- 1Department of Biology, Texas State University, San Marcos, TX, United States
- 2Department of Evolutionary Ecology, Plant Protection Institute, HUN-REN Center for Agricultural Research, Budapest, Hungary
The distribution of a species is best understood by examining the organism-environment interaction. Climate and anthropogenic habitat degradation, including urbanization, are salient features of the environment that can limit species distributions, especially for ectotherms. Comparative studies of the capacity of individuals to cope with rapid environmental change can help us understand the future success or failure of local populations or even the species. Studies of the glucocorticoid stress response are commonly used to understand how species cope with environmental stressors. Glucocorticoids modulate many aspects of physiological homeostasis including changes in energetic allocation and behavior. In a time of global amphibian decline the Gulf Coast Toad (Incilius nebulifer) is increasing its distribution and abundance. To understand how this species deals with thermal and urban stressors, we studied glucocorticoid regulation, hop performance, and lipid storage in I. nebulifer juveniles across nine populations that differed in average annual temperature and level of imperviousness (as an indication of urbanization). We measured corticosterone release rates at baseline, during agitation stress, and during recovery; then measured locomotor performance and whole-body lipids. We tested if I. nebulifer in hotter temperatures and more urbanized habitats exhibits elevated baseline corticosterone levels and either a reduced corticosterone stress response (“stress resistance” hypothesis) or quick post-stress recovery by negative feedback (“on again, off again” hypothesis). We also tested whether they exhibit reduced fat stores and decreased locomotor performance as costs of dealing with thermal and urban stressors. We found that I. nebulifer showed elevated baseline and agitation (stressed-induced) corticosterone release rates, and higher lipid storage with increasing urbanization. Climate had quadratic effects on these traits, such that populations living at the lowest and highest temperatures had the lowest corticosterone release rates and lipid stores, and the highest hop performance was observed in the least urbanized site at the warmest climate. Additionally, the rate of glucocorticoid recovery after agitation (negative feedback) decreased with increasing temperature and increased with increasing urbanization. These results indicate that I. nebulifer follows the “on again, off again” hypothesis in an adaptive pattern, which may help them cope with environmental change in terms of urbanization and climatic differences.
1 Introduction
Comparative population studies of physiological processes within a species with a broad climatic niche can give insight into the coping capacity of individuals to rapid environmental change and potentially the future success or failure of local populations, or even the species (Riddell et al., 2018; Smith et al., 2019). Understanding the organism-environment relationship is important for understanding a species’ distribution and how behavioral and physiological coping mechanisms help shape that distribution. For ectotherms in particular, climate and the various environmental degradations associated with anthropogenic activities, including urbanization, are notorious environmental factors that can limit species distributions (Lomolino et al., 2010). Urban areas are novel habitats that are characterized by increased amounts of impervious surfaces (e.g. pavement, concrete, and buildings), higher levels of synthetic chemicals, changes in noise levels and frequencies, increased light at night, increased ambient temperatures, and availability of anthropogenic food sources that are often of poor nutritional quality (Isaksson et al., 2018). While some species can adapt or tolerate the changes associated with urbanization, the physiological basis of their ability to cope with urban environments is less well-known (Bonier, 2023).
Studies of the glucocorticoid response to stress provide insights into how animals physiologically and behaviorally cope with environmental perturbations. At baseline levels in vertebrate animals, glucocorticoids are involved in growth, somatic maintenance, and homeostasis (Romero et al., 2009; Sapolsky et al., 2000). However, baseline levels can be elevated in response to persistent environmental stressors such as chemical contaminants and metabolic challenges associated with different temperatures (Goff et al., 2020; Shidemantle et al., 2021; Narayan et al., 2012; Eikenaar et al., 2012). At elevated, or stress-induced levels, glucocorticoids alter behavior and modulate the mobilization of energy, increasing the likelihood of surviving the stressful situation, but persistent stressors may lead to pathological outcomes (Dallman et al., 2003; de Kloet et al., 2005; Dhabhar, 2018; Wingfield and Sapolsky, 2003). Animals that adapt to environments with persistent stressors can employ different strategies to prevent pathological outcomes. The “stress resistance” hypothesis predicts a reduced glucocorticoid response to stressors (Wingfield et al., 2011), allowing the animal to adapt to the persistent stressor to the point it rarely perceives it as a stressor. Alternatively, the “on-again, off-again” hypothesis predicts a non-reduced stress response coupled with fast recovery by negative feedback (Zimmer et al., 2019). Strong negative feedback following the activation of the hypothalamus-pituitary-adrenal/interrenal (HPA/I) axis allows excess glucocorticoids to be metabolized and removed quicker, allowing a faster return to baseline levels (Herman et al., 1992; Jacobson and Sapolsky, 1991; Keller-Wood and Dallman, 1984). For example, Bókony et al. (2021) examined the glucocorticoid profiles of common toad (Bufo bufo) tadpoles from agricultural and urban ponds. They found that B. bufo copes with urbanization by showing a stronger stress response and more efficient negative feedback than tadpoles from natural ponds.
Even an adaptive glucocorticoid response to stressful environments may come at costs, as these changes should be accompanied by changes in other traits affected by stress such as energy allocation and performance, and when studied all together will give a more complete view of the stress phenotype (Lattin and Kelly, 2020; Vitousek et al., 2019; Telmeco and Gangloff, 2020). Locomotor performance and lipid storage have the potential to reveal energetic trade-offs affected by the glucocorticoid profile, with important fitness consequences (Sheridan, 1994; Vitousek et al., 2019; Wack et al., 2013). Glucocorticoids, being primary regulators of behavior and energy, influence locomotor endurance and lipid storage, thus playing a role in shaping the stress phenotype (Dallman et al., 2003; Lattin and Kelly, 2020). For example, elevated baseline glucocorticoid levels can promote fitness in metabolically demanding situations that decrease lipid storage (Jimeno et al., 2020). However, both locomotor endurance and lipid storage may also respond to stress independently of glucocorticoids (Alvarez and Nicieza, 2002; Crespi and Warne, 2015). Looking at the population variation in these responses in a species that is doing well in urban environments will help give an idea of what a stress phenotype looks like for an urban-tolerant organism (Harris et al., 2013; Winchell et al., 2016; Amdekar et al., 2018; Winchell et al., 2018).
The Gulf Coast toad (Incilius nebulifer) is native to central and eastern Texas and northeast Mexico but is undergoing range extensions to the north and east in the USA (Mendelson et al., 2015). It is hypothesized that I. nebulifer uses urban corridors to aid in its dispersal, as the range extension is strongly associated with disturbed habitats, including urbanized areas, indicating this species may be urban-tolerant. Studies in Louisiana on the effects of I. nebulifer range extension on amphibian communities show the presence of I. nebulifer tadpoles is associated with a decrease in the fitness of native amphibian tadpoles (Vogel and Pechmann, 2010). Therefore, the range extension of I. nebulifer is not only interesting as it relates to the stress-related consequences of urbanization but also as a conservation concern.
To explore how this urban-tolerant amphibian with a broad climatic niche copes with environmental change, we investigated the stress phenotype of I. nebulifer in response to climate and urbanization. To do so, we measured differences in the corticosterone (the principal glucocorticoid in amphibians) profile (baseline, stress response, and recovery), locomotor performance, and whole-body lipid storages of young juveniles of I. nebulifer, from nine populations across three latitudes in Texas. We predicted that I. nebulifer in more urbanized and hotter environments will show higher baseline corticosterone levels, and either weaker stress response or stronger negative feedback depending on whether they employ the strategy predicted by either the “stress resistance” hypothesis or the “on again, off again” hypothesis, respectively, to deal with thermal and urban stressors. Furthermore, we predicted that both lipid storage and locomotor performance would be decreased in more urbanized and hotter sites because of the trade-offs involved in dealing with the metabolic demands and stressors of these environments.
2 Materials and methods
2.1 Field collection
We sampled nine populations in early summer 2021 from three latitudes approximately 485 km apart, across Texas, USA under the assumption that temperature would decrease with increasing latitude. At each latitude, we sampled three populations that varied in levels of urbanization, corresponding roughly to the lowest, median, and highest range of urbanization intensity across the habitats occupied by the species (Table 1). Notably, although adults can be found in more urbanized sites than the ones we sampled (iNaturalist), breeding and young metamorphs are unlikely to be found in highly impervious areas where very little standing water persists relatively undisturbed for several weeks, especially during summers in Texas. In the field at each site, we collected recently metamorphosed I. nebulifer (20-24 individuals per population). We identified recently metamorphosed (< 2 months after completing metamorphosis) juvenile toads by size (< 2 g) and by proximity to water; average body mass was 0.17 g (range: 0.02 – 1 g).
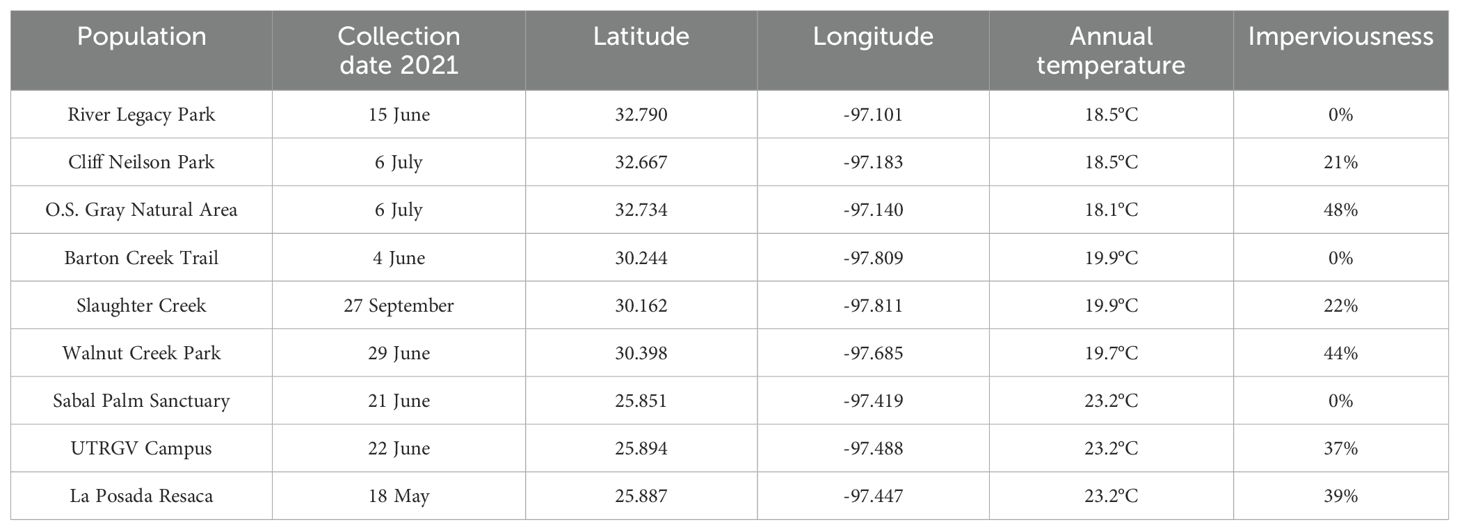
Table 1. Environmental variables and collection dates for each site where Incilius nebulifer were collected.
For each site, we determined the urbanization level from measures of imperviousness taken from the USGS (United States Geological Survey) national landcover database (NLCD2019 at ~ 30m resolution) and the average annual temperature of sites from Worldclim data (version 2.1 at ~ 1km resolution; Table 1). Average annual temperature allows for a simple, easy-to-interpret proxy of climatic differences at the landscape scale, which is appropriate for our study because I. nebulifer is active all year throughout most of its distribution (Mendelson et al., 2015). Microclimate data would potentially improve model performance over macroclimate data. However, we had no information on any geographic variation in the microhabitat use of I. nebulifer. Additionally, macroclimate data have been shown to be sufficient for predicting mechanistic responses to broad-scale environmental variables (Sanabria and Quiroga, 2019; Pateman et al., 2016). Using ArcGIS (ArcMap version 10.8.2), a 500-meter radius circle was made around each site using the buffer tool. The circles were layered over rasters of average annual temperature and imperviousness, and the average value of pixels with their center inside the circle was used as the value for that location.
2.2 Hormone field collection
Water-borne baseline, agitation, and recovery corticosterone release rates were taken on-site following an established protocol between 0700-1600h (Monroe et al., 2023). Briefly, beakers were filled with 30 ml of spring water for hormone collection. A perforated plastic insert was added to the beaker for moving juveniles to new beakers. The juveniles were then placed into the plastic insert and a mesh lid was added on top to prevent the juveniles from escaping or climbing out of the water. Juveniles were collected, placed in the first beaker setup within 30 min of collection, placed in a cardboard box with dividers, and left alone in the shade for 1h. Juveniles were then transferred using the plastic insert to a new beaker of 30 ml fresh spring water for collection of agitation levels. Juveniles were agitated by placing beakers in a cardboard box with dividers and gently swirling and rocking the box for 1 min every 3 min for 1 h. Juveniles were then transferred using the plastic insert to a third beaker of 30 ml fresh spring water and left alone in the shade for 1 h for collection of recovery levels. After hormone collection, we moved each water sample into a sterile 50 ml falcon tube, temporarily stored them on ice during transportation, then stored them at -20°C until extraction. All beakers and inserts were cleaned with 95% ethanol and rinsed with distilled water before use. Gloves were always worn during hormone collection to prevent contamination.
2.3 Extraction and quantification of hormones
Hormones were extracted from the water using C18 solid-phase extraction columns (SepPak Vac3 cc/500 mg; Waters, Inc., Milford, MA, USA) under vacuum pressure following Forsburg et al. (2019). We primed the columns with 4 ml methanol and 4 ml distilled water. After extraction, we eluted columns with 4 ml methanol into borosilicate vials. The methanol was evaporated under a gentle stream of nitrogen gas while in a 37°C water bath. We re-suspended the residue in 500 µL of 5% ethanol diluted with enzyme-immunoassay (EIA) buffer (provided by Cayman Chemicals Inc., Ann Arbor, MI, USA). After resuspension, we shook samples for 2 h to fully resuspend the residue. This suspension value ensured that corticosterone values were within the assay range of the kit. Corticosterone concentrations were measured in duplicate for all samples with an EIA kit (Cayman Chemicals Inc.) on a microplate absorbance reader (BioTek ELX800) set to 405 nm. Inter-plate coefficient of variation (CV) was 12.5% (18 plates; intra-plate CV range: 1.01–12.03). Four samples were lost while extracting hormones. We expressed corticosterone release rate for each hourly sample as corticosterone quantity divided by body mass (pg/g). All corticosterone values were then natural log transformed for statistical analyses. We calculated the magnitude of the stress response to agitation as: 100 × (agitation – baseline)/baseline untransformed, mass adjusted corticosterone. We measured negative feedback as the relative change from agitation to recovery levels as: 100 × (agitation – recovery)/agitation untransformed, mass adjusted corticosterone.
2.4 Locomotor performance
After hormone collection, we placed juveniles in individual 500 ml deli cups with a damp paper towel and a perforated lid for transporting to and housing at Texas State University, San Marcos, TX. Locomotor performance trials were modified from Llewelyn et al. (2010) to accommodate juvenile toads. In summary, we acclimated and fasted juveniles for 24 h in the animal care facility, maintained at 24 ± 2°C, before starting locomotor trials. Trials were performed in the same room where juveniles were housed, between 0900-1200h which is normal activity time for these toads. Trials consisted of placing juveniles individually into a 2-meter-long track made from a plastic rain gutter (13 cm wide × 10 cm deep) with transparent plastic ends to encourage linear travel. The bottom of the track was lined with damp paper towels to maintain moisture levels and provide traction. We facilitated travel by holding a small paintbrush behind the toads. When movement stopped, toads were encouraged to continue moving by gently tapping on the hindlegs and urostyle with the small paintbrush. No pressure was applied to the toads, just the tactile sensation of being touched was used to facilitate movement. When juveniles reached the end of the track, we turned them around by gently tapping their snout with the paintbrush. Locomotor performance was determined as the distance traveled in 10 min. In preliminary trials after one full hour of hopping, toads did not exhibit typical signs of exhaustion (not moving after 10 taps on the urostyle) most likely due to their small size allowing for quick recovery. However, speed greatly reduced after ~10 minutes so we used distance traveled in 10 minutes as our measure of locomotor performance.
2.5 Whole body lipid extraction
After the locomotor performance trials, we euthanized juveniles in an ice bath and dried them at 60°C until they attained a constant weight (36-48 h). We then placed each sample in a lipid-free filter paper (Whatman™ Grade 602H) envelope made by cutting the filter paper into a 4 × 6 cm rectangle and folding the edges over to make a 2 × 2 square envelope. Samples were sealed inside by stapling the open end of the envelope. Preliminary trials showed that staples did not change weight during the extraction process. Because of the small volume of the samples, we did not homogenize them to prevent any loss of material.
We labeled the filter paper envelopes using a graphite pencil and placed the filter paper envelopes into a Soxhlet extraction setup. For efficiency, we processed samples in batches of 12. We used petroleum ether as our extraction solvent and heated it to 45°C. This resulted in a cycle time of about 15 min. We extracted the samples for 6 h, allowed the remaining petroleum ether to evaporate off the samples, and placed the samples in a 60°C dryer for 1 h.
We weighed the samples after initial drying (S), after placing them in the filter paper envelope (X), and again after the lipid extraction and final drying (T). The weight of the filter paper and staple (FP) was calculated by subtracting initial dry weight from the total weight before lipid extraction (X – S; note that we used lipid-free filter paper so there was no weight loss due to the filter paper during lipid extraction). We determined the total lipid content as the percent of total body weight lost during the extraction process using the following formula: 100 × (1-(T-FP))/S.
2.6 Statistical analysis
All statistical analyses were performed using the statistical software R (version 4.3.2) through the interface R studio (version 19.1.3) using packages “nlme” and “emmeans”. Graphs were made using the package “ggplot2”. We used linear mixed-effects models and evaluated each model’s fit to the statistical assumptions by graphical inspection of data distributions and model residuals. These diagnostics showed that 1) there was no multi-collinearity between temperature and urbanization, 2) outlying data points were not influential, 3) homoscedasticity was not met, so we allowed for heterogeneous variances across sites in all models using the “varIdent” function.
First, we tested whether log-transformed corticosterone release rates responded to agitation and then recovered, including all three hormone samples of each individual as repeated measures. We ran this model first to test the overall effect of sample type (i.e., baseline, agitation, recovery) across all populations, adding site and animal ID as nested random factors. Then to evaluate the hormonal changes within each site, we included the interaction between sample type and site as fixed factors, and animal ID as a random factor. From the latter model, significance levels were adjusted to reduce the probability of type I error for multiple pairwise comparisons between sample types by applying the false discovery rate (FDR; Pike, 2011) method to all 18 P-values (i.e., 9 sites × 2 comparisons: one for stress response and one for recovery).
Second, we evaluated the effects of average temperature and urbanization (using both as numeric variables) on all measured response variables with site as the random intercept. Based on model-fit plots, we added the quadratic effect of temperature into all models. Locomotor performance (hop distance; mm) was divided by snout-to-urostyle length (cm) to control for differences in body size. The locomotor performance model had poor model fit without the interaction between temperature and urbanization, so we added the interaction into this model only. To evaluate our prediction for the corticosterone response to stress, we analyzed both the absolute level of stress-induced corticosterone release rate and the magnitude of the stress response (i.e. change from baseline to agitation), as both can be informative when comparing different populations (Bókony et al., 2021; Vitousek et al., 2018). By the same logic, we evaluated our prediction for the negative feedback by analyzing both the absolute level of post-agitation recovery corticosterone release rate and the rate of the negative feedback (i.e. change from agitation to recovery).
3 Results
Incilius nebulifer showed a significant stress response and recovery both within and across populations (Table 2). This indicates we were able to effectively sample the corticosterone stress profile. Climate had a significant quadradic effect on corticosterone release rates in all three sample types, meaning release rates were highest at the mid-latitude populations with an average annual temperature of ~20°C. Baseline and agitation (but not recovery) corticosterone release rates increased with increasing urbanization as well (Table 3; Figures 1A, B). The magnitude of stress response was not significantly related to temperature or urbanization (Table 3), but the rate of negative feedback decreased with increasing temperature and increased with increasing urbanization (Table 3).
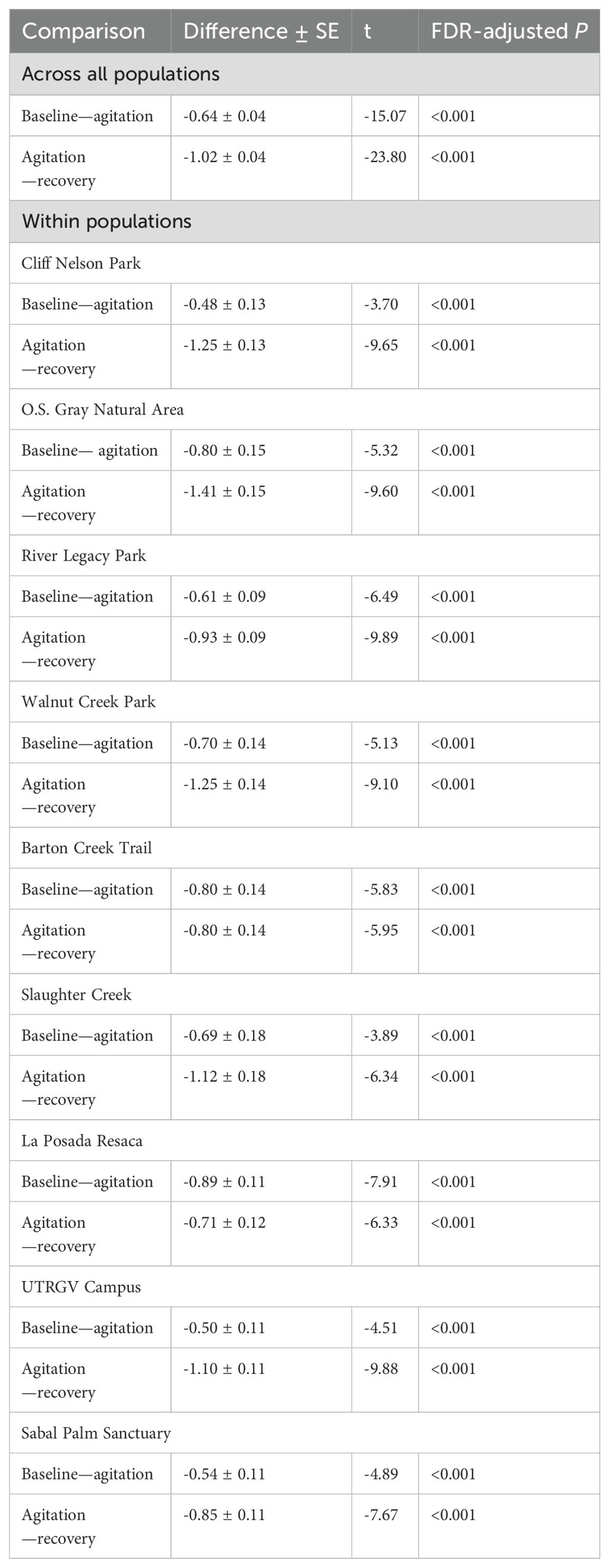
Table 2. Pairwise comparisons of log transformed corticosterone release rates (pg/g/h) between sample types for Incilius nebulifer.
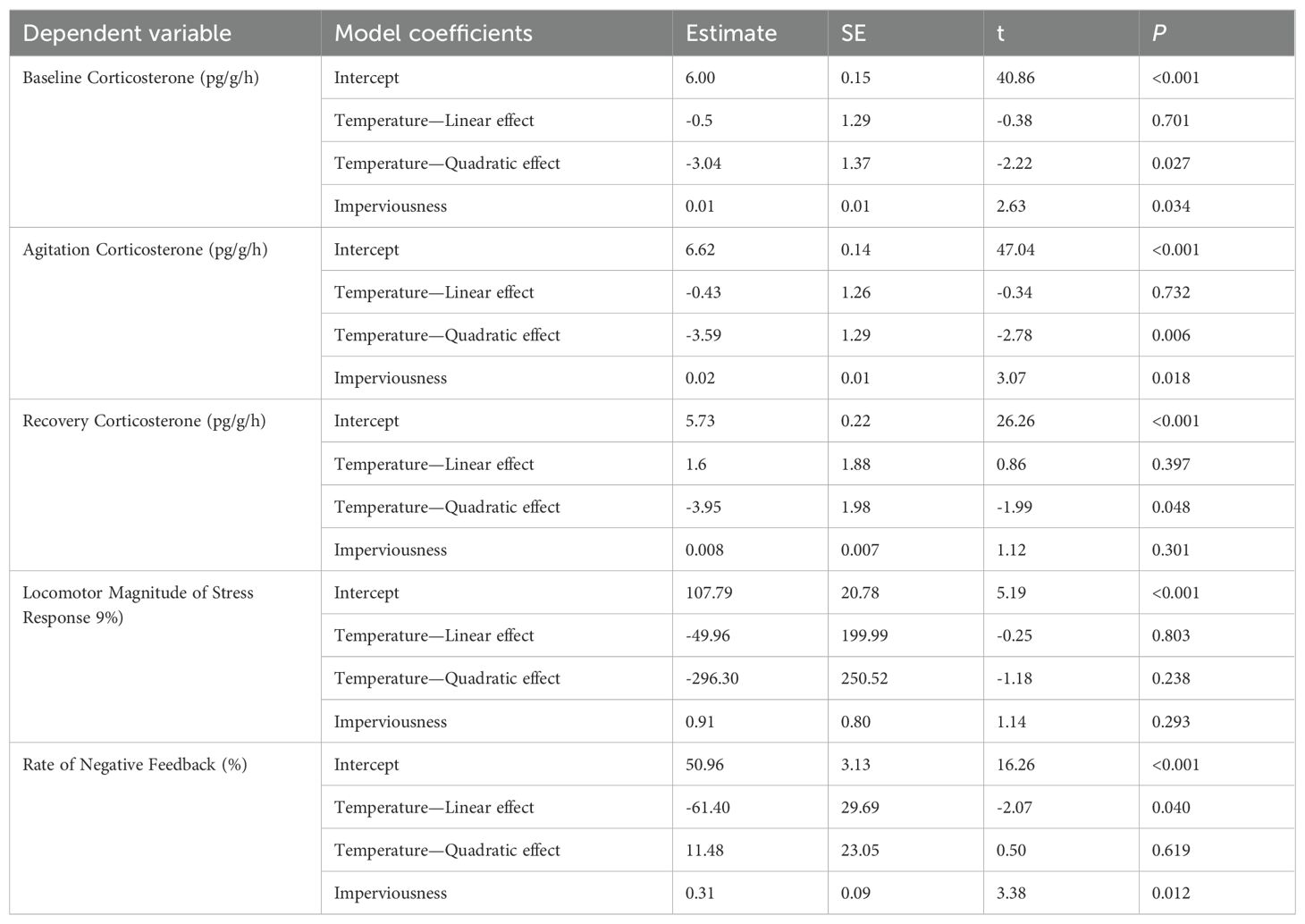
Table 3. Mixed-effects model results for measures of the corticosterone stress profile for Incilius nebulifer.
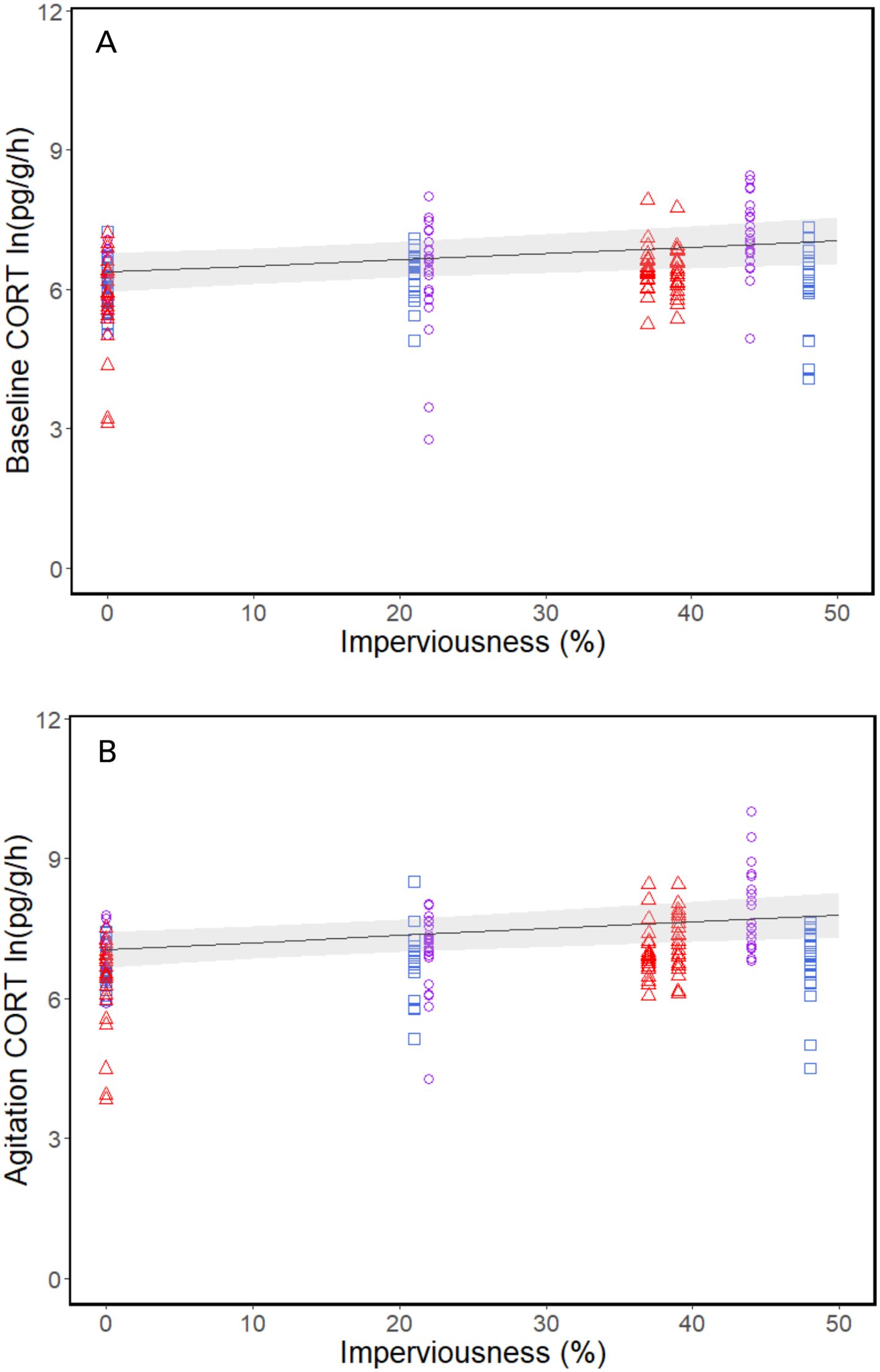
Figure 1. Log transformed corticosterone release rates (pg/g/h) of Incilius nebulifer across levels of urbanization (% imperviousness) at (A) baseline, and (B) agitation. Colors indicate latitude and average annual temperature (blue squares= high latitude, ~18.5°C; purple circles= mid latitude, ~20°C; red triangles= low latitude, ~23°C). The trend line is the linear relationship between corticosterone release rates and urbanization with the gray shading representing 95% confidence bands.
Climate had a quadratic effect on whole-body lipids (although this effect was just above the significance threshold of p=0.05), with highest lipid content being at the mid-latitude populations with an average annual temperature of ~20°C (Table 4; Figure 2). Urbanization had a linear relationship with whole-body lipids with lipid content increasing with increasing urbanization (Table 4; Figure 2). Locomotor performance was related to a significant interaction between climate and urbanization (Table 4; Figure 3): urbanization had a negative relationship with locomotor performance but only in the populations at the highest (~23°C) average annual temperature (Figure 3).
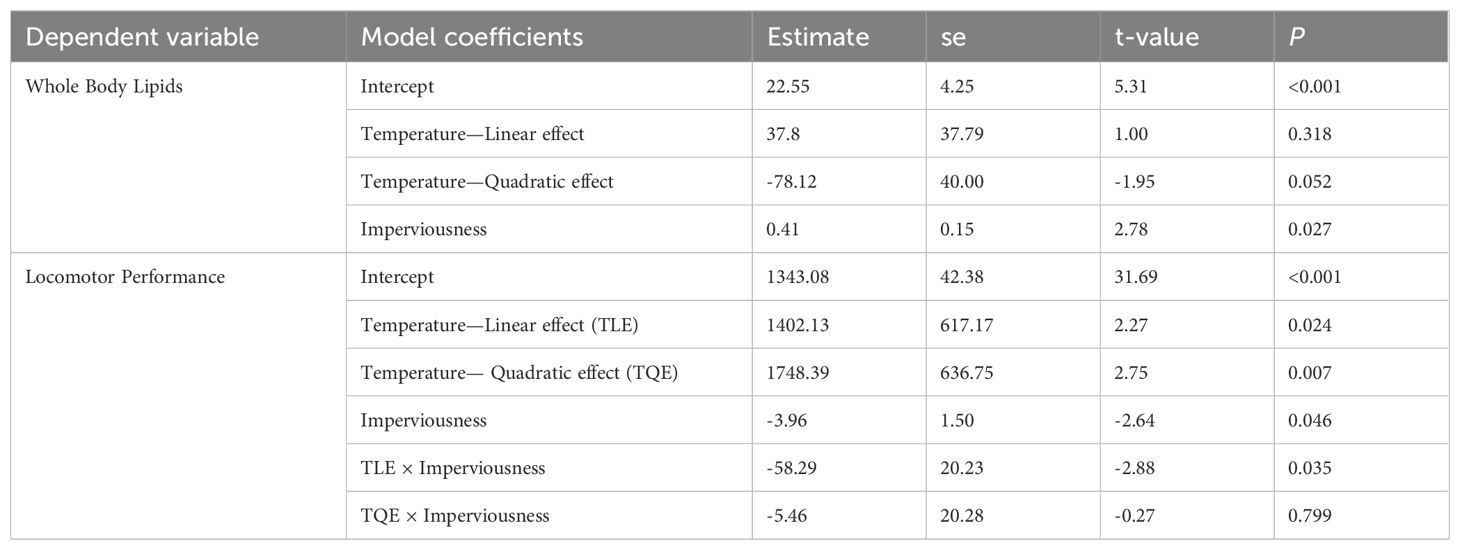
Table 4. Mixed-effects model results for whole body lipids (%) and locomotor performance (hop distance expressed as multiple of snout-to-urostyle length) for Incilius nebulifer.
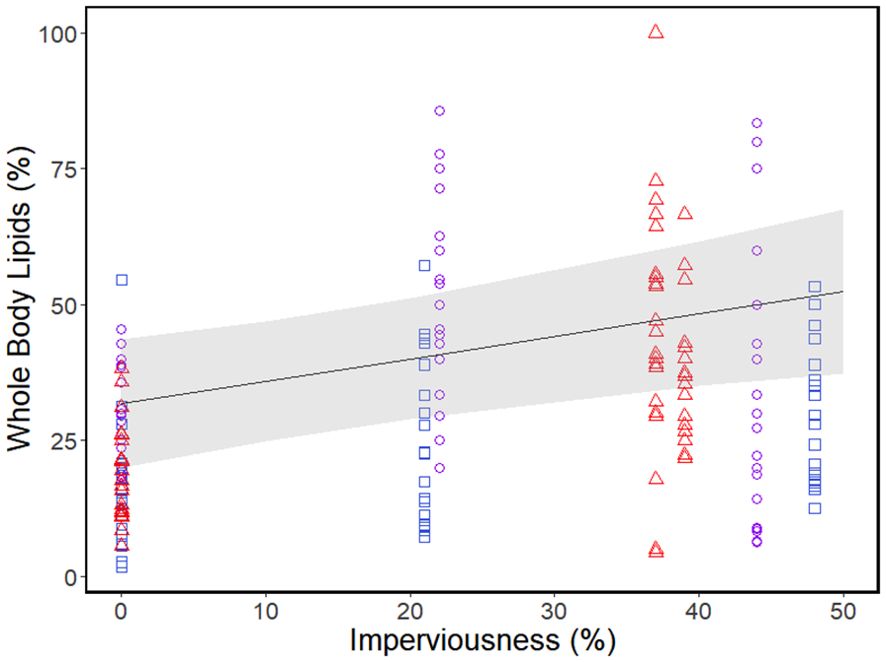
Figure 2. Whole body lipid stores of Incilius nebulifer across levels of urbanization (% imperviousness). Colors indicate latitude and average annual temperature (blue squares=high latitude, ~18.5°C; purple circles=mid latitude, ~20°C; red triangles=low latitude, ~23°C). The trend line is the linear relationship between whole-body lipid stores and urbanization with the gray shading representing 95% confidence bands.
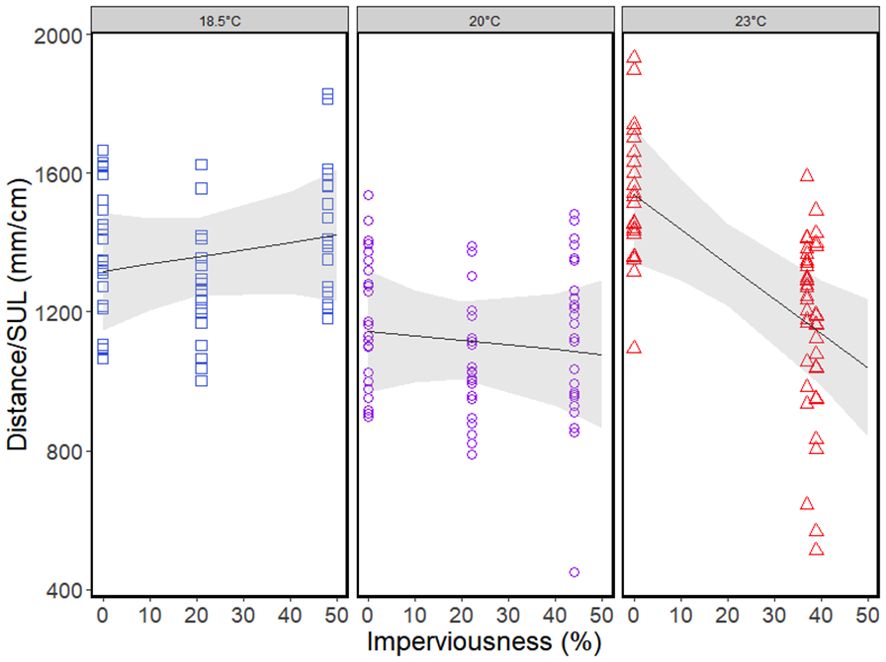
Figure 3. Locomotor performance (hop distance expressed as multiple of snout-to-urostyle length) of Incilius nebulifer across levels of urbanization (% imperviousness). Colors indicate latitude and average annual temperature (blue squares=high latitude, ~18.5°C; purple circles=mid latitude, ~20°C; red triangles=low latitude, ~23°C). The trend lines are the linear relationships between locomotor performance and urbanization with the gray shading representing 95% confidence bands. Temperatures are separated into three categories to visualize the interaction between average annual temperature and urbanization.
4 Discussion
Using physiological stress markers is central to understanding the organism-environment interaction and for understanding species distributions. Corticosterone mediates metabolic processes, and its levels often increase in metabolically challenging times or areas (McCall, 2019). However, in chronically stressful environments vertebrates may downregulate their stress response or increase the rate of negative feedback as this may reduce the detrimental effects associated with chronic stress (Bókony et al., 2021; Kolonin et al., 2022; Shidemantle et al., 2021). Furthermore, energetic trade-offs of dealing with metabolic challenges and other stressors may limit lipid stores and locomotor performance. We examined the stress phenotype of Incilius nebulifer across two environmental gradients (climate and urbanization) to test these predictions. To summarize our results, we found that populations of I. nebulifer had the highest baseline, stress-induced, and recovery corticosterone release rates and highest amounts of lipid stores at mid-latitude populations with ca. 20°C average annual temperature, while the rate of negative feedback decreased with increasing temperature. Baseline, agitation, and recovery corticosterone release rates, as well as the rate of negative feedback and lipid stores increased with increasing urbanization (imperviousness). Locomotor performance decreased with increasing urbanization but only across the low-latitude populations with ca. 23°C average annual temperature.
For urbanization, our results support our predictions for glucocorticoid regulation. Specifically, toad populations in more urbanized sites had higher baseline and agitation corticosterone release rates and faster recovery rates. These findings are strikingly similar to what we found earlier in another toad species (Bókony et al., 2021). The higher baseline levels may represent an adaptation to deal with the metabolic challenges associated with urban habitats, including the urban heat island effect (Gartland, 2008; Brans et al., 2018) and combating the effects of environmental contaminants and other urban stressors (Bókony et al., 2021; Gabor et al., 2018). The higher stress-induced levels of corticosterone indicate that toads do not display “stress resistance” in urban habitats. Instead, their non-diminished stress response allows them to adequately deal with stressful stimuli. Furthermore, higher stress-induced corticosterone levels may also have a preparative effect that allows for a more efficient response to subsequent stressors when the likelihood of unpredictable stressors is high (Romero, 2002; Sapolsky et al., 2000) and may increase the threshold of severity necessary for the subsequent stimuli to become stressors (Vera et al., 2017). Finally, the higher rate of recovery by negative feedback in more urbanized sites suggests that toads conform to the “on again off again” hypothesis”, which is likely adaptive, because the ability to downregulate the stress response quickly post-stress may protect the animals from chronic elevation of corticosterone levels and their detrimental consequences for early-life growth and development (Crespi and Warne, 2013; Romero et al., 2009; Wingfield and Sapolsky, 2013).
In contrast, our results did not support our predictions for the effects of urbanization on lipid stores and locomotor performance. We expected negative effects on these traits due to energetic trade-offs, but instead, we found a positive effect of urbanization on the percentage of body fat. This finding is in parallel with higher corticosterone release rates and faster negative feedback at more urbanized sites. Furthermore, locomotor performance did not vary with urbanization, except for a negative relationship at the warmest climate. A limitation of our measure of locomotor performance is that we were not able to measure endurance to exhaustion. However, our measure likely provided an indication of locomotor performance under a simulated predator attack, which is an ecologically important trait that relates to glucocorticoid regulation. Put together, these findings suggest that the stress phenotype of I. nebulifer in response to urbanization is adaptive, such that hormonally dealing with the stressors of more urbanized habitats does not come at the cost of lower lipid reserves or reduced locomotion performance, except to some extent at the lowest latitudes of its range. While there is no clear consensus across species on what a coping endocrine response to urbanization looks like (Bonier, 2023), the parallel trends between increased lipid storage, increased baseline and agitation corticosterone release rates, and more efficient negative feedback indicate that the altered glucocorticoid regulation of I. nebulifer in urbanized habitats might help to maintain body condition, which increases survival (Scott et al., 2007). However, further studies and experimental manipulations are needed to test whether the lipid response is causally related to the glucocorticoid response.
Climatic differences across our study sites had quadratic effects on all three corticosterone release rates as well as on lipid stores and locomotor performance. Specifically, corticosterone levels and lipid stores were highest in mid-latitude populations within the range that we studied. One potential explanation for this may be a down-regulation of the corticosterone stress response along the northern invasion front (Kosmala et al., 2020) essentially representing a switch to a stress resistance strategy to meet the metabolic demands of a different climate (Eikenaar et al., 2012). The reduced lipid levels might be due to an increased growth rate to compensate for the reduced growing season of the higher latitude. For example, Dahl et al. (2012) found some support for the hypothesis that in colder environments tadpoles down-regulate their stress response as this reduces the negative effects on growth in areas with a shorter growing season. On the other hand, the decrease in corticosterone and lipid levels from the mid-latitude to the low-latitude populations is most likely due to a decrease in growth demands and energy storage needs in warmer environments. The distribution of I. nebulifer extends to southern Mexico, and their developmental thermal tolerances are between 18°C and 38°C (Ballinger and McKinney, 1966), suggesting they prefer or at least can well tolerate high temperatures (Mendelson et al., 2015). This might also explain the higher locomotor performance of the southern populations in our study. In contrast, the increase in locomotor performance from the mid-latitude to the northern climates might be related to selection for higher activity at the invasion front, as has been found in other species (Llewelyn et al., 2010).
How species cope with urbanization has been a major topic of consideration (Alberti et al., 2020; Cordier et al., 2021; Injaian et al., 2020). Similarly, ongoing climate change is of great concern, and already existing climatic adaptations across geographic gradients have been increasingly studied to predict future outcomes (Urban et al., 2013). However, organisms are simultaneously affected by urbanization and climate change, which can interact, resulting in complex outcomes (Urban et al., 2024). In our study, such an interaction explained the variation in locomotor performance, as urbanization had a negative effect on hop distance only in the warmest climate we investigated. This suggests that the physiological strategies employed by this urban-tolerant species, specifically the higher corticosterone and lipid levels of urban populations, might come at the expense of locomotor performance under certain climatic conditions, such as the hot Texas summers. This finding highlights the need for more studies, not only in I. nebulifer, specifically designed for testing the interactions between the effects of climatic changes and anthropogenic habitat alterations. Understanding the nature, causes, and consequences of climate-dependent effects of urbanization across the diversity of life is one of the most important challenges for the protection of biodiversity and ecosystems (Urban et al., 2024).
There are several limitations to our study that should be borne in mind when interpreting the results. For feasibility, we sampled only three populations from each of three latitudes, and we assumed that the effects of urbanization would be linear across the sampled gradient of imperviousness (i.e. from zero to 48%). While this is clearly a step forward compared to earlier studies that investigated inter-population variation in glucocorticoid regulation either across latitudinal (e.g. Breuner et al., 2003; Dahl et al., 2012; Pravosudov et al., 2004) or urbanization gradient (e.g. Bókony et al., 2012; Bókony et al., 2021; Gabor et al., 2018; Kolonin et al., 2022) or both gradients but with a smaller number of sites (e.g. Bonier et al., 2006), several questions have remained unanswered. First, we cannot unambiguously separate the effects of climate from the effects of selection acting during range extension, because our coolest-climate populations were also the ones at the northern invasion front. Further studies should try to disentangle the effects of climatic effects of distribution shifts and other selection pressures of invasion on “coping styles”. Second, we assumed that gross climatic differences between latitudes reflect the temperatures the toadlets are exposed to, and we did not measure local microhabitat temperatures. Future studies should investigate whether juvenile toads adjust their microhabitat choice along gradients of temperature and urbanization to mitigate the trade-offs posed by suboptimal climates. Third, we assumed that the effects of urbanization would be linear; however, this is not always the case (Miltner et al., 2004). Although our analyses did not suggest non-linear effects of urbanization, to validate this it will be necessary to sample the gradient of urbanization at a higher resolution at each climate. Altogether, we call for more detailed studies for understanding the role played by endocrine flexibility in physiological and behavioral adaptations to urbanization and climatic changes.
Data availability statement
The raw data supporting the conclusions of this article will be made available by the authors, without undue reservation.
Ethics statement
The animal study was approved by Institutional Animal Care and Use Committee. The study was conducted in accordance with the local legislation and institutional requirements.
Author contributions
DM: Conceptualization, Data curation, Formal analysis, Funding acquisition, Investigation, Methodology, Project administration, Resources, Validation, Visualization, Writing – original draft, Writing – review & editing. VB: Data curation, Formal analysis, Visualization, Writing – original draft, Writing – review & editing. CG: Conceptualization, Methodology, Project administration, Resources, Supervision, Writing – original draft, Writing – review & editing.
Funding
The author(s) declare financial support was received for the research, authorship, and/or publication of this article. This research was funded by a Texas State University graduate fellowship, a PIER research grant to DM, and a Texas ECOLAB grant to DM and CG. VB was supported by the National Research, Development and Innovation Office of Hungary (K135016).
Acknowledgments
We thank D. Davis for help with field collection, the Sabal Palm Sanctuary for allowing us access to their refuge, and the GASP lab for help with lab work and comments on this manuscript. Finally we thank two reviewers for their helpful comments.
Conflict of interest
The authors declare that the research was conducted in the absence of any commercial or financial relationships that could be construed as a potential conflict of interest.
Publisher’s note
All claims expressed in this article are solely those of the authors and do not necessarily represent those of their affiliated organizations, or those of the publisher, the editors and the reviewers. Any product that may be evaluated in this article, or claim that may be made by its manufacturer, is not guaranteed or endorsed by the publisher.
References
Alberti M., Palkovacs E. P., Roches S. D., Meester L. D., Brans K. I., Govaert L., et al. (2020). The complexity of urban eco-evolutionary dynamics. BioScience 70, 772–793. doi: 10.1093/biosci/biaa079
Alvarez D., Nicieza A. G. (2002). Effects of temperature and food quality on anuran larval growth and metamorphosis. Funct. Ecol. 16, 640–648. doi: 10.1046/j.1365-2435.2002.00658.x
Amdekar M. S., Kakkar A., Thaker M. (2018). Measures of health provide insights into the coping strategies of urban lizards. Front. Ecol. Evol. 6. doi: 10.3389/fevo.2018.00128
Ballinger R. E., McKinney C. O. (1966). Developmental temperature tolerance of certain anuran species. J. Exp. Zoology 161, 21–28. doi: 10.1002/jez.1401610104
Bókony V., Seress G., Nagy S., Lendvai Á.Z., Liker A. (2012). Multiple indices of body condition reveal no negative effect of urbanization in adult house sparrows. Landscape Urban Plann. 104, 75–84. doi: 10.1016/j.landurbplan.2011.10.006
Bókony V., Ujhegyi N., Hamow K.Á., Bosch J., Thumsová B., Vörös J., et al. (2021). Stressed tadpoles mount more efficient glucocorticoid negative feedback in anthropogenic habitats due to phenotypic plasticity. Sci. Total Environ. 753, 141896. doi: 10.1016/j.scitotenv.2020.141896
Bonier F. (2023). Future directions in urban endocrinology – The effects of endocrine plasticity on urban tolerance. Mol. Cell. Endocrinol. 565, 111886. doi: 10.1016/j.mce.2023.111886
Bonier F., Martin P. R., Sheldon K. S., Jensen J. P., Foltz S. L., Wingfield J. C. (2006). Sex-specific consequences of life in the city. Behav. Ecol 18 (1), 121–129. doi: 10.1093/beheco/arl050
Brans K. I., Engelen J. M. T., Souffreau C., De Meester L. (2018). Urban hot-tubs: Local urbanization has profound effects on average and extreme temperatures in ponds. Landscape and Urban Planning 176, 22–29. doi: 10.1016/j.landurbplan.2018.03.013
Breuner C. W., Orchinik M., Hahn T. P., Meddle S. L., Moore I. T., Owen-Ashley N. T., et al. (2003). Differential mechanisms for regulation of the stress response across latitudinal gradients. Am. J. Physiology-Regulatory Integr. Comp. Physiol. 285, R594–R600. doi: 10.1152/ajpregu.00748.2002
Cordier J. M., Aguilar R., Lescano J. N., Leynaud G. C., Bonino A., Miloch D., et al. (2021). A global assessment of amphibian and reptile responses to land-use changes. Biol. Conserv. 253, 108863. doi: 10.1016/j.biocon.2020.108863
Crespi E. J., Warne R. W. (2013). Environmental conditions experienced during the tadpole stage alter post-metamorphic glucocorticoid response to stress in an amphibian. Integr. Comp. Biol. 53, 989–1001. doi: 10.1093/icb/ict087
Dahl E., Orizaola G., Winberg S., Laurila A. (2012). Geographic variation in corticosterone response to chronic predator stress in tadpoles: Geographical variation in corticosterone response. J. Evolutionary Biol. 25, 1066–1076. doi: 10.1111/j.1420-9101.2012.02493.x
Dallman M. F., Akana S. F., Laugero K. D., Gomez F., Manalo S., Bell M. E., et al. (2003). A spoonful of sugar: Feedback signals of energy stores and corticosterone regulate responses to chronic stress. Physiol. Behav. 79, 3–12. doi: 10.1016/S0031-9384(03)00100-8
de Kloet E. R., Joëls M., Holsboer F. (2005). Stress and the brain: From adaptation to disease. Nat. Rev. Neurosci. 6, 463–475. doi: 10.1038/nrn1683
Dhabhar F. S. (2018). The short-term stress response – Mother nature’s mechanism for enhancing protection and performance under conditions of threat, challenge, and opportunity. Front. Neuroendocrinol. 49, 175–192. doi: 10.1016/j.yfrne.2018.03.004
Eikenaar C., Jusak J., Escallón C., Moore I. T. (2012). Variation in testosterone and corticosterone in amphibians and reptiles: relationships with latitude, elevation, and breeding season length. Am. Nat. 180, 642–654. doi: 10.1086/667891
Forsburg Z. R., Goff C. B., Perkins H. R., Robicheaux J. A., Almond G. F., Gabor C. R. (2019). Validation of water-borne cortisol and corticosterone in tadpoles: Recovery rate from an acute stressor, repeatability, and evaluating rearing methods. Gen. Comp. Endocrinol. 281, 145–152. doi: 10.1016/j.ygcen.2019.06.007
Gabor C. R., Davis D. R., Kim D. S., Zabierek K. C., Bendik N. F. (2018). Urbanization is associated with elevated corticosterone in Jollyville Plateau salamanders. Ecol. Indic. 85, 229–235. doi: 10.1016/j.ecolind.2017.10.047
Gartland L. (2008). Heat islands: Understanding and mitigating heat in urban areas (London: Earthscan).
Goff C. B., Walls S. C., Rodriguez D., Gabor C. R. (2020). Changes in physiology and microbial diversity in larval ornate chorus frogs are associated with habitat quality. Conserv. Physiol. 8. doi: 10.1093/conphys/coaa047
Harris S. E., Munshi-South J., Obergfell C., O’Neill R. (2013). Signatures of rapid evolution in urban and rural transcriptomes of white-footed mice (Peromyscus leucopus) in the new york metropolitan area. PloS One 8, e74938. doi: 10.1371/journal.pone.0074938
Herman J. P., Cullinan W. E., Young E. A., Akil H., Watson S. J. (1992). Selective forebrain fiber tract lesions implicate ventral hippocampal structures in tonic regulation of paraventricular nucleus corticotropin-releasing hormone (CRH) and arginine vasopressin (AVP) mRNA expression. Brain Res. 592, 228–238. doi: 10.1016/0006-8993(92)91680-D
Injaian A. S., Francis C. D., Ouyang J. Q., Dominoni D. M., Donald J. W., Fuxjager M. J., et al. (2020). Baseline and stress-induced corticosterone levels across birds and reptiles do not reflect urbanization levels. Conserv. Physiol. 8, coz110. doi: 10.1093/conphys/coz110
Isaksson C., Rodewald A. D., Gil D. (2018). Editorial: behavioral and ecological consequences of urban life in birds. Front. Ecol. Evol. 6. doi: 10.3389/fevo.2018.00050
Jacobson L., Sapolsky R. (1991). The role of the hippocampus in feedback regulation of the hypothalamic-pituitary-adrenocortical axis*. Endocrine Rev. 12, 118–134. doi: 10.1210/edrv-12-2-118
Jimeno B., Prichard M. R., Landry D., Wolf C., Larkin B., Cheviron Z., et al. (2020). Metabolic rates predict baseline corticosterone and reproductive output in a free-living passerine. Integr. Organismal Biol. 2. doi: 10.1093/iob/obaa030
Keller-Wood M. E., Dallman M. F. (1984). Corticosteroid inhibition of ACTH secretion*. Endocrine Rev. 5, 1–24. doi: 10.1210/edrv-5-1-1
Kolonin A. M., Bókony V., Bonner T. H., Zúñiga-Vega J. J., Aspbury A. S., Guzman A., et al. (2022). Coping with urban habitats via glucocorticoid regulation: physiology, behavior, and life history in stream fishes. Integr. Comp. Biol. 62, 90–103. doi: 10.1093/icb/icac002
Kosmala G. K., Brown G. P., Shine R. (2020). Laid-back invaders: Cane toads (Rhinella marina) down-regulate their stress responses as they colonize a harsh climate. Global Ecol. Conserv. 24, e01248. doi: 10.1016/j.gecco.2020.e01248
Lattin C. R., Kelly T. R. (2020). Glucocorticoid negative feedback as a potential mediator of trade-offs between reproduction and survival. Gen. Comp. Endocrinol. 286, 113301. doi: 10.1016/j.ygcen.2019.113301
Llewelyn J., Phillips B. L., Alford R. A., Schwarzkopf L., Shine R. (2010). Locomotor performance in an invasive species: Cane toads from the invasion front have greater endurance, but not speed, compared to conspecifics from a long-colonized area. Oecologia 162, 343–348. doi: 10.1007/s00442-009-1471-1
Lomolino M. V., Riddle B. R., Whittaker R. J., Brown J. H. (2010). Biogeography. 4th ed (Massachusets: Sinauer Associates, Inc).
McCall A. L. (2019). “Glucose Transport,” in Stress: Physiology, Biochemistry, and Pathology (Vancouver: Elsevier), 293–307. doi: 10.1016/B978-0-12-813146-6.00022-9
Mendelson J. R. I., Kinsey C., Murphy J. B. (2015). A review of the biology and literature of the Gulf Coast Toad (Incilius nebulifer), native to Mexico and the United States. Zootaxa 3974, 517. doi: 10.11646/zootaxa.3974.4.4
Miltner R. J., White D., Yoder C. (2004). The biotic integrity of streams in urban and suburbanizing landscapes. Landscape Urban Plann. 69, 87–100. doi: 10.1016/j.landurbplan.2003.10.032
Monroe D. J., Barny L. A., Wu A., Minbiole K. P. C., Gabor C. R. (2023). An integrated physiological perspective on anthropogenic stressors in the Gulf coast toad (Incilius nebulifer). Front. Ecol. Evol. 11. doi: 10.3389/fevo.2023.1112982
Narayan E. J., Cockrem J. F., Hero J.-M. (2012). Effects of temperature on urinary corticosterone metabolite responses to short-term capture and handling stress in the cane toad (Rhinella marina). Gen. Comp. Endocrinol. 178, 301–305. doi: 10.1016/j.ygcen.2012.06.014
Pateman R. M., Thomas C. D., Hayward S. A. L., Hill J. K. (2016). Macro- and microclimatic interactions can drive variation in species‘ habitat associations. Global Change Biol. 22, 556–566. doi: 10.1111/gcb.2016.22.issue-2
Pike N. (2011). Using false discovery rates for multiple comparisons in ecology and evolution. Methods Ecol. Evol. 2, 278–282. doi: 10.1111/j.2041-210X.2010.00061.x
Pravosudov V. V., Kitaysky A. S., Wingfield J. C., Clayton N. S. (2004). No latitudinal differences in adrenocortical stress response in wintering black-capped chickadees (Poecile atricapilla). Comp. Biochem. Physiol. Part A: Mol. Integr. Physiol. 137, 95–103. doi: 10.1016/s1095-6433(03)00264-2
Riddell E. A., Odom J. P., Damm J. D., Sears M. W. (2018). Plasticity reveals hidden resistance to extinction under climate change in the global hotspot of salamander diversity. Sci. Adv. 4, eaar5471. doi: 10.1126/sciadv.aar5471
Romero L. M. (2002). Seasonal changes in plasma glucocorticoid concentrations in free-living vertebrates. Gen. Comp. Endocrinol. 128, 1–24. doi: 10.1016/S0016-6480(02)00064-3
Romero L. M., Dickens M. J., Cyr N. E. (2009). The reactive scope model—A new model integrating homeostasis, allostasis, and stress. Hormones Behav. 55, 375–389. doi: 10.1016/j.yhbeh.2008.12.009
Sanabria E., Quiroga L. (2019). The body temperature of active desert anurans from hyper-arid environments of South America: the reliability of WorldClim for predicted body temperatures in anurans. J. Thermal Biol. 85, 102398. doi: 10.1016/j.jtherbio.2019.102398
Sapolsky R. M., Romero L. M., Munck A. U. (2000). How do glucocorticoids influence stress responses? Integrating permissive, suppressive, stimulatory, and preparative actions. Endocrine Rev. 21, 55–89. doi: 10.1210/er.21.1.55
Scott D. E., Casey E. D., Donovan M. F., Lynch T. K. (2007). Amphibian lipid levels at metamorphosis correlate to post-metamorphic terrestrial survival. Oecologia 153, 521–532. doi: 10.1007/s00442-007-0755-6
Sheridan M. A. (1994). Regulation of lipid metabolism in poikilothermic vertebrates. Comp. Biochem. Physiol. Part B: Comp. Biochem. 107, 495–508. doi: 10.1016/0305-0491(94)90176-7
Shidemantle G., Buss N., Hua J. (2021). Are glucocorticoids good indicators of disturbance across populations that exhibit cryptic variation in contaminant tolerance? Anim. Conserv. 25, 273–284. doi: 10.1111/acv.12737
Smith G. D., Zani P. A., French S. S. (2019). Life-history differences across latitude in common side-blotched lizards (Uta stansburiana ). Ecol. Evol. 9, 5743–5751. doi: 10.1002/ece3.5157
Telmeco R. S., Gangloff E. J. (2020). Analyzing stress as a multivariate phenotype. Integr. Comp. Biol. 60, 70–78. doi: 10.1093/icb/icaa005
Urban M. C., Bürger R., Bolnick D. I. (2013). Asymmetric selection and the evolution of extraordinary defences. Nat. comm 4 (1), 2085. doi: 10.1038/ncomms3085
Urban M. C., Alberti M., De Meester L., Zhou Y., Verrelli B. C., Szulkin M., et al. (2024). Interactions between climate change and urbanization will shape the future of biodiversity. Nat. Climate Change 14, 436–447. doi: 10.1038/s41558-024-01996-2
Vera F., Zenuto R., Antenucci C. D. (2017). Expanding the actions of cortisol and corticosterone in wild vertebrates: a necessary step to overcome the emerging challenges. Gen. Comp. Endocrinol. 246, 337–353. doi: 10.1016/j.ygcen.2017.01.010
Vitousek M. N., Taff C. C., Hallinger K. K., Zimmer C., Winkler D. W. (2018). Hormones and fitness: evidence for trade-offs in glucocorticoid regulation across contexts. Front. Ecol. Evol. 6. doi: 10.3389/fevo.2018.00042
Vitousek M. N., Taff C. C., Ryan T. A., Zimmer C. (2019). Stress resilience and the dynamic regulation of glucocorticoids. Integr. Comp. Biol. 59, 251–263. doi: 10.1093/icb/icz087
Vogel L. S., Pechmann J. H. K. (2010). Response of Fowler’s Toad (Anaxyrus fowleri) to Competition and Hydroperiod in the Presence of the Invasive Coastal Plain Toad (Incilius nebulifer). J. Herpetology 44, 382–389. doi: 10.1670/08-300.1
Wack C. L., Ratay M. K., Woodley S. K. (2013). Effects of corticosterone on locomotory activity in red-legged salamanders. Herpetologica 69, 118–126. doi: 10.1655/HERPETOLOGICA-D-12-00040R2
Winchell K. M., Carlen E. J., Puente-Rolón A. R., Revell L. J. (2018). Divergent habitat use of two urban lizard species. Ecol. Evol. 8, 25–35. doi: 10.1002/ece3.3600
Winchell K. M., Reynolds R. G., Prado-Irwin S. R., Puente-Rolón A. R., Revell L. J. (2016). Phenotypic shifts in urban areas in the tropical lizard Anolis cristatellus. Evolution 70, 1009–1022. doi: 10.1111/evo.12925
Wingfield J. C., Kelly J. P., Angelier F. (2011). What are extreme environmental conditions and how do organisms cope with them? Curr. Zoology 57, 363–374. doi: 10.1093/czoolo/57.3.363
Wingfield J. C., Sapolsky R. M. (2003). Reproduction and resistance to stress: When and how. J. Neuroendocrinol. 15, 711–724. doi: 10.1046/j.1365-2826.2003.01033.x
Keywords: corticosterone, locomotor performance, glucocorticoid, lipid storage, urbanization
Citation: Monroe DJ, Bókony V and Gabor CR (2024) Physiological and behavioral variation by urbanization and climate in an urban-tolerant toad. Front. Amphib. Reptile Sci. 2:1444580. doi: 10.3389/famrs.2024.1444580
Received: 05 June 2024; Accepted: 18 September 2024;
Published: 14 October 2024.
Edited by:
Anuradha Batabyal, Flame University, IndiaReviewed by:
Leonardo Vignoli, Roma Tre University, ItalyNarahari P. Gramapurohit, Savitribai Phule Pune University, India
Copyright © 2024 Monroe, Bókony and Gabor. This is an open-access article distributed under the terms of the Creative Commons Attribution License (CC BY). The use, distribution or reproduction in other forums is permitted, provided the original author(s) and the copyright owner(s) are credited and that the original publication in this journal is cited, in accordance with accepted academic practice. No use, distribution or reproduction is permitted which does not comply with these terms.
*Correspondence: Caitlin R. Gabor, Z2Fib3JAdHhzdGF0ZS5lZHU=
†Present address: Dillon J. Monroe, Department of Biology, Southern Utah University, Cedar City, UT, United States