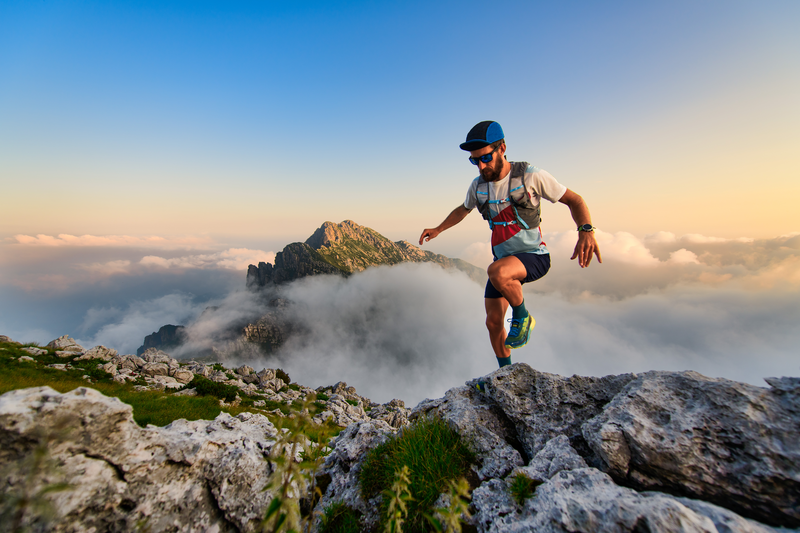
95% of researchers rate our articles as excellent or good
Learn more about the work of our research integrity team to safeguard the quality of each article we publish.
Find out more
MINI REVIEW article
Front. Allergy , 23 February 2024
Sec. Mechanisms in Allergy
Volume 5 - 2024 | https://doi.org/10.3389/falgy.2024.1359142
This article is part of the Research Topic Women in Science: Allergy Research View all 6 articles
The prevalence and severity of allergic diseases have increased over the last 30 years. Understanding the mechanisms responsible for these diseases is a major challenge in current allergology, as it is crucial for the transition towards precision medicine, which encompasses predictive, preventive, and personalized strategies. The urge to identify predictive biomarkers of allergy at early stages of life is crucial, especially in the context of major allergic diseases such as food allergy and atopic dermatitis. Identifying these biomarkers could enhance our understanding of the immature immune responses, improve allergy handling at early ages and pave the way for preventive and therapeutic approaches. This minireview aims to explore the relevance of three biomarker categories (proteome, microbiome, and metabolome) in early life. First, levels of some proteins emerge as potential indicators of mucosal health and metabolic status in certain allergic diseases. Second, bacterial taxonomy provides insight into the composition of the microbiota through high-throughput sequencing methods. Finally, metabolites, representing the end products of bacterial and host metabolic activity, serve as early indicators of changes in microbiota and host metabolism. This information could help to develop an extensive identification of biomarkers in AD and FA and their potential in translational personalized medicine in early life.
The prevalence and severity of allergic diseases have increased over the last 30 years (1). The pathophysiological mechanisms underlying allergic diseases are different depending on the organs affected, but many patients who initially had an allergic disorder, such as atopic dermatitis (AD), eventually develop other allergies such as rhinitis, allergic asthma and/or food allergy (FA). This is commonly known as the “Allergic/ Atopic March” and refers to the natural history of allergic inflammation (2–4).
Atopic dermatitis, also known as eczema, is a skin disorder characterised by skin inflammation, with lesions and intense itch. It affects up to 30% of children and persists in 10% of adults in Western countries (5, 6). Skin barrier disruption could be the first step in the atopic march as well as in AD, leading to both skin inflammation and allergic sensitization. Although it is difficult to diagnose because of its great variety of symptoms, course, and severity, skin lesions, intense pruritus, and a chronic or relapsing course are used as AD diagnosis criteria. However, infants often present with poorly defined erythema lesions with oedema, excoriations, vesicles, and serous exudate, typically distributed on the face, cheeks, and trunk (7). Therefore, AD diagnosis and treatment mostly rely on clinical scores (8).
Food allergy is an unfavourable immune reaction towards food antigens, becoming a global health concern, since it affects both industrialized and developing countries, where their populations are adopting a westernized lifestyle (9). The estimated prevalence of FA is 8% in children and 5% in adults (10). It can be IgE-mediated and non-IgE-mediated. Non-IgE-mediated FA has a late onset of symptoms, and mostly affects the gastrointestinal system and skin, whereas IgE-mediated FA is characterized by a quick onset of symptoms, ranging from mild to severe life-threatening conditions, and involve the respiratory, gastrointestinal, dermatological, and cardiovascular systems (11). The complexity of FA makes it essential to diagnose, monitor and manage this disease by a personalized approach. In children, the diagnosis of FA has fairly improved in the last years, being clinical history, skin prick test and serum allergen-specific IgE levels, together with oral food challenge, the main diagnostic methods. In contrast, the management of FA needs improvement as food avoidance remains the major treatment nowadays.
A major challenge in current allergology is to unravel the mechanisms responsible for these diseases, in order to provide the patients with preventive, and personalised strategies, which has been collectively named as precision medicine (12). Biomarkers are genes, proteins, metabolites, or features by which a particular pathological or physiological process can be identified. They are valuable tools for precision medicine, as they can shed information for diagnosis and patient stratification, identification of therapeutic targets, and monitoring treatment efficacy (13–16). Identifying biomarkers of allergic diseases in early life could improve allergy handling at early ages and pave the way for personalized preventive and therapeutic approaches. Over the past few decades, high-throughput omic techniques have been developed and used in the search for allergy biomarkers (13, 17–19).
In this review, we summarize the current knowledge on three biomarker categories (proteome, microbiome, and metabolome) in two major allergic diseases such as AD and IgE-mediated FA in early life (20–23) (Table 1).
Table 1. Biomarkers associated to AD and/or FA in early life identified by omics techniques. Each omic technique is highlighted in different color: green for proteomics; red for metabolomics; blue for microbiome sequencing (genomics).
The study of the proteome, which is the set of proteins present in a cell at a certain time, is called proteomics, and remains challenging due to its dynamic nature (57). Proteomics usually encompasses multi-dimensional separation and protein identification by mass spectrometry (MS), followed by data analysis by bioinformatic tools. Regarding allergic diseases, proteomic approaches include the measurement of allergen-specific IgE and IgG antibodies, serum tryptase, “damage-associated molecular patterns (also called alarmins), as well as basophil and mast cell activation tests, among others. Some of these procedures are commonly used for diagnosis in clinical practice with more or less satisfactory outcomes depending on the type of allergy innovations in this field are needed to provide novel and robust protein biomarkers that contribute to a better understanding of the molecular mechanisms underlying allergic diseases. In this sense, recent technological advancements in next-generation proteomics, such as OLINK® PEA (Proximity Extension Assay) technology (OLINK Proteomics, Uppsala, Sweeden), enable multiplexing thousands of proteins using minimal sample amounts (58, 59). Therefore, it is a promising tool to improve stratification of patients and characterization of allergic diseases.
The AD proteome is still largely unexplored. Searching for “atopic dermatitis” in proteomic databases (8th November 2023), such as PRIDE [proteomics identifications database; (60)] or GPM [global proteome machine; (61)] retrieved 16 entries, and entries from 3 different studies, respectively (62–64). Twenty-seven AD-related proteins (retrieved on 1st September 2017) were previously reported in Uniprot (2, 65–67).
Regarding the IgE and IgG levels in AD, it is long known that children and adults with AD had high or very high serum IgE levels (68–74). More recently, Wollenberg et al. reviewed data on the efficacy of omalizumab, an anti-IgE biological, in AD and found them inconclusive, as many studies have reported varying degrees of efficacy of this biological drug. Five years earlier, Totté et al. described the association of AD severity with the IgG response against Staphylococcus aureus in young children.
A systematic review and meta-analysis about the correlation between AD severity and biomarkers previously identified serum thymus and activation-regulated chemokine (TARC/CCL17), a member of the T-helper 2 (Th2) chemokine family (TARC), attractant of Th2 effector cells, as the most reliable AD severity biomarker for both children and adults (75, 76). Further Th2-related chemokines have also emerged as potential biomarkers, including CCL18/PARC (pulmonary and activation-regulated chemokine), CCL22/MDC (macrophage-derived chemokine), CCL26/eotaxin-3, CCL27/CTACK (cutaneous T-cell-attracting chemokine), and lactate dehydrogenase (LHD), but additional research is needed (8, 75, 77–79).
Serum interleukin (IL)-13 and IL-22 levels are known to be higher in AD patients than in healthy controls (80). However, AD cytokine biomarkers are preferably assessed in the skin. Upregulation of thymic stromal lymphopoietin (TSLP), IL-4, IL-13, and IL-33, has been related to AD pathogenesis (80–83). Mouse models of AD have demonstrated the critical role of TSLP, which is released by keratinocytes upon epithelial damage, in inducing Th2 responses and AD pathogenesis (84–86). In addition, TSLP plays an important role in the differentiation of follicular T helper cells (TFH), which are critical players in humoral immunity, and AD severity in children (83, 87, 88). In addition, the proinflammatory cytokine IL-33, which belongs to the IL-1 inflammatory cytokine family, activates group 2 innate lymphoid cells (ILC2s) that induce the expression of IL-5 or IL-13. Moreover, IL-33 directly acts on keratinocytes by reducing the expression of junctional proteins, such as filaggrin and claudin-I, leading to skin barrier disruption (82). Similarly, IL-4 and IL-13 downregulate keratinocyte filaggrin expression (26, 89). Pavel AB et al. found considerably higher levels of inflammatory markers, such as proinflammatory interleukins (IL-1R1, IL-33), and metalloproteinases (MMP12) in lesional and non-lesional skin of moderate-to-severe AD patients (26).
Searching for biomarkers by non-invasive sampling approaches, Holm et al., quantified 203 proteins in the vernix of 34 newborns, of which 18 children had developed AD at two years of age (24). The study showed that peroxiredoxin-2 and serpin A12 were present at higher levels in the AD group, and polyubiquitin-C and calmodulin-like protein 5 at lower levels when compared to healthy children. These four proteins had the highest impact on an orthogonal projection to latent structures-discriminant analysis (OPLS-DA) model. The authors concluded that the abundances of polyubiquitin-C and calmodulin-like protein 5, negatively correlated with AD development, are promising AD biomarker candidates.
When looking for reliable protein biomarkers, age at sampling is a key factor to take into account, since the proteome is dynamic over time. Stockfelt et al., phenotyped 230 proteins in plasma prepared from umbilical cord blood, and blood collected at 1, 4, 18 months of age (and further) (25). They found that samples collected at 1 month of age were the most informative for the prediction of atopy later in childhood. Precisely, they found 27 proteins, including MHC class I molecules, interleukins and chemokines that were associated either with a lower risk, such as MHC class I proteins, IL-15 as well as TNFα-induced protein 3 (TNFAIP3) and Myeloid Derived Growth Factor (MYDGF), or a higher risk of developing atopy later in life, such as the interleukins IL-9 and IL-17, the chemokine receptor-10 (CCR10), the Growth Arrest and DNA Damage Inducible-α (GADD45A), as well as the proteases MPO and MMP9.
On the other hand, stratification of patients according to a cluster of several protein biomarkers, seems to be a promising approach in the elucidation of AD endotypes, as shown by studies from Thijs JL et al. and followed up by Bakker DS et al. (90, 91). In the first study, four serum biomarker-based clusters were identified and associated with different severity scores in a cohort of 193 patients. Three years later, with a different cohort of 143 AD patients, Bakker DS et al. identified four distinct patient clusters, three of them very similar to the previously identified. However, none of the studies could show a clear association between the clusters and atopic comorbidities or epidemiological variables, such as age. More studies are needed in this sense because the elucidation of AD endotypes might contribute to the development of personalized medicine and the better election of specific therapies in the management of AD.
Component resolved diagnosis or molecular diagnosis has led to a shift in the diagnosis of FA, improving accuracy, allowing the identification of complete amino acid sequences and IgE-binding epitopes of food allergens. These advances contribute to improved diagnosis and prognosis of FA, as well as safety assessment of foods and allergy testing (92, 93). Several immunological markers, such as specific IgE (sIgE), IgG4, sIgE/total IgE ratios or cytokines, have been monitored ever since in the course of FA. Kukkonen et al., described that sIgE levels to Ara h 2 and Ara h 6 were associated with more severe reactions to peanut in a cohort of 102 patients (6- to 18-year-olds) with peanut sensitization and a high risk of suffering peanut allergy (94).
Compared to peanut and tree-nut allergies, milk and egg allergies have better outgrown outcomes in children (94). Cow's milk tolerance has been associated with elevated levels of cow's milk-specific IgG4 and an elevated IgG4/sIgE ratio in two independent studies (95, 96). However, in 2018, another study found no significant correlation between sIgE levels and milk tolerance in a cohort of 84 cow's milk allergic infants (6 months-3 years old) who tolerated baked milk in oral food challenge) (97). In the case of egg allergy, ingestion of baked egg together with decreased sIgE levels and increased egg-specific IgG4 were associated to the development of egg tolerance, while a Th1 cytokine profile and increased levels of IL-10 protein were associated to allergy resolution (98–101).
Biomarkers other than specific IgE and IgG antibodies are being studied in FA. Proteins or peptides released after immunological stimulation, degranulation, or cell damage or death, have been extensively described in the pathogenesis of asthma or AD (as discussed in the previous section). These molecules have been referred to as alarmins since 2006 and are increasingly considered in the study of the pathogenesis of FAs, as well as their potential role as biomarkers of the disease or possible therapeutic targets (102, 103). When damage occurs, due to inflammation or pathogen invasion, epithelial cells initiate a cascade of events encompassing the production of alarmins, such as TSLP, IL-33, and IL-25 (104). These signaling molecules activate innate lymphoid cells (ILC2) and Th2 cells, leading to the release of cytokines that promote a Th2-type immune response. For example, IL-5 and IL-13, produced by these alarmins, contribute to the recruitment and accumulation of eosinophils in the tissue and trigger the switch in IgE antibody production, respectively. IL-4, produced by Th2 cells, activates mast cells and basophils (105). Cutaneous infiltration of basophils may direct eosinophil recruitment and enhance food antigen sensitization. These observations emphasize the hypothesis that skin barrier disruption in children triggers transcutaneous sensitization and leads to the development of IgE-mediated FA (106).
Another type of alarmin is calprotectin, an immunomodulatory and antimicrobial protein found in mucosal epithelial cells, macrophages, and neutrophil cytoplasm (107). As its concentration in feces is six times higher than in plasma, it is considered a fecal biomarker and is well-established as a reliable biomarker of intestinal inflammation (108–110). Regarding FA, it has been proposed as a good diagnostic indicator of cow's milk protein allergy (CMPA) and shows promise for monitoring intestinal allergies due to its correlation with inflammation and immune responses to food antigens (111–114).
The heterogeneity of FA, with multiple triggers and confounding factors in the allergic history of patients, makes it necessary to continue the search for reliable and robust biomarkers and validate them for use in daily clinical practice.
The term microbiota refers to the living microorganisms inhabiting a defined environment or body site, such as the skin, the gut, the oral cavity, and the vagina. The term microbiome refers to “the collection of genomes from all the microorganisms” in a specific environment. This “also includes the microbial structural elements, metabolites, and environmental conditions” (22, 115, 116).
Initial colonization of the microbiota at the body's interface occurs at birth. During natural delivery, the mother provides the neonate with the founder commensals, derived from her vagina and faeces (117, 118). Breast milk feeding further helps shaping microbiota diversification by providing secretory IgA and prebiotic glycans that promote the expansion of specific species (119–121). In contrast, delivery by caesarean section provides the infants with the mother's skin-derived microbiota (122–124). A study found that caesarean section delivery predisposed to the development of FA but not AD in early childhood (125). In turn, epidemiological studies have linked perinatal (pre- and post-) antibiotic use to the appearance of AD and cow's milk allergy in infants (126–128). Whether early disruption of the microbiota directly affects future risk of allergic diseases remains elusive.
Skin microbiota composition in infants shifts over time being distinct from that observed later in life. Therefore, age affects skin microbiome composition, together with the birth delivery mode and maternal commensals (27, 129). Longitudinal studies found alterations in skin microbiota that predate AD onset. Two-day-old infants have site-specific differences in their skin microbiomes that might influence the future development of AD. Precisely, the abundance of Staphylococcus species was higher on the extremities, whereas Gemella and Propionibacterium species were more abundant on facial sites (27). Further evidence showed that 12-month-old infants with affected skin had significantly less commensal Staphylococci at month 2 when compared with unaffected infants (27, 130). Thus, commensal Staphylococci might confer protection against AD development. In a prospective birth cohort study, Staphylococcus aureus' prevalence was higher on the skin of 3-month-old infants who developed AD later, as compared with age-matched, non-atopic infants. Moreover, at AD onset, infants with S. aureus on their skin were younger than those without it (30). Several genomic studies confirmed S. aureus abundance and that of other coagulase-negative Staphylococci (CoNS) to increase in AD, whereas Streptococcus, Propionibacterium, Acinetobacter, Cutibacterium, and Corynebacterium genera are typically present in the homeostatic skin (28, 29, 131–134). These data suggest that alterations in skin colonization may contribute to AD onset in early life. Currently ongoing, the “Munich Atopic Prediction Study” collects information on pregnancy, parental exposures to potential allergens, environmental factors, child development, and acute or chronic diseases of both children and parents together with microbiome analyses from stool and skin swabs, and clinical examination by trained dermatologists at 2 months after birth and every 6 months thereafter. Results from this study will certainly be useful for the identification of AD biomarkers (135).
Studies in mice showed that the establishment of a homeostatic skin microbiota are preferential in early life, and likely to be more significant in the skin than in other tissues (136). When neonatal mice are colonized by S. epidermidis, a CoNS commensal, a large proportion of Tregs specific for S. epidermidis develops, ensuring homeostasis to this microbe. In contrast, delaying exposure to S. epidermidis abrogates its protective effect and promotes skin inflammation (137).
Prominent research shows the impact of the gut microbiome on the skin microbiome and the development of skin diseases (138, 139). This is referred to as the gut-skin axis (140). Wang et al., found a reduced diversity in the faecal microbiota of infants with AD during the first 18 months of life (31). Moreover, Penders et al., observed an association between the colonization with Clostridium species at 5 and 13 weeks of age with an increased risk of AD in the subsequent 6 months of life (33). In turn, Marrs et al., described that a greater abundance of Clostridium sensu stricto was associated with AD in 3-month-old breastfed infants (32). In contrast, recently, Park et al. found low levels of Clostridium and Akkermansia and high levels of Streptococcus in children with persistent AD. Moreover, the relative abundance of Streptococcus positively correlated with AD score (SCORAD), whereas that of Clostridium negatively correlated with SCORAD. (34). The findings of these two studies may seem contradictory. However, the different experimental approaches may account for their different findings. Park et al. did not define any correction for confounding factors such as introduction to solid foods or mode of delivery, and they collected faecal samples at 6 months of age, particularly when Marrs et al. found the larger differences in gut microbiota composition due to diet. Moreover, the definition of the Clostridia species also differed in these two studies.
Understanding the factors modulating skin and gut microbiomes is essential for identifying predictive AD biomarkers and maintaining a homeostatic skin.
The gut microbiota is a major ecosystem, which changes in response to environmental factors such as diet, pathogens, and antibiotic treatment, significantly influencing immune system maturation through dendritic cell–mediated regulation (124, 141, 142). Alterations in the gut microbiota composition together with decreased levels of Tregs have been found in subjects with rhinitis, asthma, AD, and FA to peanuts, eggs, or cow's milk (22, 50, 124, 143–145). The amount of Tregs in the gut seems to associate to specific genera of bacteria; therefore, this could be a mechanism by which the gut microbiota modulates FA course. Protective effects against FA have been linked with genera such as Lactobacillus, Bifidobacterium, Faecalibacterium, Akkermansia, Staphylococcus, and Clostridium (51, 146–148). SCFA-producing bacteria from phylum Firmicutes, including Lactobacillaceae, Ruminococcaceae and Lachnospiracea families, actively contribute to regulating intestinal immune responses, by enhancing mucin production, improving barrier integrity, and preventing the colonization of harmful strains (149–152). On the contrary, certain genera, such as Clostridium, Enterococcus, Klebsiella, and Enterobacter, have been related to a higher likelihood of developing FA (146, 147, 153).
Mera-Berriatua et al., analysed the faecal microbiota of 34 cow's milk allergic infants vs. 16 non-allergic controls (51). They found an increased frequency of the Prevotellaceae family in control and formula-fed infants compared to allergic and hydrolysate-fed infants, respectively. However, since samples were collected at only one timepoint (6 months old) when cow's milk allergy was already established, they could not discern whether differences were due to the infants’ allergy or their diet. When analysing the gut microbiota of the mothers, they did not find anymicrobial signatures predisposing to FA and transmitted by the mothers. Abdel-Gadir et al., analysed the faecal microbiota of 56 infants with FA and 98 controls at different times (154). They found that infants with FA presented a dysbiotic faecal microbiota composition that evolved over time. Therapy with Clostridiales or Bacteroidales suppressed FA in mice. Recently, early inoculation with certain Clostridium species was reported to decrease IgE levels in adulthood. Conversely, 3-week-old infants that presented higher proportions of Clostridium difficile than Bifidobacterium had higher chances of being allergic to food and aeroallergens (155).
These findings underscore the intricate relationship between gut microbiota, immune function, and FA course. Despite advances in this field, it is still unknown whether a dysbiosed gut microbiota triggers FA or is the disease itself the one altering microbiota composition and functionality.
Metabolites are the final products of cellular activity and significantly impact immune responses. Therefore, the identification and analysis of metabolic biomarkers is essential to improve our understanding of AD and FA. The Human Metabolome Database [HMDB; (156)] offers an efficient electronic resource readily accessible for comprehensive information on human metabolites. It includes links to various databases, along with numerous tools for structure and pathway visualization. Additionally, this database facilitates guidance on diseases wherein the target metabolite can act as a biomarker.
The skin of mammals, especially the epidermis, contains extensive amounts of lipids. These lipids, including ceramides, cholesterol, and free fatty acids, are integral epidermal barrier components (140, 157). Lipid alterations in the outer skin layer led to barrier impairment and eventually to AD. The alteration of epidermal ceramide composition also promotes inflammatory and allergic events in infants with AD (36). Moreover, reduced levels of ceramides associate with S. aureus colonization in AD patients (35). A recent study on newborns showed that at the age of 2 months, children with future AD had reduced protein-bound ceramides and increased unsaturated sphingomyelin species in the skin. This study demonstrated a significant predictive power for AD in 2-month-old children. Precisely, TSLP, at the age of 2 months was predictive of the onset of atopic dermatitis by the age of 24 months with an odds ratio (OR) of 4.1 (95% CI, 1.7–10.1). When combined with family history of atopic diseases, the predictive power of TSLP level rose to the OR of 6.0 (with 95% CI of 2.3–15.8). Additionally, the combination of high TSLP, high 24:1-SM, and low O30:0(C20S)-CER had the highest OR of developing AD by the age of 24 months at 29.6 (95% CI, 4.9–179.7) (37).
Moreover, alterations in the fatty acid composition of plasma [decreased levels of omega-3 polyunsaturated fatty acids (PUFAs) and increased levels of omega-6 PUFAs] have been observed in infants who later develop AD. Furthermore, the findings suggest that adjusting the diet to include more omega-3 fatty acids, particularly through fish oil supplementation during pregnancy and lactation, could effectively restore this balance and potentially reduce the risk of AD in infants (40). However, information on other relevant metabolites in adult AD, such as sphingosine 1-phosphate and acylcarnitines, is lacking in children (158). These data suggest that an in-depth analysis of cutaneous lipid profile could aid in the early diagnosis and effective management of AD (159).
As previously said, the gut-skin axis is important in AD. Gut microbiota generates numerous metabolites, which can permeate the circulatory system and reach remote body sites (160). SCFA, including acetate, propionate, butyrate, and valerate, are byproducts of intestinal microbiota fermentation of fibre (44, 149, 161–163). A distinct SCFA profile has been identified in AD patients. Therefore, nutrition plays a key role. Atopy in children has been associated with deficiencies of micronutrients such as iron (164, 165). Iron deficiency leads to immune cell activation (166). A study reported that when mothers were supplemented for both iron and folic acid, their children had a 4-fold decreased risk for AD (42). In a systematic review, Venter et al., found that maternal intake of beta-carotene, vitamin E, zinc, calcium, magnesium, and copper during pregnancy resulted in a decreased risk of AD for the offspring. Supplementation with omega-3 PUFAs in pregnant women has been shown to reduce infant sensitization to egg, and the risk and severity of atopic dermatitis in the first year of life, especially in children of mothers with a low habitual intake of omega-3 PUFAs (40, 43, 167–169). Several studies provided preliminary evidence of a protective effect of prenatal vitamin D supplementation on the likelihood of childhood AD. Notably, infants with AD had a significant vitamin D deficiency and insufficiency, which correlated with the severity of IL-17A-dependent AD (170). Additionally, prenatal maternal depression and anxiety were reported to increase the risk of AD in the offspring by decreasing the ratio of placental glutathione to glutathione disulfide (38). This implies that oxidative stress plays a part in the pathogenesis of AD. In fact, thiol-disulfide balance has been proposed as a biomarker of childhood AD (39). These findings highlight the importance of diet in the maternal period and early life, as alterations of the metabolome in AD infants have been in correlation to the gut microbiota.
Various metabolites have been recognized as potential biomarkers for FA. Crestani et al., described reduced sphingolipids and ceramides in children with FA, indicating a potential association between altered gut microbiota and immune function. Children with a history of anaphylaxis and multiple allergies display alterations in tryptophan metabolites, including decreased levels of metabolites in the kynurenine and serotonin pathways, and increased levels in the indole pathway. Additionally, changes in eicosanoids, plasmalogens, and fatty acids have been observed. Children with FA exhibited unique dysregulation of lysoplasmalogens, lysophospholipids, N2-acetyllysine, β/γ tocopherol, and sphingomyelins, compared to controls (52). A recent prospective study established the relationship between newborn lipid profiles and the development of FA. Cord triacylglycerols of long carbon chains and multiple double bonds were identified as potential early-life biomarkers for high-risk infant FA (54). Another study described a significant enrichment of diacylglycerol in healthy twins compared to those with FA (53). Additionally, children with FA exhibited a significant increase in sphingolipids and a decrease in acylcarnitines when compared to healthy controls. Interestingly, at the time of diagnosis, individuals with resolving FA displayed elevated omega-3 PUFAs levels and decreased platelet-activating factors in comparison to those with persistent FA. However, over time, a decline in omega-3 PUFAs levels was observed in resolving FA cases, while an increase occurred in persistent FA cases (56). Omega-3 metabolites, derived from eicosapentaenoic acid (EPA) and docosahexaenoic acid (DHA), play a crucial role in this context. In individuals with resolving FA, high levels of hydroxydocosahexaenoic acid (HDoHE) were observed, while levels of the pro-resolving mediators RvD1 and RvD2 were low. This may be attributed to the utilization of 17-HDoHE during the production of resolvins. Such a process could reduce IgE production by human B cells and suppress the differentiation of naive B cells into IgE-secreting cells, which are relevant in the context of allergies. Additionally, RvD1 has been reported to modulate allergic airway responses by reducing eosinophils and pro-inflammatory mediators. Furthermore, the association between HDoHE levels and the PAK5 gene, previously linked to atopy and psoriasis, supports their relevance to allergic diseases. The study suggests that HDoHEs and D-series resolvins may play a significant role in the pathophysiology of FA and FA resolution, potentially serving as markers for FA resolution. However, it is important to note that studies on omega-3 exposure in childhood allergic diseases have produced inconsistent results, highlighting the complexity of these processes.
Distinct patterns of bile acids, amino acids, steroid hormones, and SCFA were found in individuals with FA. At 3–6 months and/or 1 year of age, reductions were observed in bile acids, amino acids, and steroid hormones. Conversely, individuals with FA exhibited elevated levels of diacylglycerols at 3–6 months. Variations in primary bile acid biosynthesis and reduced levels of intestinal bile acid metabolites synthesized through the alternative pathway have been described to distinguish subjects with persistent FA from those in FA remission (55). Furthermore, the microbiome's production of SCFA is crucial for gut health and immune modulation. Changes in the microbiome that lead to alterations in bile acid metabolism and SCFA production may impact the persistence or remission of food allergies. Precisely, lower levels of propionate, butyrate, and valerate have been associated with FA in infants (45, 46).
Although there is limited information on immune metabolism in AD and FA, both conditions have been associated with impaired barrier function of epithelial cells, characterized by a predominantly glycolytic phenotype and mitochondrial dysfunction (171, 172). Increased lactate levels, resulting from increased lactic acid bacteria, have been proposed as a non-invasive marker for assessing temporary changes in cellular stress and toxicity in AD and FA (41, 47–49).
Figure 1 summarizes the major biomarkers identified by omics techniques associated with AD and/or FA in early life.
Figure 1. Summary of major common and exclusive biomarkers for atopic dermatitis and food allergy in early life. Created with Biorender.com.
The identification of potential biomarkers in early life could improve the diagnosis, monitoring, and management of AD and FA. Exploring the relationship between proteomics, microbiota and metabolomics is crucial, as some alterations in the microbiome can induce changes in the host. For example, common microbiota-derived metabolites such as SCFA and lactate, along with the presence of certain bacteria in faecal samples such as the genus Streptococcus, may play a role in shaping the host immune response. The integration of data from various omics technologies coupled with advances in statistical and computational techniques, is expected to enable the application of precision medicine and targeted therapy in allergic patients within the next decades, allowing the classification of heterogeneous diseases like FA and AD into endotypes and phenotypes. There is therefore an urgent need of extensive clinical and basic research to identify biomarkers in AD and FA specific for different allergic phenotypes.
EZ-V: Conceptualization, Investigation, Methodology, Software, Visualization, Writing – original draft, Writing – review & editing. MI-S: Formal Analysis, Funding acquisition, Resources, Writing – review & editing. CG-C: Conceptualization, Investigation, Methodology, Supervision, Validation, Visualization, Writing – original draft, Writing – review & editing. MP-G: Conceptualization, Investigation, Methodology, Project administration, Resources, Supervision, Validation, Writing – original draft, Writing – review & editing.
The author(s) declare financial support was received for the research, authorship, and/or publication of this article.
This study was supported by the ISCIII (Project number PI20/01366), the Ministerio de Ciencia, Innovación y Universidades (RTI2018-095166-B-I00), and the European Regional Development Fund “Investing in your future” for the thematic network and co-operative research centers ARADyAL (RD16/0006/0015 and RD16/0006/0016), RICORS “Red de Enfermedades Inflamatorias (REI)” (RD21/0002/0008) and co-funded by the European program ERA HDHL – Nutrition & the Epigenome, Project Dietary Intervention in Food Allergy: Microbiome, Epigenetic and Metabolomic Interactions DIFAMEM.
The authors declare that the research was conducted in the absence of any commercial or financial relationships that could be construed as a potential conflict of interest.
All claims expressed in this article are solely those of the authors and do not necessarily represent those of their affiliated organizations, or those of the publisher, the editors and the reviewers. Any product that may be evaluated in this article, or claim that may be made by its manufacturer, is not guaranteed or endorsed by the publisher.
1. Singh AB, Kumar P. Climate change and allergic diseases: an overview. Front Allergy. (2022) 3:964987. doi: 10.3389/falgy.2022.964987
2. Ghosh D, Bernstein JA, Khurana Hershey GK, Rothenberg ME, Mersha TB. Leveraging multilayered “omics” data for atopic dermatitis: a road map to precision medicine. Front Immunol. (2018) 9:2727. doi: 10.3389/fimmu.2018.02727
3. Galli SJ, Tsai M, Piliponsky AM. The development of allergic inflammation. Nature. (2008) 454:445–54. doi: 10.1038/nature07204
4. Spergel J. Atopic dermatitis and the atopic march. J Allergy Clin Immunol. (2003) 112:S118–27. doi: 10.1016/j.jaci.2003.09.033
5. Bylund S, Kobyletzki L, Svalstedt M, Svensson Å. Prevalence and incidence of atopic dermatitis: a systematic review. Acta Dermato Venereologica. (2020) 100:adv00160. doi: 10.2340/00015555-3510
6. Gomez-Casado C, Unger Z, Olah P, Homey B. Microbiome in atopic dermatitis: is it all about Staphylococcus aureus? Curr Treat Options Allergy. (2023) 10:351–63. doi: 10.1007/s40521-023-00350-9
7. Langan SM, Irvine AD, Weidinger S. Atopic dermatitis. Lancet. (2020) 396:345–60. doi: 10.1016/S0140-6736(20)31286-1
8. Renert-Yuval Y, Thyssen JP, Bissonnette R, Bieber T, Kabashima K, Hijnen D, et al. Biomarkers in atopic dermatitis—a review on behalf of the international eczema council. J Allergy Clin Immunol. (2021) 147:1174–1190.e1. doi: 10.1016/j.jaci.2021.01.013
9. Koplin JJ, Peters RL, Ponsonby A-L, Gurrin LC, Hill D, Tang MLK, et al. Increased risk of peanut allergy in infants of Asian-born parents compared to those of Australian-born parents. Allergy. (2014) 69:1639–47. doi: 10.1111/all.12487
10. Sicherer SH, Sampson HA. Food allergy: a review and update on epidemiology, pathogenesis, diagnosis, prevention, and management. J Allergy Clin Immunol. (2018) 141:41–58. doi: 10.1016/J.JACI.2017.11.003
11. Calvani M, Anania C, Caffarelli C, Martelli A, Miraglia Del Giudice M, Cravidi C, et al. Food allergy: an updated review on pathogenesis, diagnosis, prevention and management. Acta Biomed. (2020) 91:e2020012. doi: 10.23750/abm.v91i11-S.10316
12. Muraro A, Lemanske RF, Castells M, Torres MJ, Khan D, Simon H-U, et al. Precision medicine in allergic disease—food allergy, drug allergy, and anaphylaxis—PRACTALL document of the European academy of allergy and clinical immunology and the American academy of allergy, asthma and immunology. Allergy. (2017) 72:1006–21. doi: 10.1111/all.13132
13. Devonshire A, Gautam Y, Johansson E, Mersha TB. Multi-omics profiling approach in food allergy. World Allergy Organ J. (2023) 16:100777. doi: 10.1016/j.waojou.2023.100777
14. Ogulur I, Pat Y, Ardicli O, Barletta E, Cevhertas L, Fernandez-Santamaria R, et al. Advances and highlights in biomarkers of allergic diseases. Allergy. (2021) 76:3659–86. doi: 10.1111/all.15089
15. Rosace D, Gomez-Casado C, Fernandez P, Perez-Gordo M, Dominguez MdC, Vega A, et al. Profilin-mediated food-induced allergic reactions are associated with oral epithelial remodeling. J Allergy Clin Immunol. (2019) 143:681–690.e1. doi: 10.1016/J.JACI.2018.03.013
16. Pablo-Torres C, Izquierdo E, Tan TJ, Obeso D, Layhadi JA, Sánchez-Solares J, et al. Deciphering the role of platelets in severe allergy by an integrative omics approach. Allergy. (2023) 78:1319–32. doi: 10.1111/all.15621
17. Kelly RS, Dahlin A, McGeachie MJ, Qiu W, Sordillo J, Wan ES, et al. Asthma metabolomics and the potential for integrative omics in research and the clinic. Chest. (2017) 151:262–77. doi: 10.1016/j.chest.2016.10.008
18. Irizar H, Kanchan K, Mathias RA, Bunyavanich S. Advancing food allergy through omics sciences. J Allergy Clin Immunol Pract. (2021) 9:119–29. doi: 10.1016/j.jaip.2020.07.044
19. Czolk R, Klueber J, Sørensen M, Wilmes P, Codreanu-Morel F, Skov PS, et al. IgE-mediated peanut allergy: current and novel predictive biomarkers for clinical phenotypes using multi-omics approaches. Front Immunol. (2021) 11:594350. doi: 10.3389/fimmu.2020.594350
20. Obeso D, Contreras N, Dolores-Hernández M, Carrillo T, Barbas C, Escribese MM, et al. Development of a novel targeted metabolomic LC-QqQ-MS method in allergic inflammation. Metabolites. (2022) 12:592. doi: 10.3390/metabo12070592
21. Donald K, Finlay BB. Early-life interactions between the microbiota and immune system: impact on immune system development and atopic disease. Nat Rev Immunol. (2023) 23:735–48. doi: 10.1038/s41577-023-00874-w
22. Zubeldia-Varela E, Barker-Tejeda TC, Obeso D, Villaseñor A, Barber D, Pérez-Gordo M. Microbiome and allergy: new insights and perspectives. J Investig Allergol Clin Immunol. (2022) 32:327–44. doi: 10.18176/jiaci.0852
23. Obeso D, Mera-Berriatua L, Rodríguez-Coira J, Rosace D, Fernández P, Martín-Antoniano IA, et al. Multi-omics analysis points to altered platelet functions in severe food-associated respiratory allergy. Allergy. (2018) 73:2137–49. doi: 10.1111/ALL.13563
24. Holm T, Rutishauser D, Kai-Larsen Y, Lyutvinskiy Y, Stenius F, Zubarev RA, et al. Protein biomarkers in vernix with potential to predict the development of atopic eczema in early childhood. Allergy. (2014) 69:104–12. doi: 10.1111/all.12308
25. Stockfelt M, Hong M-G, Hesselmar B, Adlerberth I, Wold AE, Schwenk JM, et al. Circulating proteins associated with allergy development in infants—an exploratory analysis. Clin Proteomics. (2021) 18:11. doi: 10.1186/s12014-021-09318-w
26. Pavel AB, Zhou L, Diaz A, Ungar B, Dan J, He H, et al. The proteomic skin profile of moderate-to-severe atopic dermatitis patients shows an inflammatory signature. J Am Acad Dermatol. (2020) 82:690–9. doi: 10.1016/j.jaad.2019.10.039
27. Kennedy EA, Connolly J, Hourihane JO, Fallon PG, McLean WHI, Murray D, et al. Skin microbiome before development of atopic dermatitis: early colonization with commensal staphylococci at 2 months is associated with a lower risk of atopic dermatitis at 1 year. J Allergy Clin Immunol. (2017) 139:166–72. doi: 10.1016/j.jaci.2016.07.029
28. Kong HH, Oh J, Deming C, Conlan S, Grice EA, Beatson MA, et al. Temporal shifts in the skin microbiome associated with disease flares and treatment in children with atopic dermatitis. Genome Res. (2012) 22:850–9. doi: 10.1101/GR.131029.111
29. Byrd AL, Deming C, Cassidy SKB, Harrison OJ, Ng W-I, Conlan S, et al. Staphylococcus aureus and staphylococcus epidermidis strain diversity underlying pediatric atopic dermatitis. Sci Transl Med. (2017) 9(397):eaal4651. doi: 10.1126/scitranslmed.aal4651
30. Meylan P, Lang C, Mermoud S, Johannsen A, Norrenberg S, Hohl D, et al. Skin colonization by staphylococcus aureus precedes the clinical diagnosis of atopic dermatitis in infancy. J Invest Dermatol. (2017) 137:2497–504. doi: 10.1016/j.jid.2017.07.834
31. Wang M, Karlsson C, Olsson C, Adlerberth I, Wold AE, Strachan DP, et al. Reduced diversity in the early fecal microbiota of infants with atopic eczema. J Allergy Clin Immunol. (2008) 121:129–34. doi: 10.1016/j.jaci.2007.09.011
32. Marrs T, Jo J-H, Perkin MR, Rivett DW, Witney AA, Bruce KD, et al. Gut microbiota development during infancy: impact of introducing allergenic foods. J Allergy Clin Immunol. (2021) 147:613–621.e9. doi: 10.1016/j.jaci.2020.09.042
33. Penders J, Gerhold K, Stobberingh EE, Thijs C, Zimmermann K, Lau S, et al. Establishment of the intestinal microbiota and its role for atopic dermatitis in early childhood. J Allergy Clin Immunol. (2013) 132:601–607.e8. doi: 10.1016/j.jaci.2013.05.043
34. Park YM, Lee S-Y, Kang M-J, Kim B-S, Lee M-J, Jung SS, et al. Imbalance of gut streptococcus, clostridium, and akkermansia determines the natural course of atopic dermatitis in infant. Allergy Asthma Immunol Res. (2020) 12:322. doi: 10.4168/aair.2020.12.2.322
35. Bhattacharya N, Sato WJ, Kelly A, Ganguli-Indra G, Indra AK. Epidermal lipids: key mediators of atopic dermatitis pathogenesis. Trends Mol Med. (2019) 25:551–62. doi: 10.1016/j.molmed.2019.04.001
36. Ishikawa J, Narita H, Kondo N, Hotta M, Takagi Y, Masukawa Y, et al. Changes in the ceramide profile of atopic dermatitis patients. J Invest Dermatol. (2010) 130:2511–4. doi: 10.1038/jid.2010.161
37. Berdyshev E, Kim J, Kim BE, Goleva E, Lyubchenko T, Bronova I, et al. Stratum corneum lipid and cytokine biomarkers at age 2 months predict the future onset of atopic dermatitis. J Allergy Clin Immunol. (2023) 151:1307–16. doi: 10.1016/j.jaci.2023.02.013
38. Chang HY, Suh DI, Yang S-I, Kang M-J, Lee S-Y, Lee E, et al. Prenatal maternal distress affects atopic dermatitis in offspring mediated by oxidative stress. J Allergy Clin Immunol. (2016) 138:468–475.e5. doi: 10.1016/j.jaci.2016.01.020
39. Karacan G, Ercan N, Bostanci I, Alisik M, Erel O. A novel oxidative stress marker of atopic dermatitis in infants: thiol–disulfide balance. Arch Dermatol Res. (2020) 312:697–703. doi: 10.1007/s00403-020-02054-5
40. Miles EA, Childs CE, Calder PC. Long-chain polyunsaturated fatty acids (LCPUFAs) and the developing immune system: a narrative review. Nutrients. (2021) 13:247. doi: 10.3390/nu13010247
41. Assfalg M, Bortoletti E, D'Onofrio M, Pigozzi R, Molinari H, Boner AL, et al. An exploratory 1 H-nuclear magnetic resonance metabolomics study reveals altered urine spectral profiles in infants with atopic dermatitis. Br J Dermatol. (2012) 166:1123–5. doi: 10.1111/j.1365-2133.2011.10711.x
42. Fortes C, Mastroeni S, Mannooranparampil TJ, Di Lallo D. Pre-natal folic acid and iron supplementation and atopic dermatitis in the first 6 years of life. Arch Dermatol Res. (2019) 311:361–7. doi: 10.1007/s00403-019-01911-2
43. Venter C, Meyer RW, Nwaru BI, Roduit C, Untersmayr E, Adel-Patient K, et al. EAACI position paper: influence of dietary fatty acids on asthma, food allergy, and atopic dermatitis. Allergy. (2019) 74:1429–44. doi: 10.1111/ALL.13764
44. Wang L, Huang Y, Lu C, Chiang B, Shen Y, Huang H, et al. Lower caprylate and acetate levels in the breast milk is associated with atopic dermatitis in infancy. Pediatr Allergy Immunol. (2022) 33(2):e13744. doi: 10.1111/pai.13744
45. Gio-Batta M, Spetz K, Barman M, Bråbäck L, Norin E, Björkstén B, et al. Low concentration of fecal valeric acid at 1 year of age is linked with eczema and food allergy at 13 years of age: findings from a Swedish birth cohort. Int Arch Allergy Immunol. (2022) 183:398–408. doi: 10.1159/000520149
46. Roduit C, Frei R, Ferstl R, Loeliger S, Westermann P, Rhyner C, et al. High levels of butyrate and propionate in early life are associated with protection against atopy. Allergy. (2019) 74:799–809. doi: 10.1111/all.13660
47. Huang Y, Chen G, Liu X, Shao Y, Gao P, Xin C, et al. Serum metabolomics study and eicosanoid analysis of childhood atopic dermatitis based on liquid chromatography–mass spectrometry. J Proteome Res. (2014) 13:5715–23. doi: 10.1021/pr5007069
48. Wopereis H, Sim K, Shaw A, Warner JO, Knol J, Kroll JS. Intestinal microbiota in infants at high risk for allergy: effects of prebiotics and role in eczema development. J Allergy Clin Immunol. (2018) 141:1334–1342.e5. doi: 10.1016/j.jaci.2017.05.054
49. Guadamuro L, Diaz M, Jiménez S, Molinos-Norniella C, Pérez-Solis D, Rodríguez JM, et al. Fecal changes following Introduction of milk in infants with outgrowing non-IgE cow’s milk protein allergy are influenced by previous consumption of the probiotic LGG. Front Immunol. (2019) 10:1819. doi: 10.3389/fimmu.2019.01819
50. Simonyté Sjödin K, Hammarström M-L, Rydén P, Sjödin A, Hernell O, Engstrand L, et al. Temporal and long-term gut microbiota variation in allergic disease: a prospective study from infancy to school age. Allergy. (2019) 74:176–85. doi: 10.1111/all.13485
51. Mera-Berriatua L, Zubeldia-Varela E, Martín-Antoniano IA, López de Maturana E, Rojo D, Bazire R, et al. Unravelling the gut Microbiota of cow’s milk-allergic infants, their mothers, and their grandmothers. J Investig Allergol Clin Immunol. (2022) 32:395–8. doi: 10.18176/jiaci.0781
52. Crestani E, Harb H, Charbonnier LM, Leirer J, Motsinger-Reif A, Rachid R, et al. Untargeted metabolomic profiling identifies disease-specific signatures in food allergy and asthma. J Allergy Clin Immunol. (2019) 45(3):897–906. doi: 10.1016/j.jaci.2019.10.014
53. Bao R, Hesser LA, He Z, Zhou X, Nadeau KC, Nagler CR. Fecal microbiome and metabolome differ in healthy and food-allergic twins. J Clin Invest. (2021) 131:e141935. doi: 10.1172/JCI141935
54. Hong X, Liang L, Ji H, Frischmeyer-Guerrerio P, Wang G, Pearson C, et al. Fetal lipidome and incident risk of food allergy: a prospective birth cohort study. Pediatr Allergy Immunol. (2022) 33(2):e13722. doi: 10.1111/pai.13722
55. Lee S, Park YM, Yoo HJ, Lee S, Choi EJ, Baek EY, et al. The alternative bile acid pathway can predict food allergy persistence in early childhood. Pediatr Allergy Immunol. (2023) 34(8):e14003. doi: 10.1111/pai.14003
56. Jang H, Kim EG, Kim M, Kim SY, Kim YH, Sohn MH, et al. Metabolomic profiling revealed altered lipid metabolite levels in childhood food allergy. J Allergy Clin Immunol. (2022) 149:1722–1731.e9. doi: 10.1016/j.jaci.2021.10.034
57. Al-Amrani S, Al-Jabri Z, Al-Zaabi A, Alshekaili J, Al-Khabori M. Proteomics: concepts and applications in human medicine. World J Biol Chem. (2021) 12:57–69. doi: 10.4331/wjbc.v12.i5.57
58. Altelaar AFM, Munoz J, Heck AJR. Next-generation proteomics: towards an integrative view of proteome dynamics. Nat Rev Genet. (2013) 14:35–48. doi: 10.1038/nrg3356
59. Lundberg M, Thorsen SB, Assarsson E, Villablanca A, Tran B, Gee N, et al. Multiplexed homogeneous proximity ligation assays for high-throughput protein biomarker research in serological material. Mol Cell Proteomics. (2011) 10:M110.004978. doi: 10.1074/mcp.M110.004978
60. Vizcaíno JA, Côté RG, Csordas A, Dianes JA, Fabregat A, Foster JM, et al. The proteomics identifications (PRIDE) database and associated tools: status in 2013. Nucleic Acids Res. (2012) 41:D1063–9. doi: 10.1093/nar/gks1262
61. Fenyö D, Eriksson J, Beavis R. Mass spectrometric protein identification using the global proteome machine. Comput Biol. (2010) 673:189–202. doi: 10.1007/978-1-60761-842-3_11
62. Seltmann K, Meyer M, Sulcova J, Kockmann T, Wehkamp U, Weidinger S, et al. Humidity-regulated CLCA2 protects the epidermis from hyperosmotic stress. Sci Transl Med. (2018) 10(440):eaao4650. doi: 10.1126/scitranslmed.aao4650
63. Rusbjerg-Weberskov CE, Johansen ML, Nowak JS, Otzen DE, Pedersen JS, Enghild JJ, et al. Periostin C-terminal is intrinsically disordered and interacts with 143 proteins in an in vitro epidermal model of atopic dermatitis. Biochemistry. (2023) 62:2803–15. doi: 10.1021/acs.biochem.3c00176
64. Triana S, de Cock H, Ohm RA, Danies G, Wösten HAB, Restrepo S, et al. Lipid metabolic versatility in malassezia spp. yeasts studied through metabolic modeling. Front Microbiol. (2017) 8:1772. doi: 10.3389/fmicb.2017.01772
65. Sakabe J-I, Kamiya K, Yamaguchi H, Ikeya S, Suzuki T, Aoshima M, et al. Proteome analysis of stratum corneum from atopic dermatitis patients by hybrid quadrupole-orbitrap mass spectrometer. J Allergy Clin Immunol. (2014) 134:957–960.e8. doi: 10.1016/j.jaci.2014.07.054
66. Broccardo CJ, Mahaffey S, Schwarz J, Wruck L, David G, Schlievert PM, et al. Comparative proteomic profiling of patients with atopic dermatitis based on history of eczema herpeticum infection and Staphylococcus aureus colonization. J Allergy Clin Immunol. (2011) 127:186–193.e11. doi: 10.1016/j.jaci.2010.10.033
67. Broccardo CJ, Mahaffey SB, Strand M, Reisdorph NA, Leung DYM. Peeling off the layers: skin taping and a novel proteomics approach to study atopic dermatitis. J Allergy Clin Immunol. (2009) 124:1113–1115.e11. doi: 10.1016/j.jaci.2009.07.057
68. Sampson HA, McCaskill CC. Food hypersensitivity and atopic dermatitis: evaluation of 113 patients. J Pediatr. (1985) 107:669–75. doi: 10.1016/S0022-3476(85)80390-5
69. O’Loughlin S, Diaz-Perez JL, Gleich GJ, Winkelmann RK. Serum IgE in dermatitis and dermatosis: an analysis of 497 cases. Arch Dermatol. (1977) 113:309–15. doi: 10.1001/archderm.1977.01640030055008
70. de Benedictis FM, Franceschini F, Hill D, Naspitz C, Simons FER, Wahn U, et al. The allergic sensitization in infants with atopic eczema from different countries. Allergy. (2009) 64:295–303. doi: 10.1111/j.1398-9995.2008.01779.x
71. Noh G, Jin H, Lee J, Noh J, Lee WM, Lee S. Eosinophilia as a predictor of food allergy in atopic dermatitis. Allergy Asthma Proc. (2010) 31:e18–24. doi: 10.2500/aap.2010.31.3312
72. Johnson EE, Irons JS, Patterson R, Roberts M. Serum IgE concentration in atopic dermatitis. Relationship to severity of disease and presence of atopic respiratory disease. J Allergy Clin Immunol. (1974) 54:94–9. doi: 10.1016/0091-6749(74)90037-2
73. Ogawa M, Berger PA, McIntyre OR, Clendenning WE, Ishizaka K. IgE in atopic dermatitis. Arch Dermatol. (1971) 103:575–80. doi: 10.1001/archderm.1971.04000180001001
74. Clendenning WE, Clack WE, Ogawa M, Ishizaka K. Serum IgE studies in atopic dermatitis. J Invest Dermatol. (1973) 61:233–6. doi: 10.1111/1523-1747.ep12676481
75. Thijs J, Krastev T, Weidinger S, Buckens CF, de Bruin-Weller M, Bruijnzeel-Koomen C, et al. Biomarkers for atopic dermatitis. Curr Opin Allergy Clin Immunol. (2015) 15:453–60. doi: 10.1097/ACI.0000000000000198
76. Halling A-S, Rinnov MR, Ruge IF, Gerner T, Ravn NH, Knudgaard MH, et al. Skin TARC/CCL17 increase precedes the development of childhood atopic dermatitis. J Allergy Clin Immunol. (2023) 151:1550–1557.e6. doi: 10.1016/j.jaci.2022.11.023
77. Hijnen D, de Bruin-Weller M, Oosting B, Lebre C, de Jong E, Bruijnzeel-Koomen C, et al. Serum thymus and activation-regulated chemokine (TARC) and cutaneous T cell–attracting chemokine (CTACK) levels in allergic diseasesTARC and CTACK are disease-specific markers for atopic dermatitis. J Allergy Clin Immunol. (2004) 113:334–40. doi: 10.1016/j.jaci.2003.12.007
78. Kataoka Y. Thymus and activation-regulated chemokine as a clinical biomarker in atopic dermatitis. J Dermatol. (2014) 41:221–9. doi: 10.1111/1346-8138.12440
79. Yu L, Li L. Potential biomarkers of atopic dermatitis. Front Med (Lausanne). (2022) 9:1028694. doi: 10.3389/fmed.2022.1028694
80. Ungar B, Garcet S, Gonzalez J, Dhingra N, Correa da Rosa J, Shemer A, et al. An integrated model of atopic dermatitis biomarkers highlights the systemic nature of the disease. J Invest Dermatol. (2017) 137:603–13. doi: 10.1016/j.jid.2016.09.037
81. Facheris P, Jeffery J, Del Duca E, Guttman-Yassky E. The translational revolution in atopic dermatitis: the paradigm shift from pathogenesis to treatment. Cell Mol Immunol. (2023) 20:448–74. doi: 10.1038/s41423-023-00992-4
82. Imai Y. Interleukin-33 in atopic dermatitis. J Dermatol Sci. (2019) 96:2–7. doi: 10.1016/j.jdermsci.2019.08.006
83. Szabó K, Gáspár K, Dajnoki Z, Papp G, Fábos B, Szegedi A, et al. Expansion of circulating follicular T helper cells associates with disease severity in childhood atopic dermatitis. Immunol Lett. (2017) 189:101–8. doi: 10.1016/j.imlet.2017.04.010
84. Li M, Hener P, Zhang Z, Ganti KP, Metzger D, Chambon P. Induction of thymic stromal lymphopoietin expression in keratinocytes is necessary for generating an atopic dermatitis upon application of the active vitamin D3 analogue MC903 on mouse skin. J Invest Dermatol. (2009) 129:498–502. doi: 10.1038/jid.2008.232
85. Marschall P, Wei R, Segaud J, Yao W, Hener P, German BF, et al. Dual function of langerhans cells in skin TSLP-promoted TFH differentiation in mouse atopic dermatitis. J Allergy Clin Immunol. (2021) 147:1778–94. doi: 10.1016/j.jaci.2020.10.006
86. Kitajima M, Kubo M, Ziegler SF, Suzuki H. Critical role of TSLP receptor on CD4 T cells for exacerbation of skin inflammation. The J Immunol. (2020) 205:27–35. doi: 10.4049/jimmunol.1900758
87. Ballesteros-Tato A, Randall TD, Lund FE, Spolski R, Leonard WJ, León B. T follicular helper cell plasticity shapes pathogenic T helper 2 cell-mediated immunity to inhaled house dust mite. Immunity. (2016) 44:259–73. doi: 10.1016/j.immuni.2015.11.017
88. Dolence JJ, Kobayashi T, Iijima K, Krempski J, Drake LY, Dent AL, et al. Airway exposure initiates peanut allergy by involving the IL-1 pathway and T follicular helper cells in mice. J Allergy Clin Immunol. (2018) 142:1144–1158.e8. doi: 10.1016/j.jaci.2017.11.020
89. Furue M, Ulzii D, Vu YH, Tsuji G, Kido-Nakahara M, Nakahara T. Pathogenesis of atopic dermatitis: current paradigm. Iran J Immunol. (2019) 16:97–107. doi: 10.22034/IJI.2019.80253
90. Thijs JL, Strickland I, Bruijnzeel-Koomen CAFM, Nierkens S, Giovannone B, Csomor E, et al. Moving toward endotypes in atopic dermatitis: identification of patient clusters based on serum biomarker analysis. J Allergy Clin Immunol. (2017) 140:730–7. doi: 10.1016/j.jaci.2017.03.023
91. Bakker DS, Nierkens S, Knol EF, Giovannone B, Delemarre EM, van der Schaft J, et al. Confirmation of multiple endotypes in atopic dermatitis based on serum biomarkers. J Allergy Clin Immunol. (2021) 147:189–98. doi: 10.1016/j.jaci.2020.04.062
92. Hoffmann-Sommergruber K, Pfeifer S, Bublin M. Applications of molecular diagnostic testing in food allergy. Curr Allergy Asthma Rep. (2015) 15:56. doi: 10.1007/s11882-015-0557-6
93. Di Girolamo F, Muraca M, Mazzina O, Lante I, Dahdah L. Proteomic applications in food allergy. Curr Opin Allergy Clin Immunol. (2015) 15:259–66. doi: 10.1097/ACI.0000000000000160
94. Kukkonen AK, Pelkonen AS, Mäkinen-Kiljunen S, Voutilainen H, Mäkelä MJ. Ara h 2 and Ara 6 are the best predictors of severe peanut allergy: a double-blind placebo-controlled study. Allergy. (2015) 70:1239–45. doi: 10.1111/all.12671
95. Ruiter B, Knol EF, Van Neerven RJJ, Garssen J, Bruijnzeel-Koomen CAFM, Knulst AC, et al. Maintenance of tolerance to cow’s milk in atopic individuals is characterized by high levels of specific immunoglobulin G4. Clin Exp Allergy. (2007) 37:1103–10. doi: 10.1111/j.1365-2222.2007.02749.x
96. Sommanus S. Cow’s milk protein allergy: immunological response in children with cow’s milk protein tolerance. Asian Pac J Allergy Immunol. (2013) 32(2):171–7. doi: 10.12932/AP0319.32.2.2013
97. Esmaeilzadeh H, Alyasin S, Haghighat M, Nabavizadeh H, Esmaeilzadeh E, Mosavat F. The effect of baked milk on accelerating unheated cow’s milk tolerance: a control randomized clinical trial. Pediatr Allergy Immunol. (2018) 29:747–53. doi: 10.1111/pai.12958
98. Fishbein AB, Qamar N, Erickson KA, Kwasny MJ, Cai M, Szychlinski C, et al. Cytokine responses to egg protein in previously allergic children who developed tolerance naturally. Ann Allergy Asthma Immunol. (2014) 113:667–670.e4. doi: 10.1016/j.anai.2014.09.002
99. Qamar N, Fishbein AB, Erickson KA, Cai M, Szychlinski C, Bryce PJ, et al. Naturally occurring tolerance acquisition to foods in previously allergic children is characterized by antigen specificity and associated with increased subsets of regulatory T cells. Clin Exp Allergy. (2015) 45:1663–72. doi: 10.1111/cea.12570
100. Turcanu V, Maleki SJ, Lack G. Characterization of lymphocyte responses to peanuts in normal children, peanut-allergic children, and allergic children who acquired tolerance to peanuts. J Clin Invest. (2003) 111:1065–72. doi: 10.1172/JCI16142
101. Leonard SA, Sampson HA, Sicherer SH, Noone S, Moshier EL, Godbold J, et al. Dietary baked egg accelerates resolution of egg allergy in children. J Allergy Clin Immunol. (2012) 130:473–480.e1. doi: 10.1016/j.jaci.2012.06.006
102. Yang D, Han Z, Oppenheim JJ. Alarmins and immunity. Immunol Rev. (2017) 280:41–56. doi: 10.1111/imr.12577
103. Oppenheim JJ, Tewary P, de la Rosa G, Yang D. Alarmins initiate host defense. Adv Exp Med Biol. (2007) 601:185–94. doi: 10.1007/978-0-387-72005-0_19
104. Sahiner UM, Layhadi JA, Golebski K, István Komlósi Z, Peng Y, Sekerel B, et al. Innate lymphoid cells: the missing part of a puzzle in food allergy. Allergy. (2021) 76:2002–16. doi: 10.1111/all.14776
105. Hussain M, Borcard L, Walsh KP, Pena Rodriguez M, Mueller C, Kim BS, et al. Basophil-derived IL-4 promotes epicutaneous antigen sensitization concomitant with the development of food allergy. J Allergy Clin Immunol. (2018) 141:223–234.e5. doi: 10.1016/j.jaci.2017.02.035
106. Brough HA, Nadeau KC, Sindher SB, Alkotob SS, Chan S, Bahnson HT, et al. Epicutaneous sensitization in the development of food allergy: what is the evidence and how can this be prevented? Allergy. (2020) 75:2185–205. doi: 10.1111/all.14304
107. Koszewicz M, Mulak A, Dziadkowiak E, Budrewicz S. Is fecal calprotectin an applicable biomarker of gut immune system activation in chronic inflammatory demyelinating polyneuropathy?—A pilot study. Front Hum Neurosci. (2021) 15:733070. doi: 10.3389/fnhum.2021.733070
108. Ayling RM, Kok K. Fecal calprotectin. Adv Clin Chem. (2018) 87:161–90. doi: 10.1016/bs.acc.2018.07.005
109. Shabani F, Farasat A, Mahdavi M, Gheibi N. Calprotectin (S100A8/S100A9): a key protein between inflammation and cancer. Inflammation Res. (2018) 67:801–12. doi: 10.1007/s00011-018-1173-4
110. Abraham BP, Kane S. Fecal markers: calprotectin and lactoferrin. Gastroenterol Clin North Am. (2012) 41:483–95. doi: 10.1016/J.GTC.2012.01.007
111. Calvani M, Anania C, Cuomo B, D'auria E, Decimo F, Indirli GC, et al. Non-IgE- or mixed IgE/non-IgE-mediated gastrointestinal food allergies in the first years of life: old and new tools for diagnosis. Nutrients. (2021) 13:1–31. doi: 10.3390/NU13010226
112. Zhang Z, Wang W, Zhang X, Pan J, Chen X. Fecal calprotectin in children with cow’s milk protein allergy: a systematic review and meta-analysis. Int Arch Allergy Immunol. (2022) 183:1189–97. doi: 10.1159/000525961
113. Qiu L, Wang J, Ren F, Shen L, Li F. Can fecal calprotectin levels be used to monitor infant milk protein allergies? Allergy, Asthma Clin Immunol. (2021) 17:132. doi: 10.1186/s13223-021-00636-0
114. Xiong L-J, Xie X-L, Li Y, Deng X-Z. Current status of fecal calprotectin as a diagnostic or monitoring biomarker for cow’s milk protein allergy in children: a scoping review. World J Pediatr. (2021) 17:63–70. doi: 10.1007/s12519-020-00364-2
115. Hou K, Wu Z-X, Chen X-Y, Wang J-Q, Zhang D, Xiao C, et al. Microbiota in health and diseases. Signal Transduct Target Ther. (2022) 7:135. doi: 10.1038/s41392-022-00974-4
116. Berg G, Rybakova D, Fischer D, Cernava T, Vergès M-CC, Charles T, et al. Microbiome definition re-visited: old concepts and new challenges. Microbiome. (2020) 8:103. doi: 10.1186/s40168-020-00875-0
117. Pantoja-Feliciano IG, Clemente JC, Costello EK, Perez ME, Blaser MJ, Knight R, et al. Biphasic assembly of the murine intestinal microbiota during early development. ISME J. (2013) 7:1112–5. doi: 10.1038/ismej.2013.15
118. Koenig JE, Spor A, Scalfone N, Fricker AD, Stombaugh J, Knight R, et al. Succession of microbial consortia in the developing infant gut microbiome. Proc Natl Acad Sci USA. (2011) 108:4578–85. doi: 10.1073/pnas.1000081107
119. Coppa GV, Bruni S, Morelli L, Soldi S, Gabrielli O. The first prebiotics in humans. J Clin Gastroenterol. (2004) 38:S80–3. doi: 10.1097/01.mcg.0000128926.14285.25
120. Olson S. The national academy of sciences at 150. Proc Natl Acad Sci USA. (2014) 111:9327–64. doi: 10.1073/pnas.1406109111
121. Barile D, Rastall RA. Human milk and related oligosaccharides as prebiotics. Curr Opin Biotechnol. (2013) 24:214–9. doi: 10.1016/j.copbio.2013.01.008
122. Dominguez-Bello MG, Costello EK, Contreras M, Magris M, Hidalgo G, Fierer N, et al. Delivery mode shapes the acquisition and structure of the initial microbiota across multiple body habitats in newborns. Proc Natl Acad Sci USA. (2010) 107:11971–5. doi: 10.1073/pnas.1002601107
123. Mueller NT, Bakacs E, Combellick J, Grigoryan Z, Dominguez-Bello MG. The infant microbiome development: mom matters. Trends Mol Med. (2015) 21:109–17. doi: 10.1016/J.MOLMED.2014.12.002
124. Wesemann DR, Nagler CR. The microbiome, timing, and barrier function in the context of allergic disease. Immunity. (2016) 44:728–38. doi: 10.1016/j.immuni.2016.02.002
125. Papathoma E, Triga M, Fouzas S, Dimitriou G. Cesarean section delivery and development of food allergy and atopic dermatitis in early childhood. Pediatr Allergy Immunol. (2016) 27:419–24. doi: 10.1111/pai.12552
126. Metsälä J, Lundqvist A, Virta LJ, Kaila M, Gissler M, Virtanen SM. Mother’s and offspring’s use of antibiotics and infant allergy to cow’s milk. Epidemiology. (2013) 24:303–9. doi: 10.1097/EDE.0b013e31827f520f
127. Lee S-Y, Yu J, Ahn K-M, Kim KW, Shin YH, Lee K, et al. Additive effect between IL-13 polymorphism and cesarean section delivery/prenatal antibiotics use on atopic dermatitis: a birth cohort study (COCOA). PLoS One. (2014) 9:e96603. doi: 10.1371/journal.pone.0096603
128. El-Heis S, Crozier SR, Harvey NC, Healy E, Godfrey KM. Early life exposure to antibiotics and laxatives in relation to infantile atopic eczema. Pediatr Allergy Immunol. (2023) 34(5):e13964. doi: 10.1111/pai.13964
129. Dhariwala MO, Scharschmidt TC. Baby’s skin bacteria: first impressions are long-lasting. Trends Immunol. (2021) 42:1088–99. doi: 10.1016/j.it.2021.10.005
130. Paller AS, Kong HH, Seed P, Naik S, Scharschmidt TC, Gallo RL, et al. The microbiome in patients with atopic dermatitis. J Allergy Clin Immunol. (2019) 143:26–35. doi: 10.1016/j.jaci.2018.11.015
131. Koh LF, Ong RY, Common JE. Skin microbiome of atopic dermatitis. Allergol Int. (2022) 71:31–9. doi: 10.1016/J.ALIT.2021.11.001
132. Key FM, Khadka VD, Romo-González C, Blake KJ, Deng L, Lynn TC, et al. On-person adaptive evolution of Staphylococcus aureus during treatment for atopic dermatitis. Cell Host Microbe. (2023) 31:593–603.e7. doi: 10.1016/j.chom.2023.03.009
133. Geoghegan JA, Irvine AD, Foster TJ. Staphylococcus aureus and atopic dermatitis: a complex and evolving relationship. Trends Microbiol. (2018) 26:484–97. doi: 10.1016/j.tim.2017.11.008
134. Waldman S, Terzic A. Clinical pharmacology and therapeutics : past, present, and future. Clin Pharmacol Ther. (2017) 101:300–3. doi: 10.1002/cpt.592
135. Preis S, Schmidt L, Tizek L, Schielein M, Lang V, Bleuel R, et al. Munich atopy prediction study (MAPS): protocol for a prospective birth cohort addressing clinical and molecular risk factors for atopic dermatitis in early childhood. BMJ Open. (2022) 12:e059256. doi: 10.1136/bmjopen-2021-059256
136. Dwyer LR, Scharschmidt TC. Early life host-microbe interactions in skin. Cell Host Microbe. (2022) 30:684–95. doi: 10.1016/j.chom.2022.02.016
137. Scharschmidt TC, Vasquez KS, Truong H-A, Gearty SV, Pauli ML, Nosbaum A, et al. A wave of regulatory T cells into neonatal skin mediates tolerance to commensal microbes. Immunity. (2015) 43:1011–21. doi: 10.1016/j.immuni.2015.10.016
138. Belkaid Y, Segre JA. Dialogue between skin microbiota and immunity. Science. (2014) 346:954–9. doi: 10.1126/science.1260144
139. O’Neill CA, Monteleone G, McLaughlin JT, Paus R. The gut-skin axis in health and disease: a paradigm with therapeutic implications. BioEssays. (2016) 38:1167–76. doi: 10.1002/bies.201600008
140. Moniaga CS, Tominaga M, Takamori K. An altered skin and gut microbiota are involved in the modulation of itch in atopic dermatitis. Cells. (2022) 11:3930. doi: 10.3390/cells11233930
141. Lee N, Kim WU. Microbiota in T-cell homeostasis and inflammatory diseases. Exp Mol Med. (2017) 49:e340. doi: 10.1038/EMM.2017.36
142. Halsey TM, Thomas AS, Hayase T, Ma W, Abu-Sbeih H, Sun B, et al. Microbiome alteration via fecal microbiota transplantation is effective for refractory immune checkpoint inhibitor–induced colitis. Sci Transl Med. (2023) 15(700):eabq4006. doi: 10.1126/scitranslmed.abq4006
143. Aitoro R, Paparo L, Amoroso A, Di Costanzo M, Cosenza L, Granata V, et al. Gut Microbiota as a target for preventive and therapeutic intervention against food allergy. Nutrients. (2017) 9:672. doi: 10.3390/nu9070672
144. Dong P, Feng J-J, Yan D-Y, Lyu Y-J, Xu X. Early-life gut microbiome and cow’s milk allergy- a prospective case—control 6-month follow-up study. Saudi J Biol Sci. (2018) 25:875–80. doi: 10.1016/j.sjbs.2017.11.051
145. Renz H, Skevaki C. Early life microbial exposures and allergy risks: opportunities for prevention. Nat Rev Immunol. (2021) 21:177–91. doi: 10.1038/s41577-020-00420-y
146. Round JL, Mazmanian SK. The gut microbiota shapes intestinal immune responses during health and disease. Nat Rev Immunol. (2009) 9:313–23. doi: 10.1038/NRI2515
147. West CE, Jenmalm MC, Prescott SL. The gut microbiota and its role in the development of allergic disease: a wider perspective. Clin Exp Allergy. (2015) 45:43–53. doi: 10.1111/cea.12332
148. Egert M, Simmering R, Riedel CU. The association of the skin microbiota with health, immunity, and disease. Clin Pharmacol Ther. (2017) 102:62–9. doi: 10.1002/CPT.698
149. Smith PM, Howitt MR, Panikov N, Michaud M, Gallini CA, Bohlooly-Y M, et al. The microbial metabolites, short-chain fatty acids, regulate colonic treg cell homeostasis. Science. (2013) 341:569–73. doi: 10.1126/SCIENCE.1241165
150. Fusco W, Lorenzo MB, Cintoni M, Porcari S, Rinninella E, Kaitsas F, et al. Short-chain fatty-acid-producing bacteria: key components of the human gut microbiota. Nutrients. (2023) 15:2211. doi: 10.3390/nu15092211
151. Lozo J, Topisirovic L, Kojic M. Natural bacterial isolates as an inexhaustible source of new bacteriocins. Appl Microbiol Biotechnol. (2021) 105:477–92. doi: 10.1007/s00253-020-11063-3
152. Wen X, Xie R, Wang H-G, Zhang M-N, He L, Zhang M-H, et al. Fecal microbiota transplantation alleviates experimental colitis through the toll-like receptor 4 signaling pathway. World J Gastroenterol. (2023) 29:4657–70. doi: 10.3748/wjg.v29.i30.4657
153. Ortega-Martín L, Sastre B, Rodrigo-Muñoz J, Cañas J, Valverde-Monge M, Del Pozo V. Anaphylaxis after mango fruit intake: identification of new allergens. J Investig Allergol Clin Immunol. (2022) 32:401–3. doi: 10.18176/jiaci.0766
154. Abdel-Gadir A, Stephen-Victor E, Gerber GK, Noval Rivas M, Wang S, Harb H, et al. Microbiota therapy acts via a regulatory T cell MyD88/RORγt pathway to suppress food allergy. Nat Med. (2019) 25:1164–74. doi: 10.1038/s41591-019-0461-z
155. Peroni DG, Nuzzi G, Trambusti I, Di Cicco ME, Comberiati P. Microbiome composition and its impact on the development of allergic diseases. Front Immunol. (2020) 11:700. doi: 10.3389/fimmu.2020.00700
156. Wishart DS, Guo A, Oler E, Wang F, Anjum A, Peters H, et al. HMDB 5.0: the human metabolome database for 2022. Nucleic Acids Res. (2022) 50:D622–31. doi: 10.1093/nar/gkab1062
157. Darlenski R, Fluhr JW. How do the skin barrier and microbiome adapt to the extra-uterine environment after birth? Implications for the clinical practice. Int J Cosmet Sci. (2023) 45:288–98. doi: 10.1111/ics.12844
158. Díaz-Perales A, Escribese MM, Garrido-Arandia M, Obeso D, Izquierdo-Alvarez E, Tome-Amat J, et al. The role of sphingolipids in allergic disorders. Front Allergy. (2021) 2:675557. doi: 10.3389/falgy.2021.675557
159. Dessì A, Di Maria C, Pintus R, Fanos V, Bosco A. Lipidomics and metabolomics in infant atopic dermatitis: what’s the correlation with early nutrition? Curr Pediatr Rev. (2023) 20. doi: 10.2174/1573396320666230411093122
160. De Pessemier B, Grine L, Debaere M, Maes A, Paetzold B, Callewaert C. Gut–skin axis: current knowledge of the interrelationship between microbial dysbiosis and skin conditions. Microorganisms. (2021) 9:353. doi: 10.3390/microorganisms9020353
161. Schwarz A, Bruhs A, Schwarz T. The short-chain fatty acid sodium butyrate functions as a regulator of the skin immune system. J Invest Dermatol. (2017) 137:855–64. doi: 10.1016/J.JID.2016.11.014
162. Bowe WP, Logan AC. Acne vulgaris, probiotics and the gut-brain-skin axis—back to the future? Gut Pathog. (2011) 3:1. doi: 10.1186/1757-4749-3-1
163. Arck P, Handjiski B, Hagen E, Pincus M, Bruenahl C, Bienenstock J, et al. Is there a "gut–brain–skin axis"? Exp Dermatol. (2010) 19:401–5. doi: 10.1111/j.1600-0625.2009.01060.x
164. Rhew K, Oh JM. Association between atopic disease and anemia in pediatrics: a cross-sectional study. BMC Pediatr. (2019) 19:455. doi: 10.1186/s12887-019-1836-5
165. Drury KE, Schaeffer M, Silverberg JI. Association between atopic disease and anemia in US children. JAMA Pediatr. (2016) 170:29. doi: 10.1001/jamapediatrics.2015.3065
166. Roth-Walter F. Iron-deficiency in atopic diseases: innate immune priming by allergens and siderophores. Front Allergy. (2022) 3:859922. doi: 10.3389/falgy.2022.859922
167. Golpanian RS, Aickara DJ, Bellodi Schmidt F, Smith PK, Yosipovitch G. Hydrolysed formula, delayed food introduction and fatty acids for atopic dermatitis prevention in infancy. Acta Paediatr. (2021) 110:1784–7. doi: 10.1111/apa.15742
168. Pavel P, Leman G, Hermann M, Ploner C, Eichmann TO, Minzaghi D, et al. Peroxisomal fatty acid oxidation and glycolysis are triggered in mouse models of lesional atopic dermatitis. JID Innov. (2021) 1:100033. doi: 10.1016/j.xjidi.2021.100033
169. Pike KC, Calder PC, Inskip HM, Robinson SM, Roberts GC, Cooper C, et al. Maternal plasma phosphatidylcholine fatty acids and atopy and wheeze in the offspring at age of 6 years. Clin Dev Immunol. (2012) 2012:1–13. doi: 10.1155/2012/474613
170. Barlianto W, Wulandari D, Sari T, Firdayanti V, Avandi M. Vitamin D, cytokine profiles, and disease severity in infants with atopic dermatitis: a single centre, cross-sectional study. Adv Dermatol Allergol. (2022) 39:793–9. doi: 10.5114/ada.2022.118923
171. Goretzki A, Zimmermann J, Lin Y-J, Schülke S. Immune metabolism–an opportunity to better understand allergic pathology and improve treatment of allergic diseases? Front Allergy. (2022) 3:825931. doi: 10.3389/falgy.2022.825931
Keywords: atopy, atopic dermatitis, biomarkers, early life, food allergy, metabolome, microbiome, proteome
Citation: Zubeldia-Varela E, Ibáñez-Sandín MD, Gomez-Casado C and Pérez-Gordo M (2024) Allergy-associated biomarkers in early life identified by Omics techniques. Front. Allergy 5:1359142. doi: 10.3389/falgy.2024.1359142
Received: 20 December 2023; Accepted: 12 February 2024;
Published: 23 February 2024.
Edited by:
Elina Toskala, Thomas Jefferson University, United StatesReviewed by:
Anna Maria Ehlers, Utrecht University, Netherlands© 2024 Zubeldia-Varela, Ibáñez-Sandín, Gomez-Casado and Pérez-Gordo. This is an open-access article distributed under the terms of the Creative Commons Attribution License (CC BY). The use, distribution or reproduction in other forums is permitted, provided the original author(s) and the copyright owner(s) are credited and that the original publication in this journal is cited, in accordance with accepted academic practice. No use, distribution or reproduction is permitted which does not comply with these terms.
*Correspondence: Marina Pérez-Gordo bWFyaW5hLnBlcmV6Z29yZG9AY2V1LmVz
†These authors have contributed equally to this work
‡ORCID Elisa Zubeldia-Varela orcid.org/0000-0002-3030-6313 María Dolores Ibáñez-Sandín orcid.org/0000-0001-8291-5121 Cristina Gomez-Casado orcid.org/0000-0002-7707-6367 Marina Pérez-Gordo orcid.org/0000-0003-2003-4841
Disclaimer: All claims expressed in this article are solely those of the authors and do not necessarily represent those of their affiliated organizations, or those of the publisher, the editors and the reviewers. Any product that may be evaluated in this article or claim that may be made by its manufacturer is not guaranteed or endorsed by the publisher.
Research integrity at Frontiers
Learn more about the work of our research integrity team to safeguard the quality of each article we publish.