- 1Department of Speech, Language, and Hearing Sciences, University of Connecticut, Storrs, CT, United States
- 2Institute for Brain and Cognitive Sciences, University of Connecticut, Storrs, CT, United States
- 3Department of Psychology and Neuroscience, Duke University, Durham, NC, United States
- 4Department of Psychiatry and Behavioral Sciences, Stanford University School of Medicine, Stanford, CA, United States
- 5Duke Institute for Brain Sciences, Duke University, Durham, NC, United States
- 6Center for Cognitive Neuroscience, Duke University, Durham, NC, United States
Introduction: Among several controversies surrounding the field of Auditory Processing Disorder (APD), one of the central unresolved topics is the putative neural origin of APD. More specifically, it is debated whether basic sensory auditory neural processes are affected in individuals with APD. The objective of the current study was to understand whether or not basic sensory auditory neural processes at the level of the brainstem are affected in those with APD.
Methods: We approached this question by conducting a meta-analysis of studies that compared the Auditory Brainstem Response (ABR) to brief non-speech sounds in individuals with vs. without APD. The ultimate criterion for a study to be included in this meta-analysis was the presence of both APD and non-APD groups on whom ABR waves I, III, and V were collected in response to clicks. In order to extract these studies, a list of inclusion and exclusion criteria were employed during our search using Google Scholar and PubMed databases (accessed between March 2021 and July 2023), resulting in the inclusion of 8 studies. From these studies, we retrieved ABR waves I, III, and V peak amplitude and latency measures.
Results: Overall, we found no significant differences between those with and without APD on the ABR waves peak latency (wave I: effect size = −0.0365, C.I. = 0.0384; wave III: effect size = −0.0540, C.I. = 0.1417; wave V: effect size = −0.0577, C.I. = 0.1589) and peak amplitude measures (wave I: effect size = 0.0327, C.I. = 0.0473; wave III: effect size = 0.1415, C.I. = 0.1648; wave V: effect size = 0.1281, C.I. = 0.1346).
Conclusion: These findings suggest that the click-evoked ABR does not seem to be implicated in those with APD.
Introduction
Auditory Processing Disorder (APD) has been a controversial topic in the field of Audiology for decades. Among the several controversies surrounding APD [sometimes also referred to as “Central Auditory Processing Disorder” or “(Central) Auditory Processing Disorder”], one of the key issues is the putative neural origins of APD (Moore, 2018; Vermiglio, 2018). Here we investigated whether or not APD is associated with deficits in basic sensory auditory processing at the level of the auditory brainstem by conducting a meta-analysis of the existing literature. More specifically, we examined whether studies that measured the click-evoked auditory brainstem response (ABR), a key index of basic sensory auditory neural processing at the brainstem, revealed differences between individuals with vs. without a clinical indication of APD.
Based on the current definition by the American Speech Language and Hearing Association (American Speech-Language-Hearing Association, 2005a, Definition and Nature of APD, paragraph 1), an APD diagnosis is considered in the case of reduced performance on behavioral tests involving auditory discrimination, temporal processing, binaural processing, and speech perception in adverse listening conditions. The current diagnostic criteria entail performance at 2 standard deviations (SD) below the respective age-matched normative data on two or more behavioral tests (Chermak and Musiek, 1997), or performance below 3 SD on at least one behavioral test (American Speech-Language-Hearing Association, 2005b). Even though the current diagnostic criteria are based solely on behavioral performance metrics, ASHA states that APD has origins in the auditory nervous system, is specific to the auditory domain, and is unaffected by other higher-order processes such as language and cognition (American Speech-Language-Hearing Association, 2005b). Similarly, the report from the consensus conference of 14 experts in the field of APD (Jerger and Musiek, 2000) suggested that “An APD may be broadly classified as a deficit in the processing of information that is specific to the auditory modality” (page 468). Given the assumed specificity of APD to the auditory domain (and, therefore, its neural origins in the auditory system), a thorough investigation of such putative neural origins in the auditory system is warranted.
Prevalence of APD in school-aged children has been found to range between 2.5% (Schow et al., 2020) to 6.2% (Esplin and Wright, 2014). Children with APD may exhibit delay in their speech and language acquisition (Magimairaj et al., 2020, 2021) and lags in their academic performance (de Carvalho et al., 2017). These difficulties in listening extend well into adolescence (Kojima et al., 2024) and adulthood (Del Zoppo et al., 2015). As a result, the current meta-analysis focused on the findings from studies that included participants over an age range of 5–24 years.
Scalp recorded auditory evoked potentials (AEPs) provide viable means to investigate the neural origins of APD. AEPs can be classified as those elicited from the peripheral (subcortical) level of the auditory nervous system (e.g., ABR), and those elicited from the central (cortical) level of the auditory nervous system (e.g., Long Latency Response) (Picton et al., 1981). Cortical AEPs are known to be more prone to modulation by higher-order cognitive and linguistic processes (Cacace and McFarland, 2013). For example, attending to auditory stimuli enhances the amplitudes of the corresponding cortical components (e.g., N1 and P2) (Davis, 1964; Gross et al., 1965; Satterfield, 1965; Picton and Hillyard, 1974). Similarly, language experience enhances the amplitude of the cortical components. For example, individuals with tone language experience exhibit enhanced amplitude for pitch-related cortical components in the latency range of 600–900 ms (e.g., Pa, Na, Pb, Nb, Pc, and Nc) (Krishnan et al., 2014a,b). In contrast, depending on the type of stimuli used, subcortical AEPs are less affected by higher-order processes (e.g., attention, memory, and language) and can therefore be considered an indicator of basic sensory auditory processing. For example, the amplitude of the click-evoked ABR is generally unaffected by attention and thus, can be recorded while participants are asleep (Elsayed et al., 2015).
Over the years, the ABR has emerged as a popular subcortical AEP technique in the field of audiology for auditory threshold estimation (Stapells, 2000) and retrocochlear site-of-lesion testing (Starr, 1976; Glasscock et al., 1979; Achor and Starr, 1980; Bauch et al., 1982; Musiek and Geurkink, 1982; Rhee et al., 1999). Typically, the ABR occurs within the first 8-10 ms following stimulus onset and contains five-seven peaks, namely waves I, II, III, IV, and V, VI, and VII (Jewett and Williston, 1971). While the later waves of the ABR may have overlapping anatomical generators, predominantly, wave I of the ABR originates from the distal part of the auditory nerve, wave II from the proximal part of the auditory nerve and cochlear nucleus, wave III from the superior olivary colliculi, wave IV from the lateral lemniscus, and wave V from the inferior colliculi (Møller and Jannetta, 1985; Melcher et al., 1996a,b). The ABR has clinical relevance in that it is sensitive to detecting vestibular schwannomas, acoustic neuromas, intra- and extra-axial brainstem lesions, demyelination, and auditory neuropathy (Young et al., 2021). Individuals with disorders such as auditory neuropathy and acoustic neuroma exhibit difficulties in speech perception in noise (Apeksha and Kumar, 2017) and individuals with lower brainstem lesions (e.g., superior olivary colliculi) exhibit problems with sound localization (Masterton and Imig, 1984), both of which are characteristics of APD (American Speech-Language-Hearing Association, 2005a). More specifically, a reduced wave I amplitude of the ABR at higher intensities indicates damaged low spontaneous rate type-1 auditory nerve fibers (Kujawa and Liberman, 2009). Similarly, a reduced ABR wave amplitude and prolonged ABR wave latency at high stimulus repetition rates (e.g., 90.1/s) are indicative of neural processing dys-synchrony or auditory neuropathy (Cone-Wesson, 2004). An inter-aural I-V interpeak latency difference is indicative of vestibular schwannomas (Tanaka et al., 1996). To differentially diagnose the lesion in the lower (cochlear nucleus and superior olivary colliculi) vs. upper brainstem (lateral lemniscus and inferior colliculi), interaural and interpeak (I-III, III-V, I-V) latencies and amplitude are useful (Hood, 1998). Given the usefulness of the click-evoked ABR in detecting auditory deficits, an investigation into the waves I, III, and V amplitudes and latencies of the click-evoked ABR for detecting deficits in auditory processing is warranted.
In order to investigate whether basic sensory auditory neural processes (up to the inferior colliculi) are aberrant in APD, we conducted a meta-analysis of the existing literature that recorded ABRs for individuals diagnosed with APD (American Speech-Language-Hearing Association, 2005a,b). The metrics of interest included peak amplitude and peak latency for waves I, III, and V, which are routinely recorded in auditory electrophysiological evaluations, especially for site-of-lesion testing purposes (Xie et al., 2018). Clinically, the most commonly used stimuli for recording ABRs are clicks. Since these stimuli are transients of very short duration (e.g., 100 μs), devoid of cognitive or linguistic load, the ABRs evoked by such stimuli are largely unaffected by the higher-order processes of attention or language and thus can be considered as measures of “pure”, or selective auditory processing that is unaffected by the nonauditory factors (e.g., sleep, cognition, language experience) (Davis and Beagley, 1985; Bansal, 2018). If the meta-analysis revealed an overall difference between APD vs. non-APD groups with respect to their ABR metrics (latencies and/or amplitudes of waves I, III, and/or V), this would indicate that the click-evoked ABR peak amplitude and latency are sensitive measures for detecting APD. Conversely, if the meta-analysis revealed no difference between APD vs. non-APD groups with respect to the ABR metrics, this would indicate that the click-evoked ABR peak amplitude and latency are not sensitive for detecting APD.
Material and methods
Eligibility criteria
For the identification of relevant studies, our inclusion criterion was that studies needed to compare APD vs. non-APD groups with respect to the amplitude and latency measures of the traditional click-evoked ABR (i.e., waves I, III, and/or V).
Search strategy and information sources
The following keywords were used to search for the relevant literature in Google Scholar and PubMed: Auditory Processing Disorder, Auditory Processing deficits, Auditory Processing Disorder testing, Auditory Processing Disorder electrophysiological testing, Auditory Processing Disorder Auditory Evoked potentials, Auditory Processing Disorder EEG, Auditory Processing Disorder objective testing, and Auditory Processing Disorder physiological testing. The search used Boolean OR and no wild card characters were used to truncate or combine search terms. Out of the search results, we identified those studies that were pertinent to Auditory Processing testing. As a result, we excluded, amongst others, studies that focused on the developmental maturation of auditory processing, studies that were based on screening and diagnostic test development for APD, studies that investigated neuroimaging data (e.g., MRI), studies that were based on evoked potentials other than ABR (e.g., long latency response, mismatch negativity), and studies that were solely based on auditory training for APD.
Coding
The included studies were coded and summarized by the first and the second authors with respect to their APD diagnostic criteria, ABR parameters, and overall findings (see Table 1).
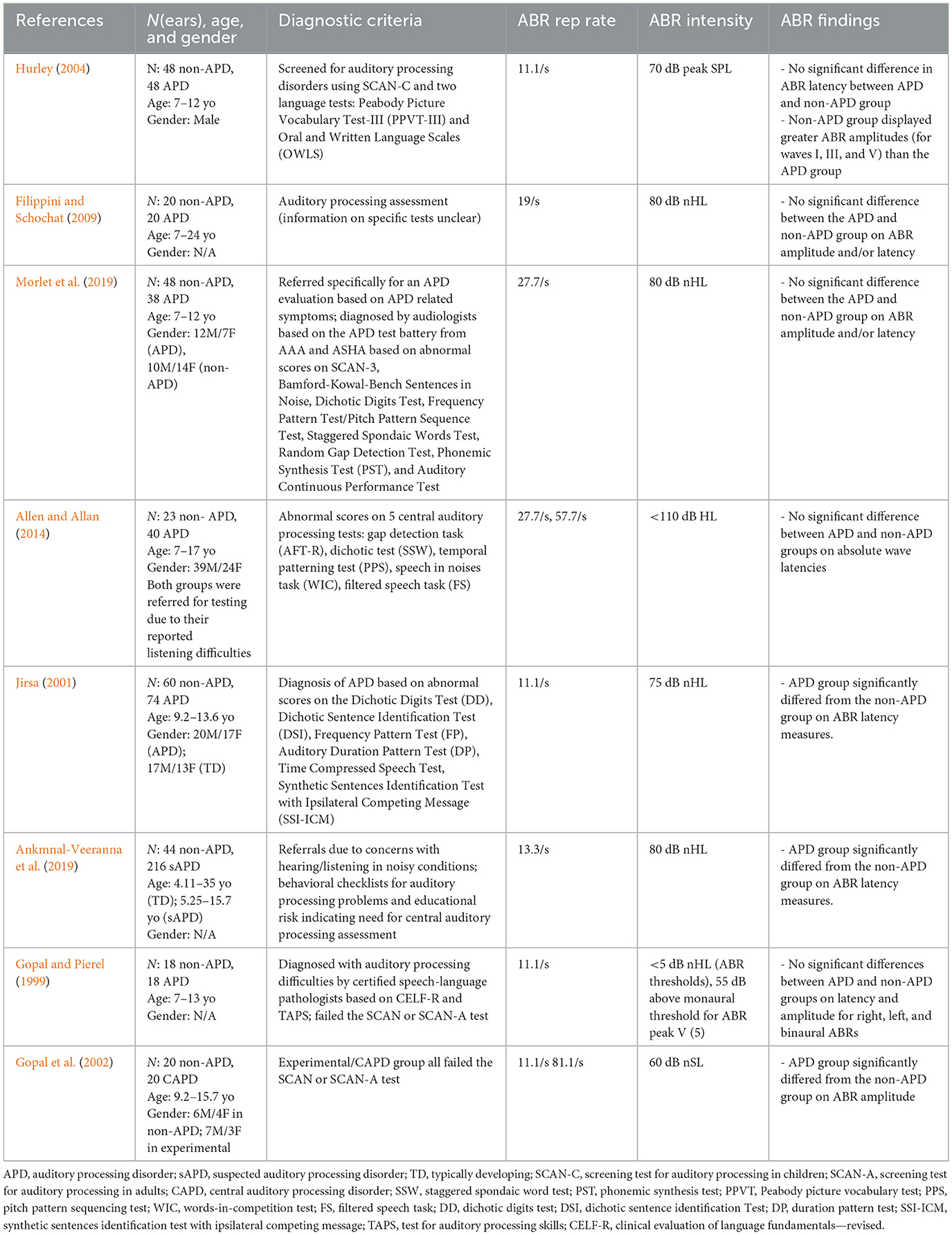
Table 1. Summary of studies included in the current meta-analysis review along with their characteristics.
Participants
Participants in the included studies were in the age range of 5–24 years.
Extraction of data
Data for the APD and non-APD groups were extracted from the text, tables, and/or graphs (using a pencil and a ruler; Beeson and Robey, 2006) in the published studies.
Calculation of effect size
The effect size (Cohen's d) was calculated via the following formula
where and denote the mean of the data for non-APD and APD groups, respectively; SD is the standard deviation, which was calculated using the following formula
where n1 refers to the number of ears and SDCtrl denotes the standard deviation for the non-APD group, while n2 refers to the number of ears and SDAPD denotes the standard deviation for the APD group. This formula was used specifically to control for the unequal number of ears in the APD vs. non-APD groups.
Effect sizes for these studies were plotted together using forest plots for comparison. Effect sizes derived from each study were weighted for the number of ears evaluated in each study (see e.g., Maggu et al., 2021). For example, if all studies combined constituted 100 ears that were tested, study A with 40 ears was weighted more than study B with 10 ears. Finally, weighted average effect sizes were calculated for all the studies, and these were plotted as diamond plots for an overall comparison. Both forest plots and diamond plots were plotted using R studio (RStudio Team, 2019).
Results
Study selection and characteristics
This pre-selection resulted in an initial 84 studies via title analysis; we then applied an additional four selection steps (Figure 1). In the first exclusion step, 31 studies that were based on other disorders (e.g., ADHD) and did not actually contain APD participants were excluded (e.g., Wible et al., 2005; Kwon et al., 2007; Ramezani et al., 2018). In the second exclusion step, 27 studies that did not contain original data (e.g., reviews, research commentaries) were excluded (e.g., Bamiou et al., 2001; Johnson et al., 2005; McFarland and Cacace, 2012) resulting in 26 studies where analysis of abstracts was conducted. In the third exclusion step, 9 studies were excluded due to an insufficient number of participants i.e., studies with less than three participants were excluded (e.g., Lenhardt, 1981; Jerger et al., 2002; Krishnamurti et al., 2013), resulting in 17 studies where critical analysis were conducted. In the fourth exclusion step, nine studies were excluded because they did not actually report any of ABR waves I, III, or V (e.g., Filippini et al., 2012; Rocha-Muniz et al., 2014; Wimalarathna et al., 2021), which were the variables of interest here. The inclusion and selection of the studies adhered to the reporting standards of PRISMA (Moher et al., 2009) and SCRIBE (Tate et al., 2016).
Table 1 provides a summary of the studies included in the current meta-analysis. Since we placed an emphasis on the comparison of APD vs. non-APD groups, including studies containing data for both groups (i.e., APD and non-APD) was a pre-requisite (inclusion step #3). The diagnostic criteria used in these studies ranged from administering checklists (Ankmnal-Veeranna et al., 2019) to administering standard APD tests (Hurley, 2004). In general, the stimulus presentation level for ABR measurements was >70 dB HL. Repetition rates varied across studies from 11.1/s (Jirsa, 2001) to 81.1/s (Gopal et al., 2002). Data collected with different repetition rates within a study were extracted as separate data points (e.g., Gopal et al., 2002; Allen and Allan, 2014). This variability across parameters was minimized by calculating the normalized effect size (Cohen's d) within each study using the formulae in Equations 1, 2.
Seven of the eight studies reported ABR latencies, while six studies reported ABR amplitudes as well. With respect to ABR latency, seven studies reported wave V latency, and six studies reported wave I and wave III latencies. With respect to ABR amplitude, six studies reported wave V, five studies reported wave I, and four studies reported Wave III amplitudes (Table 2).
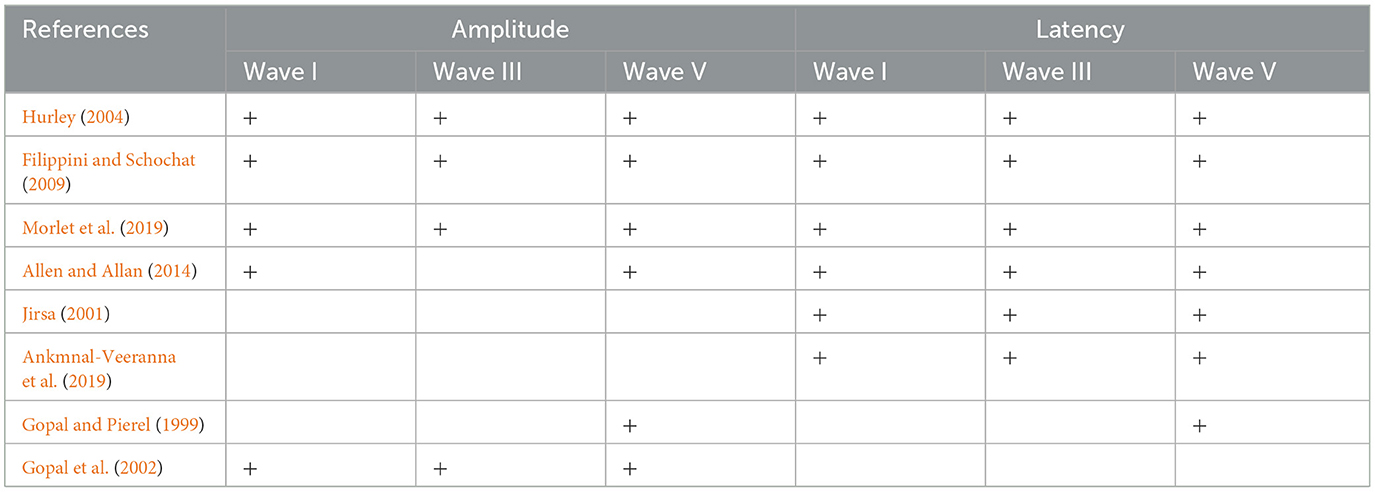
Table 2. Distribution of the included studies across the amplitude and latency measures of ABR waves I, III, and V.
Figures 2, 3 display forest plots of the effect sizes for ABR latency and ABR amplitude, respectively. Here, for ABR latency, a negative Cohen's d value indicates a longer latency in the APD group than in the non-APD group. For ABR amplitude, a positive Cohen's d value indicates a smaller wave amplitude in the APD group than in the non-APD group. Effect sizes whose 95% confidence intervals do not cross zero are considered statistically significant at the α = 5% level (Cochrane, 2020).
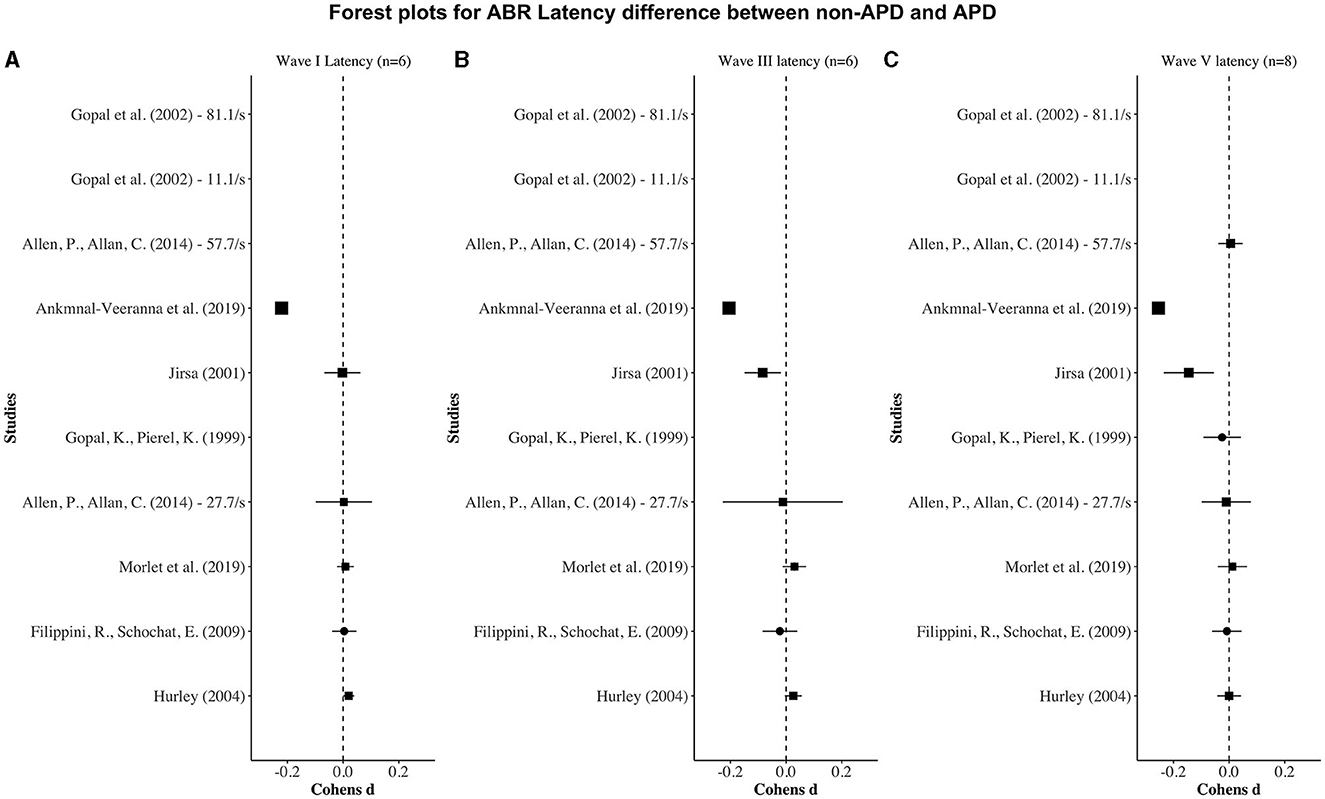
Figure 2. Forest plots depicting the Cohen's d (x-axis) latency difference between the APD and non-APD groups for ABR waves I (A), III (B), and V (C) in each study (y-axis). The width of the solid squares reflects the weight contributed by the respective study toward the overall effect size, with extending horizontal lines denoting 95% confidence intervals.
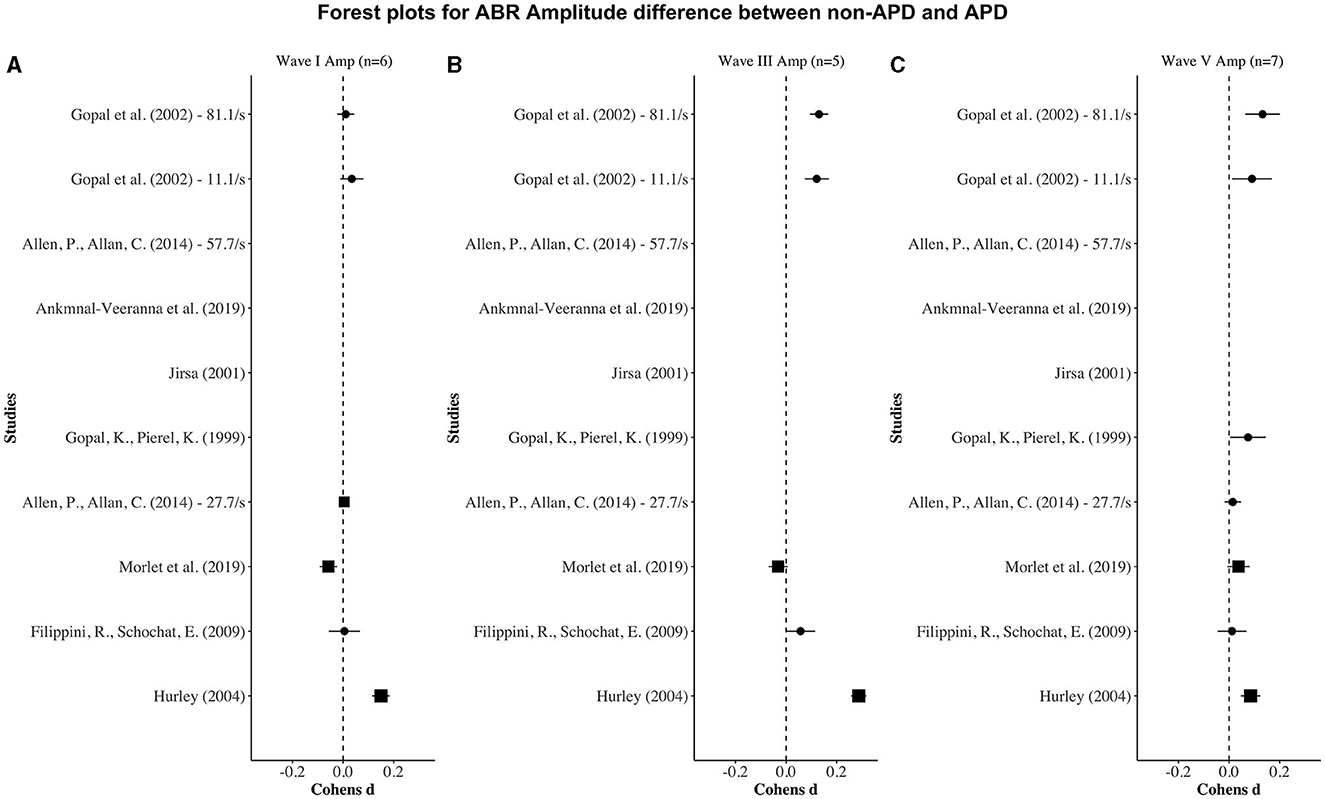
Figure 3. Forest plots depicting the Cohen's d (x-axis) peak amplitude difference between the APD and non-APD groups for ABR waves I (A), III (B), and V (C) in each study (y-axis). The width of the solid squares reflects the weight contributed by the respective study toward the overall effect size, with extending horizontal lines denoting 95% confidence intervals.
ABR latency
For wave I latency, one study (i.e., Ankmnal-Veeranna et al., 2019) revealed a significantly longer ABR wave I latency in the APD group than the non-APD group. For wave III and V latencies, two studies (i.e., Jirsa, 2001; Ankmnal-Veeranna et al., 2019) revealed significantly longer ABR wave III and V latencies in the APD group than in the non-APD group.
ABR amplitude
For wave I amplitude, one study (i.e., Hurley, 2004) revealed a significantly smaller APD wave I amplitude in the APD group than in the non-APD group. For wave III and wave V amplitudes, three studies (i.e., Gopal and Pierel, 1999; Gopal et al., 2002; Hurley, 2004) revealed significantly smaller APD wave III and wave V amplitudes in the APD group than in the non-APD group.
Finally, we examined the average effects across studies. Overall, while there were trends toward longer latencies and smaller amplitudes in the APD group, we found no dissimilarity between the APD and non-APD groups with respect to the latency (Figure 4A) and amplitude (Figure 4B) measures for ABR waves I, III, and V.
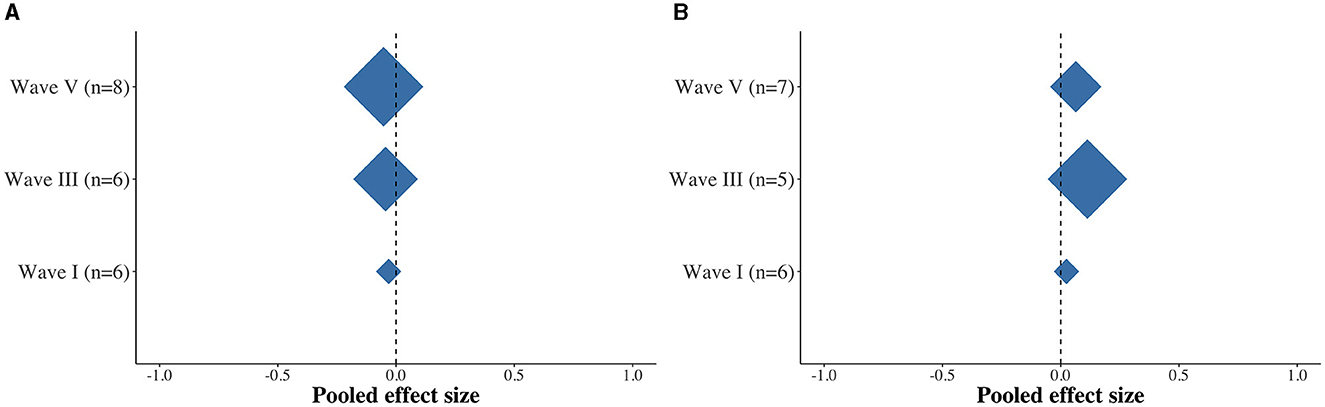
Figure 4. Diamond plots depicting the pooled effect size differences between the APD and non-APD groups for latency (A) and amplitude (B) for ABR waves I, III, and V.
Discussion
The current meta-analysis investigated whether individuals with APD exhibit deficits in basic sensory auditory neural processes (elicited from the neural centers between auditory nerve and inferior colliculi), as assessed with click-evoked ABR. Across the studies reviewed here, we found no statistically significant difference between APD and non-APD individuals with respect to the peak amplitude and latency measures of the three main ABR waves (i.e., I, III, and V), which are key indicators of neural processing in the early stages of auditory processing. Overall, despite the recent emphasis on the use of ABR for detecting APD in individuals (Ankmnal-Veeranna et al., 2019), the current findings suggest that APD is not significantly implicated with deficits in basic sensory auditory processes, at least with respect to traditional non-speech ABR stimuli (i.e., clicks). Overall, the findings from the current meta-analysis are consistent with the findings of Filippini and Schochat (2009), Allen and Allan (2014), and Morlet et al. (2019) in terms of ABR peak amplitudes and latencies in those with APD.
These findings could be explained by a variety of factors, such as:
(1) ABR metrics: the current meta-analysis analyzed the metrics that were most commonly available across the included studies, i.e., amplitude and latency of ABR waves I, III, and V. Even though these three waves have historically been considered as major landmarks of traditional ABR (Xie et al., 2018), there are additional potentially informative metrics, such as the interpeak latency difference (e.g., III:I, V:I, V:III), amplitude ratios (e.g., V/I amplitude ratio), and the slope of the peak, each of which play key roles in the identification and differential diagnosis of the loci of lesions or processing deficits (Montaguti et al., 2007; Sato et al., 2009; McCullagh et al., 2020). Future studies should therefore routinely report these additional metrics to enable a more comprehensive understanding of the putative brainstem contributions toward APD.
(2) APD diagnostic criteria: In most of the studies included in the current meta-analysis, individuals were diagnosed with APD based on their reduced performance on a behavioral APD test battery. However, diagnosing APD on the basis of behavioral tests remains problematic because this might additionally recruit higher-order confounding factors such as language, memory, and attention (Dawes and Bishop, 2009, 2010; Sharma et al., 2009; Miller and Wagstaff, 2011; de Wit et al., 2016, 2018). As a result, the existing behavioral tests and criteria for an APD diagnosis might be unable to capture the deficits that are specific to the auditory domain (Moore, 2018; Vermiglio, 2018; Ankmnal-Veeranna et al., 2019; Maggu and Overath, 2021). Put differently, the current null result (no significant difference in the ABR measures between individuals with and without APD) does not preclude the possibility that there exist basic auditory processing differences between non-APD and APD individuals.
(3) Stimuli: As stated above, the majority of the previous APD studies using ABRs used clicks (or tonebursts) as stimuli. It is possible that these stimuli are simply not sensitive or complex enough for revealing deficits in the auditory brainstem as they pertain to APD. For example, clicks are known to stimulate the basal region (i.e., higher frequencies) of the basilar membrane more than the apical region (Neely et al., 1988; Dau et al., 2000), while tonebursts excite only a narrow region on the basilar membrane that is sensitive to their particular frequency (e.g., 1,000 Hz). In contrast, a more holistic stimulus such as the “optimized chirp” (Dau et al., 2000), which stimulates both basal and apical regions of the cochlear partition and thereby more evenly captures responses from both low and high frequencies, could be employed to more comprehensively study the ABR. Similarly, frequency following responses elicited with brief speech stimuli (e.g., 40 ms/da/) (Skoe and Kraus, 2010) could potentially be useful in understanding APD-related deficits in auditory processing at the level of the subcortical auditory system.
In addition to the above-mentioned general factors, it is worth noting that with respect to peak latency, one study stood out (Ankmnal-Veeranna et al., 2019), in that it reported large differences between the APD and non-APD groups for wave I, III, and V latencies. Compared to the other studies in this meta-analysis, the study by Ankmnal-Veeranna et al. (2019) differs from the others in at least two respects. First, their study had a larger sample size (n = 216 for APD, n = 44 for non-APD) compared to the other studies (whose sample sizes ranged from 20 to 60 subjects in each group). Second, the participants that were included in their APD group were not actually formally diagnosed using the currently accepted behavioral APD test battery (American Speech-Language-Hearing Association, 2005b); instead, they were included simply based on referrals by physicians, teachers, community audiologists, family friends, or parents for their concern regarding the children's hearing abilities. If these children exhibited reduced performance on the screening checklists for auditory processing problems and educational risk [Children Auditory Performance Scale (Smoski et al., 1998) and Screening Identification for Targeting Educational Risk (Anderson, 1989)], Ankmnal-Veeranna et al. (2019) included them in the APD group. As a result, they referred to their participants as suspected-APD or sAPD. In comparison, it is noteworthy that the other studies (which used behavioral APD tests to diagnose APD) only showed a small to negligible effect on ABR peak latencies. For example, the participants in both APD and non-APD groups in the study by Allen and Allan (2014), were reported to have listening difficulties but were classified as APD vs. non-APD based on their performance on behavioral tests and were found to not significantly differ on the ABR measures.
The lack of significant ABR differences between individuals with vs. without APD can be interpreted in (at least) two ways. One possibility is that APD is indeed not associated with basic sensory auditory processing deficits, but only arises from later, central auditory processing deficits. Second, differences in basic auditory processes between individuals with and without APD do indeed exist, but the standard simple ABR measures alone are not sensitive enough to capture them. For example, deficits in the auditory brainstem at the level of the superior olivary colliculi can be detected by evaluating binaural listening. However, given the paucity of studies investigating binaural ABRs in APD [e.g., binaural interaction component (BIC)], at the moment it is difficult to understand the effects of APD on binaural ABRs.
In order to better understand these possibilities, we suggest targeting the early auditory system with a battery of objective (physiological and electrophysiological) tests—rather than purely behavioral tests—that may prove instrumental in identifying the deficits associated with APD in the early auditory nervous system (Maggu and Overath, 2021), particularly those children who exhibit challenges in their listening abilities (e.g., speech perception in noise). By employing such an objective test battery, APD testing will adhere to the standpoint of American Speech-Language-Hearing Association, 2005a,b) with respect to the domain specificity of APD to the “auditory domain” and the auditory neural origins of APD. We suggest an objective test battery that contains both tests that evaluate for deficits in the peripheral auditory nervous system (also known as site-based tests), as well as tests that evaluate for deficits in the basic auditory “processes” such as speech-in-noise, frequency discrimination, localization, and binaural interaction (also known as process-based tests). We propose that an APD diagnosis is only warranted if performance is poor on the objective test battery (Maggu and Overath, 2021).
Limitations
One of the chief limitations of the current study is the heterogeneity across the selected studies. More specifically, the included studies differed from each other in terms of intensity levels, repetition rates, and behavioral diagnostic criteria. Another key limitation of the current study could be the relatively smaller sample of the included studies (i.e., n = 8). We believe that this is mainly due to the paucity of studies that have been conducted in this direction comparing the APD and non-APD groups across the click-ABR measures. Although we tried to include as many studies as possible in the current meta-analysis, as a result of the limited sample size, the current meta-analysis could not perform statistical analysis pertaining to selection bias.
Future directions
The current meta-analysis provides a starting point toward understanding the basic sensory auditory processing in individuals with APD. We found no significant difference between those with and without APD on click-evoked ABR waves I, III, and V latencies and amplitudes. However, there is a need for future studies to revisit this research question and strengthen the volume of research to achieve a more comprehensive and conclusive understanding. In addition to expanding the range of click-evoked ABR metrics (peak amplitude and latency, interpeak amplitude and latency, amplitude ratio, and wave slope), BIC, and ABR stimuli (e.g., optimized chirp, 40 ms/da/), future studies should also consider investigating other neural aspects of basic auditory processing (e.g., efferent system).
Data availability statement
The raw data supporting the conclusions of this article will be made available by the authors, without undue reservation.
Author contributions
AM: Conceptualization, Formal analysis, Methodology, Visualization, Writing – original draft, Writing – review & editing. YY: Data curation, Formal analysis, Writing – original draft, Writing – review & editing. TO: Conceptualization, Methodology, Validation, Writing – original draft, Writing – review & editing.
Funding
The author(s) declare that no financial support was received for the research, authorship, and/or publication of this article.
Conflict of interest
The authors declare that the research was conducted in the absence of any commercial or financial relationships that could be construed as a potential conflict of interest.
Publisher's note
All claims expressed in this article are solely those of the authors and do not necessarily represent those of their affiliated organizations, or those of the publisher, the editors and the reviewers. Any product that may be evaluated in this article, or claim that may be made by its manufacturer, is not guaranteed or endorsed by the publisher.
Supplementary material
The Supplementary Material for this article can be found online at: https://www.frontiersin.org/articles/10.3389/fauot.2024.1369716/full#supplementary-material
References
Achor, L. J., and Starr, A. (1980). Auditory brain stem responses in the cat. II. Effects of lesions. Electroencephal. Clini. Neurophysiol. 48, 174–190. doi: 10.1016/0013-4694(80)90302-8
Allen, P., and Allan, C. (2014). Auditory processing disorders: relationship to cognitive processes and underlying auditory neural integrity. Int. J. Pediatr. Otorhinolaryngol. 78, 198–208. doi: 10.1016/j.ijporl.2013.10.048
American Speech-Language-Hearing Association (2005a). (Central) Auditory Processing Disorders-The Role of the Audiologist. Available at: https://www.asha.org/policy/ps2005-00114/
American Speech-Language-Hearing Association (2005b). Central Auditory Processing Disorder. Available at: https://www.asha.org/practiceportal/clinical-topics/centralauditory-processing-disorder/#collapse_8
Anderson, K. L. (1989). SIFTER: Screening Instrument For Targeting Educational Risk in Children Identified by Hearing Screening or Who Have Known Hearing Loss: User's Manual. Johnson City: Interstate Printers & Publishers.
Ankmnal-Veeranna, S., Allan, C., and Allen, P. (2019). Auditory brainstem responses in children with auditory processing disorder. J. Am. Acad. Audiol. 30, 904–917. doi: 10.3766/jaaa.18046
Apeksha, K., and Kumar, A. U. (2017). Speech perception in quiet and in noise condition in individuals with auditory neuropathy spectrum disorder. J. Int. Adv. Otol. 13, 83–87. doi: 10.5152/iao.2017.3172
Bamiou, D.-E., Musiek, F. E., and Luxon, L. M. (2001). Aetiology and clinical presentations of auditory processing disorders—a review. Arch. Dis. Child. 85, 361–365. doi: 10.1136/adc.85.5.361
Bansal, M. (2018). Diseases of Ear, Nose & Throat: With Head & Neck Surgery. New York: JP Medical Ltd.
Bauch, C. D., Rose, D. E., and Harner, S. G. (1982). Auditory brain stem response results from 255 patients with suspected retrocochlear involvement. Ear Hear. 3, 83–86. doi: 10.1097/00003446-198203000-00006
Beeson, P. M., and Robey, R. R. (2006). Evaluating single-subject treatment research: Lessons learned from the aphasia literature. Neuropsychol. Rev. 16, 161–169. doi: 10.1007/s11065-006-9013-7
Cacace, A. T., and McFarland, D. J. (2013). Factors influencing tests of auditory processing: a perspective on current issues and relevant concerns. J. Am. Acad. Audiol. 24, 572–589. doi: 10.3766/jaaa.24.7.6
Chermak, G. D., and Musiek, F. E. (1997). Central Auditory Processing Disorders: New Perspectives. San Diego: Singular Pub. Group.
Cochrane (2020). How to Read a Forest Plot? Available at: https://uk.cochrane.org/news/how-read-forest-plot (accessed June 10, 2021).
Cone-Wesson, B. (2004). “Repetition Rate and Auditory Neuropathy Barbara Cone-Wesson,” in AudiologyOnline. Available at: https://www.audiologyonline.com/ask-the-experts/repetition-rate-and-auditory-neuropathy-546 (accessed July 3, 2022).
Dau, T., Wegner, O., Mellert, V., and Kollmeier, B. (2000). Auditory brainstem responses with optimized chirp signals compensating basilar-membrane dispersion. J. Acoust. Soc. Am. 107, 1530–1540. doi: 10.1121/1.428438
Davis, A. E., and Beagley, H. A. (1985). Acoustic brainstem responses for clinical use: the effect of attention. Clin. Otolaryngol. Allied Sci. 10, 311–314. doi: 10.1111/j.1365-2273.1985.tb00262.x
Davis, H. (1964). Enhancement of evoked cortical potentials in humans related to a task requiring a decision. Science 145, 182–183. doi: 10.1126/science.145.3628.182
Dawes, P., and Bishop, D. (2009). Auditory processing disorder in relation to developmental disorders of language, communication and attention: a review and critique. Int. J. Lang. Commun. Disord. 44, 440–465. doi: 10.1080/13682820902929073
Dawes, P., and Bishop, D. V. (2010). Psychometric profile of children with auditory processing disorder and children with dyslexia. Arch. Dis. Child. 95, 432–436. doi: 10.1136/adc.2009.170118
de Carvalho, N. G., Novelli, C. V. L., and Colella-Santos, M. F. (2017). Evaluation of speech in noise abilities in school children. Int. J. Pediatr. Otorhinolaryngol. 99, 66–72. doi: 10.1016/j.ijporl.2017.05.019
de Wit, E., van Dijk, P., Hanekamp, S., Visser-Bochane, M. I., Steenbergen, B., van der Schans, C. P., et al. (2018). Same or different: the overlap between children with auditory processing disorders and children with other developmental disorders: a systematic review. Ear Hear. 39, 1–19. doi: 10.1097/AUD.0000000000000479
de Wit, E., Visser-Bochane Margot, I., Steenbergen, B., van Dijk, P., van der Schans Cees, P., and Luinge Margreet, R. (2016). Characteristics of auditory processing disorders: a systematic review. J. Speech, Lang. Hear. Res. 59, 384–413. doi: 10.1044/2015_JSLHR-H-15-0118
Del Zoppo, C., Sanchez, L., and Lind, C. (2015). A long-term follow-up of children and adolescents referred for assessment of auditory processing disorder. Int. J. Audiol. 54, 368–375. doi: 10.3109/14992027.2014.972523
Elsayed, A. M., Hunter, L. L., Keefe, D. H., Feeney, M. P., Brown, D. K., Meinzen-Derr, J. K., et al. (2015). Air and bone conduction click and tone-burst auditory brainstem thresholds using Kalman adaptive processing in non-sedated normal hearing infants. Ear Hear. 36, 471–481. doi: 10.1097/AUD.0000000000000155
Esplin, J., and Wright, C. (2014). Auditory Processing Disorder: New Zealand Review. Wellington: Sapere Research Group Wellington, New Zealand. Available at: https://www.health.govt.nz/system/files/documents/publications/auditory_processing_disorder_review_report.docx (accessed July 3, 2022).
Filippini, R., Befi-Lopes, D. M., and Schochat, E. (2012). Efficacy of auditory training using the auditory brainstem response to complex sounds: auditory processing disorder and specific language impairment. Folia Phoniatrica et Logopaedica 64, 217–226. doi: 10.1159/000342139
Filippini, R., and Schochat, E. (2009). Brainstem evoked auditory potentials with speech stimulus in the auditory processing disorder. Braz. J. Otorhinolaryngol. 75, 449–455. doi: 10.1016/S1808-8694(15)30665-0
Glasscock, M. E., Jackson, C. G., Josey, A. F., Dickins, J. R., and Wiet, R. J. (1979). Brain stem evoked response audiometry in a clinical practice. Laryngoscope 89, 1021–1035. doi: 10.1288/00005537-197907000-00001
Gopal, K. V., Daily, C. S., and Kao, K. (2002). Auditory brainstem responses to regular and high stimulus repetition rates in children at risk for central auditory processing disorders. J. Audiol. Med. 11, 146–160.
Gopal, K. V., and Pierel, K. (1999). Binaural interaction component in children at risk for central auditory processing disorders. Scand. Audiol. 28, 77–84. doi: 10.1080/010503999424798
Gross, M. M., Begleiter, H., Tobin, M., and Kissin, B. (1965). Auditory evoked response comparison during counting clicks and reading. Electroencephalogr. Clin. Neurophysiol. 18, 451–454. doi: 10.1016/0013-4694(65)90125-2
Hood, L. J. (1998). Clinical Applications of the Auditory Brainstem Response. St, San Diego: Singular Publishing Group.
Hurley, A. (2004). Behavioral and Electrophysiological Assessment of Children with a Specific Temporal Processing Disorder (LSU Doctoral Dissertation). Available at: https://digitalcommons.lsu.edu/gradschool_dissertations/2323 (accessed July 3, 2022).
Jerger, J., and Musiek, F. (2000). Report of the consensus conference on the diagnosis of auditory processing disorders in school-aged children. J. Am. Acad. Audiol. 11, 467–474. doi: 10.1055/s-0042-1748136
Jerger, J., Thibodeau, L., Martin, J., Mehta, J., Tillman, G., Greenwald, R., et al. (2002). Behavioral and electrophysiologic evidence of auditory processing disorder: a twin study. J. Am. Acad. Audiol. 13, 438–460. doi: 10.1055/s-0040-1716007
Jewett, D. L., and Williston, J. S. (1971). Auditory-evoked far fields averaged from the scalp of humans. Brain 94, 681–696. doi: 10.1093/brain/94.4.681
Jirsa, R. E. (2001). Maximum length sequences-auditory brainstem responses from children with auditory processing disorders. J. Am. Acad. Audiol. 12, 155–164. doi: 10.1055/s-0042-1745592
Johnson, K. L., Nicol, T. G., and Kraus, N. (2005). Brain stem response to speech: a biological marker of auditory processing. Ear Hear. 26, 424–434. doi: 10.1097/01.aud.0000179687.71662.6e
Kojima, K., Lin, L., Petley, L., Clevenger, N., Perdew, A., Bodik, M., et al. (2024). Childhood listening and associated cognitive difficulties persist into adolescence. Ear Hear. doi: 10.1097/AUD.0000000000001517
Krishnamurti, S., Forrester, J., Rutledge, C., and Holmes, G. W. (2013). A case study of the changes in the speech-evoked auditory brainstem response associated with auditory training in children with auditory processing disorders. Int. J. Pediatr. Otorhinolaryngol. 77, 594–604. doi: 10.1016/j.ijporl.2012.12.032
Krishnan, A., Gandour, J. T., Ananthakrishnan, S., and Vijayaraghavan, V. (2014a). Cortical pitch response components index stimulus onset/offset and dynamic features of pitch contours. Neuropsychologia 59, 1–12. doi: 10.1016/j.neuropsychologia.2014.04.006
Krishnan, A., Gandour, J. T., and Suresh, C. H. (2014b). Cortical pitch response components show differential sensitivity to native and nonnative pitch contours. Brain Lang. 138, 51–60. doi: 10.1016/j.bandl.2014.09.005
Kujawa, S. G., and Liberman, M. C. (2009). Adding insult to injury: cochlear nerve degeneration after “temporary” noise-induced hearing loss. J. Neurosci. 29, 14077–14085. doi: 10.1523/JNEUROSCI.2845-09.2009
Kwon, S., Kim, J., Choe, B.-H., Ko, C., and Park, S. (2007). Electrophysiologic Assessment of Central Auditory Processing by Auditory Brainstem Responses in Children with Autism Spectrum Disorders. Available at: https://synapse.koreamed.org/articles/1020478 (accessed July 9, 2022).
Lenhardt, M. L. (1981). Childhood central auditory processing disorder with brainstem evoked response verification. Arch. Otolaryngol. 107, 623–625. doi: 10.1001/archotol.1981.00790460035011
Maggu, A. R., Kager, R., To, C. K. S., Kwan, J. S. K., and Wong, P. C. M. (2021). Effect of complexity on speech sound development: evidence from meta-analysis review of treatment-based studies. Front. Psychol. 12:651900. doi: 10.3389/fpsyg.2021.651900
Maggu, A. R., and Overath, T. (2021). An objective approach towards understanding Auditory Processing Disorder. Am. J. Audiol. 30, 790–795. doi: 10.1044/2021_AJA-21-00007
Magimairaj, B. M., Nagaraj, N. K., Champlin, C. A., Thibodeau, L. K., Loeb, D. F., and Gillam, R. B. (2021). Speech perception in noise predicts oral narrative comprehension in children with developmental language disorder. Front. Psychol. 12:735026. doi: 10.3389/fpsyg.2021.735026
Magimairaj, B. M., Nagaraj, N. K., Sergeev, A. V., and Benafield, N. J. (2020). Comparison of auditory, language, memory, and attention abilities in children with and without listening difficulties. Am. J. Audiol. 29, 710–727. doi: 10.1044/2020_AJA-20-00018
Masterton, R. B., and Imig, T. J. (1984). Neural mechanisms for sound localization. Annu. Rev. Physiol. 46, 275–287. doi: 10.1146/annurev.ph.46.030184.001423
McCullagh, E. A., Rotschafer, S. E., Auerbach, B. D., Klug, A., Kaczmarek, L. K., Cramer, K. S., et al. (2020). Mechanisms underlying auditory processing deficits in Fragile X syndrome. FASEB J. 34, 3501–3518. doi: 10.1096/fj.201902435R
McFarland, D. J., and Cacace, A. T. (2012). Questionable reliability of the speech-evoked auditory brainstem response (sABR) in typically-developing children. Hear. Res. 287, 1–2. doi: 10.1016/j.heares.2012.02.014
Melcher, J. R., Guinan, J. J., Knudson, I. M., and Kiang, N. Y. (1996a). Generators of the brainstem auditory evoked potential in cat. II. Correlating lesion sites with waveform changes. Hear. Res. 93, 28–51. doi: 10.1016/0378-5955(95)00179-4
Melcher, J. R., Knudson, I. M., Fullerton, B. C., Guinan, J. J., Norris, B. E., and Kiang, N. Y. (1996b). Generators of the brainstem auditory evoked potential in cat. I. An experimental approach to their identification. Hear. Res. 93, 1–27. doi: 10.1016/0378-5955(95)00178-6
Miller, C. A., and Wagstaff, D. A. (2011). Behavioral profiles associated with auditory processing disorder and specific language impairment. J. Commun. Disord. 44, 745–763. doi: 10.1016/j.jcomdis.2011.04.001
Moher, D., Liberati, A., Tetzlaff, J., Altman, D. G., and PRISMA Group (2009). Preferred reporting items for systematic reviews and meta-analyses: the PRISMA statementthe PRISMA statement. Ann. Intern. Med. 151, 264–269. doi: 10.7326/0003-4819-151-4-200908180-00135
Møller, A. R., and Jannetta, P. J. (1985). “Neural generators of the auditory brainstem response,” in The Auditory Brainstem Response (San Diego, CA: College Hill Press), 13–31.
Montaguti, M., Bergonzoni, C., Zanetti, M., and Rinaldi Ceroni, A. (2007). Comparative evaluation of ABR abnormalities in patients with and without neurinoma of VIII cranial nerve. Acta Otorhinolaryngologica Italica 27, 68–72.
Moore, D. R. (2018). Auditory processing disorder (APD). Ear Hear. 39, 617–620. doi: 10.1097/AUD.0000000000000582
Morlet, T., Nagao, K., Greenwood, L. A., Cardinale, R. M., Gaffney, R. G., and Riegner, T. (2019). Auditory event-related potentials and function of the medial olivocochlear efferent system in children with auditory processing disorders. Int. J. Audiol. 58, 213–223. doi: 10.1080/14992027.2018.1551632
Musiek, F. E., and Geurkink, N. A. (1982). Auditory brain stem response and central auditory test findings for patients with brain stem lesions: a preliminary report. Laryngoscope 92, 891–900. doi: 10.1288/00005537-198208000-00010
Neely, S. T., Norton, S. J., Gorga, M. P., and Jesteadt, W. (1988). Latency of auditory brain-stem responses and otoacoustic emissions using tone-burst stimuli. J. Acoust. Soc. Am. 83, 652–656. doi: 10.1121/1.396542
Picton, T. W., and Hillyard, S. A. (1974). Human auditory evoked potentials. II: effects of attention. Electroencephalogr. Clin. Neurophysiol. 36, 191–200. doi: 10.1016/0013-4694(74)90156-4
Picton, T. W., Stapells, D. R., and Campbell, K. B. (1981). Auditory evoked potentials from the human cochlea and brainstem. J. Otolaryngol. Supplement 9, 1–41.
Ramezani, M., Lotfi, Y., Moossavi, A., and Bakhshi, E. (2018). Auditory Brainstem Response to Speech in Children with High Functional Autism Spectrum Disorder. Available at: https://link.springer.com/article/10.1007/s10072-018-3594-9 (accessed July 9, 2022).
Rhee, C.-K., Park, H.-M., and Jang, Y.-J. (1999). Audiologic evaluation of neonates with severe hyperbilirubinemia using transiently evoked otoacoustic emissions and auditory brainstem responses. Laryngoscope 109, 2005–2008. doi: 10.1097/00005537-199912000-00021
Rocha-Muniz, C. N., Befi-Lopes, D. M., and Schochat, E. (2014). Sensitivity, specificity and efficiency of speech-evoked ABR. Hear. Res. 317, 15–22. doi: 10.1016/j.heares.2014.09.004
RStudio Team (2019). RStudio: Integrated Development for R [Computer software]. Boston: R Studio Inc. http://www.rstudio.com/ (accessed June 15, 2024).
Sato, S., Yamada, M., Koizumi, H., Onozawa, Y., Shimokawa, N., Kawashima, E., et al. (2009). Neurophysiological mechanisms of conduction impairment of the auditory nerve during cerebellopontine angle surgery. Clini. Neurophysiol 120, 329–335. doi: 10.1016/j.clinph.2008.11.005
Satterfield, J. H. (1965). Evoked cortical response enhancement and attention in man. A study of responses to auditory and shock stimuli. Electroencephal. Clini. Neurophysiol. 19, 470–475. doi: 10.1016/0013-4694(65)90185-9
Schow, R. L., Whitaker, M. M., Seikel, J. A., Brockett, J. E., and Domitz, V. D. M. (2020). Validity of the multiple auditory processing assessment−2: a test of auditory processing disorder. Lang. Speech Hear. Serv. Sch. 51, 993–1006. doi: 10.1044/2020_LSHSS-20-00001
Sharma, M., Purdy, S. C., and Kelly, A. S. (2009). Comorbidity of auditory processing, language, and reading disorders. J. Speech, Lang. Hear Res. 52, 706–722. doi: 10.1044/1092-4388(2008/07-0226)
Skoe, E., and Kraus, N. (2010). Auditory brainstem response to complex sounds: A tutorial. Ear Hear. 31, 302–324. doi: 10.1097/AUD.0b013e3181cdb272
Smoski, W., Brunt, M. A., and Tannahill, J. C. (1998). Children's auditory performance scale (CHAPS). Tampa, FL: Educational Audiology AssocIation.
Stapells, D. R. (2000). Threshold estimation by the tone-evoked auditory brainstem response: a literature meta-analysis. J. Speech Lang. Pathol. Audiol. 24, 74–83.
Starr, A. (1976). Correlation between confirmed sites of neurological lesions and abnormalities of far-field auditory brainstem responses. Electroencephalogr. Clin. Neurophysiol. 41, 595–608. doi: 10.1016/0013-4694(76)90005-5
Tanaka, H., Komatsuzaki, A., and Hentona, H. (1996). Usefulness of auditory brainstem responses at high stimulus rates in the diagnosis of acoustic neuroma. ORL 58, 224–228. doi: 10.1159/000276841
Tate, R. L., Perdices, M., Rosenkoetter, U., Shadish, W., Vohra, S., Barlow, D. H., et al. (2016). The single-case reporting guideline in behavioural interventions (SCRIBE) 2016 statement. J. Clin. Epidemiol. 73, 142–152. doi: 10.1016/j.jclinepi.2016.04.006
Vermiglio, A. (2018). The gold standard and auditory processing disorder. Persp. ASHA Special Inter. Groups 3, 6–17. doi: 10.1044/persp3.SIG6.6
Wible, B., Nicol, T., and Kraus, N. (2005). Correlation between brainstem and cortical auditory processes in normal and language-impaired children. Brain 128, 417–423. doi: 10.1093/brain/awh367
Wimalarathna, H., Ankmnal-Veeranna, S., Allan, C., Agrawal, S., Allen, P., Samarabandu, J., et al. (2021). Comparison of machine learning models to classify Auditory Brainstem Responses recorded from children with Auditory Processing Disorder. Comput. Methods Programs Biomed. 200:105942. doi: 10.1016/j.cmpb.2021.105942
Xie, L., Wang, M., Liao, T., Tan, S., Sun, K., Li, H., et al. (2018). The characterization of auditory brainstem response (ABR) waveforms: a study in tree shrews (Tupaia belangeri). J. Otol. 13, 85–91. doi: 10.1016/j.joto.2018.05.004
Young, A., Cornejo, J., and Spinner, A. (2021). Auditory brainstem response. In StatPearls. St. Petersburg: StatPearls Publishing. Available at: http://www.ncbi.nlm.nih.gov/books/NBK564321/ (accessed June 15, 2024).
Keywords: click-evoked ABR, Auditory Processing Disorder (APD), APD diagnosis, electrophysiology, auditory brainstem
Citation: Maggu AR, Yu Y and Overath T (2024) The click-evoked auditory brainstem response is not affected in auditory processing disorder: a meta-analysis systematic review. Front. Audiol. Otol. 2:1369716. doi: 10.3389/fauot.2024.1369716
Received: 12 January 2024; Accepted: 30 July 2024;
Published: 19 August 2024.
Edited by:
Joseph P. Walton, University of South Florida, United StatesReviewed by:
Eliane Schochat, University of São Paulo, BrazilXiaopeng Liu, University of Illinois at Urbana-Champaign, United States
Michelle Arnold, University of South Florida, United States
Copyright © 2024 Maggu, Yu and Overath. This is an open-access article distributed under the terms of the Creative Commons Attribution License (CC BY). The use, distribution or reproduction in other forums is permitted, provided the original author(s) and the copyright owner(s) are credited and that the original publication in this journal is cited, in accordance with accepted academic practice. No use, distribution or reproduction is permitted which does not comply with these terms.
*Correspondence: Akshay R. Maggu, YWtzaGF5Lm1hZ2d1JiN4MDAwNDA7dWNvbm4uZWR1