- College of Sciences and Engineering, Tasmanian Institute of Agriculture, University of Tasmania, Hobart, TAS, Australia
A better understanding of factors that influence learning of cattle with respect to new virtual fencing technology is required to inform the development of best practice training protocols and guide the introduction of the technology to naïve dairy cattle. This experiment examined the effect of age on (1) the efficiency of associative pairing of audio and electrical stimuli in dairy heifers and (2) the retention of this associative pairing over a long period of time without use. Fifty-nine Holstein dairy heifers were used in feed attractant trials where audio cues and electrical stimuli were delivered through manually controlled training collars. Heifers were allocated to four treatments that differed in the age at which naïve animals underwent training; these were 6-months (“6M”; n = 15), 9-months (“9M”; n = 15), 12-months (“12M”; n = 15), or 22-months of age (“22M”; n = 14). Animals in the 6, 9, and 12M treatments underwent a second round of training at 22-months of age (i.e., at the same time as naïve 22M heifers). Heifers received an audio stimulus (2 s; 84 dB) when they breached a virtual fence after which a short electrical stimulus (0.5 s; 3 V, 120 mW) was administered if they continued to move forward. If the animal stopped moving forward no further stimuli were applied. There were no effects of age treatment on the total number of interactions with the virtual fence (P > 0.05). During initial training, 22M heifers received a lower proportion of electrical stimuli (i.e., responded to audio without requiring the electrical stimulus; P < 0.001) and more frequently stopped walking (P = 0.01) and turned back (P = 0.008) following administration of the audio cue compared to younger heifers. Previous training at an early age did not improve the responsiveness of heifers to virtual fencing when re-trained at 22-months of age (P > 0.05). We conclude that dairy heifers should be trained to virtual fencing technology close to calving age rather than earlier in their ontogeny and that stock be re-trained following an extended period without virtual fencing technology.
Introduction
Virtual fencing is an emerging technology that has the potential to reduce labor and fencing costs and facilitate more intense or complex grazing regimes in pasture-based dairy systems. Animals are trained to associate an audio cue that is delivered via a neckband mounted device as they approach a boundary set via global positioning system with a pending electrical stimulus, unless they stop moving toward the virtual boundary (e.g., Campbell et al., 2019; Lomax et al., 2019). Acute stress is expected when animals are undergoing this type of avoidance learning, but this learning is essential to successful operation of virtual fencing technology (Lee et al., 2018). The stress response should be minimal once animals have learnt to avoid the electrical stimulus which restores predictability and controllability to their environment (Lee et al., 2018). Efficient and rapid learning of the association between audio and electrical stimuli may minimize the duration and intensity of the acute stress experienced during training to virtual fencing technology. A better understanding of the factors that influence associative learning of the pairing of the audio and electrical stimuli in cattle is required to inform the development of best practice training protocols and guide the introduction of virtual fencing technology to naïve dairy cattle.
Age at training is one factor that may affect the rate of associative learning. For example, Kovalčik and Kovalčik (1986) found that 15-month old heifers were more efficient than primiparous and multiparous cows at learning the location of food in a maze. Theoretical modeling identifies several periods of increased neural plasticity during ontogeny, known as “sensitive periods” (Taborsky, 2017). The brain is highly sensitive to environmental stimuli during these periods which enables rapid learning (Sokolowski and Levine, 2010). To be clear, sensitive periods do not sharply define phases during which learning can occur and outside of which it cannot, but rather a gradual change in the ease or probability of learning is observed around these phases of development (Hinde, 1970). Sensitive periods often coincide with times of rapid morphogenesis, metamorphosis, sexual maturation, or other stages of ontogeny when physiological or morphological systems are undergoing major reorganization (Stamps and Groothuis, 2010). We thus hypothesized that training dairy heifers to virtual fencing technology at periods of their ontogeny that coincide with physiological or morphological change (i.e., pre-puberty juvenile period, around puberty early adolescence period, post-puberty late adolescence period) would increase the rate of associative learning compared to training more developed heifers that are close to calving age.
It is unknown whether heifers trained to virtual fencing technology at a younger age would retain their associative learnings over long periods of time without use, ensuring more rapid adaptation when managed with virtual fencing as an adult. There is a paucity in the scientific literature regarding the long-term memory abilities of cattle. Hirata et al. (2016) found Japanese Black cows were able to retain the memory of a complex maze configuration for 6 weeks, while Kovalčik and Kovalčik (1986) observed that 77% of cows but only 46% of heifers remembered the location of food in a maze after 6 weeks. In other livestock, Lee et al. (2006) found sheep to retain the memory of a maze configuration after 6 weeks. Considering that experiences during sensitive periods can produce long lasting neurobiological and behavioral change (Sokolowski and Levine, 2010), our secondary hypothesis was that the heifers that received training to virtual fencing technology early in their ontogeny would retain these learnings and show improved responsiveness to the technology when re-trained around calving age, compared to naïve heifers that are also around calving age.
Using dairy heifers in a feed attractant trial, this experiment aimed to determine the effects of age at training to virtual fencing technology on (1) the efficiency of associative pairing of a benign audio cue with an aversive electrical stimulus, and (2) the retention of this associative training over a long period of time without reinforcement. We predicted that virtual fencing training early in ontogeny (i.e., ≤ 12-months of age) would increase the rate of associative learning compared to training close to calving age, and that early training would improve the responsiveness of heifers to virtual fencing when re-trained ≥ 10-months later.
Materials and Methods
Ethical Statement
All animal procedures were conducted with institutional animal ethics approval obtained prior to the start of the experiment (University of Tasmania Animal Ethics Committee A0017004).
Animals and Experimental Design
This experiment was conducted over 17 months at the Tasmanian Institute of Agriculture Dairy Research Facility (TDRF) (41°08′S, 145°77′E; 155.0 m a.m.s.l), Elliott, north-west Tasmania, Australia. Fifty-nine weaned Holstein dairy heifers (Bos Taurus L.) were studied from ~6-months of age (mean ± sd; 185 ± 6.2 days). Heifers were separated from their dams at birth and housed in semi-enclosed pens (3 walls and a roof; 3.5 × 7 m) of 10 to 12 animals until weaning at ~90 days. After weaning, heifers were housed at pasture in a single mob of 138 similarly aged females and managed as per normal commercial practice. Heifers were fed a primarily pasture-based diet that was supplemented with silage when required. Water was supplied ad libitum. All studied heifers were impregnated via artificial insemination at ~15-months of age.
The experimental timeline is visually presented in Figure 1. The studied heifers were selected from the larger cohort of 138 animals and allocated to four treatments that differed in the age at which the naïve animals underwent training of the pairing of the audio and electrical stimuli. The treatments were as follows: 6M—initial training at 6-months of age (n = 15), 9M—initial training at 9-months of age (n = 15), 12M—initial training at 12-months of age (n = 15), and 22M—initial training at 22-months of age (n = 14). Typical of seasonal calving patterns, a period of 3-months separated the oldest and the youngest heifer in the cohort, so animals were assigned to treatments to reduce variation in age within treatments. The mean, standard variation, and coefficient of variation in the age and weights of heifers at the time of their initial training (i.e., at 6, 9, 12, or 22-months of age) are presented in Table 1. Heifers in the 22M treatment were 7-months pregnant during their initial training sessions. The seasonal calving pattern also meant that initial training of each treatment was conducted at different times of the year. The month of training and mean temperature, rainfall, and windspeed (during training hours) are detailed in Table 1. Fourteen heifers from the 6, 9, and 12M treatments underwent associative training for a second time when all the animals were 22-months of age (i.e., at the same time as heifers in the 22M treatment). All animals were 7-months pregnant during training at 22-months of age. Thus, the present experiment assessed the relationships between age at training of naïve heifers on (1) the rate of learning of the association between audio and electrical stimuli and (2) the retainment of this learning after an extended period of time without reinforcement (Figure 1).
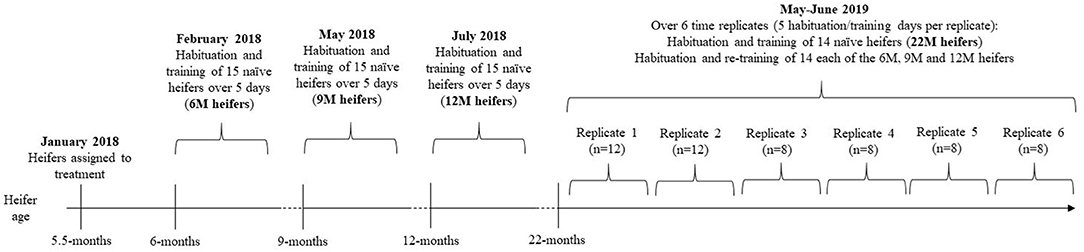
Figure 1. Experimental timeline. Groups of heifers that were naïve to virtual fencing underwent training to the association between audio and electrical stimuli at 6-months (6M), 9-months (9M), 12-months (12M), or 22-months (22M) of age. The 6, 9, and 12M heifers underwent a second round of training at 22-months of age (i.e., at the same time as the naïve 22M heifers). The second round of training was conducted over 6 time-replicates, with even representation of treatments within each replicate. Treatment heifers were separated from the herd 3 days prior to commencement of habituation and training. Habituation to the test arenas and to collars was conducted over 3 days and training was conducted over 2 days (2 training sessions per day). This experimental design allowed for an assessment of the effects of age on rate of learning and the retainment of learning after an extended period without reinforcement.
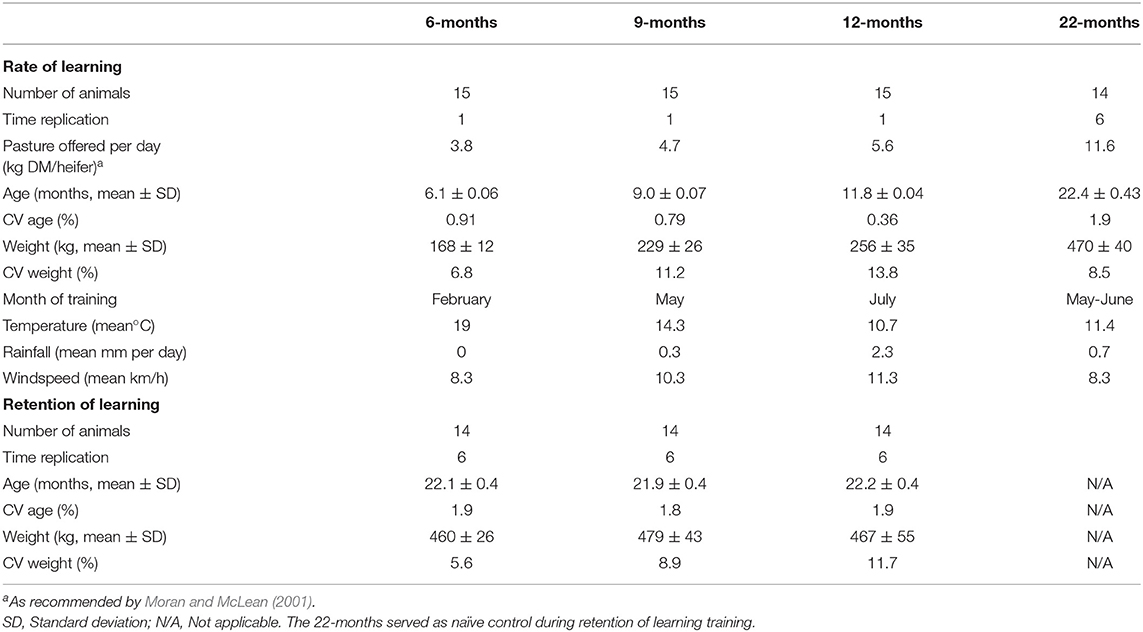
Table 1. Details relating to heifers when introduced to the virtual fencing technology at 6, 9, 12, or 22-months of age and when re-trained to the technology at 22-months of age.
Initial training sessions for the naïve heifers in the 6M, 9, and 12M treatments were conducted in a single week (i.e., time replicate). Restrictions on time and collar availability meant that associative training during the second training sessions (i.e., at 22-months of age) were conducted over 6 successive time replicates (Figure 1). An equal number of heifers from the 6, 9, 12, and 22M treatments were trained in each of these replicates (n = 12 heifers in replicates 1 and 2, n = 8 heifers in replicates 3–6; total n = 56 heifers).
Heifers were separated from the larger mob of animals 3 days prior to the commencement of their associative training sessions and housed as a single group in a paddock close to the training arena (< 100 m). During this period heifers received a fresh allocation of pasture each day and were provided with water ad libitum (see Table 1 for details on pasture allocation). Animals remained in these groups for the duration of training after which they were returned to the larger mob of heifers.
Training of the Pairing of Audio and Electrical Stimuli
The Collars
The electronic collars used to remotely deliver the audio cues and electrical stimuli were the same as those used by Verdon et al. (2020). The collars were based on those used for dog training (ET300 Mini-educator, E-Collar Technologies, Garrett, IN, USA), fitted into a custom casing (MooMonitor+, Dairymaster Inc., Kerney, Ireland) and enabled an operator to manually deliver audio or electrical stimuli through a remote control device. The range of the collar and remote-control device communication system was 800 m. The electronic collar was secured around the neck of the heifers and two electrodes that delivered the electrical stimulus were positioned to contact the skin in a shaved area behind the poll. The audio stimulus was a constant polyphonic tone (84 dB) delivered from two speakers just behind the ears of the animal. The electrical stimulus intensity was set to 3 V (120 mW), which equated to a setting of 50 on the 100-point scale provided with the remote-control device.
The Test Arena
The layout of the test arena where animals were trained to the association between the audio and electrical stimuli differed between the initial training of 6M heifers and all other training sessions. The layout of the test arena used in the initial associative training of the 6M heifers is presented in Supplementary Figure 1 and the test arena used in all other associative training sessions is presented in this manuscript as Figure 2. Changes to the layout were made to improve training procedures following observations during 6M training sessions. These changes are detailed below.
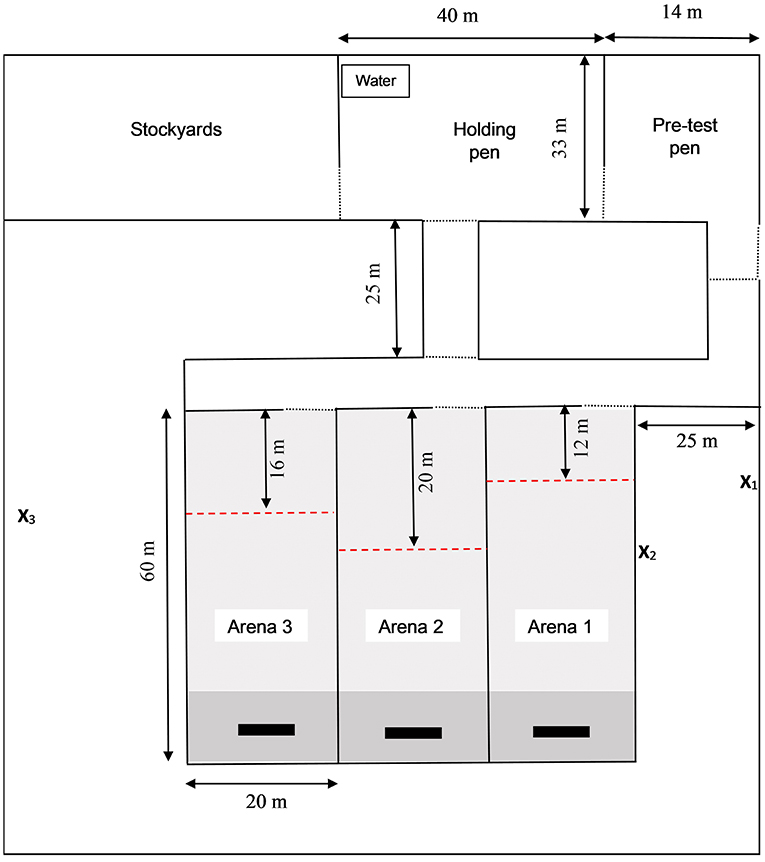
Figure 2. Layout of the test arena where training of the association between audio and electrical stimuli was conducted. The test arena consisted of stockyards, pre- and post-test holding pens and three training arenas which were rotated between training sessions. Collars that delivered the audio and electrical stimuli to heifers were fitted in the stockyards after which animals were housed as a group in the pre-test pen. Individual heifers were removed from the pre-test pen for associative training and housed in the holding pen after training. A trough of grain was positioned at the far end of the training paddock (indicated by black rectangles, ) to motivate animals to move down the paddock. Pasture was mown for the first 90% of each arena (
) leaving longer pasture as an additional attractant at the far end (
). During training, a virtual fence boundary separated an “inclusion zone” (i.e., area in which animals could move freely) from an “exclusion zone” (i.e., area beyond which stimuli would be applied). The exclusion zone was set at a different length from the entry of each test arena, as indicated by red dashed line (
). The position of video cameras recording heifer behavior and of the researcher responsible for manually administering the audio and electrical stimuli via a remote control device are indicated by X1, X2, and X3 for training arenas 1, 2, and 3 respectively. Lockable gates are represented by a dotted line (…). Note that the test arena differed slightly during the introduction training for 6-month old heifers (see Supplementary Figure 1 for this test arena).
Both test arenas consisted of stockyards, two temporary holding paddocks, and three training paddocks. A trough of grain was positioned at the end of the training paddock to motivate the animals to move down the far end of the paddock. To account for seasonal variability in pasture growth and quality, and to further encourage heifers to move to the far end of the paddock, pasture was mown so that only the final 10% of the paddock area contained fresh pasture. The first test arena used for initial training of 6M heifers was constructed using temporary electrified poly-wire fencing, did not include a walkway leading up to test paddock 1, and the post-test holding paddock was located 80 m beyond the end of the training paddocks (Supplementary Figure 1). Observations made during the 6M training sessions suggested that one or a combination of these factors were motivating heifers to challenge the virtual fence despite effective associative learning, particularly when training was being conducted in arena 1. The second test arena was built using non-electrified permanent fencing and included a 25-meter walkway between the pre-test pen and the training paddocks. The new arena also positioned the post-test holding pen in front of training paddock. This second test area is the same as that described by Verdon et al. (2020) and was utilized for all training in the present experiment excluding the initial training of the 6M heifers.
During training, animals were relocated from their paddock to the stockyards at ~0900 h for the fitting of the electronic collars and individualized marking of both flanks using stockmarker. Heifers were then held as a single group in the pre-test paddock located in front of the training paddocks. After each habituation or training session, heifers were moved to the post-test holding paddock where they remained until all animals had been tested (habituation and training procedures are described in the following sections). Animals were able to graze available pasture in the pre- and post-test holding paddocks (<1800 kg DM/ha) and water was provided ad libitum. Collars remained fitted for the two habituation or training sessions held each day (session 1 between 10:00 and 11:00, session 2 between 14:30 and 15:30). At ~16:00, heifers were moved back to the stockyards where collars were removed before animals were returned to their paddock.
Habituation Procedures
Heifers underwent a 3-day habituation period prior to training to familiarize them with the test areas and the location of the feed attractant. The electronic collars were not activated during the six habituation sessions (2 per day). Heifers were introduced to the training paddocks in groups of 5 for the first habituation, in pairs for the second and individually for habituation sessions 3–6. The training paddock being utilized was rotated with each session, ensuring that heifers received two habituations per paddock (one AM and one PM). Heifers were given free access to the training paddock during habituation and provided with as much time as required to start consuming the grain. Once feeding commenced, heifers were permitted to feed for 3 min. By the final habituation day, all animals began consuming grain following entry to the training paddock in a median of 40 s (range 14–140 s) during the initial training of naïve animals and 26.5 s (range 9.5–139 s) during the retention of learning training sessions at 22-months of age.
Training Procedures
Four sessions of training of the pairing of the audio and electrical stimuli with activated collars were held over 2 days immediately following the habituation period. This number of sessions was chosen because previous research found that from the fourth training sessions heifers could be categorized as consistently avoiding interacting with the virtual fence or consistently tolerating the electrical stimuli to reach the feed attractant (Verdon et al., 2020). Individual heifers were introduced to the test arenas during each training session. The training paddock being utilized followed the same rotation as that used during habituation. A virtual fence boundary separating an “inclusion zone” (i.e., area in which animals could move freely) from an “exclusion zone” (i.e., an area beyond which the audio and electrical stimuli would be applied) was established at either 12, 16, or 20 m from the entrance to the training paddock, depending on the paddock being utilized. Distances varied between paddocks to delay animals learning an association between delivery of stimuli and the location of the exclusion zone. A researcher with experience in using the manual collars for training heifers was located ~20 m outside the training paddock to administer audio or electrical stimuli remotely (Figure 2). There was no visual indication of the exclusion zone apart from a small amount of white tape on the fence to aid the researcher.
The following procedures determined the application of the audio and electrical stimuli by the researcher, as utilized by Verdon et al. (2020). Based on the researcher's visual estimation, the audio stimulus was applied for 2 s as the heifer entered the exclusion zone. If the heifer stopped moving further into the exclusion zone, the application of the audio stimulus immediately ceased. If the heifer continued to move forward, however, an electrical stimulus (< 0.5 s) was immediately administered by the researcher. If the heifer recommenced or continued movement into the exclusion zone after the delivery of the electrical stimulus, the audio stimulus was re-applied, immediately followed by another electrical stimulus if again she continued to move into the exclusion zone. No further stimuli were applied to an animal unless she was further proceeding into the exclusion zone. The training session concluded if (1) no breaches into the exclusion zone were made within 3-min of entry into the training paddock, (2) a period exceeding 3-min separated two breaches into the exclusion zone, or (3) a maximum number of five electrical stimuli were delivered. Heifers were videotaped during training (Panasonic camcorder, model NV-DS60; Panasonic Corporation, Osaka, Japan) so that their behavior could be translated at a later date.
Measures Recorded
The following data were collected during both the initial training sessions of naïve heifers and the retention of learning training sessions conducted at 22-months of age. The number of interactions with the virtual fence and the number of audio and electrical stimuli delivered were recorded in situ and confirmed by a single observer that was blinded to treatment using video records. The number of interactions the heifer had with the virtual fence before responding to the audio cue alone was retrospectively determined using these stimulus data. The proportion of interactions with an electrical stimulus was calculated as the number of electrical stimuli delivered ÷ the number of audio cues delivered. The following measures were also obtained from video records taken during the initial training sessions of naïve animals by a single observer: the time taken to interact with the virtual fence; the time taken for the heifer to reach feed attractant; the behavioral response of heifers to the audio or electrical stimuli (see Table 2 for ethogram). The ethogram for behavior observations was adapted from Verdon et al. (2020) but to improve inter-observer reliability the behavioral response of animals immediately following the application of the stimulus was assessed (i.e., within 2 s). Observations on a subset of 7 heifers were repeated by the original observer and by one other observer. This determined high intra-observer reliability for most behaviors (rs ≥ 0.95) and high inter-observer reliability for all behaviors (rs ≥ 0.94). A single discrepancy lowered the intra-observer reliability for the behavior “stop feeding/grazing” (rs = 0.76), but this is still considered an acceptable level of agreement (Martin and Bateson, 1993).
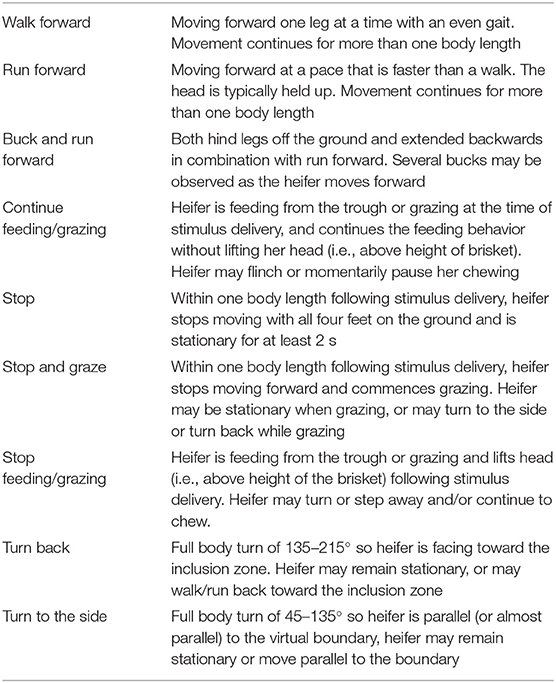
Table 2. Ethogram of cattle behaviors recorded during associative training. Intra-observer reliability rs > 0.76, inter-observer reliability rs > 0.94.
Statistical Analysis
Analyses were restricted to use of data collected from the first three training sessions. This decision was made on the basis that only around 50% of animals interacted with the virtual fence at the fourth training session during both the initial training of naïve animals and the retention of learning training at 22-months of age (Cochran's Q-test—Initial training χ2(3) = 24.1, P < 0.001; Retention of learning training χ2(3) = 39.5, P < 0.001; Figure 3). Similar findings were reported by Verdon et al. (2020). As such, only data from sessions 1–3 were considered most representative of the associative learning process. Data relating to the time taken to interact with the virtual fence and for the heifer to reach the feed attractant were averaged over training sessions 1 to 3. The number of audio cues and electrical stimuli delivered were summed over sessions 1–3. The proportion of total interactions with the virtual fence in which an electrical stimulus was delivered was calculated from the summed stimuli data. Behavioral responses of individual heifers to the audio cue or electrical stimuli during the initial training sessions were calculated as a proportion of total behavioral responses (to audio or electrical stimuli) observed over sessions 1–3.
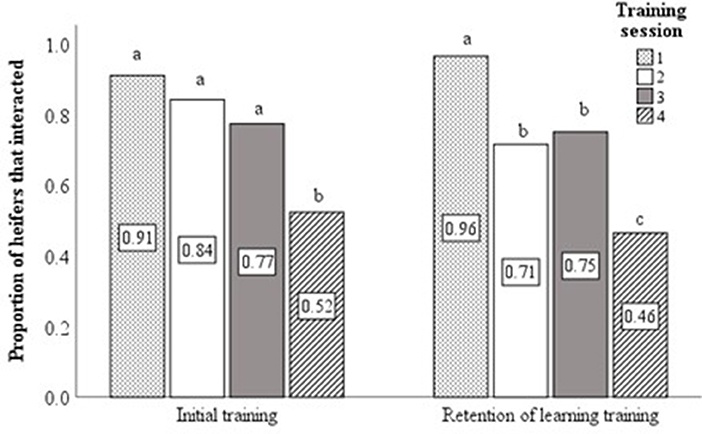
Figure 3. Using data from the four training sessions, bar charts displaying the proportion of heifers that interacted with virtual fence during the initial (n = 59) and retention of learning (n = 56) training sessions. Different superscript lettersabc show where treatment means differ.
All statistical analyses were carried out using the SPSS statistical software package (SPSS 26.0, SPSS Inc., Chicago, IL, USA) and the unit of analyses was the individual heifer. The effects of age at training on the rate of learning (initial training at 6, 9, 12, or 22M) and retention of learning (all animals trained at 22-months of age) were analyzed separately using generalized linear mixed models (GLMM). A visual inspection of the quantile-quantile plots and histograms were conducted prior to both sets of analysis. Data relating to the proportion of interactions in which an electrical stimulus was delivered were subsequently arcsine square-root transformed while duration data were logarithmically transformed (time to interact with the virtual fence, time to reach feed attractant), so that residual variation was homogenous between treatments.
Effects of Age on Rate of Learning
Differences in the design of the test arena meant that the 6M treatment could not be included in the analysis on the effects of age at training on the rate of associative learning. A technical malfunction at day 1 (training sessions 1 and 2) also meant that the time 6M heifers took to interact with the virtual fence and their behavioral response to audio and electrical stimuli could not be obtained from video records. For the 6M heifers, the proportion of interactions with an electrical stimulus, the total number of interactions with the virtual fence and the time to reach the feed attractant are presented to facilitate a descriptive comparison to the other treatments.
The initial training sessions of naïve animals were conducted in a single time replicate for 6, 9, and 12M treatments, but 22M heifers were trained over 6 successive time replicates. A new factor named “time replicated” was created to account for this unbalanced replication. The treatments that were not replicated in time (i.e., 9 and 12M) were assigned to one level of this factor, while the 22M treatment that was replicated over time was assigned to a second level. The effect of treatment (i.e., initial training at 9, 12, or 22M) nested within “time replicated” was then included in each analysis as a fixed factor. The number of interactions the heifer had with the virtual fence before responding to the audio cue alone and the total number of audio cues delivered were analyzed with a poisson distribution and log link. The time heifers took to interact with the virtual fence, the proportion of interactions that included an electrical stimulus, and the time taken for them to reach the feed attractant were analyzed with a normal distribution and identify link. Heifers that did not breach the exclusion zone were recorded as missing values for the time to interact with the virtual fence or reach feed attractant. As such, the Satterwaite approximation was used to calculate degrees of freedom. The behavioral response of heifers to the audio and electrical stimuli were analyzed for treatment effects using a non-parametric Kruskal-Wallis test. A separate analysis was conducted for each behavior observed after administration of the audio cue or the electrical stimulus. Heifers that did not breach the exclusion zone were recorded as missing values in these analyses. Inter- and intra-observer reliabilities for each behavior were assessed using non-parametric spearman rank correlations.
Effects of Age on Retention of Learning
All heifers were 22-months of age during the retention of learning training sessions. Each model included the main effect of treatment (heifers that were initially trained at 6, 9, 12M and undergoing a second round of training compared to naïve 22M heifers undergoing their first round of training) and time replicate (1–6) as a random blocking factor. The number of interactions the heifer had with the virtual fence before responding to the audio cue alone was analyzed with a poisson distribution and log link, while the proportion of interactions that included an electrical stimulus was analyzed with a normal distribution and identify link. A Wilcoxon Signed Rank test was used to examine differences in the proportion of interactions with the virtual fence that included an electrical stimulus during the initial and second training sessions for 6, 9, and 12M treatments.
Results
To aid with interpretation, raw means are presented with estimated marginal means ± SEM (and backtransformed estimated marginal means where relevant) presented in Supplementary Table 1.
Effects of Age on Rate of Learning
Age at training affected the rate of learning of the association between audio and electrical stimuli. Heifers that were trained at 22-months of age (called 22M) required fewer interactions with the virtual fence before responding to the audio cue alone, compared to training at an earlier age [i.e., 9 or 12-months; called 9 and 12M, respectively; F(2, 40) = 11.4, P < 0.001; Figure 4A]. Consequently, the proportion of interactions with the virtual fence in which an electrical stimulus was delivered was lower at 22M than 9 or 12M [F(2, 40) = 21.2, P < 0.001; Figure 4B]. Data from the heifers trained at 6-months of age (called 6M) were not included in these analyses, but a descriptive comparison suggests 6M heifers behaved more similarly to 9 and 12M heifers than the 22M heifers (Figures 4A,B). Age at training did not affect the time it took heifers to interact with the virtual fence [F(2, 39) = 2.5, P = 0.1; Figure 4C] or the total number of interactions [F(2, 41) = 1.5, P = 0.23; Figure 4D], but heifers trained at 12M reached the feed attractant more quickly than 22M heifers [F(2, 29) = 4.5, P = 0.02; Figure 4E].
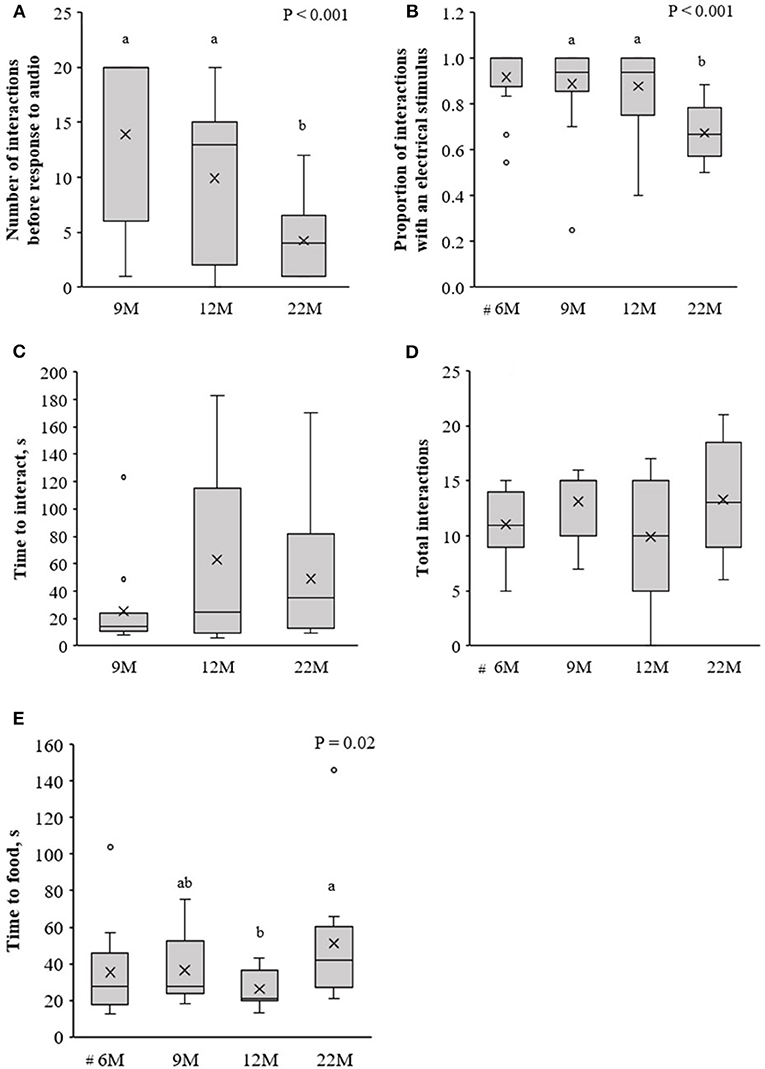
Figure 4. Naïve heifers that were trained to the virtual fencing technology at 6, 9, 12, or 22-months of age (6, 9, 12, 22M, respectively). Using data from the first three training sessions of naïve animals, (A) the number of interactions with the virtual fence before the heifer responded to the audio cue alone, (B) the proportion of interactions with the virtual fence during which an electrical stimulus was delivered, (C) the time to interact with the virtual fence, (D) the total number of interactions with the virtual fence, and (E) the time to reach the feed attractant. #The test arena layout differed for the initial training of 6M heifers compared to all other training sessions, so these animals were not included in this analysis. Raw data are presented. Estimated marginal means are presented in Supplementary Table 1. Boxplots show the median and the first and third quartiles (25 and 75% of data), with whiskers extending to the lowest and highest values. Values >1.5 × the interquartile range (IQR) are indicated by o. Different superscript lettersabc show where treatment means differ.
Behavior During Training
Nine different behavioral responses were observed following both the delivery of the audio cue and electrical stimuli (Table 3). The most frequently observed responses to the audio cue included run forward (34% of responses), walk forward (28% of responses), or continue grazing (19% of responses). The most frequently observed responses to the electrical stimuli were run forward (30% of responses), walk forward (18% of responses), continue grazing (14% of responses), and buck while running forward (13% of responses). Compared to the 22M heifers, following the audio cue, animals in the 9 and 12M treatments were more likely to run forward and less likely to stop (moving forward or feeding) and turn back (Table 3). Heifers in the 9M treatment were more likely to continue grazing following an electrical stimulus than 12 and 22M heifers (Table 3). There were few other effects of age at training on the behavioral response of heifers to electrical stimulus (see Table 3).
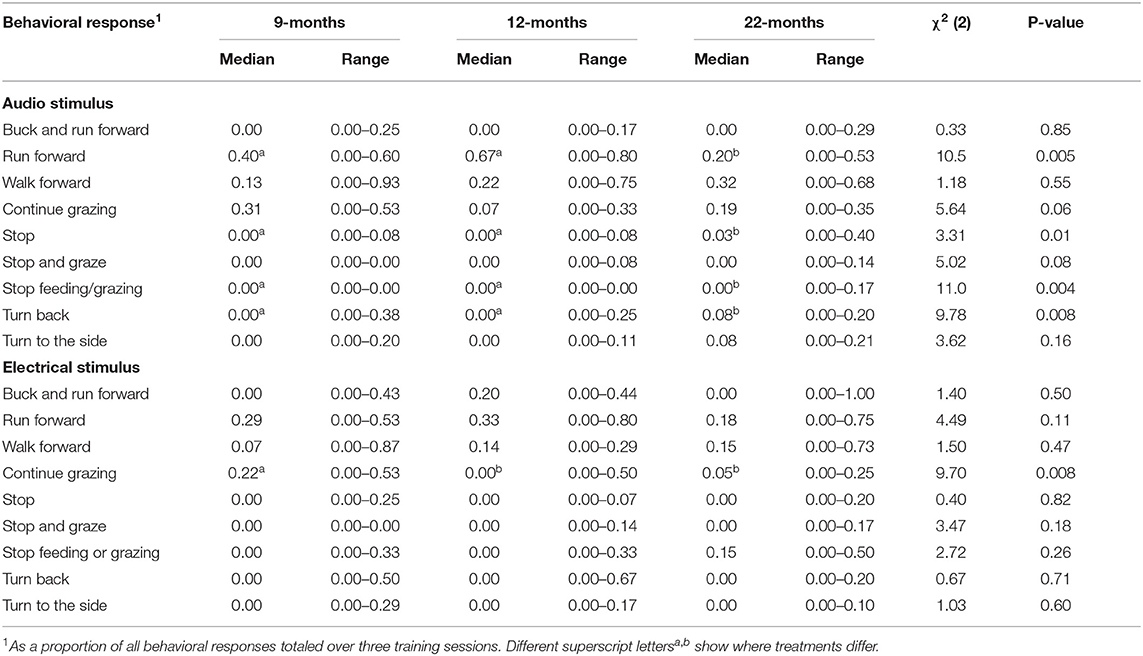
Table 3. The effect of age at training of the pairing of audio and electrical stimuli (9, 12, or 22-months of age) on the behavioral response to audio and electrical stimuli (n = 42).
Effects of Age on Retention of Learning
There were no beneficial effects of previous training at an early age (i.e., 6, 9, or 12-months of age) on the responsiveness of heifers to the audio and electrical stimulus when re-trained at 22-months of age. Compared to naïve 22M heifers, animals that were initially trained at 6 and 9M required more interactions with the virtual fence before responding to the audio cue alone, with 9M heifers requiring the highest number of interactions [F(3, 52) = 10.5, P < 0.001; Figure 5A]. There was an effect of treatment on the proportion of interactions in which an electrical stimulus was delivered [F(3, 58) = 2.8, P = 0.046; Figure 5B]. The 9M heifers received a higher proportion of electrical stimuli compared to 12M heifers and tended receive a higher proportion than 22M heifers (LSD test P = 0.055). Heifers that underwent training at 6 and 12M did not differ from the naïve heifers in the proportion of interactions with an electrical stimulus.
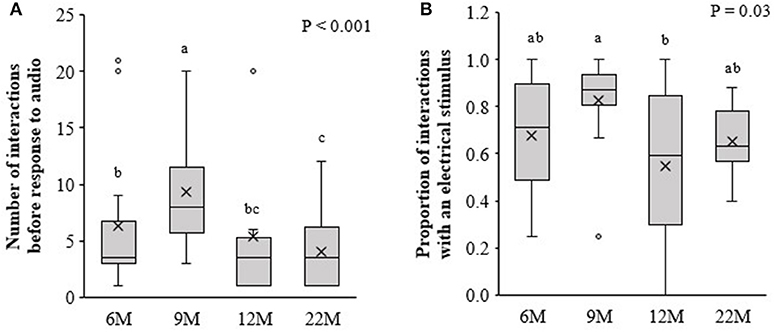
Figure 5. Retention of learning: Heifers that were introduced to virtual fencing at 6, 9, or, 12-months of age (6, 9, and 12M, respectively) and re-trained at 22-months of age, alongside a cohort of naïve heifers being introduced to virtual fencing at 22-months of age (22M). Using data from the first three retention of learning training sessions, (A) the number of interactions with the virtual fence before the heifer responded to the audio cue alone and (B) the proportion of interactions with the virtual fence during which an electrical stimulus was delivered. Raw data are presented. Estimated marginal means are presented in Supplementary Table 1. Boxplots show the median and the first and third quartiles (25 and 75% of data), with whiskers extending to the lowest and highest values. Values >1.5 × the interquartile range (IQR) are indicated by o. Different superscript lettersabc show where treatment means differ.
Wilcoxon Signed Rank tests showed that the proportion of interactions with an electrical stimulus was higher during initial training than during re-training for the 6M (Z = −2.5, P = 0.013), 9M (Z = −2.1, P = 0.04), and 12M (Z = −3.0, P = 0.003) treatments. The reduction in the proportion of interactions with electrical stimulus over time was more pronounced for 6 and 12M heifers than for 9M heifers.
Discussion
This experiment used a manual training collar to assess the effect of dairy heifer age at training on the rate of learning of the association between a benign audio cue and aversive electrical stimulus, and on the retention of this learning over a long period without reinforcement. Contrary to expectations, the rate of learning was accelerated in older (i.e., 22-months of age) compared to younger heifers (i.e., 6, 9, or 12-months of age), and there were no beneficial effects of previous training at an early age on the responsiveness of heifers when re-trained ≥10-months later.
Effects of Age on Rate of Learning
Heifers that underwent training at 22-months of age took fewer interactions to respond to the audio cue alone, received a lower proportion of electrical stimuli, and were less likely to run forward and more likely to stop moving forward after the application of the audio cue, compared to the younger heifers. Reproductive status is one important difference between the older and younger heifers in this experiment, as the older heifers were ~7-months pregnant at training. Humans and animals experience significant hormonal and neurochemical changes during pregnancy (reviewed by Kim, 2016). These changes may affect the salience of the stimuli delivered during training. For example, pregnant women show heightened neural activity and increased attention to pictures of fearful or angry faces, particularly when tested toward late pregnancy (Kim, 2016). This maternal hyper-vigilance is thought to maximize the protection of offspring from potential dangers (Barba-Müller et al., 2019). Thus, hormonal changes related to pregnancy may have increased the attention of the 22-month old heifers to the electrical stimulus, allowing them more quickly to learn to control receipt of the stimulus through behavioral change.
A second explanation for the faster rate of learning observed in the 22-month old heifers is that their older age had provided greater opportunities for experiences that improve associative learning or other cognitive skills. For example, Verdon et al. (2020) found that experience with electric fencing resulted in more rapid associative pairing of audio and electrical stimuli in dairy heifers compared to those that had no experience with electric fencing, and the more interactions a heifer had with an electric fence the more responsive she was to the audio cue during associative training. Compared to their younger counterparts, the older heifers in this experiment would also have had greater exposure to the unpredictable environmental changes common in pasture-based dairy systems (e.g., variable environmental conditions, movement to new areas of the farm, variability in the quantity, quality and composition of feed, interactions with humans). Exposure to environmental unpredictability may strengthen cognitive skills by inducing higher levels of neuronal plasticity allowing the animal to cope better with fluctuating environmental conditions (Taborsky, 2017). This has been demonstrated by research in fish, where environmental uncertainty induced higher levels of neuronal and behavioral plasticity as well as improved cognitive abilities (Kotrschal and Taborsky, 2010; Roy and Bhat, 2016; Carbia and Brown, 2019).
The motivation of younger heifers to access the feed attractant may have been greater than for the older heifers, making them more willing to tolerate the electrical stimulus. According to the framework proposed by Mendl and Paul (2020), a heifer's current internal status (e.g., state of hunger) would affect its estimation of the value, or rewarding properties, of the feed attractant. A feed attractant trial with a similar design to the present experiment observed the proportion of electrical stimuli delivered to 6-month old heifers to decline after the provision of fresh silage (Verdon et al., 2020). We consider this likelihood to be low in the present experiment, however, because all animals were fed to recommendations for their age. The lack of time replication in the training of younger heifers (training conducted over 1 week compared to over 6 weeks for the 22-month heifers) is a limitation of this research that prevents us from accounting for any variation in the rate of learning within treatments that could be associated with fluctuations in feeding (e.g., quality of pasture) or environmental conditions.
Effects of Age on Retention of Learning
Relative to naïve 22-month old heifers, animals that were initially trained at 9-months required more interactions with the virtual fence before responding to the audio cue alone and tended to have more interactions with an electrical stimuli when re-trained at 22-month old, however, there were few differences between heifers that underwent training at 6 or 12-months of age and the naïve heifers. These findings suggest that heifers did not retain their associative learnings over a long period (i.e., ≥ 10-months). Other research on adult cows shows that they can be trained by conditioning to a stimulus using negative reinforcement, but that behavior is soon extinguished without regular stimulus use (Albright, 1981). Our data indicate that stock need to be re-trained following an extended period of time without virtual fencing technology (e.g., heifers that have been reared by a contractor, dry cows that have been moved to a different location during periods of low pasture growth), however, more research is required to determine how long the audio and electrical stimuli association is maintained.
The 9-month old Holstein heifers in this experiment would likely have been in the early adolescent period of development at the time of their initial training, given that Holstein-Friesian heifers experience their first ovulation at an average of 9.5-months (Wathes et al., 2014). Adolescence is characterized by dramatic neurophysiological, hormonal and behavioral change (Lo Iacono and Carola, 2018). A recent study suggests that individual consistency in the behavioral response of dairy cattle to novelty is poor across the developmental period of puberty compared to pre-weaning and in the first lactation (Neave et al., 2020). The HPA-axis and brain regions involved in learning, memory and higher cognitive abilities (e.g., behavioral suppression, attention and decision making) are highly plastic and particularly susceptible to environmental stressors during adolescence (see reviews by Green and McCormick, 2013; Baker et al., 2014; Lo Iacono and Carola, 2018). We thus hypothesize that the stressful experience of associative training during the sensitive early adolescence developmental period caused neurological changes with possible long-term effects on emotionality and/or cognitive processing for the 9-month old heifers in this experiment.
Scientific understanding of the physiological and behavioral effects of stress during adolescence has been developed primarily through research on rodents under laboratory conditions. Morrissey et al. (2011) exposed adolescent or adult rats to 16 days of social instability stress, followed by fear conditioning weeks later. The adolescent and adult rats did not differ in rate of conditioning but stress during adolescence decreased context and cue memory later in life. Other evidence from laboratory rats shows that stress during adolescence increases fear or anxiety like behavior (e.g., Brydges et al., 2012; Yee et al., 2012; Müller et al., 2018) and risk-taking in the adult (Toledo and Sandi, 2011; Brydges et al., 2012; Traslaviña et al., 2014). We are unable to conclude whether the reduced performance of the 9-month old heifers during re-training in this experiment is related to impaired cognitive function (e.g., behavioral suppression, attention, or decision making) or changes in emotional state (e.g., anxiety). Parts of the human brain relating to stimulus salience, which is used in threat detection, and emotional regulation, used in behavioral suppression, are frequently co-activated (Barba-Müller et al., 2019). Indeed, a major function of emotional states is to organize and guide behavioral choices (Mendl and Paul, 2020). Thus, dysfunction in the cognitive domain is often related to dysfunction in the emotional domain and vice versa (see review by Green and McCormick, 2013).
An emotionally resilient cow with high cognitive abilities may be better equipped to cope with the increasingly complex environment provided by modern dairy farms (in terms of uptake of technologies and herd sizes). This includes the possible use of virtual fencing to implement increasingly intensive and complex grazing regimes in pastoral dairy systems (e.g., Verdon et al., 2018). We encourage future work to assess the effects of stress at sensitive periods of ontogeny on the development of emotionality and cognition in farm animals.
Conclusions
Results of this experiment indicate that training heifers close to calving age (i.e., 22-months) achieves more rapid associative pairing of audio and electrical stimuli compared to training at a younger age (≤ 12-months). There were no benefits of previous training at an early age on the responsiveness of heifers when re-trained at 22-months of age, and some evidence that the experience of associative training during early adolescence (at about 9-months of age) has long-term negative effects on emotionality and/or cognitive processing. We conclude that dairy heifers should be trained to virtual fencing technology close to calving age rather than earlier in their ontogeny and recommend that stock be re-trained following an extended period without virtual fencing technology.
Data Availability Statement
The original contributions presented in the study are included in the article/Supplementary Material, further inquiries can be directed to the corresponding author/s.
Ethics Statement
The animal study was reviewed and approved by University of Tasmania Animal Ethics Committee A0017004. Written informed consent for participation was not obtained from the owners because these animals were University owned livestock for research purposes.
Author Contributions
MV and RR contributed conception and design of the experiment. MV conducted the experiment, organized the database, performed the statistical analyses, and wrote the first draft of the manuscript. All authors contributed to manuscript revision, read, and approved the submitted version.
Funding
This research was funded by the Australian Commonwealth Government Department of Agriculture and Water Resources as part of its Rural R&D for Profit Programme, Grant Number 15-02-019.
Conflict of Interest
The authors declare that the research was conducted in the absence of any commercial or financial relationships that could be construed as a potential conflict of interest.
Acknowledgments
Thanks to Nathan Bakker, Adam Langworthy, Andrew Bailey, Andrew Gellie, and the staff at TDRF for their support in operating this project.
Supplementary Material
The Supplementary Material for this article can be found online at: https://www.frontiersin.org/articles/10.3389/fanim.2020.618070/full#supplementary-material
References
Baker, K. D., Den, M. L., Graham, B. M., and Richardson, R. (2014). A window of vulnerability: Impaired fear extinction in adolescence. Neurobiol. Learn. Mem. 113, 90–100. doi: 10.1016/j.nlm.2013.10.009
Barba-Müller, E., Craddock, S., Carmona, S., and Hoekzema, E. (2019). Brain plasticity in pregnancy and the postpartum period: links to maternal caregiving and mental health. Arch. Women's Mental Health 22, 289–299. doi: 10.1007/s00737-018-0889-z
Brydges, N. M., Hall, L., Nicolson, R., Holmes, M. C., and Hall, J. (2012). The effects of juvenile stress on anxiety, cognitive bias and decision making in adulthood: a rat model. PLoS ONE 7:e48143. doi: 10.1371/journal.pone.0048143
Campbell, D., Haynes, S., Lea, J., Farrer, W., and Lee, C. (2019). Temporary exclusion of cattle from a riparian zone using virtual fencing technology. Animals 9:5. doi: 10.3390/ani9010005
Carbia, P. S., and Brown, C. (2019). Environmental enrichment influences spatial learning ability in captive-reared intertidal gobies (Bathygobius cocosensis). Anim. Cogn. 22, 89–98. doi: 10.1007/s10071-018-1225-8
Green, M. R., and McCormick, C. M. (2013). Effects of stressors in adolescence on learning and memory in rodent models. Horm. Behav. 64, 364–379. doi: 10.1016/j.yhbeh.2012.09.012
Hinde, R. A. (1970). Animal Behaviour: A Synthesis of Ethology and Comparative Psychology. New York, NY: McGraw-Hill.
Hirata, M., Tomita, C., and Yamada, K. (2016). Use of a maze test to assess spatial learning and memory in cattle: Can cattle traverse a complex maze? Appl. Anim. Behav. Sci. 180, 18–25. doi: 10.1016/j.applanim.2016.04.004
Kim, P. (2016). Human maternal brain plasticity: adaptation to parenting. New Dir. Child Adolesc. Dev. 2016, 47–58. doi: 10.1002/cad.20168
Kotrschal, A., and Taborsky, B. (2010). Environmental change enhances cognitive abilities in fish. PLoS Biol. 8:e1000351. doi: 10.1371/journal.pbio.1000351
Kovalčik, K., and Kovalčik, M. (1986). Learning ability and memory testing in cattle of different ages. Appl. Anim. Behav. Sci. 15, 27–29. doi: 10.1016/0168-1591(86)90019-5
Lee, C., Colditz, I. G., and Campbell, D. L. (2018). A framework to assess the impact of new animal management technologies on welfare: a case study of virtual fencing. Front. Vet. Sci. 5:187. doi: 10.3389/fvets.2018.00187
Lee, C., Colegate, S., and Fisher, A. D. (2006). Development of a maze test and its application to assess spatial learning and memory in Merino sheep. Appl. Anim. Behav. Sci. 96, 43–51. doi: 10.1016/j.applanim.2005.06.001
Lo Iacono, L., and Carola, V. (2018). The impact of adolescent stress experiences on neurobiological development. Semin. Cell Dev. Biol. 77, 93–103. doi: 10.1016/j.semcdb.2017.09.040
Lomax, S., Colusso, P., Gargulio, J., and Clark, C. (2019). Determining learning and behavioural response to a virtual fence for dairy cows. Animals 9:429. doi: 10.3390/ani9070429
Martin, P., and Bateson, P. (1993). Measuring Behaviour: An Introductory Guide. Cambridge: Cambridge University Press.
Mendl, M., and Paul, E. S. (2020). Animal affect and decision-making. Neurosci. Biobehav. Rev. 112, 144–163. doi: 10.1016/j.neubiorev.2020.01.025
Moran, J., and McLean, D. (2001). Heifer Rearing: A Guide to Rearing Dairy Replacement Heifers in Australia. Bolwarrah, VIC: Bolwarrah Press.
Morrissey, M. D., Mathews, I. Z., and McCormick, C. M. (2011). Enduring deficits in contextual and auditory fear conditioning after adolescent, not adult, social instability stress in male rats. Neurobiol. Learn. Mem. 95, 46–56. doi: 10.1016/j.nlm.2010.10.007
Müller, I., Brinkman, A. L., Sowinski, E. M., and Sangha, S. (2018). Adolescent conditioning affects rate of adult fear, safety and reward learning during discriminative conditioning. Sci. Rep. 8:17315. doi: 10.1038/s41598-018-35678-9
Neave, H. W., Costa, J. H., Weary, D. M., and Von Keyserlingk, M. A. (2020). Long-term consistency of personality traits of cattle. R. Soc. Open Sci. 7:191849. doi: 10.1098/rsos.191849
Roy, T., and Bhat, A. (2016). Learning and memory in juvenile zebrafish: what makes the difference–population or rearing environment? Ethology 122, 308–318. doi: 10.1111/eth.12470
Sokolowski, M. B., and Levine, J. D. (2010). “Nature-nurture interactions,” in Social Behaviour: Genes, Ecology and Evolution, eds T. Szekely, A.J. Moore, and J. Komdeur (New York, NY: Cambridge University Press), 11–25. doi: 10.1017/CBO9780511781360.003
Stamps, J., and Groothuis, T. G. (2010). The development of animal personality: relevance, concepts and perspectives. Biol. Rev. 85, 301–325. doi: 10.1111/j.1469-185X.2009.00103.x
Taborsky, B. (2017). Developmental plasticity: preparing for life in a complex world. Adv. Study Behav. 49, 49–99. doi: 10.1016/bs.asb.2016.12.002
Toledo, M., and Sandi, C. (2011). Stress during adolescence increases novelty seeking and risk-taking behavior in male and female rats. Front. Behav. Neurosci. 5:17. doi: 10.3389/fnbeh.2011.00017
Traslaviña, G. A. A., de Oliveira, F. L., and Franci, C. R. (2014). Early adolescent stress alters behavior and the HPA axis response in male and female adult rats: the relevance of the nature and duration of the stressor. Physiol. Behav. 133, 178–189. doi: 10.1016/j.physbeh.2014.05.031
Verdon, M., Lee, C., Marini, D., and Rawnsley, R. (2020). Pre-exposure to an electrical stimulus primes associative pairing of audio and electrical stimuli for dairy heifers in a virtual fencing feed attractant trial. Animals 10:217. doi: 10.3390/ani10020217
Verdon, M., Rawnsley, R., Raedts, P., and Freeman, M. (2018). The behaviour and productivity of mid-lactation dairy cows provided daily pasture allowance over 2 or 7 intensively grazed strips. Animals 8:115. doi: 10.3390/ani8070115
Wathes, D., Pollott, G., Johnson, K., Richardson, H., and Cooke, J. (2014). Heifer fertility and carry over consequences for life time production in dairy and beef cattle. Animal 8, 91–104. doi: 10.1017/S1751731114000755
Keywords: associative learning, conditioning, development, sensitive period, shock
Citation: Verdon M and Rawnsley R (2020) The Effects of Dairy Heifer Age at Training on Rate of Learning and Retention of Learning in a Virtual Fencing Feed Attractant Trial. Front. Anim. Sci. 1:618070. doi: 10.3389/fanim.2020.618070
Received: 16 October 2020; Accepted: 30 November 2020;
Published: 21 December 2020.
Edited by:
Maria José Hötzel, Federal University of Santa Catarina, BrazilReviewed by:
Ruan Rolnei Daros, Pontifical Catholic University of Parana, BrazilDaniella Moura, Campinas State University, Brazil
Copyright © 2020 Verdon and Rawnsley. This is an open-access article distributed under the terms of the Creative Commons Attribution License (CC BY). The use, distribution or reproduction in other forums is permitted, provided the original author(s) and the copyright owner(s) are credited and that the original publication in this journal is cited, in accordance with accepted academic practice. No use, distribution or reproduction is permitted which does not comply with these terms.
*Correspondence: Megan Verdon, bWVnYW4udmVyZG9uJiN4MDAwNDA7dXRhcy5lZHUuYXU=