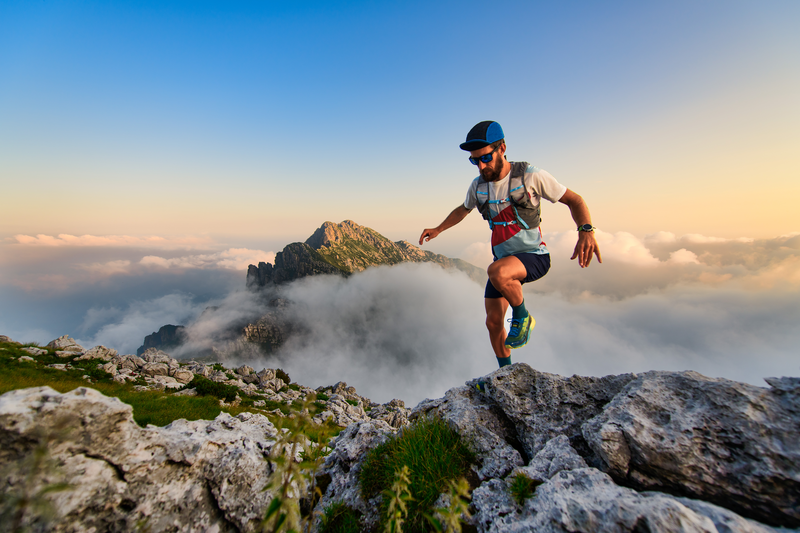
94% of researchers rate our articles as excellent or good
Learn more about the work of our research integrity team to safeguard the quality of each article we publish.
Find out more
MINI REVIEW article
Front. Bioeng. Biotechnol. , 29 October 2024
Sec. Nanobiotechnology
Volume 12 - 2024 | https://doi.org/10.3389/fbioe.2024.1482637
This article is part of the Research Topic Exploration of innovative strategies focusing on advanced nano-/biomaterials for optimizing oncological treatment and tissue restoration, Volume II View all 4 articles
Self-assembled lipid-based nanoparticles have been shown to have improved therapeutic efficacy and lower toxic side effects. Breast cancer is a common type of malignant tumor in women. Conventional drugs such as doxorubicin (DOX) have shown low therapeutic efficacy and high drug toxicity in antitumor therapy. This paper surveys research on self-assembled lipid-based nanoparticles by categorizing them under three groups: self-assembled liposomal nanostructures, self-assembled niosomes, and self-assembled lipid–polymer hybrid nanoparticles. Subsequently, the structural features and operating mechanisms of each group are summarized individually along with examples of representative drugs from each group.
Breast cancer is a common type of malignant tumor among women (Ashkbar et al., 2020). Between 2008 and 2020, the number of female breast cancer cases increased from 1.38 million to 2.25 million (Tseu and Kamaruzaman, 2023; Riis, 2020). The main factors that induce breast cancer include heredity, menstruation, fertility, and living habits (Kshivets, 2023; Jemal et al., 2011; Britt et al., 2020)}. The 5-year survival rate for breast cancer is over 90% (Riis, 2020); however, breast cancer remains the second leading cause of tumor-related deaths among women worldwide (Yang et al., 2021). Breast cancer treatments have now entered the era of precision medicine (Sarhangi et al., 2022). Traditional chemotherapeutic drugs have low bioavailability and poor efficacy owing to their poor selectivity toward cancer cells, poor solubility, low stability, and high side effects (Yang et al., 2021; Qiao et al., 2020). In addition, the ability of chemotherapeutic drugs to penetrate cancer cells is limited, often resulting in drug resistance (Tredan et al., 2007; Grantab et al., 2006; Ning, 2022). Thus, improving the drug concentration at the cancer site and reducing the side effects are important directions of current research (Lim and Ma, 2019).
Self-assembled nanoparticles loaded with drugs constitute a class of medications characterized by their nanoscale structures. These nanoparticles are formed by self-assembly and incorporate small molecules of chemotherapeutic or macromolecular drugs, such as proteins and nucleic acids. During this process, they may experience interactions involving hydrogen bonds, electrostatic forces, van der Waals forces, and other related forces (Song et al., 2012). Currently, most nanoparticles used in clinical practice have diameters in the range of 1–200 nm. Upon entering the body, the nanoparticles maintain balanced surface charges to prevent infiltration of the encapsulated drugs into the surrounding tissues (Song et al., 2012; Hoshyar et al., 2016; Cheng et al., 2020; von Roemeling et al., 2017; Schwartz, 2017; Xie et al., 2017). Various self-assembled nanoparticles have been synthesized from carbohydrates, nucleic acids, peptides, and other biomaterials for biomedical and pharmaceutical applications (Dahiya and Dahiya, 2021; Fan et al., 2016; Kaur et al., 2023; Jain et al., 2015). The comprehensive treatment of cancer using self-assembled nanoparticles not only enhances the survival rates but also mitigates the risks associated with low local drug utilization and excessive systemic adverse drug reactions (Pérez-Herrero and Fernández-Medarde, 2015; Sabit et al., 2022; Kitsios et al., 2023).
Existing research classifies self-assembled nanocarriers into six categories for various medical applications as lipid-based nanoparticles (Lasic, 2019; Puri et al., 2009; Sabahi, 2023), polymeric nanostructures, carbon-based nanoparticles, ceramic nanostructures, biological nanoparticles, and micelles (Lasic, 1996; Torchilin, 2006; McClements, 2012; Allen and Cullis, 2013; Cabral and Kataoka, 2014; Menjoge, 2010; Varma et al., 2020; Da, 2021; Xie and Pedrielli, 2023). Self-assembled lipid-based nanoparticles offer numerous advantages, including versatility, biocompatibility, controlled drug-release capabilities, enhanced stability, and targeted delivery potential. Some representative drugs in this category include DOX-Lip, pegylated liposomal doxorubicin (PLD), nitric oxide (NO)-donor-loaded bioinspired lipoprotein system (NO-BLP), albumin-bound paclitaxel lipid nanoparticles (ABPLN), and liposomal daunorubicin.
This review comprehensively introduces three distinct categories of self-assembled lipid-based nanoparticles, namely self-assembled liposomal nanostructures, self-assembled niosomes, and self-assembled lipid–polymer hybrid nanoparticles (LPHNPs). We describe the structures and compositions of these nanoparticles; furthermore, we provide a summary of the related drugs and current research status by analyzing their advantages and disadvantages, thereby enabling discussion of the extent to which self-assembled lipid-based nanoparticles can optimize chemotherapy in breast cancer (as illustrated in Table 1).
The Guidelines for Clinical Diagnosis and Treatment of Advanced Breast Cancer in China (2023 Edition) clearly state that anthracyclines, including epirubicin and doxorubicin (DOX) (Devi et al., 2023; Hossain et al., 2023), are the preferred first-line treatment drugs for breast cancer. Nevertheless, the main challenge is reducing the toxicity of anthracyclines, which significantly impacts the clinical management of breast cancer. As a representative drug delivered by self-assembled lipid-based nanoparticles, such as PLD/ABPLN, it enhances drug utilization while mitigating the toxic side effects.
Through in vitro experiments, animal studies, and clinical trials, it has been found that self-assembled lipid-based nanoparticles can provide increased drug concentration while minimizing the toxicity and side effects. In this context, the three self-assembled lipid-based nanoparticles used in chemotherapy against breast cancer are self-assembled liposomal nanostructures, self-assembled niosomes, and self-assembled LPHNPs.
Liposomes are nanomaterials used for drug delivery (Bhattacharya et al., 2022; Zylberberg et al., 2017). Nanoparticles prepared as liposomes were among the first to be applied in clinical drug delivery systems, offering advantages such as biocompatibility and degradability (Torchilin, 2005; Allen and Cullis, 2013; Al-Jamal and Kostarelos, 2011). Liposomes also have disadvantages, such as being thin, being fragile, and having poor storage stability. Bangham’s phospholipid experiment in the 1960s revealed that hydrophilic groups of self-assembled liposomes exposed to water with hydrophobic groups hidden inside facilitate liposomal delivery systems through molecular interactions. The amphiphilic components of the liposomes self-assemble in aqueous media spontaneously to form stable structures. Self-assembled liposomal nanostructures can adjust their performances through alterations to the composition and surface (Das et al., 2013; Logigan et al., 2024; Ewert et al., 2023; Stephanopoulos et al., 2013; Genio et al., 2022).
The cytotoxic drug DOX can stop the proliferation of cancer cells by inhibiting the syntheses of topoisomerase II and nucleic acids. DOX combined with liposomes can reduce the side effects of DOX itself and overcome the limitations of clinical medications (Makowski et al., 2019). Rahman et al. (1989) at Georgetown University found that liposomes with encapsulated DOX could inhibit DNA synthesis in human breast cancer (MDA-435) cells. Upon addition of a monoclonal antibody against human laminin receptor to the surface of the liposomal DOX, the binding to the target cells was found to have increased tenfold. Since the mid-1990s, long-acting DOX (also known as Doxil in the United States and Caelyx in Europe) encapsulated in polyethylene glycol (PEG) liposomes has been marketed and applied to metastatic breast cancer (Waterhouse et al., 2001; Duggan and Keating, 2011).
Unlike DOX, which inhibits lipid peroxidation and induces the formation of free radicals, mitoxantrone is superior in terms of its acute toxicity and cross-resistance, especially in the treatment of metastatic breast cancer (Durr et al., 1983; Pestalozzi et al., 1992). Poujol et al. (1999) found that in patients with multidrug-resistant breast cancer, certain compounds could alter the P-glycoprotein function through plasma membrane stabilization and modulate multidrug resistance in human cancers based on their lipid compositions. Mitoxantrone-loaded liposomes have improved drug safety but have not significantly improved the antitumor abilities of the drug. Moreover, they do not show evident advantages over self-assembled liposomal nanostructures loaded with DOX. Therefore, Chowdhury et al. (2020) continued their research on DOX in self-assembled liposomal nanostructures; they used phosphatidylcholines, cholesterol, and 1,2-distearoyl-sn-glycero-3-phosphoethanolamine with conjugated methoxy PEG (DSPE-mPEG) to develop a lipid–polymer coating for DOX. Their experiments on HER-2-positive breast cancer cells showed increased uptake of DOX with a lower IC50 value. It was observed that the presence of liposomes in the HER-2-positive MCF-7 and SKBR-3 breast cancer cells increased the uptake and targeted delivery of DOX. Yang et al. (2022) modified DSPE-PEG-biotin, conjugated streptavidin (STA), biotin, and PEG on double-layer phospholipids to encapsulate DOX and form DOX-Lip (Figure 1A). Furthermore, DOX-Lip was connected to macrophages to form the macrophage liposome (MA lip). A study on a triple-negative breast cancer cell line (4T1 cells) showed that MA lip was conducive to the migration of DOX into the deep cells of the tumor and its release into the deep regions of the tumor.
Figure 1. Structures of self-assembled lipid-based nanoparticles. (A) Self-assembled liposomal nanostructures: modified DSPE-PEG-biotin (streptavidin, biotin, PEG, and macrophage synthesis) on a double-layer structure to encapsulate DOX to form DOX-Lip (Yang et al., 2022) ⓒ 2022, American Chemical Society. (B) Self-assembled niosomes: Tween-80-conjugated niosomes with cholesterol encapsulate DOX and D-limonene in lipid-based nanoparticles. (C) Self-assembled lipid–polymer hybrid nanoparticles: NO-donor-loaded bioinspired lipoprotein system (NO-BLP) was constructed using 1,2-dimyristoyl-sn-glycero-3-phosphatidylcholine (DMPC), DSPE-PEG 2000, NO-C16, and ApoA1 mimetic peptide (Wu et al., 2023) ⓒ 2023, American Chemical Society.
It was shown that DOX induces the immune cells to activate antitumor immunity (Galluzzi et al., 2020), and it was also confirmed that macrophages actively migrate into hypoxic areas within tumors (Chaturvedi et al., 2014; Germain and Kastenmüller, 2010). Existing research shows that self-assembled liposomal nanostructures are formed using single-layer or double-layer lipid outer membranes composed of substances such as phospholipids, cholesterols, DSPE-mPEG, and lipids encapsulating chemotherapeutic drugs like mitoxantrone or DOX with or without surface modification by DSPE-PEG-biotin (Figure 1A) (Chowdhury et al., 2020; Yang et al., 2022; Pestalozzi et al. (1992) and Pestalozzi et al. (1995); Wang et al., 2020). The representative drugs PLD and liposome-complexed mitoxantrone were also shown to have improved safety.
Niosomes are nanovesicles of non-ionic surfactants that promise efficient drug delivery by encapsulating hydrophilic and hydrophobic drugs. Riccardi et al. (2018) used the AS1411 aptamer to target cancer cell nucleolin, whereby the drugs were delivered directly to the breast tumors with reduced toxicity and enhanced treatment efficacy. Liu et al. (2017) studied liposomal daunorubicin as a representative drug of self-assembled niosomes to enhance its retention time in tumor tissues. Wheat germ agglutinin (WGA)-modified daunorubicin antiresistant liposomes were produced by the thin-film hydration method using coated WGA (Li et al., 2014, Li et al., 20142015) with a lipid composition of error-producing condition (EPC)/cholesterol/DSPE-PEG 2000. In tumor-bearing mice with MCF-7/ADR cells, the WGA-modified daunorubicin antiresistant liposomes were found to retain daunorubicin in the tumor tissues for 24 h and significantly reduce the size of the tumor cells; free daunorubicin stayed in the tumor tissues for only a short time. However, liposomal daunorubicin also has some limitations, such as its high molecular weight. Although liposomal daunorubicin has low toxicity even with its lengthy retention time in living tissues, further studies are needed to assess whether there will be toxicity accumulation. Kulkarni and Rawtani (2019) from the American Pharmaceutical Association used the Box Behnken design to study MCF-7 breast cancer cells in vitro. They found that niosomes containing the lipophilic drug tamoxifen and hydrophilic drug DOX had 70% encapsulation efficiency and could be stable for up to 6 months under refrigeration at 4°C; this composition shows increased encapsulation efficiency and stability simultaneously. Although blank niosomes are non-toxic to normal cells, the niosomes used in this study could not reduce the cumulative toxicity of the drugs in combination therapy for the time being. Assali et al. (2022) used the Peppas–Sahlin model and found that niosomes containing Tween 80 with cholesterol and encapsulating DOX as well as D-limonene exhibited higher stability; in vitro experiments also showed that the release of DOX was more stable (Figure 1B). However, the study did not identify the protective effects of D-limonene combined with DOX on the heart, indicating that further research is needed.
Unlike self-assembled liposomal nanostructures using DSPE-PEG 2000 as the medium, self-assembled niosomes contain Tween 80 as the medium. As shown in Figure 1B (Assali et al., 2022; Liu et al., 2017), liposomal daunorubicin as a representative drug controls the progress of breast cancer by increasing the retention time of daunorubicin in the tumor tissues.
Self-assembled LPHNPs have advantages as nanocarriers for the delivery of anticancer agents (Pang et al., 2020; Martinelli et al., 2019; Govindan et al., 2023); these benefits include compositions of diverse synthetic drugs, simple and repeatable synthesis process, and good stability (Du et al., 2019). These nanoparticles also enable individualized treatments for tumor patients through different chemical modifications (Kumar et al., 2020). However, owing to the use of organic solvents during synthesis, the LPHNPs exhibit shortcomings, such as generating toxic byproducts after in vivo degradation and limited capacity to capture drugs (Ulbrich et al., 2016). Rao and Prestidge (2016) encapsulated polymer particles in a lipid shell to synthesize LPHNPs and explored their feasibility for oral administration. LPHNPs mainly comprise an external lipid shell encapsulating biodegradable hydrophobic polymers, with the inner core serving as the primary carrier of lipid-soluble drugs (Shi et al., 2015; Li, 2024; Dehaini et al., 2016; Wu et al., 2020; Sengel-Turk et al., 2021). LPHNPs have strong lipid-based drug-loading capacities and can affect the drug release rates (Bakar-Ates et al., 2020; Klibanov et al., 1990; Hamdi et al., 2020). Moreover, LPHNPs possess biomimetic properties, biocompatibility, ideal drug-release characteristics for polymer nanocarriers, and the ability for various surface chemical modifications (Al-Jipouri et al., 2023; Shi et al., 2014; Tahir et al., 2020). Zhang et al. (2021) prepared nanoparticles using the Michael-type step polymerization (Lynn and Langer, 2000), where a mixed lipid shell comprising DSPE-PEG 2000, FA-DSPE-PEG 2000, and lecithin encapsulates a degradable poly β-amino ester (PBAE) carrying docetaxel (DTX) to form PBAE/DTX nanoparticles. FA-targeted phospholipid monolayers form the shell of the FA/PBAE/DTX nanoparticles. The study of 4T1 breast cancer cells showed that compared to free DTX or PBAE/DTX nanoparticles, FA/PBAE/DTX nanoparticles had significantly enhanced intracellular uptake efficiency and cytotoxicity. The modification with FA enhances the tumor-targeting ability of the polymeric lipid nanoparticles. Finally, FA/PBAE/DTX nanoparticles for drug delivery enhanced the antitumor effects and had less systemic toxicity in 4T1 breast cancer cells. A sunitinib-loaded self-nanoemulsifying formulation has been reported to have better antitumor activity against MCF-7 breast cancer cells (Nazari-Vanani et al., 2017; Adams and Leggas, 2007; Alshahrani et al., 2018). Further studies are being conducted on sunitinib malate (SM) in 28 ongoing clinical trials (Chen et al., 2017). Another study on MCF-7 cells involved the use of lecithin as a stabilizer along with emulsification-solvent evaporation technology (Ahmed et al., 2022; Anwer et al., 2021). SM, Lipoid 90H, and chitosan have been combined to form LPHNPs; by adjusting the concentration of chitosan, the composition of the nanoparticles can be altered. Accordingly, four formulations (SLPN1–SLPN4) were developed and tested separately on MCF-7 cells. The results showed that SLPN4 significantly enhances the release and accessibility of SM in MCF-7 cells and that SM-loaded LPHNPs may be a promising option for cancer treatment.
Existing clinical studies have shown that albumin-bound paclitaxel increases the safety of paclitaxel over traditional paclitaxel for HER-2-positive, weak-positive, or HER-2-negative patients (Shi et al., 2023; Futamura et al., 2023). However, no significant changes have been found in the survival rates of breast cancer patients. Ruttala and Ko (2015b) improved the antitumor effects of albumin-bound paclitaxel nanoparticles (APN); the lipid–liposome bilayer was coated with albumin paclitaxel to prepare liposome-encapsulated APN (i.e., L-APN or ABPLN). In vitro tests then showed that L-APN could significantly improve the stability of paclitaxel and enhance the cytotoxic activity of APN in MCF-7 cells; it was also found via in vitro studies that when curcumin and APN are codelivered to MCF-7 cells, the presence of the liposomes enhances the synergistic antitumor ability of curcumin and albumin-bound paclitaxel (Ruttala and Ko, 2015a).
Compared to self-assembled LPHNPs coated with APN, liposomal nanoparticles coated with curcumin and albumin-bound paclitaxel exhibit greater cytotoxicity and superior anticancer effects on breast cancer cells. Based on these findings, scholars from Fudan University in Shanghai constructed a NO-BLP (Wu et al., 2023) composed of 1,2-dimyristoyl-sn-glycero-3-phosphatidylcholine (DMPC), DSPE-PEG 2000, glutathione (GSH)-sensitive NO donor agent (NO-C16), and an apolipoprotein A1 (ApoA1) mimetic peptide (Figure 1C) (Stylianopoulos et al., 2018; Martin et al., 2019). In mice carrying 4T1 tumors of breast cancer, NO-BLP can effectively accumulate at the tumor site and release active NO molecules to normalize the disordered tumor vessels, promoting intratumoral administration and chemotherapy using APN. Self-assembled lipid–polymer hybrid nanoparticles can modify the tumor microenvironment and enhance the efficacy of chemotherapeutic drugs by releasing NO into the tumor cells. Therefore, NO-BLP improves the efficacy of APNs. However, as this study was conducted in animals, only the antitumor effects were evaluated while the drug safety was not assessed; thus, drug safety evaluations are necessary for further clinical trials.
Most recent studies show that self-assembled lipid-based nanoparticles can prolong the residence times of chemotherapeutic drugs in tumor tissues (Makowski et al., 2019; Chowdhury et al., 2020; Yang et al., 2022). Yang et al. (2022) showed that DOX-Lip links with macrophages through DSPE-PEG-STA interactions (Figure 2A) and deeply penetrates the tumor cells to release DOX, thereby activating robust immune responses through the CD4+/CD8+/NK cells. MA-DOX-Lip effectively inhibited the tumor growth model of 4T1 triple-negative breast cancer in mice and improved the killing rate of the tumor cells (Figure 2A). Recent studies have also proven that the presence of liposomes improves the utilization rates of chemotherapeutic drugs and their safety. However, some studies have shown that increasing the utilization of chemotherapeutic drugs has no apparent advantages for prolonging the overall survival (OS) of cancer patients.
Figure 2. Prolonging the retention times of chemotherapeutic drugs in tumor tissues and activating strong immune responses. (A) DOX-Lip links with macrophages through DSPE-PEG-STA interactions; mechanism of in vivo action of self-assembled lipid-based nanoparticles (Yang et al., 2022) ⓒ 2022, American Chemical Society. (B) Schematic illustration of self-assembled lipid-based nanoparticles acting on breast cancer tissues to restore normal blood supply (Wu et al., 2023) ⓒ 2023, American Chemical Society. Here, DSPE-PEG denotes 1,2-distearoyl-sn-glycero-3-phosphoethanolamine-poly (ethylene glycol); DMPC denotes 1,2-dimyristoyl-sn-glycero-3-phosphatidylcholine; PAN denotes paclitaxel nanoparticles; NO-BLP denotes NO-donor-loaded bioinspired lipoprotein system.
Although traditional research efforts have prolonged the retention times of chemotherapeutic drugs in tumor tissues, they have not significantly prolonged the OS of breast cancer patients because of the influence of the tumor microenvironment on antitumor efficacy (Tredan et al., 2007). Wu et al. (2023) prepared NO-BLP loaded with GSH-activated NO donors to normalize tumors, improve the delivery efficiency of APN in the tumors, change the tumor microenvironment by restoring regular blood supply to the tumor tissues, and sequentially treat tumors (Figure 2B). The tumor inhibition rate for the NO-BLP + PAN treatment group was 81.03%, and the tumor sizes were only 29.89% for the NO-BLP group and 39.85% for the PAN group (Wu et al., 2023).
Changes in the tumor microenvironment are likely to promote the effects of chemotherapeutic drugs and reduce drug resistance (Tredan et al., 2007). Unlike traditional research on activating the immune responses, research on self-assembled lipid-based nanoparticles offers an alternative approach by which the nanoparticles change the tumor microenvironment to improve the drug utilization rate. Research on restoring regular blood supply to the tumor tissue with nanomaterials can improve the killing rates of tumor cells using chemotherapeutic drugs through the changes to the tumor microenvironment. However, such research efforts are still within the scope of animal research. Although animal research proves the safety and reliability of a drug—supported by the weights of the tumor-bearing mice and histological examination results of their main organs—there is a lack of relevant clinical trials, resulting in inadequate evaluations of the safety and antitumor effects. The extent to which NO-BLP improves the effectiveness of other liposomal chemotherapeutic drugs remains an open research question.
Existing clinical antitumor treatments widely use liposomal adriamycin, and clinical trials have improved its safety, reduced the toxic and side effects, and ensured a smoother process for chemotherapy, thereby prolonging the progression-free survival (PFS) (China, 2023). Unlike the results of in vitro studies and animal experiments, the aforementioned drugs have not improved the OS times of breast cancer patients over 5 and 10 years, which may be related to insufficient sample sizes and short research times (Gucalp et al., 2018; Fujiwara et al., 2019; Pestalozzi et al., 1992, 1995; Fabi et al., 2020). Pestalozzi et al. (1992); Pestalozzi et al. (1995) found that in the phase II study of liposome-complexed mitoxantrone, although there was no significant improvement in the PFS over treatment with free mitoxantrone, there were reductions in the cardiac and hematological toxicities. To verify whether breast cancer patients could benefit from self-assembled liposomal nanostructures, O’brien et al. conducted a phase III study comparing the use of DOX and PLD as first-line treatments in 509 patients with metastatic breast cancer (Wang et al., 2020). PLD was formulated as photolipid bilayers coated with methoxy-PEG-encapsulated DOX. The OS rates of patients showed no differences for the two treatments (PLD over 21 months vs. DOX over 22 months); however, the pathological complete response (PCR) of the PLD group was significantly prolonged and cardiac toxicity was significantly reduced (hazard ratio [Hr] 1/4 = 3.16; 95% confidence interval [cI] 1/4 = 1.58–6.31;
Clinical experiments on PLD and liposomal mitoxantrone, which are some representative drugs used in self-assembled liposomal nanostructures, show that liposomal nanostructures can indeed increase drug safety and relatively improve drug utilization compared to free DOX/mitoxantrone. However, there are no apparent benefits in terms of drug resistance, especially in prolonging the survival of breast cancer patients.
Although self-assembled niosomes have produced good results in vitro and in animal experiments and liposomal daunorubicin used as their representative drug significantly reduced the growth rates of breast cancer cells in MCF-7/ADR-tumor-bearing mice, there are no clinical trials that clearly show that self-assembled niosomes can simultaneously improve the efficacy and safety of antitumor drugs. Liposomal daunorubicin as a representative drug used in self-assembled niosomes has improved the antitumor efficacy in chemotherapy because it prolongs the residence time of daunorubicin in the tumor tissues. However, the safety of relevant drugs and nanomaterials are still subject to verification. Compared with self-assembled liposomal nanostructures that improve the safety of antitumor treatments, self-assembled niosomes have more advantages in reducing the tumor growth rates; however, unlike the phase I/II study of self-assembled liposomal nanostructures, there are no available clinical studies of self-assembled niosomes, suggesting that breast cancer patients can significantly benefit from more studies on self-assembled niosomes. Furthermore, clinical experiments are needed to clarify whether self-assembled niosomes can achieve better antitumor effects in humans.
As a representative drug used in self-assembled LPHNPs, APN (or L-APN), ABPLN can improve the safety of chemotherapy and is more convenient for clinical application (Ruttala and Ko, 2015b; Meng et al., 2015). However, there is limited research on these drugs against breast cancer. In vitro research shows that NO-BLP or self-assembled LPHNPs have better anticancer effects and can further improve the antitumor effects in chemotherapy by changing the tumor microenvironment. A phase I study of APN also showed that self-assembled lipid-based nanoparticles could reduce the toxicity of chemotherapeutic drugs (Fabi et al., 2020). Compared with traditional free paclitaxel/DOX, liposome-encapsulated DOX combined with APN has significantly reduced toxicity. Nevertheless, as a phase I study involving only 12 patients, this approach needs improvement given the inadequate number of participants, samples, and data.
Nanomedicine as a field is still limited for the comprehensive treatment of breast cancer (Kim et al., 2007; Fabian et al., 2020; Gucalp et al., 2018; Malloizel-Delaunay et al., 2024; Wu et al., 2017), and clinical trials related to nanomedicine have also proved the importance of nanobioengineering for disease control in clinical tumor patients (Fujiwara et al., 2019; Mohammadpour and Majidzadeh, 2020; Hekmat et al., 2022). The presently available self-assembled lipid-based nanoparticles increase the drug utilization concentrations in tumor tissues as well as reduce the toxic and side effects of drugs, thereby improving the safety of tumor treatment.
Nowadays, chemotherapy as a treatment for breast cancer is limited by the toxic and side effects of the drugs used, which affects the treatment efficacy. Although the phase I studies conducted by Pestalozzi et al. (1992) and Pestalozzi et al. (1995), O’brien (Wang et al., 2020), and Fabi et al. (2020) lack adequate sample sizes, there is evidence that self-assembled liposomal nanostructures encapsulated with DOX or mitoxantrone can improve the safety of chemotherapy drugs as well as significantly reduced their toxic and side effects, especially in terms of cardiotoxicity. However, it is debatable whether improving the chemotherapy cycle of DOX can further prolong the OS times of breast cancer patients and must be verified with additional clinical studies. Moreover, in vivo and in vitro experiments have shown that the representative drugs used in self-assembled lipid-based nanoparticles, such as DOX-Lip, liposome-complexed mitoxantrone, ABPLN, and liposomal daunorubicin, significantly improve the safety of chemotherapy in the free state and reduce cardiotoxicity. At the same time, extant studies show that DOX-Lip, liposome-complexed mitoxantrone, and ABPLN mainly enhance safety by reducing the toxic and side effects of chemotherapeutic drugs, with no significant changes in the OS times of the patients. However, NO-BLP as a typical drug used in self-assembled LPHNPs enables easier entry into tumor cells by improving the tumor microenvironment.
In conclusion, although self-assembled LPHNPs, such as ABPLN, can increase the efficacies of chemotherapeutic medications by improving the tumor microenvironment, it is unclear whether self-assembled lipid nanostructures and self-assembled niosomes carrying different drugs can produce similar changes. Another open question is whether self-assembled lipid-based nanoparticles can change the tumor microenvironment by carrying different substances. In the future, such treatments must aim to target different types of tumors through various substances.
Lipid-based nanoparticles thicken the liposomes and increase their storage stability to a certain extent. Nevertheless, their ability to capture drugs remains to be improved. The utilization of organic solvents during preparation of specific lipid-based nanoparticles requires further research for reducing the toxicity of these nanoparticles. The present review clearly shows that data on animal and human experiments are lacking with regard to the use of nanomaterials in breast cancer chemotherapy.
This mini review summarizes recent research progress on lipid nanoparticles and provides detailed descriptions of the structures, mechanisms, and representative drugs associated with three types of self-assembled nanoparticles. Furthermore, we discuss the applications of lipid nanoparticles in the treatment of breast cancer.
SL: conceptualization, data curation, formal analysis, funding acquisition, investigation, methodology, project administration, resources, software, supervision, validation, visualization, writing–original draft, and writing–review and editing.
The authors declare that financial support was received for the research, authorship, and/or publication of this article. This study was supported by grants from the National Natural Science Foundation of China (NSFC No. 82072902).
The authors declare that the research was conducted in the absence of any commercial or financial relationships that could be construed as a potential conflict of interest.
All claims expressed in this article are solely those of the authors and do not necessarily represent those of their affiliated organizations, or those of the publisher, the editors, and the reviewers. Any product that may be evaluated in this article, or claim that may be made by its manufacturer, is not guaranteed or endorsed by the publisher.
Adams, V. R., and Leggas, M. (2007). Sunitinib malate for the treatment of metastatic renal cell carcinoma and gastrointestinal stromal tumors. Clin. Ther. 29, 1338–1353. doi:10.1016/j.clinthera.2007.07.022
Ahmed, M. M., Anwer, M. K., Fatima, F., Aldawsari, M. F., Alalaiwe, A., Alali, A. S., et al. (2022). Boosting the anticancer activity of sunitinib malate in breast cancer through lipid polymer hybrid nanoparticles approach. Polymers 14, 2459. doi:10.3390/polym14122459
Al-Jamal, W. T., and Kostarelos, K. (2011). Liposomes: from a clinically established drug delivery system to a nanoparticle platform for theranostic nanomedicine. Accounts Chem. Res. 44, 1094–1104. doi:10.1021/ar200105p
Al-Jipouri, A., Almurisi, S. H., Al-Japairai, K., Bakar, L. M., and Doolaanea, A. A. (2023). Liposomes or extracellular vesicles: a comprehensive comparison of both lipid bilayer vesicles for pulmonary drug delivery. Polymers 15, 318. doi:10.3390/polym15020318
Allen, T. M., and Cullis, P. R. (2013). Liposomal drug delivery systems: from concept to clinical applications. Adv. Drug Deliv. Rev. 65, 36–48. doi:10.1016/j.addr.2012.09.037
Alshahrani, S. M., Alshetaili, A. S., Alalaiwe, A., Alsulays, B. B., Anwer, M. K., Al-Shdefat, R., et al. (2018). Anticancer efficacy of self-nanoemulsifying drug delivery system of sunitinib malate. AAPS PharmSciTech 19, 123–133. doi:10.1208/s12249-017-0826-x
Anwer, M. K., Ali, E. A., Iqbal, M., Ahmed, M. M., Aldawsari, M. F., Saqr, A. A., et al. (2021). Development of sustained release baricitinib loaded lipid-polymer hybrid nanoparticles with improved oral bioavailability. Molecules 27, 168. doi:10.3390/molecules27010168
Ashkbar, A., Rezaei, F., Attari, F., and Ashkevarian, S. (2020). Treatment of breast cancer in vivo by dual photodynamic and photothermal approaches with the aid of curcumin photosensitizer and magnetic nanoparticles. Sci. Rep. 10, 21206. doi:10.1038/s41598-020-78241-1
Assali, M., Jaradat, N., and Maqboul, L. (2022). The Formation of self-assembled nanoparticles loaded with doxorubicin and d-limonene for cancer therapy. ACS Omega 7, 42096–42104. doi:10.1021/acsomega.2c04238
Bakar-Ates, F., Ozkan, E., and Sengel-Turk, C. T. (2020). Encapsulation of cucurbitacin b into lipid polymer hybrid nanocarriers induced apoptosis of mdamb231 cells through parp cleavage. Int. J. Pharm. 586, 119565. doi:10.1016/j.ijpharm.2020.119565
Bhattacharya, S., Saindane, D., and Prajapati, B. G. (2022). Liposomal drug delivery and its potential impact on cancer research. Anti-Cancer Agents Med. Chem. 22, 2671–2683. doi:10.2174/1871520622666220418141640
Britt, K. L., Cuzick, J., and Phillips, K.-A. (2020). Key steps for effective breast cancer prevention. Nat. Rev. Cancer 20, 417–436. doi:10.1038/s41568-020-0266-x
Cabral, H., and Kataoka, K. (2014). Progress of drug-loaded polymeric micelles into clinical studies. J. Control. Release 190, 465–476. doi:10.1016/j.jconrel.2014.06.042
Chaturvedi, P., Gilkes, D. M., Takano, N., and Semenza, G. L. (2014). Hypoxia-inducible factor-dependent signaling between triple-negative breast cancer cells and mesenchymal stem cells promotes macrophage recruitment. Proc. Natl. Acad. Sci. 111. E2120, E2129. doi:10.1073/pnas.1406655111
Chen, S., Liang, Q., Liu, E., Yu, Z., Sun, L., Ye, J., et al. (2017). Curcumin/sunitinib co-loaded bsa-stabilized spios for synergistic combination therapy for breast cancer. J. Mater. Chem. B 5, 4060–4072. doi:10.1039/c7tb00040e
Cheng, Y.-H., He, C., Riviere, J. E., Monteiro-Riviere, N. A., and Lin, Z. (2020). Meta-analysis of nanoparticle delivery to tumors using a physiologically based pharmacokinetic modeling and simulation approach. ACS Nano 14, 3075–3095. doi:10.1021/acsnano.9b08142
China, N. H. C. P. R. (2023). “Guidelines for diagnosis and treatment of advanced breast cancer in China (2022 edition),” in Journal of the national cancer center. doi:10.1016/j.jncc.2023.12.001
Chowdhury, N., Chaudhry, S., Hall, N., Olverson, G., Zhang, Q., Mandal, T., et al. (2020). Targeted delivery of doxorubicin liposomes for her-2+ breast cancer treatment. AAPS PharmSciTech 21, 202. doi:10.1208/s12249-020-01743-8
Da, A. (2021). “Applications of nano-drugs and tumor microenvironment sensitive nano-drug delivery systems,” in Proceedings of the 2020 9th international conference on bioinformatics and biomedical science (New York, NY, USA: Association for Computing Machinery), 1–7. ICBBS ’20. doi:10.1145/3431943.3431944
Dahiya, R., and Dahiya, S. (2021). Advanced drug delivery applications of self-assembled nanostructures and polymeric nanoparticles. 297, 339. doi:10.1016/b978-0-12-821013-0.00021-0
Das, N., Murray, D. T., and Cross, T. A. (2013). Lipid bilayer preparations of membrane proteins for oriented and magic-angle spinning solid-state nmr samples. Nat. Protoc. 8, 2256–2270. doi:10.1038/nprot.2013.129
Dehaini, D., Fang, R. H., Luk, B. T., Pang, Z., Hu, C. J., Kroll, A. V., et al. (2016). Ultra-small lipid–polymer hybrid nanoparticles for tumor-penetrating drug delivery. Nanoscale 8, 14411–14419. doi:10.1039/c6nr04091h
Devi, S., Sharma, Y. K., and Shah, D. (2023). Randomized phase iii trial comparing epirubicin/doxorubicin plus docetaxel and epirubicin/doxorubicin plus paclitaxel as first line treatment in women with advanced breast cancer. Int. J. Med. Biomed. Stud. 5. doi:10.32553/ijmbs.v5i2.1746
Du, M., Ouyang, Y., Meng, F., Zhang, X., Ma, Q., Zhuang, Y., et al. (2019). Polymer-lipid hybrid nanoparticles: a novel drug delivery system for enhancing the activity of psoralen against breast cancer. Int. J. Pharm. 561, 274–282. doi:10.1016/j.ijpharm.2019.03.006
Duggan, S. T., and Keating, G. M. (2011). Pegylated liposomal doxorubicin. Drugs 71, 2531–2558. doi:10.2165/.11207510-000000000-00000
Durr, F. E., Wallace, R. E., and Citarella, R. V. (1983). Molecular and biochemical pharmacology of mitoxantrone. Cancer Treat. Rev. 10, 3–11. doi:10.1016/0305-7372(83)90016-6
Ewert, K. K., Scodeller, P., Simón-Gracia, L., Steffes, V. M., Wonder, E. A., Teesalu, T., et al. (2023). Cationic liposomes as vectors for nucleic acid and hydrophobic drug therapeutics. Pharmaceutics 13, 1365. doi:10.3390/pharmaceutics13091365
Fabi, A., Ferretti, G., Malaguti, P., Gasparro, S., Nisticò, C., Arpino, G., et al. (2020). Nanoparticle albumin-bound paclitaxel/liposomal-encapsulated doxorubicin in her2-negative metastatic breast cancer patients. Future Oncol. 16, 1631–1639. doi:10.2217/fon-2019-0742
Fabian, C. J., Khan, S. A., Garber, J. E., Dooley, W. C., Yee, L. D., Klemp, J. R., et al. (2020). Randomized phase iib trial of the lignan secoisolariciresinol diglucoside in premenopausal women at increased risk for development of breast cancer. Cancer Prev. Res. 13, 623–634. doi:10.1158/1940-6207.capr-20-0050
Fan, Z., Sun, L., Huang, Y., Wang, Y., and Zhang, M. (2016). Bioinspired fluorescent dipeptide nanoparticles for targeted cancer cell imaging and real-time monitoring of drug release. Nat. Nanotechnol. 11, 388–394. doi:10.1038/nnano.2015.312
Fujiwara, Y., Mukai, H., Saeki, T., Ro, J., Lin, Y.-C., Nagai, S. E., et al. (2019). A multi-national, randomised, open-label, parallel, phase iii non-inferiority study comparing nk105 and paclitaxel in metastatic or recurrent breast cancer patients. Br. J. Cancer 120, 475–480. doi:10.1038/s41416-019-0391-z
Futamura, M., Ishihara, K., Nagao, Y., Ogiso, A., Niwa, Y., Nakada, T., et al. (2023). Neoadjuvant chemotherapy using nanoparticle albumin-bound paclitaxel plus trastuzumab and pertuzumab followed by epirubicin and cyclophosphamide for operable her2-positive primary breast cancer: a multicenter phase ii clinical trial (perseus-bc04). Breast Cancer 30, 293–301. doi:10.1007/s12282-022-01425-2
Galluzzi, L., Vitale, I., Warren, S., Adjemian, S., Agostinis, P., Martinez, A. B., et al. (2020). Consensus guidelines for the definition, detection and interpretation of immunogenic cell death. J. Immunother. Cancer 8, e000337. doi:10.1136/jitc-2019-000337
Genio, V. D., Falanga, A., Allard-Vannier, E., Hervé-Aubert, K., Leone, M., Bellavita, R., et al. (2022). Design and validation of nanofibers made of self-assembled peptides to become multifunctional stimuli-sensitive nanovectors of anticancer drug doxorubicin. Pharmaceutics 14, 1544. doi:10.3390/pharmaceutics14081544
Germain, R., and Kastenmüller, W. (2010). Faculty opinions recommendation of therapeutic cell engineering with surface-conjugated synthetic nanoparticles. doi:10.3410/f.5179959.5117058
Govindan, B., Sabri, M. A., Hai, A., Banat, F., and Haija, M. A. (2023). A review of advanced multifunctional magnetic nanostructures for cancer diagnosis and therapy integrated into an artificial intelligence approach. Pharmaceutics 15, 868. doi:10.3390/pharmaceutics15030868
Grantab, R., Sivananthan, S., and Tannock, I. F. (2006). The penetration of anticancer drugs through tumor tissue as a function of cellular adhesion and packing density of tumor cells. Cancer Res. 66, 1033–1039. doi:10.1158/0008-5472.can-05-3077
Gucalp, A., Zhou, X. K., Cook, E. D., Garber, J. E., Crew, K. D., Nangia, J. R., et al. (2018). A randomized multicenter phase ii study of docosahexaenoic acid in patients with a history of breast cancer, premalignant lesions, or benign breast disease. Cancer Prev. Res. 11, 203–214. doi:10.1158/1940-6207.capr-17-0354
Hamdi, M., Abdel-Bar, H. M., Elmowafy, E., Al-Jamal, K. T., and Awad, G. A. S. (2020). An integrated vitamin e-coated polymer hybrid nanoplatform: a lucrative option for an enhanced in vitro macrophage retention for an anti-hepatitis b therapeutic prospect. PLOS ONE 15, e0227231. doi:10.1371/journal.pone.0227231
Hekmat, A., Saso, L., Lather, V., Pandita, D., Kostova, I., and Saboury, A. A. (2022). Recent advances in nanomaterials of group xiv elements of periodic table in breast cancer treatment. Pharmaceutics 14, 2640. doi:10.3390/pharmaceutics14122640
Hoshyar, N., Gray, S., Han, H., and Bao, G. (2016). The effect of nanoparticle size on in vivo pharmacokinetics and cellular interaction. Nanomedicine 11, 673–692. doi:10.2217/nnm.16.5
Hossain, S. S., Ebrahimi, M. R., Padmanabhan, B., Naqa, I. E., Kuo, P. C., Beard, A., et al. (2023). Robust ai-enabled simulation of treatment paths with markov decision process for breast cancer patients. IEEE 53, 105–108. doi:10.1109/CAI54212.2023.00053
Jain, A., Kesharwani, P., Garg, N. K., Jain, A., Jain, S. A., Jain, A. K., et al. (2015). Galactose engineered solid lipid nanoparticles for targeted delivery of doxorubicin. Colloids Surfaces B Biointerfaces 134, 47–58. doi:10.1016/j.colsurfb.2015.06.027
Jemal, A., Bray, F., Center, M. M., Ferlay, J., and Forman, D. (2011). Erratum: global cancer statistics. CA A Cancer J. Clin. 61, 134. doi:10.3322/caac.20115
Kaur, M., Guleria, P., and Kumar, V. (2023). Nanomaterials for diagnosis and treatment of lung cancer: a review of recent patents. Recent Pat. Anti-Cancer Drug Discov. 18, 114–124. doi:10.2174/1574892817666220629104641
Kim, D.-W., Kim, S.-Y., Kim, H.-K., Kim, S.-W., Shin, S. W., Kim, J. S., et al. (2007). Multicenter phase ii trial of genexol-pm, a novel cremophor-free, polymeric micelle formulation of paclitaxel, with cisplatin in patients with advanced non-small-cell lung cancer. Ann. Oncol. 18, 2009–2014. doi:10.1093/annonc/mdm374
Kitsios, K., Sharifi, S., and Mahmoudi, M. (2023). Nanomedicine technologies for diagnosis and treatment of breast cancer. ACS Pharmacol. and Transl. Sci. 6, 671–682. doi:10.1021/acsptsci.3c00044
Klibanov, A. L., Maruyama, K., Torchilin, V. P., and Huang, L. (1990). Amphipathic polyethyleneglycols effectively prolong the circulation time of liposomes. FEBS Lett. 268, 235–237. doi:10.1016/0014-5793(90)81016-h
Kshivets, O. (2023). Gastric cancer: neural networks, complex system analysis, statistics and computer modeling for best management. JCO Glob. Oncol. 9, 37. doi:10.1200/go.2023.9.supplement_1.37
Kulkarni, P., and Rawtani, D. (2019). Application of box-behnken design in the preparation, optimization, and in vitro evaluation of self-assembly–based tamoxifen- and doxorubicin-loaded and dual drug–loaded niosomes for combinatorial breast cancer treatment. J. Pharm. Sci. 108, 2643–2653. doi:10.1016/j.xphs.2019.03.020
Kumar, R., Mondal, K., Panda, P. K., Kaushik, A., Abolhassani, R., Ahuja, R., et al. (2020). Core–shell nanostructures: perspectives towards drug delivery applications. J. Mater. Chem. B 8, 8992–9027. doi:10.1039/d0tb01559h
Li, X., He, M., Zhou, Z., Jiang, Y., and Cheng, L. (2015). The antitumor activity of pna modified vinblastine cationic liposomes on lewis lung tumor cells: in vitro and in vivo evaluation. Int. J. Pharm. 487, 223–233. doi:10.1016/j.ijpharm.2015.04.035
Li, X., Ju, R., Li, X., Zeng, F., Shi, J., Liu, L., et al. (2014). Multifunctional targeting daunorubicin plus quinacrine liposomes, modified by wheat germ agglutinin and tamoxifen, for treating brain glioma and glioma stem cells. Oncotarget 5, 6497–6511. doi:10.18632/oncotarget.2267
Li, Y., Wang, T., Hou, X., Zhang, J., Bai, W., et al. (2024). Extracellular vesicles: opening up a new perspective for the diagnosis and treatment of mitochondrial dysfunction. , 22, 487, 515. doi:10.1186/s12951-024-02750-8
Lim, Z.-F., and Ma, P. C. (2019). Emerging insights of tumor heterogeneity and drug resistance mechanisms in lung cancer targeted therapy. J. Hematol. and amp; Oncol. 12, 134. doi:10.1186/s13045-019-0818-2
Liu, S., Song, X.-L., Wang, Y.-H., Wang, X.-M., Xiao, Y., Wang, X., et al. (2017). The efficacy of wga modified daunorubicin anti-resistant liposomes in treatment of drug-resistant mcf-7 breast cancer. J. Drug Target. 25, 541–553. doi:10.1080/1061186x.2017.1298602
Logigan, C. L., Peptu, C., and Peptu, C. A. (2024). Liposomes for delivery of substances for other (non-therapeutic) applications. 435, 460. doi:10.1016/b978-0-443-15491-1.00014-6
Lynn, D. M., and Langer, R. (2000). Degradable poly(β-amino esters): synthesis, characterization, and self-assembly with plasmid DNA. J. Am. Chem. Soc. 122, 10761–10768. doi:10.1021/ja0015388
Makowski, M., Ítala, C. S., do Amaral, C. P., Gonçalves, S., and Santos, N. C. (2019). Advances in lipid and metal nanoparticles for antimicrobial peptide delivery. Pharmaceutics 11, 588. doi:10.3390/pharmaceutics11110588
Malloizel-Delaunay, J., Weyl, A., Brusq, C., Chaput, B., Garmy-Susini, B., Bongard, V., et al. (2024). New strategy for breast cancer related lymphedema treatment by endermology: elocs phase ii randomized controlled trial. Clin. Breast Cancer 24, 533–540. doi:10.1016/j.clbc.2024.05.009
Martin, J. D., Seano, G., and Jain, R. K. (2019). Normalizing function of tumor vessels: progress, opportunities, and challenges. Annu. Rev. Physiology 81, 505–534. doi:10.1146/annurev-physiol-020518-114700
Martinelli, C., Pucci, C., and Ciofani, G. (2019). Nanostructured carriers as innovative tools for cancer diagnosis and therapy. Apl. Bioeng. 3, 011502. doi:10.1063/1.5079943
McClements, D. J. (2012). Nanoemulsions versus microemulsions: terminology, differences, and similarities. Soft Matter 8, 1719–1729. doi:10.1039/c2sm06903b
Meng, H., Wang, M., Liu, H., Liu, X., Situ, A., Wu, B., et al. (2015). Use of a lipid-coated mesoporous silica nanoparticle platform for synergistic gemcitabine and paclitaxel delivery to human pancreatic cancer in mice. ACS Nano 9, 3540–3557. doi:10.1021/acsnano.5b00510
Menjoge, A. R. (2010). Poly(amidoamine) dendrimer-based multifunctional engineered nanodevice for cancer therapy. J. Am. Chem. Soc. 132 (26), 9482–9484. doi:10.1021/jm0401863
Mohammadpour, Z., and Majidzadeh, K. (2020). Applications of two-dimensional nanomaterials in breast cancer theranostics. ACS Biomaterials Sci. and amp; Eng. 6, 1852–1873. doi:10.1021/acsbiomaterials.9b01894
Nazari-Vanani, R., Azarpira, N., Heli, H., Karimian, K., and Sattarahmady, N. (2017). A novel self-nanoemulsifying formulation for sunitinib: evaluation of anticancer efficacy. Colloids Surfaces B Biointerfaces 160, 65–72. doi:10.1016/j.colsurfb.2017.09.008
Ning, Z. (2022). Combined effects of pc002 and anticancer drugs in ewing’s sarcoma cells. Proc. 3rd Int. Symposium Artif. Intell. Med. Sci. 22, 420–424. doi:10.1145/3570773.3570869
Pang, J., Xing, H., Sun, Y., Feng, S., and Wang, S. (2020). Non-small cell lung cancer combination therapy: hyaluronic acid modified, epidermal growth factor receptor targeted, ph sensitive lipid-polymer hybrid nanoparticles for the delivery of erlotinib plus bevacizumab. Biomed. and Pharmacother. 125, 109861. doi:10.1016/j.biopha.2020.109861
Pérez-Herrero, E., and Fernández-Medarde, A. (2015). Advanced targeted therapies in cancer: drug nanocarriers, the future of chemotherapy. Eur. J. Pharm. Biopharm. 93, 52–79. doi:10.1016/j.ejpb.2015.03.018
Pestalozzi, B., Schwendener, R., and Sauter, C. (1992). Phase i/ii study of liposome-complexed mitoxantrone in patients with advanced breast cancer. Ann. Oncol. 3, 445–449. doi:10.1093/oxfordjournals.annonc.a058232
Pestalozzi, B. C., Vass, A., Adam, H., Horber, D. H., Schwendener, R. A., and Sauter, C. (1995). Phase ii study of liposome-complexed mitoxantrone in patients with advanced breast cancer. Eur. J. Cancer 31, 1024. doi:10.1016/0959-8049(95)00010-0
Poujol, S., Tilleul, P., Astre, C., Martel, P., Fabbro, M., and Pinguet, F. (1999). Effect of mitoxantrone liposomes on multidrug-resistant breast cancer cells. Anticancer Res. 19, 3327–3331.
Puri, A., Loomis, K., Smith, B., Lee, J., Yavlovich, A., Heldman, E., et al. (2009). Lipid-based nanoparticles as pharmaceutical drug carriers: from concepts to clinic. Crit. Rev. Ther. Drug Carr. Syst. 26, 523–580. doi:10.1615/critrevtherdrugcarriersyst.v26.i6.10
Qiao, L., Han, M., Gao, S., Shao, X., Wang, X., Sun, L., et al. (2020). Research progress on nanotechnology for delivery of active ingredients from traditional Chinese medicines. J. Mater. Chem. B 8, 6333–6351. doi:10.1039/d0tb01260b
Rahman, A., Panneerselvam, M., Guirguis, R., Castronovo, V., Sobel, M. E., Abraham, K., et al. (1989). Anti-laminin receptor antibody targeting of liposomes with encapsulated doxorubicin to human breast cancer cells in vitro. JNCI J. Natl. Cancer Inst. 81, 1794–1800. doi:10.1093/jnci/81.23.1794
Rao, S., and Prestidge, C. A. (2016). Polymer-lipid hybrid systems: merging the benefits of polymeric and lipid-based nanocarriers to improve oral drug delivery. Expert Opin. Drug Deliv. 13, 691–707. doi:10.1517/17425247.2016.1151872
Riccardi, C., Fàbrega, C., Grijalvo, S., Vitiello, G., D’Errico, G., Eritja, R., et al. (2018). As1411-decorated niosomes as effective nanocarriers for ru(iii)-based drugs in anticancer strategies. J. Mater. Chem. B 6, 5368–5384. doi:10.1039/c8tb01563e
Riis, M. (2020). Modern surgical treatment of breast cancer. Ann. Med. Surg. 56, 95–107. doi:10.1016/j.amsu.2020.06.016
Ruttala, H. B., and Ko, Y. T. (2015a). Liposomal co-delivery of curcumin and albumin/paclitaxel nanoparticle for enhanced synergistic antitumor efficacy. Colloids Surfaces B Biointerfaces 128, 419–426. doi:10.1016/j.colsurfb.2015.02.040
Ruttala, H. B., and Ko, Y. T. (2015b). Liposome encapsulated albumin-paclitaxel nanoparticle for enhanced antitumor efficacy. Pharm. Res. 32, 1002–1016. doi:10.1007/s11095-014-1512-2
Sabahi, F. (2023). A design of mrna-based covid-19 vaccine through fuzzy neural network. IEEE/ACM Trans. Comput. Biol. Bioinform. 20, 3648–3659. doi:10.1109/TCBB.2023.3309650
Sabit, H., Abdel-Hakeem, M., Shoala, T., Abdel-Ghany, S., Abdel-Latif, M. M., Almulhim, J., et al. (2022). Nanocarriers: a reliable tool for the delivery of anticancer drugs. Pharmaceutics 14, 1566. doi:10.3390/pharmaceutics14081566
Sarhangi, N., Hajjari, S., Heydari, S. F., Ganjizadeh, M., Rouhollah, F., and Hasanzad, M. (2022). Breast cancer in the era of precision medicine. Mol. Biol. Rep. 49, 10023–10037. doi:10.1007/s11033-022-07571-2
Schwartz, S. (2017). Unmet needs in developing nanoparticles for precision medicine. Nanomedicine 12, 271–274. doi:10.2217/.nnm-2016-0390
Sengel-Turk, C. T., Ozmen, N., and Bakar-Ates, F. (2021). Design, characterization and evaluation of cucurbitacin b-loaded core–shell-type hybrid nano-sized particles using doe approach. Polym. Bull. 78, 3327–3351. doi:10.1007/.s00289-020-03256-7
Shi, J., Xu, Y., Xu, X., Zhu, X., Pridgen, E., Wu, J., et al. (2014). Hybrid lipid–polymer nanoparticles for sustained sirna delivery and gene silencing. Nanomedicine Nanotechnol. Biol. Med. 10, e897–e900. doi:10.1016/j.nano.2014.03.006
Shi, K., Zhou, J., Zhang, Q., Gao, H., Liu, Y., Zong, T., et al. (2015). Arginine-glycine-aspartic acid-modified lipid-polymer hybrid nanoparticles for docetaxel delivery in glioblastoma multiforme. J. Biomed. Nanotechnol. 11, 382–391. doi:10.1166/jbn.2015.1965
Shi, W., Wan, X., Wang, Y., He, J., Huang, X., Xu, Y., et al. (2023). Nanoparticle albumin-bound paclitaxel-based neoadjuvant regimen: a promising treatment option for her2-low-positive breast cancer. Nanomedicine Nanotechnol. Biol. Med. 49, 102666. doi:10.1016/j.nano.2023.102666
Song, J., Zhou, J., and Duan, H. (2012). Self-assembled plasmonic vesicles of sers-encoded amphiphilic gold nanoparticles for cancer cell targeting and traceable intracellular drug delivery. J. Am. Chem. Soc. 134, 13458–13469. doi:10.1021/ja305154a
Stephanopoulos, N., Ortony, J. H., and Stupp, S. I. (2013). Self-assembly for the synthesis of functional biomaterials. Acta Mater. 61, 912–930. doi:10.1016/j.actamat.2012.10.046
Stylianopoulos, T., Munn, L. L., and Jain, R. K. (2018). Reengineering the tumor vasculature: improving drug delivery and efficacy. Trends Cancer 4, 258–259. doi:10.1016/j.trecan.2018.02.010
Tahir, N., Madni, A., Correia, A., Rehman, M., Balasubramanian, V., Khan, M. M., et al. (2020). Lipid-polymer hybrid nanoparticles forcontrolled delivery of hydrophilic and lipophilic doxorubicin for breast cancer therapy [erratum]. Int. J. Nanomedicine 15, 839–840. doi:10.2147/ijn.s247761
Torchilin, V. P. (2005). Recent advances with liposomes as pharmaceutical carriers. Nat. Rev. Drug Discov. 4, 145–160. doi:10.1038/nrd1632
Torchilin, V. P. (2006). Micellar nanocarriers: pharmaceutical perspectives. Pharm. Res. 24, 1–16. doi:10.1007/.s11095-006-9132-0
Tredan, O., Galmarini, C. M., Patel, K., and Tannock, I. F. (2007). Drug resistance and the solid tumor microenvironment. JNCI J. Natl. Cancer Inst. 99, 1441–1454. doi:10.1093/jnci/djm135
Tseu, G. Y. W., and Kamaruzaman, K. A. (2023). A review of different types of liposomes and their advancements as a form of gene therapy treatment for breast cancer. Molecules 28, 1498. doi:10.3390/molecules28031498
Ulbrich, K., Holá, K., Šubr, V., Bakandritsos, A., Tuček, J., and Zbořil, R. (2016). Targeted drug delivery with polymers and magnetic nanoparticles: covalent and noncovalent approaches, release control, and clinical studies. Chem. Rev. 116, 5338–5431. doi:10.1021/acs.chemrev.5b00589
Varma, L. T., Singh, N., Gorain, B., Choudhury, H., Tambuwala, M. M., Kesharwani, P., et al. (2020). Recent advances in self-assembled nanoparticles for drug delivery. Curr. Drug Deliv. 17, 279–291. doi:10.2174/1567201817666200210122340
von Roemeling, C., Jiang, W., Chan, C. K., Weissman, I. L., and Kim, B. Y. S. (2017). Breaking down the barriers to precision cancer nanomedicine. Trends Biotechnol. 35, 159–171. doi:10.1016/j.tibtech.2016.07.006
Wang, L., Hong, Y., Ma, J., Han, M., Zhang, S., Shan, B., et al. (2020). Combination of pegylated liposomal doxorubicin and docetaxel as neoadjuvant therapy for breast cancer with axillary lymph node metastasis. J. Int. Med. Res. 48, 030006052094431. doi:10.1177/0300060520944310
Waterhouse, D. N., Tardi, P. G., Mayer, L. D., and Bally, M. B. (2001). A comparison of liposomal formulations of doxorubicin with drug administered in free form. Drug Saf. 24, 903–920. doi:10.2165/00002018-200124120-00004
Wu, D., Si, M., Xue, H., and Wong, H. L. (2017). Nanomedicine applications in the treatment of breast cancer: current state of the art. Int. J. Nanomedicine 12, 5879–5892. doi:10.2147/ijn.s123437
Wu, R., Zhang, Z., Wang, B., Chen, G., Zhang, Y., Deng, H., et al. (2020). Combination chemotherapy of lung cancer – co-delivery of docetaxel prodrug and cisplatin using aptamer-decorated lipid–polymer hybrid nanoparticles. Drug Des. Dev. Ther. 14, 2249–2261. doi:10.2147/dddt.s246574
Wu, Y., Xie, H., Li, Y., Bao, X., Lu, G.-L., Wen, J., et al. (2023). Nitric oxide-loaded bioinspired lipoprotein normalizes tumor vessels to improve intratumor delivery and chemotherapy of albumin-bound paclitaxel nanoparticles. Nano Lett. 23, 939–947. doi:10.1021/acs.nanolett.2c04312
Xie, W., and Pedrielli, G. (2023). “From discovery to production: challenges and novel methodologies for next generation biomanufacturing,” in Proceedings of the winter simulation conference (Singapore, Singapore: IEEE Press), WSC ’22), 238–252.
Xie, X., Liao, J., Shao, X., Li, Q., and Lin, Y. (2017). The effect of shape on cellular uptake of gold nanoparticles in the forms of stars, rods, and triangles. Sci. Rep. 7, 3827. doi:10.1038/s41598-017-04229-z
Yang, B., ping Song, B., Shankar, S., Guller, A., and Deng, W. (2021). Recent advances in liposome formulations for breast cancer therapeutics. Cell. Mol. Life Sci. 78, 5225–5243. doi:10.1007/s00018-021-03850-6
Yang, L., Zhang, Y., Zhang, Y., Xu, Y., Li, Y., Xie, Z., et al. (2022). Live macrophage-delivered doxorubicin-loaded liposomes effectively treat triple-negative breast cancer. ACS Nano 16, 9799–9809. doi:10.1021/acsnano.2c03573
Zhang, H., Dong, S., Zhang, S., Li, Y., Li, J., Dai, Y., et al. (2021). pH-responsive lipid polymer hybrid nanoparticles (LPHNs) based on poly (β-amino ester) as a promising candidate to resist breast cancers. J. Drug Deliv. Sci. Technol. 61, 102102. doi:10.1016/j.jddst.2020.102102
Keywords: self-assembly, lipids, nanoparticles, breast cancer, chemotherapy
Citation: Liu S (2024) Self-assembled lipid-based nanoparticles for chemotherapy against breast cancer. Front. Bioeng. Biotechnol. 12:1482637. doi: 10.3389/fbioe.2024.1482637
Received: 18 August 2024; Accepted: 18 September 2024;
Published: 29 October 2024.
Edited by:
Weifeng Lin, Beihang University, ChinaReviewed by:
Venkatesh Srinivasan, University of Maryland, United StatesCopyright © 2024 Liu. This is an open-access article distributed under the terms of the Creative Commons Attribution License (CC BY). The use, distribution or reproduction in other forums is permitted, provided the original author(s) and the copyright owner(s) are credited and that the original publication in this journal is cited, in accordance with accepted academic practice. No use, distribution or reproduction is permitted which does not comply with these terms.
*Correspondence: Shan Liu, bGl1c2hhbnNqemxAMTYzLmNvbQ==
Disclaimer: All claims expressed in this article are solely those of the authors and do not necessarily represent those of their affiliated organizations, or those of the publisher, the editors and the reviewers. Any product that may be evaluated in this article or claim that may be made by its manufacturer is not guaranteed or endorsed by the publisher.
Research integrity at Frontiers
Learn more about the work of our research integrity team to safeguard the quality of each article we publish.