- 1Department of Architectural Engineering, College of Engineering, University of Sharjah, Sharjah, United Arab Emirates
- 2Department of Interior Design, College of Archictecture, Art, and Design Healthy and Sustainable Built Environment Research Center Ajman University, Ajman, United Arab Emirates
In response to the growing concern of air pollution in Dubai, this study was undertaken to measure and analyze indoor and outdoor particulate matter (PM) concentrations in residential buildings during the spring dust storm period. The research focused on the infiltration of PM into indoor spaces and its impact on indoor air quality, exploring the relationship between PM particle diameter, building infiltration rates, and the indoor influence of outdoor PM. Conducted in a two-bedroom residential unit near a busy road, the study utilized particle size analysis and the indoor-outdoor (I/O) ratio for measurements. The findings revealed that smaller particles, particularly ultrafine PM2.5, had a more substantial influence on indoor PM concentrations than larger particles. It was noted that buildings with higher infiltration rates, especially those with natural ventilation, were more susceptible to outdoor PM infiltration. Additionally, the study highlighted the significant role of occupant behavior, such as cooking and cleaning, in generating indoor PM. However, further research is necessary to better understand the correlation between architectural characteristics, infiltration rates, and the indoor influence of outdoor PM in residential buildings. The study underscores the importance of improved ventilation systems, raising awareness of indoor air quality, and implementing effective mitigation strategies to reduce indoor air pollution and enhance indoor air quality in urban environments like Dubai. These findings contribute significantly to our understanding of indoor and outdoor PM dynamics, emphasizing the urgent need to address indoor air pollution in urban areas.
1 Introduction
Dubai places considerable importance on environmental consciousness, particularly about air quality and pollution (Jung and Awad, 2021a; Awad and Jung, 2021; Awad and Jung, 2022; Jung and Al Qassimi, 2022). The Dubai Municipality actively undertakes initiatives and arranges events to enhance environmental awareness, which likely encompasses educating individuals about the risks associated with PM2.5 and PM10 particles (Nasser et al., 2015; Benchrif et al., 2021a). To monitor and analyze air quality in real time, the UAE has implemented advanced tools such as the Air Quality Index (AQI) and the National Air Quality Platform (Jung et al., 2021a; Jung and Awad, 2021b). These platforms enable the public to assess the air quality in specific areas, comprehend the associated health hazards, and make informed decisions regarding visits based on air quality data (Arar and Jung, 2021; Mahmoud et al., 2023). Furthermore, the UAE has introduced the National Air Quality Agenda 2031, which establishes a comprehensive framework for monitoring and managing air quality while concurrently reducing air pollution (Abbas et al., 2021; Al-Dabbagh, 2021).
Despite these efforts, the PM2.5 concentration in Dubai is currently reported to be 15 times more than the World Health Organization’s (WHO) annual air quality guideline value, indicating that there is still a significant challenge to overcome in terms of air pollution in the city (Mushtaha and Helmy, 2017).
The sources of air pollution in the UAE are diverse, with industry accounting for just over two-thirds of PM2.5 and road transport causing almost one-fifth (Mushtaha et al., 2021a). Power generation and desalination cause about 3% (Bani Mfarrej et al., 2020). Dust storms, carrying sand and sea salt, are another significant source of Particulate Matter (PM) in the UAE (Jung et al., 2021b).
Air pollution, particularly PM2.5, is associated with serious health conditions such as lung cancer and cardiovascular disease (Chen et al., 2017; Al-Hemoud et al., 2019; Mushtaha et al., 2021b; González-Lezcano, 2023). Short-term exposure can increase the risk of asthma, chronic obstructive pulmonary disease, and respiratory infection (Amoatey et al., 2020). Reports have indicated that more than 120,000 people die prematurely each year in the Middle East and North Africa (MENA) region because of air pollution, equating to 7% of premature deaths (Benchrif et al., 2021b; Arar and Jung, 2022). Given the ongoing trajectory of sustained economic expansion and insufficient environmental safeguard measures, the expeditious resolution of the decline in atmospheric conditions caused by PM poses a formidable challenge (Arar et al., 2022).
The degradation of the atmospheric environment also contributes to the rise in indoor PM concentrations (Jung and El Samanoudy, 2023). The interior of a building undergoes a continuous exchange of air with the external environment through ventilation and infiltration (Abdelaziz Mahmoud and Jung, 2023). During this process, PM infiltrates the indoor spaces through crevices or openings in the building’s structure and windows (Jung et al., 2022a). The extent of its impact within a room depends on the specific pathways these particulates penetrate (Al Qassimi and Jung, 2022).
In contrast to numerous commercial buildings where the air is filtered through air conditioning systems before being supplied to the indoor spaces during the introduction of outside air, residential buildings typically rely on infiltration and natural ventilation (Jung et al., 2021c; Jung et al., 2022b; Jung et al., 2022c). While there has been a recent surge in the construction of residential buildings equipped with mechanical ventilation systems, residents’ reliance on such systems remains relatively low (Jung et al., 2022c). Of particular significance is the fact that elderly individuals and infants, who are more susceptible to respiratory ailments, often reside in residential buildings for extended periods (Kharrufa et al., 2022). Therefore, evaluating the indoor effects of outdoor PM in residential buildings can be deemed of utmost importance (Huang et al., 2018).
Prior research has predominantly concentrated on multi-purpose establishments like daycare centers, and there exists only a limited number of studies on measurement and simulation methodologies (Jung and Mahmoud, 2022). While a published study has examined the inflow of PM through the ventilation system in residential buildings, assessing its impact through infiltration remains constrained (Hussien et al., 2023a). Recently, there have been investigations into the penetration of PM via natural infiltration in office buildings (Elsayed et al., 2021). However, exploring PM penetration targeting residential buildings is still nascent (Stasiulaitiene et al., 2019a; Sultan et al., 2020).
Hence, this study aims to measure and analyze indoor and outdoor PM status during the spring dust storm period in residential buildings along roadways, where a substantial inflow of PM into indoor spaces is anticipated. Furthermore, it seeks to evaluate the extent of indoor influence caused by outdoor PM. To scrutinize the impact of outdoor PM on Indoor Air Quality (IAQ), a particle size analysis was conducted based on the indoor-outdoor concentration ratio, commonly referred to as the (I/O ratio).
2 Materials and methods
2.1 Example of PM I/O ratio measurement in residential buildings
As shown in Table 1, numerous researchers have conducted measurements of the indoor-outdoor (I/O) ratio to examine the correlation between outdoor and indoor PM (Hussien et al., 2023b). The results of these measurements have revealed a wide range of I/O ratios (Wang et al., 2016a).
In Dai et al. (2018) study, the I/O ratio of PM2.5 was analyzed during winter and summer for 18 residential units. Bralewska et al. (2019) investigated the I/O ratio of PM2.5 in high-traffic areas, distinguishing between residential dwellings’ heating and non-heating periods. Gao et al. (2021) assessed pollutant levels, such as PM2.5 concentration, where similar residential units were situated in the city center and suburbs, exhibiting significant traffic volume variations. The study examined the influence of pollution sources.
Nevertheless, it is worth noting that the I/O ratio varies depending on PM diameter (Jodeh et al., 2018). The studies above only present the I/O ratio for specific diameter groups of fine dust, thereby lacking information on the I/O ratios corresponding to various fine dust diameters (Wang et al., 2016b). Conversely, some studies have specifically analyzed the I/O ratio based on PM diameter (Ji et al., 2018). Tran et al. (2021) investigated PM1, PM2.5, and PM10 concentrations in 50 residential units. Faria et al. (2020) conducted monitoring of PM1, PM2.5, and PM10 in five dwellings near roadways and five urban dwellings. Ścibor et al. (2020) analyzed PM1, PM2.5, and PM10 concentrations in 19 city and suburban residential units.
However, it is important to note that the primary objective of these studies is to evaluate indoor PM while considering occupant behavior (Hodas et al., 2016). Moreover, these studies do not distinctly differentiate between residential and non-residential periods regarding the I/O ratio (Krstić et al., 2016). Consequently, there are limitations in determining the extent of influence of outdoor PM solely based on the provided measurement values (Jung and Awad, 2023).
2.2 Outdoor PM10 concentrations in Dubai
Figure 1 displays the concentration patterns of PM in the outdoor air of Dubai, where the designated residential location for the measurement experiment is situated (Taleb and Kayed, 2021). The data from the Dubai Municipality, encompassing the years 2014–2017, were analyzed to examine the concentrations of PM particles, temperature, and humidity (Klepeis et al., 2001). This analysis aimed to determine the suitable experimental period for data analysis in this study (Azimi et al., 2018a; Singer and Delp, 2018).
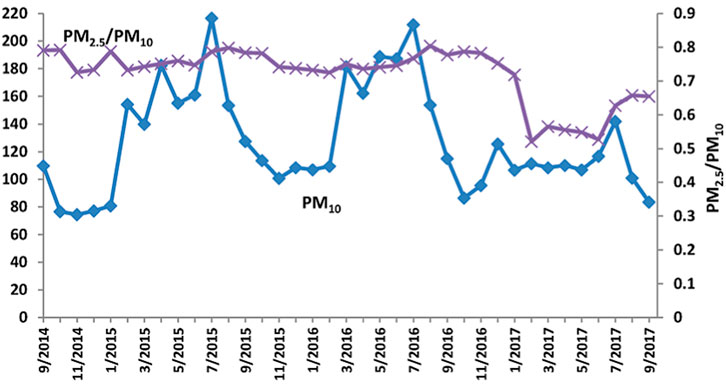
FIGURE 1. Annual variations in PM10 concentrations and PM2.5/PM10 ratio in Dubai between 2014 and 2017.
Given the specific attributes of the designated area, meticulous planning was undertaken for the experiment, with a concentrated focus on July, during which elevated levels of outdoor PM10 are anticipated (Jung et al., 2019).
2.3 Methodology for measurement of indoor and outdoor PM and indoor environment
2.3.1 Target building
The target is a 127 m2 two-bedroom unit in Noora, Al Habtoor City building (Figure 2), in Business Bay, Dubai (Propsearch.ae. Al, 2021). The floor plan of the specific area to be measured is presented in Figure 3.
Architectural Layout Analysis: The residential unit under investigation boasts a strategic architectural layout integral to understanding potential PM infiltration dynamics. With two balconies, multiple windows, a lengthy entrance corridor, and three measurement locations near conceivable PM entry points (as depicted in the provided floor plan), the design and positioning of these elements could significantly influence PM infiltration rates. This is especially pertinent considering their proximity to potential outdoor PM sources and entry pathways. Balconies, particularly, can serve as primary ingress points if frequently left open. Additionally, the extended entrance corridor may channel outdoor PM, especially during recurrent door operations. The floor plan thus offers invaluable insights into the potential regions of PM concentration and its relation to architectural features Figure 3.
The targeted building is positioned adjacent to a bustling 14-lane Sheikh Zayed Road, implying that the exterior concentration of PM is expected to be substantial, owing to the continuous vehicular traffic outside commuting hours (Azimi et al., 2018b; Otuyo et al., 2023). The 14-lane thoroughfare runs parallel to the southern side of the apartment complex, with an approximate distance of 20 m separating the two. It was essential to consider the outdoor concentration levels to ensure that the evaluation of indoor penetration of outdoor PM is discernible (Tham et al., 2021). Hence, the measurement time and location were carefully selected (Fermo et al., 2021).
During the measurement periods, a log was maintained of various activities conducted by the occupants, including cooking, cleaning, smoking, and window/door usage. While comprehensive profiling of each family’s habits and the number of occupants was not conducted in this study, we observed and noted predominant indoor activities.
2.3.2 Indoor and outdoor PM measurement methods and conditions
Measurements were conducted to assess the PM levels indoors, specifically in a central living room, and outdoors at a designated location (Raysoni et al., 2016). It should be noted that the extent of indoor penetration of outdoor PM varies depending on the diameter of the particles (Wang et al., 2016c).
To obtain accurate data, Particle Number Concentration (PNC) was measured using two Optical Particle Counters (TSI 9306-v2, United States) for different particle diameters: 0.3, 0.5, 1.0, 5.0, and 10.0 μm (Molho et al., 2019). Given the substantial fluctuations in the quantity and concentration of PM over time, a short collection interval of 5 min was set to capture these variations effectively (Etzion and Broday, 2018).
During the occupancy of the residential unit, occupants were allowed to engage in routine activities such as cooking, cleaning, and ventilation without any specific restrictions (Pietrogrande et al., 2021). Additionally, the duration of non-occupancy was recorded separately (Zhao et al., 2018). Based on the collected measurements, the indoor-outdoor (I/O) ratio was calculated for both the entire measurement period and the non-occupancy period (Silva et al., 2017). By considering the I/O ratio over the whole measurement period, which accounts for fine particulate matter generation from various sources, such as outdoor penetration and indoor generation, valuable insights into the overall trends of fine dust generation within the actual space can be obtained (Singer et al., 2020). Moreover, by analyzing the I/O ratio during the non-occupancy period, the impact of external fine dust penetration on the indoor fine dust concentration can be assessed and understood (Santin et al., 2021).
While the measurements account for indoor PM sources like cooking and cleaning, they also inherently consider PM from outdoor sources, thus providing an I/O ratio that simulates the combined effects of both ventilation (intentional introduction of outdoor air) and infiltration (unintentional leakage of outdoor air into a building). This dynamic evaluation ensures that the concentrations recorded are representative of both active ventilation processes and passive infiltration processes.
2.3.3 Infiltration rate estimation
The indoor-outdoor (I/O) ratio of PM in the measured household can be influenced by the infiltration rate during the measurement period (Stasiulaitiene et al., 2019b). To estimate the infiltration rate of the target unit during the PM period, the infiltration rate using carbon monoxide (CO) gas generated by occupants was employed (Peng et al., 2017). Previous studies have proposed a correlation between the detailed methods and procedures of the occupant-generated CO2 gas method and other tracer gas methods (Remion et al., 2019). This study used the same way as previous studies suggested to estimate the infiltration rate. The carbon dioxide (CO2) concentration within the unit was measured using a CO2 monitor (TSI 7545, United States) placed at the center of the household (Savdie et al., 2020). These measurements were recorded every minute. The collected CO2 data served not only to estimate the infiltration rate but also to verify the occupancy and non-occupancy periods of the residents (Asif and Zeeshan, 2020).
Carbon dioxide emissions based on the number of occupants in the target space were calculated using Formula 1 (Zhong et al., 2019).
VO2: Oxygen Consumption Rate (L/s).
RQ: Respiratory Coefficient (−)
M: Amount of Activity (met).
AD: DuBois Body Surface Area (m2).
During the estimation of the infiltration rate for the target unit, it was assumed that the occupants resided in the space for a sufficient duration, allowing for the complete diffusion of CO gas indoors (Militello-Hourigan and Miller, 2018). The air exchange rate was determined by calculating the minimal difference between the indoor CO2 concentration value measured using the least square method and the indoor CO2 concentration value estimated using the mass conservation equation (Zhu et al., 2019). This calculated air exchange rate represents the infiltration rate of the unit (Formula 2).
V: Room Volume (m3).
t: Time (h).
Q: Air Exchange Rate (m3/h).
C: CO2 Concentration (g/m3).
C0: Outdoor CO2 Concentration (g/m3).
Ci: Indoor CO2 Concentration (g/m3).
G: Amount of Tracer Gas (g/h).
In our attempt to simulate real-world conditions, the infiltration rate is a pivotal factor, representing the unintended air exchange between indoor and outdoor environments. Using occupant-generated CO2 as a tracer reflects the building’s actual conditions, incorporating both natural ventilation and the effects of building envelope integrity. The calculated air exchange rate, thus, does not just consider the mechanical or active introduction of fresh air but also the inherent building characteristics that allow air to infiltrate. In combining both the I/O PM measurements and the infiltration rate estimations, our methodology captures a multi-faceted view of air quality dynamics within residential units, accounting for both intentional ventilation processes and unintentional infiltration events. This ensures a comprehensive representation of air quality in real-life living scenarios.
3 Results
3.1 Concentration measurement result by indoor and outdoor diameter
Figure 4 illustrates the days (Day 1–18 July 2022, Day 2 - 22 July 2022, and Day 3 - 26 July 2022) during the measurement period when the concentration of PM in the outdoor air was notably high, thereby revealing its impact on indoor fine particulate matter concentration. The figure showcases the number of outdoor and indoor PM particles categorized by diameter. The characteristics of these 3 days are segmented based on variations in the outdoor air concentration of PM. Day 1 observed a high outdoor air concentration in the morning, followed by a gradual decrease over time. Conversely, Day 2 and Day 3 experienced low outdoor air concentrations in the morning, which progressively increased as the day unfolded. Day 2 and Day 3 displayed differences in infiltration rates.
As presented in Table 2, the infiltration rate on July 22 was recorded as 0.11 times per hour, a lower value than Day 3’s 0.19 times per hour. For Day 1 and Day 3, when the infiltration rates were 0.20 times per hour and 0.19 times per hour, respectively, the indoor PM concentration generally tended to follow the fluctuations in outdoor concentration. However, on Day 2, with an infiltration rate of 0.11 times per hour, this correlation was more evident, indicating a clear relationship. Furthermore, it can be observed that the disparity between outdoor and indoor number concentrations of PM diminishes as the particle size decreases from larger-sized fine particles to smaller ones.
3.2 Indoor/outdoor PM concentration ratio
For a quantitative comparison of the variation in the number and concentration of delicate particulate matter between indoor and outdoor environments, the indoor-outdoor (I/O) ratio was analyzed, as demonstrated in Figure 5 and Figure 6. Figure 5 presents the I/O ratio encompassing both occupancy and non-occupancy periods, while Figure 6 exclusively showcases the I/O ratio during non-occupancy periods. As depicted in Figure 5, the I/O ratio occasionally exceeds 1.0. This is attributed to the influence of PM generated indoors due to occupants’ indoor activities. These findings indicate that individuals tend to seal windows during high outdoor dust concentrations, inadvertently causing an increase in indoor PM concentration. Consequently, the indoor air quality may be poorer than the outdoor environment. It is worth noting that due to the experimental design lacking systematic control over occupants’ activities, it is challenging to quantitatively analyze the individual impact of the I/O ratio resulting from each occupant’s behavior. However, the analysis reveals that the I/O ratio is notably high for PM with a diameter smaller than 0.5 μm.
Figure 6 illustrates the analysis of the indoor-outdoor (I/O) ratio during the non-occupancy period, excluding occupant behavior’s influence. The I/O ratio is consistently below 1.0 for all fine dust diameter groups. As explained earlier, the results shown in Figure 6 primarily reflect the impact of indoor penetration of fine dust from the outdoor environment. It can be observed that the effect on the indoor climate varies depending on the diameter of the PM. Some of the external fine dust infiltrates the room through gaps in the building, while some accumulates on the surface of these gaps. The experimental findings analyzed a higher I/O ratio for smaller dust diameters, indicating that smaller particles tend to deposit less on the gap surfaces and penetrate more into the room. On Day 3, the average I/O ratios for the diameters of 0.3, 0.5, and 1.0 μm were notably high at 0.77, 0.80, and 0.53, respectively. In contrast, larger dust particles with diameters of 3.0, 5.0, and 10.0 μm exhibited lower average I/O ratios of 0.30, 0.30, and 0.47, respectively.
This suggests a more significant concern for indoor air pollution due to the indoor infiltration of outdoor fine dust, particularly fine dust with diameters classified as PM2.5 or smaller, which falls under ultrafine dust.
On Day 2, when the infiltration rate was low, the I/O ratio was lower for all diameter groups than Day 3. The average I/O ratios for the diameters of 0.3, 0.5, 1.0, 3.0, 5.0, and 10.0 μm were 0.26, 0.19, 0.11, 0.03, 0.02, and 0.00, respectively. These findings demonstrate that the degree of indoor influence due to the infiltration of external fine dust is significantly affected by fluctuations in the infiltration rate.
Based on these findings, it can be concluded that the indoor penetration of outdoor PM in residential buildings is influenced by the diameter of the particles and the infiltration rate of the target unit. Notably, in the ultrafine dust category, which includes particles classified as PM2.5, the impact of outdoor PM indoors was significant. This emphasizes the importance of conducting further comprehensive research in this area. Moreover, the study revealed that outdoor PM’s indoor influence is linked to residential building infiltration rate. Consequently, it is recommended that future investigations be conducted to elucidate the correlation between architectural characteristics, which affect the infiltration rate, and the indoor influence of outdoor PM.
4 Discussion
The study results indicate significant penetration of outdoor PM into residential buildings in Dubai, particularly during periods of high outdoor PM concentrations. The indoor-outdoor (I/O) ratio analysis showed that the I/O ratio occasionally exceeds 1.0, indicating that indoor PM concentration can be higher than outdoor levels. This is attributed to PM generated indoors due to occupants’ activities, such as cooking, cleaning, and window sealing during high outdoor PM concentrations.
Compared to prior research, our findings are consistent with those observed in urban environments where indoor PM concentrations often exceeded outdoor levels due to specific indoor activities (Wang et al., 2016a; Dai et al., 2018; Hussien et al., 2023b).
The study also found that the indoor penetration of outdoor PM is influenced by the diameter of the particles and the infiltration rate of the building. Smaller particles, classified as ultrafine dust (PM2.5 or smaller), tend to penetrate more into the indoor environment than larger particles. Previous research has similarly highlighted the differential penetration of PM based on particle size (Jodeh et al., 2018; Bralewska et al., 2019; Gao et al., 2021). The infiltration rate, influenced by factors such as building design and ventilation, also plays a significant role in the indoor influence of outdoor PM.
Our observations from the measurement periods for the influence of ultrafine PM2.5 on indoor concentrations underline the pronounced influence of ultrafine PM2.5 in indoor environments, particularly in a residential unit near a traffic-dense street. In alignment with earlier studies, vehicular emissions, especially from diesel vehicles, are identified as a significant contributor to indoor PM2.5 concentrations (Wang et al., 2016b). Several factors may account for this notable influence.
Firstly, the proximity of the residential unit to a bustling street exposes it to increased concentrations of vehicular emissions. Diesel vehicles, particularly, are renowned for emitting significant quantities of ultrafine PM2.5. These minute particles can stay airborne for prolonged durations, thus leading to their sustained presence indoors.
The ultrafine nature of PM2.5 particles facilitates their more efficient penetration into indoor spaces. Their capability to navigate through gaps and cracks has also been emphasized in previous research (Taleb and Kayed, 2021). They can navigate through gaps, cracks, and even conventional ventilation systems that might not be equipped to trap these smaller-sized particles efficiently. In addition, certain indoor activities, such as cooking, can be a source of ultrafine particle generation, further elevating indoor concentrations. Additionally, our study reaffirms the findings from other studies that indoor activities, such as cooking, play a vital role in generating ultrafine particles (Wang et al., 2016c).
This observed trend highlights the critical importance of devising effective mitigation measures, especially for residences near high-traffic zones. Enhanced filtration systems and awareness about indoor activities that contribute to ultrafine PM2.5 generation can be key strategies to maintain healthier indoor air quality.
Our findings on indoor PM concentrations are influenced not just by outdoor sources but also by various indoor activities and the profile of occupants. Cooking, cleaning, and smoking can significantly alter indoor PM levels. The number and demographics of the occupants, their habits, and lifestyle choices play a pivotal role in determining indoor air quality. Our findings on indoor PM concentrations, though unique in Dubai, share parallels with global observations on the influence of both outdoor sources and indoor activities. As echoed in earlier research, the demographics and habits of occupants are instrumental in shaping indoor air quality (Etzion and Broday, 2018).
While our study provides insights into the influence of some of these factors, a more detailed analysis based on individual family profiles and habits would provide a clearer picture. Such an analysis could be valuable for future research to devise effective mitigation strategies for indoor air pollution.
Based on these findings, it is recommended that further research be conducted to explore the correlation between architectural characteristics, infiltration rates, and the indoor influence of outdoor PM in residential buildings. Understanding how building design and ventilation systems affect the infiltration of outdoor PM can help develop strategies to mitigate indoor air pollution and improve indoor air quality. Additionally, future studies should consider different seasons and locations to account for variations in outdoor PM concentrations and sources.
The results of this study contribute to the understanding of the indoor-outdoor relationship of PM in residential buildings and highlight the need for measures to address indoor air pollution in Dubai. Given the high levels of PM2.5 concentration in the city and its associated health risks, efforts should be made to improve ventilation systems, promote awareness among residents about indoor air quality, and implement effective mitigation strategies to reduce the penetration of outdoor PM into indoor environments.
5 Conclusion
This study aimed to assess the impact of outdoor particulate matter (PM) on indoor PM concentrations in residential buildings in Dubai and explore the relationship between the infiltration rate and the indoor influence of external PM based on particle diameter. The findings of this study have significant implications for understanding and addressing indoor air pollution in Dubai. The study’s results revealed that smaller particles, particularly ultrafine dust such as PM2.5, had a more pronounced influence on indoor PM levels than larger particles. This suggests that the indoor penetration of outdoor PM is size-dependent, with smaller particles being more likely to infiltrate indoor spaces. The higher degree of indoor impact observed for ultrafine dust emphasizes the importance of addressing PM2.5 concentrations, which are associated with adverse health effects such as respiratory ailments and cardiovascular diseases.
Furthermore, the study demonstrated that the infiltration rate, influenced by architectural characteristics such as building design and ventilation systems, played a significant role in the indoor influence of outdoor PM. Residential buildings, particularly those relying on natural ventilation rather than mechanical ventilation systems, are more susceptible to outdoor PM infiltration.
The implications of this study extend beyond the specific context of Dubai. Indoor air pollution is a global concern, and residential buildings are often overlooked in air quality studies compared to commercial or industrial settings. This study fills the knowledge gap by shedding light on indoor and outdoor PM dynamics in residential buildings. It emphasizes the need to address indoor air pollution in various urban environments.
In urban environments, effectively mitigating indoor air pollution requires a multifaceted approach. Source control stands out as the premier method; by eliminating or managing pollutant sources, such as choosing low-emission construction materials and household products and ensuring proper maintenance of heating and cooking equipment, significant strides can be made. Enhancing the influx of fresh air through improved ventilation—be it by frequently opening windows, utilizing exhaust fans, or deploying mechanical air supply systems—can drastically lower indoor pollutants. For targeted pollutant removal, various air cleaners are available, from compact table-top versions to expansive whole-house systems; their efficacy lies in their ability to filter out specific particles and gaseous contaminants when used correctly. Regular maintenance and cleaning of HVAC systems, complemented by routine dusting and vacuuming with HEPA filters, curtails indoor pollution accumulation. Additionally, integrating certain indoor plants, such as spider or rubber plants, can act as natural purifiers by reducing contaminants like benzene and formaldehyde. However, the bedrock of any successful mitigation strategy remains awareness and education. By keeping residents informed about the origins of indoor pollution, its detrimental effects, and potential countermeasures, they can make informed decisions about indoor practices and product choices.
To further validate and expand upon the findings of this study, future research should investigate various types of residential buildings, considering different architectural characteristics and geographical locations. A more comprehensive understanding of the indoor-outdoor relationship of PM can be achieved by considering variations in outdoor PM concentrations and sources across seasons and regions. Based on the results of this study, several recommendations can be made to mitigate indoor air pollution in residential buildings.
1. Improving ventilation systems in existing and new residential constructions can help reduce the infiltration of outdoor PM. This can involve installing adequate air filtration systems, promoting mechanical ventilation systems, or adopting energy-efficient building designs that balance ventilation and energy consumption.
2. Raising awareness among residents about indoor air quality and the potential health risks associated with PM exposure is crucial. Education campaigns and informational materials can guide indoor air quality management, including proper ventilation practices, regular cleaning, and air purifiers or filters. Encouraging residents to seal windows and doors during periods of high outdoor PM concentrations can also minimize the infiltration of PM into indoor spaces.
3. Implementing effective mitigation strategies at a broader scale is essential. This includes developing and enforcing air quality regulations and policies targeting outdoor and indoor PM pollution sources. Collaborative efforts involving government agencies, urban planners, architects, and building developers can lead to the design and construction of more sustainable and healthy residential buildings.
4. The findings underscore the importance of revisiting and enhancing ventilation systems in residential settings. Effective ventilation not only mitigates the incursion of outdoor pollutants but also plays an essential role in promoting better indoor air quality, which is imperative for residents’ health and wellbeing.
This study contributes to our understanding of the impact of outdoor PM on indoor PM concentrations in residential buildings. The findings emphasize the importance of addressing indoor air pollution, particularly in urban environments like Dubai, where high levels of PM2.5 concentration pose significant health risks. By considering the infiltration rate and architectural characteristics, future research can further explore strategies to mitigate the penetration of outdoor PM and improve indoor air quality. Implementing these measures can contribute to creating healthier and more sustainable living environments for residents in residential buildings worldwide.
Data availability statement
The raw data supporting the conclusion of this article will be made available by the authors, without undue reservation.
Author contributions
CJ: Conceptualization, Data curation, Formal Analysis, Investigation, Methodology, Project administration, Writing–original draft. NA: Conceptualization, Investigation, Resources, Software, Supervision, Validation, Visualization, Writing–review and editing.
Funding
The author(s) declare that no financial support was received for the research, authorship, and/or publication of this article.
Acknowledgments
The authors would like to express their gratitude to University of Sharjah and Ajman University for APC support and providing great research environment.
Conflict of interest
The authors declare that the research was conducted in the absence of any commercial or financial relationships that could be construed as a potential conflict of interest.
Publisher’s note
All claims expressed in this article are solely those of the authors and do not necessarily represent those of their affiliated organizations, or those of the publisher, the editors and the reviewers. Any product that may be evaluated in this article, or claim that may be made by its manufacturer, is not guaranteed or endorsed by the publisher.
References
Abbas, S., Anser, M. K., Nassani, A. A., Haffar, M., and Zaman, K. (2021). Security challenges and air quality management in India: emissions inventory and forecasting estimates. Atmosphere 12 (12), 1644. doi:10.3390/atmos12121644
Abdelaziz Mahmoud, N. S., and Jung, C. (2023). Analyzing the bake-out effect in winter for the enhancement of indoor air quality at new apartments in UAE. Buildings 13 (4), 846. doi:10.3390/buildings13040846
Al-Dabbagh, R. (2021). Waste management strategy and development in Ajman, UAE. Renew. Energy Environ. Sustain. 6, 14. doi:10.1051/rees/2021005
Al-Hemoud, A., Gasana, J., Al-Dabbous, A., Alajeel, A., Al-Shatti, A., Behbehani, W., et al. (2019). Exposure levels of air pollution (PM2. 5) and associated health risk in Kuwait. Environ. Res. 179, 108730. doi:10.1016/j.envres.2019.108730
Al Qassimi, N., and Jung, C. (2022). Impact of air-purifying plants on the reduction of volatile organic compounds in the indoor hot desert climate. Front. Built Environ. 7, 188. doi:10.3389/fbuil.2021.803516
Amoatey, P., Sicard, P., De Marco, A., and Khaniabadi, Y. O. (2020). Long-term exposure to ambient PM2. 5 and impacts on health in Rome, Italy. Clin. Epidemiol. Glob. Health 8 (2), 531–535. doi:10.1016/j.cegh.2019.11.009
Arar, M., and Jung, C. (2021). Improving the indoor air quality in nursery buildings in United Arab Emirates. Int. J. Environ. Res. Public Health 18 (22), 12091. doi:10.3390/ijerph182212091
Arar, M., and Jung, C. (2022). Analyzing the perception of indoor air quality (IAQ) from a survey of new townhouse residents in Dubai. Sustainability 14 (22), 15042. doi:10.3390/su142215042
Arar, M., Jung, C., and Qassimi, N. A. (2022). Investigating the influence of the building material on the indoor air quality in apartment in Dubai. Front. Built Environ. 7, 804216. doi:10.3389/fbuil.2021.804216
Asif, A., and Zeeshan, M. (2020). Indoor temperature, relative humidity and CO2 monitoring and air exchange rates simulation utilizing system dynamics tools for naturally ventilated classrooms. Build. Environ. 180, 106980. doi:10.1016/j.buildenv.2020.106980
Awad, J., and Jung, C. (2021). Evaluating the indoor air quality after renovation at the Greens in Dubai, United Arab Emirates. Buildings 11 (8), 353. doi:10.3390/buildings11080353
Awad, J., and Jung, C. (2022). Extracting the planning elements for sustainable urban regeneration in Dubai with AHP (analytic hierarchy process). Sustain. Cities Soc. 76, 103496. doi:10.1016/j.scs.2021.103496
Azimi, P., Zhao, H., Fazli, T., Zhao, D., Faramarzi, A., Leung, L., et al. (2018a). Pilot study of the vertical variations in outdoor pollutant concentrations and environmental conditions along the height of a tall building. Build. Environ. 138, 124–134. doi:10.1016/j.buildenv.2018.04.031
Azimi, P., Zhao, H., Fazli, T., Zhao, D., Faramarzi, A., Leung, L., et al. (2018b). Pilot study of the vertical variations in outdoor pollutant concentrations and environmental conditions along the height of a tall building. Build. Environ. 138, 124–134. doi:10.1016/j.buildenv.2018.04.031
Bani Mfarrej, M. F., Qafisheh, N. A., and Bahloul, M. M. (2020). Investigation of indoor air quality inside houses from UAE. Air, Soil Water Res. 13, 117862212092891. doi:10.1177/1178622120928912
Benchrif, A., Wheida, A., Tahri, M., Shubbar, R. M., and Biswas, B. (2021a). Air quality during three covid-19 lockdown phases: AQI, PM2. 5 and NO2 assessment in cities with more than 1 million inhabitants. Sustain. Cities Soc. 74, 103170. doi:10.1016/j.scs.2021.103170
Benchrif, A., Wheida, A., Tahri, M., Shubbar, R. M., and Biswas, B. (2021b). Air quality during three covid-19 lockdown phases: AQI, PM2. 5 and NO2 assessment in cities with more than 1 million inhabitants. Sustain. Cities Soc. 74, 103170. doi:10.1016/j.scs.2021.103170
Bralewska, K., Rogula-Kozłowska, W., and Bralewski, A. (2019). Size-segregated particulate matter in a selected sports facility in Poland. Sustainability 11 (24), 6911. doi:10.3390/su11246911
Chen, L., Shi, M., Gao, S., Li, S., Mao, J., Zhang, H., et al. (2017). Assessment of population exposure to PM2. 5 for mortality in China and its public health benefit based on BenMAP. Environ. Pollut. 221, 311–317. doi:10.1016/j.envpol.2016.11.080
Dai, X., Liu, J., Li, X., and Zhao, L. (2018). Long-term monitoring of indoor CO2 and PM2. 5 in Chinese homes: concentrations and their relationships with outdoor environments. Build. Environ. 144, 238–247. doi:10.1016/j.buildenv.2018.08.019
Elsayed, Y., Kanan, S., and Farhat, A. (2021). Meteorological patterns, technical validation, and chemical comparison of atmospheric dust depositions and bulk sand in the Arabian Gulf region. Environ. Pollut. 269, 116190. doi:10.1016/j.envpol.2020.116190
Etzion, Y., and Broday, D. M. (2018). Highly resolved spatiotemporal variability of fine particle number concentrations in an urban neighborhood. J. Aerosol Sci. 117, 118–126. doi:10.1016/j.jaerosci.2018.01.004
Faria, T., Martins, V., Correia, C., Canha, N., Diapouli, E., Manousakas, M., et al. (2020). Children's exposure and dose assessment to particulate matter in Lisbon. Build. Environ. 171, 106666. doi:10.1016/j.buildenv.2020.106666
Fermo, P., Artíñano, B., De Gennaro, G., Pantaleo, A. M., Parente, A., Battaglia, F., et al. (2021). Improving indoor air quality through an air purifier able to reduce aerosol particulate matter (PM) and volatile organic compounds (VOCs): experimental results. Environ. Res. 197, 111131. doi:10.1016/j.envres.2021.111131
Gao, Y., Wang, Z., Li, C. Y., Zheng, T., and Peng, Z. R. (2021). Assessing neighborhood variations in ozone and PM2. 5 concentrations using decision tree method. Build. Environ. 188, 107479. doi:10.1016/j.buildenv.2020.107479
González-Lezcano, R. A. (2023). Editorial: design of efficient and healthy buildings. Front. Built Environ. 9. doi:10.3389/fbuil.2023.1210956
Hodas, N., Loh, M., Shin, H. M., Li, D., Bennett, D., McKone, T. E., et al. (2016). Indoor inhalation intake fractions of fine particulate matter: review of influencing factors. Indoor air 26 (6), 836–856. doi:10.1111/ina.12268
Huang, K., Song, J., Feng, G., Chang, Q., Jiang, B., Wang, J., et al. (2018). Indoor air quality analysis of residential buildings in northeast China based on field measurements and longtime monitoring. Build. Environ. 144, 171–183. doi:10.1016/j.buildenv.2018.08.022
Hussien, A., Jannat, N., Mushtaha, E., and Al-Shammaa, A. (2023b). A holistic plan of flat roof to green-roof conversion: towards a sustainable built environment. Ecol. Eng. 190, 106925. doi:10.1016/j.ecoleng.2023.106925
Hussien, A., Saleem, A. A., Mushtaha, E., Jannat, N., Al-Shammaa, A., Ali, S. B., et al. (2023a). A statistical analysis of life cycle assessment for buildings and buildings’ refurbishment research. Ain Shams Eng. J. 14, 102143. doi:10.1016/j.asej.2023.102143
Ji, W., Li, H., Zhao, B., and Deng, F. (2018). Tracer element for indoor PM2. 5 in China migrated from outdoor. Atmos. Environ. 176, 171–178. doi:10.1016/j.atmosenv.2017.12.034
Jodeh, S., Hasan, A. R., Amarah, J., Judeh, F., Salghi, R., Lgaz, H., et al. (2018). Indoor and outdoor air quality analysis for the city of Nablus in Palestine: seasonal trends of PM 10, PM 5.0, PM 2.5, and PM 1.0 of residential homes. Air Qual. Atmos. Health 11, 229–237. doi:10.1007/s11869-017-0533-5
Jung, C., and Al Qassimi, N. (2022). Investigating the emission of hazardous chemical substances from mashrabiya used for indoor air quality in hot desert climate. Sustainability 14 (5), 2842. doi:10.3390/su14052842
Jung, C., Al Qassimi, N., Abdelaziz Mahmoud, N. S., and Lee, S. Y. (2022b). Analyzing the housing consumer preferences via analytic hierarchy process (AHP) in Dubai, United Arab Emirates. Behav. Sci. 12 (9), 327. doi:10.3390/bs12090327
Jung, C., Al Qassimi, N., Arar, M., and Awad, J. (2021b). The analysis of indoor air pollutants from finishing material of new apartments at business Bay, Dubai. Front. Built Environ. 7, 765689. doi:10.3389/fbuil.2021.765689
Jung, C., Al Qassimi, N., Arar, M., and Awad, J. (2022c). The improvement of user satisfaction for two urban parks in Dubai, UAE: Bay avenue park and Al ittihad park. Sustainability 14 (6), 3460. doi:10.3390/su14063460
Jung, C., Alqassimi, N., and El Samanoudy, G. (2022a). Evaluating the adsorption performance of functional building material with HCHO remover. Front. Built Environ. 179. doi:10.3389/fbuil.2022.998872
Jung, C., and Awad, J. (2021a). The improvement of indoor air quality in residential buildings in Dubai, UAE. Buildings 11 (6), 250. doi:10.3390/buildings11060250
Jung, C., and Awad, J. (2021b). Improving the IAQ for learning efficiency with indoor plants in university classrooms in Ajman, United Arab Emirates. Buildings 11 (7), 289. doi:10.3390/buildings11070289
Jung, C., and Awad, J. (2023). Sharjah sustainable city: an analytic hierarchy process approach to urban planning priorities. Sustainability 15 (10), 8217. doi:10.3390/su15108217
Jung, C., Awad, J., and Al Qassimi, N. (2021c). Evaluation of residents’ comfort in high-rise residential buildings in Dubai, United Arab Emirates. Front. Built Environ. 145. doi:10.3389/fbuil.2021.766057
Jung, C., Awad, J., Sami Abdelaziz Mahmoud, N., and Salameh, M. (2021a). An analysis of indoor environment evaluation for the Springs development in Dubai, UAE. Open House Int. 46 (4), 651–667. doi:10.1108/ohi-11-2020-0165
Jung, C., and El Samanoudy, G. (2023). Mitigating indoor air pollution in university dormitory: the need for better ventilation and resident awareness. Buildings 13 (5), 1144. doi:10.3390/buildings13051144
Jung, C., and Mahmoud, N. S. A. (2022). Extracting the critical points of formaldehyde (HCHO) emission model in hot desert climate. Air, Soil Water Res. 15, 117862212211050. doi:10.1177/11786221221105082
Jung, M., Cho, D., and Shin, K. (2019). The impact of particulate matter on outdoor activity and mental health: a matching approach. Int. J. Environ. Res. Public Health 16 (16), 2983. doi:10.3390/ijerph16162983
Kharrufa, S. N., Awad, J., Jung, C., and Sherzad, M. (2022). Evaluating an active low-energy cooling upgrade to the building envelope in the hot climates of the Middle East. Int. J. Low-Carbon Technol. 17, 118–129. doi:10.1093/ijlct/ctab091
Klepeis, N. E., Nelson, W. C., Ott, W. R., Robinson, J. P., Tsang, A. M., Switzer, P., et al. (2001). The National Human Activity Pattern Survey (NHAPS): a resource for assessing exposure to environmental pollutants. J. Expo. Analysis Environ. Epidemiol. 11 (3), 231–252. doi:10.1038/sj.jea.7500165
Krstić, H., Otković, I. I., Kosiński, P., and Wojcik, R. (2016). Validation of neural network model for predicting airtightness of residential and non-residential units in Poland. Energy Build. 133, 423–432. doi:10.1016/j.enbuild.2016.10.011
Mahmoud, N. S. A., El Samanoudy, G., and Jung, C. (2023). Simulating the natural lighting for a physical and mental Well-being in residential building in Dubai, UAE. Ain Shams Eng. J. 14 (1), 101810. doi:10.1016/j.asej.2022.101810
Militello-Hourigan, R. E., and Miller, S. L. (2018). The impacts of cooking and an assessment of indoor air quality in Colorado passive and tightly constructed homes. Build. Environ. 144, 573–582. doi:10.1016/j.buildenv.2018.08.044
Molho, H. M., Zivan, O., Broday, D. M., and Raz, R. (2019). Application of a sensor network of low cost optical particle counters for assessing the impact of quarry emissions on its vicinity. Atmos. Environ. 211, 29–37. doi:10.1016/j.atmosenv.2019.04.054
Mushtaha, E., and Helmy, O. (2017). Impact of building forms on thermal performance and thermal comfort conditions in religious buildings in hot climates: a case study in Sharjah city. Int. J. Sustain. Energy 36 (10), 926–944. doi:10.1080/14786451.2015.1127234
Mushtaha, E., Salameh, T., Kharrufa, S., Mori, T., Aldawoud, A., Hamad, R., et al. (2021a). The impact of passive design strategies on cooling loads of buildings in temperate climate. Case Stud. Therm. Eng. 28, 101588. doi:10.1016/j.csite.2021.101588
Mushtaha, E., Shareef, S., Alsyouf, I., Mori, T., Kayed, A., Abdelrahim, M., et al. (2021b). A study of the impact of major Urban Heat Island factors in a hot climate courtyard: the case of the University of Sharjah, UAE. UAE. Sustain. Cities Soc. 69, 102844. doi:10.1016/j.scs.2021.102844
Nasser, Z., Salameh, P., Nasser, W., Abou Abbas, L., Elias, E., and Leveque, A. (2015). Outdoor particulate matter (PM) and associated cardiovascular diseases in the Middle East. Int. J. Occup. Med. Environ. Health 28 (4), 641–661. doi:10.13075/ijomeh.1896.00186
Otuyo, M. K., Mohd Nadzir, M. S., Latif, M. T., and Saw, L. H. (2023). In-train particulate matter (PM10 and PM2.5) concentrations: level, source, composition, mitigation measures and health risk effect–A systematic literature review. Indoor Built Environ. 32 (3), 460–493. doi:10.1177/1420326x221131947
Peng, Z., Deng, W., and Tenorio, R. (2017). Investigation of indoor air quality and the identification of influential factors at primary schools in the North of China. Sustainability 9 (7), 1180. doi:10.3390/su9071180
Pietrogrande, M. C., Casari, L., Demaria, G., and Russo, M. (2021). Indoor air quality in domestic environments during periods close to Italian COVID-19 lockdown. Int. J. Environ. Res. public health 18 (8), 4060. doi:10.3390/ijerph18084060
Propsearch.ae. Al, (2021). Habtoor city. Available online: https://www.vision2021.ae/en/national-agenda-2021/list/environment-circle.
Raysoni, A. U., Armijos, R. X., Weigel, M. M., Montoya, T., Eschanique, P., Racines, M., et al. (2016). Assessment of indoor and outdoor PM species at schools and residences in a high-altitude Ecuadorian urban center. Environ. Pollut. 214, 668–679. doi:10.1016/j.envpol.2016.04.085
Remion, G., Moujalled, B., and El Mankibi, M. (2019). Review of tracer gas-based methods for the characterization of natural ventilation performance: comparative analysis of their accuracy. Build. Environ. 160, 106180. doi:10.1016/j.buildenv.2019.106180
Santin, O. G., Grave, A., Jiang, S., Tweed, C., and Mohammadi, M. (2021). Monitoring the performance of a Passivhaus care home: lessons for user-centric design. J. Build. Eng. 43, 102565. doi:10.1016/j.jobe.2021.102565
Savdie, J., Canha, N., Buitrago, N., and Almeida, S. M. (2020). Passive exposure to pollutants from a new generation of cigarettes in real life scenarios. Int. J. Environ. Res. Public Health 17 (10), 3455. doi:10.3390/ijerph17103455
Ścibor, M., Bokwa, A., and Balcerzak, B. (2020). Impact of wind speed and apartment ventilation on indoor concentrations of PM10 and PM2. 5 in Kraków, Poland. Air Qual. Atmos. Health 13 (5), 553–562. doi:10.1007/s11869-020-00816-8
Silva, M. F., Maas, S., de Souza, H. A., and Gomes, A. P. (2017). Post-occupancy evaluation of residential buildings in Luxembourg with centralized and decentralized ventilation systems, focusing on indoor air quality (IAQ). Assessment by questionnaires and physical measurements. Energy Build. 148, 119–127. doi:10.1016/j.enbuild.2017.04.049
Singer, B. C., Chan, W. R., Kim, Y. S., Offermann, F. J., and Walker, I. S. (2020). Indoor air quality in California homes with code-required mechanical ventilation. Indoor air 30 (5), 885–899. doi:10.1111/ina.12676
Singer, B. C., and Delp, W. W. (2018). Response of consumer and research grade indoor air quality monitors to residential sources of fine particles. Indoor Air 28 (4), 624–639. doi:10.1111/ina.12463
Stasiulaitiene, I., Krugly, E., Prasauskas, T., Ciuzas, D., Kliucininkas, L., Kauneliene, V., et al. (2019a). Infiltration of outdoor combustion-generated pollutants to indoors due to various ventilation regimes: a case of a single-family energy efficient building. Build. Environ. 157, 235–241. doi:10.1016/j.buildenv.2019.04.053
Stasiulaitiene, I., Krugly, E., Prasauskas, T., Ciuzas, D., Kliucininkas, L., Kauneliene, V., et al. (2019b). Infiltration of outdoor combustion-generated pollutants to indoors due to various ventilation regimes: a case of a single-family energy efficient building. Build. Environ. 157, 235–241. doi:10.1016/j.buildenv.2019.04.053
Sultan, Z. M., Pantelic, J., and Tham, K. W. (2020). Infiltration of fine particles in urban daycares. Indoor air 30 (5), 955–965. doi:10.1111/ina.12679
Taleb, H. M., and Kayed, M. (2021). Applying porous trees as a windbreak to lower desert dust concentration: case study of an urban community in Dubai. Case study urban community Dubai 57, 126915. doi:10.1016/j.ufug.2020.126915
Tham, K. W., Parshetti, G. K., Anand, P., Cheong, D. K. W., and Sekhar, C. (2021). Performance characteristics of a fan filter unit (FFU) in mitigating particulate matter levels in a naturally ventilated classroom during haze conditions. Indoor air 31 (3), 795–806. doi:10.1111/ina.12771
Tran, L. K., Morawska, L., Quang, T. N., Jayaratne, R. E., Hue, N. T., Dat, M. V., et al. (2021). The impact of incense burning on indoor PM2. 5 concentrations in residential houses in Hanoi, Vietnam. Build. Environ. 205, 108228. doi:10.1016/j.buildenv.2021.108228
Wang, F., Meng, D., Li, X., and Tan, J. (2016a). Indoor-outdoor relationships of PM2. 5 in four residential dwellings in winter in the Yangtze River Delta, China. Environ. Pollut. 215, 280–289. doi:10.1016/j.envpol.2016.05.023
Wang, F., Meng, D., Li, X., and Tan, J. (2016b). Indoor-outdoor relationships of PM2. 5 in four residential dwellings in winter in the Yangtze River Delta, China. Environ. Pollut. 215, 280–289. doi:10.1016/j.envpol.2016.05.023
Wang, F., Meng, D., Li, X., and Tan, J. (2016c). Indoor-outdoor relationships of PM2. 5 in four residential dwellings in winter in the Yangtze River Delta, China. Environ. Pollut. 215, 280–289. doi:10.1016/j.envpol.2016.05.023
Zhao, L., Liu, J., and Ren, J. (2018). Impact of various ventilation modes on IAQ and energy consumption in Chinese dwellings: first long-term monitoring study in Tianjin, China. Build. Environ. 143, 99–106. doi:10.1016/j.buildenv.2018.06.057
Zhong, L., Yuan, J., and Fleck, B. (2019). Indoor environmental quality evaluation of lecture classrooms in an institutional building in a cold climate. Sustainability 11 (23), 6591. doi:10.3390/su11236591
Keywords: particulate matter (PM), indoor air quality (IAQ), infiltration rate, indoor and outdoor concentration, residential buildings
Citation: Jung C and Abdelaziz Mahmoud NS (2023) Navigating dust storms and urban living: an analysis of particulate matter infiltration in Dubai’s residences. Front. Built Environ. 9:1297520. doi: 10.3389/fbuil.2023.1297520
Received: 20 September 2023; Accepted: 13 November 2023;
Published: 22 November 2023.
Edited by:
Klara Slezakova, University of Porto, PortugalReviewed by:
Roberto Alonso González-Lezcano, CEU San Pablo University, SpainHasim Altan, Prince Mohammad bin Fahd University, Saudi Arabia
Copyright © 2023 Jung and Abdelaziz Mahmoud. This is an open-access article distributed under the terms of the Creative Commons Attribution License (CC BY). The use, distribution or reproduction in other forums is permitted, provided the original author(s) and the copyright owner(s) are credited and that the original publication in this journal is cited, in accordance with accepted academic practice. No use, distribution or reproduction is permitted which does not comply with these terms.
*Correspondence: Naglaa Sami Abdelaziz Mahmoud, bi5hYmRlbGF6aXpAYWptYW4uYWMuYWU=