sulfide attenuates high glucose-induced myocardial injury in rat cardiomyocytes by suppressing Wnt/beta-catenin pathway. Curr. Med. Sci. 39, 938–946. doi: 10.1007/s11596-019-2120-5
Zhang, Y., Chen, X., Zhao, Y., Ponnusamy, M., and Liu, Y. (2017). The role of ubiquitin proteasomal system and autophagy-lysosome pathway in Alzheimer's disease. Rev. Neurosci. 28, 861–868. doi: 10.1515/revneuro-2017-0013
Zhao, A.-S., Zou, D., Wang, H.-H., Han, X., Yang, P., and Huang, N. (2019). Hydrogen sulphide-releasing aspirin enhances cell capabilities of anti-oxidative lesions and anti-inflammation. Med. Gas Res. 9, 145–152. doi: 10.4103/2045-9912.266990
Zhao, M.-X., Zhou, B., Ling, L., Xiong, X.-Q., Zhang, F., Chen, Q., et al. (2017). Salusin-beta contributes to oxidative stress and inflammation in diabetic cardiomyopathy. Cell Death Dis. 8, e2690. doi: 10.1038/cddis.2017.106
Zhou, H., Ding, L., Wu, Z., Cao, X., Zhang, Q., Lin, L., et al. (2017). Hydrogen sulfide reduces RAGE toxicity through inhibition of its dimer formation. Free Radic. Biol. Med. 104, 262–271. doi: 10.1016/j.freeradbiomed.2017.01.026
Zhou, X., An, G., and Lu, X. (2015). Hydrogen sulfide attenuates the development of diabetic cardiomyopathy. Clin. Sci. 128, 325–335. doi: 10.1042/CS20140460
Keywords: hydrogen sulfide, diabetic, cardiovascular diseases, diabetic cardiomyopathy, diabetic vascular diseases
Citation: Zhao S, Li X, Li X, Wei X and Wang H (2021) Hydrogen Sulfide Plays an Important Role in Diabetic Cardiomyopathy. Front. Cell Dev. Biol. 9:627336. doi: 10.3389/fcell.2021.627336
Received: 09 November 2020; Accepted: 04 January 2021;
Published: 18 February 2021.
Edited by:
Sandra Donnini, University of Siena, ItalyReviewed by:
Valentina Citi, University of Pisa, ItalyLara Testai, University of Pisa, Italy
Zhongjian Cheng, Temple University, United States
Copyright © 2021 Zhao, Li, Li, Wei and Wang. This is an open-access article distributed under the terms of the Creative Commons Attribution License (CC BY). The use, distribution or reproduction in other forums is permitted, provided the original author(s) and the copyright owner(s) are credited and that the original publication in this journal is cited, in accordance with accepted academic practice. No use, distribution or reproduction is permitted which does not comply with these terms.
*Correspondence: Honggang Wang, whg197167@vip.henu.edu.cn