- 1Multiphase Chemistry Department, Max Planck Institute for Chemistry, Mainz, Germany
- 2Division 1.5 - Protein Analysis, Federal Institute for Materials Research and Testing (BAM), Berlin, Germany
Protein modifications such as oligomerization and tyrosine nitration alter the immune response to allergens and may contribute to the increasing prevalence of allergic diseases. In this mini-review, we summarize and discuss relevant findings for the major birch and grass pollen allergens Bet v 1 and Phl p 5 modified with tetranitromethane (laboratory studies), peroxynitrite (physiological processes), and ozone and nitrogen dioxide (environmental conditions). We focus on tyrosine nitration and the formation of protein dimers and higher oligomers via dityrosine cross-linking and the immunological effects studied.
1. Introduction
Tyrosine nitration and dityrosine cross-linking are post-translational protein modifications that occur under oxidative conditions. Tyrosine nitration involves the replacement of a hydrogen atom on the aromatic ring of the amino acid with a nitro group, while dityrosine cross-linking refers to the formation of covalent bonds between two tyrosine residues resulting in protein dimers and oligomers. These modifications affect protein structure and function and are associated with various diseases (1–3). They serve as biomarkers of oxidative damage in proteins, providing insight into the role of oxidative stress in disease development and progression (4–6). These tyrosine modifications also play a crucial role in altering the immune responses to allergens and may contribute to the increasing prevalence of allergic diseases and their association with traffic-related air pollution (7–12).
There are a variety of airborne allergen sources including plant pollen, fungal spores, and other biological aerosol particles. The allergenic proteins are released into the atmosphere when these particles rupture in response to humidity, exposure to anthropogenic air pollutants such as ozone (O) and nitrogen dioxide (NO), or mechanical influences (13–20). In the atmosphere, the proteins can react with reactive oxygen and nitrogen species (ROS/RNS), peroxyacetyl nitrate, or undergo photo-oxidation by UV radiation (10, 21–27). These reactions promote chemical protein modifications such as tyrosine nitration and dityrosine cross-linking via the formation of radical intermediates. In particular, summer smog conditions with high O and NO concentrations have been shown to efficiently nitrate and cross-link proteins within hours to days (21, 28–30). Air pollutants such as O and NO, and particulate matter can also induce or enhance oxidative stress and inflammatory processes leading to the formation of endogenous ROS/RNS such as peroxynitrite (ONOO), which is the main mediator of physiological tyrosine nitration and oligomerization (10, 31–34). In addition to the naturally occurring tyrosine nitration and cross-linking reactions, artificial protein nitration and dityrosine formation can be achieved by reaction with tetranitromethane (TNM), a standard laboratory reagent. While the TNM nitration lacks biological relevance, its usage in laboratory studies provides valuable insights into the consequences of tyrosine nitration and cross-linking in a protein molecule (8, 9, 28, 29, 35, 36). In addition to tyrosine nitration and dityrosine cross-linking, the reaction of proteins with oxidants can also result in other tyrosine modifications such as tyrosine nitrosylation, in the modification of other amino acids, and in protein degradation (29, 37–40).
The birch pollen allergen Bet v 1 and grass pollen allergen Phl p 5 are major airborne allergens in Central Europe (41–45). Both allergens serve as important diagnostic markers for genuine birch or grass pollen sensitization (44–47). In recent years, several studies have investigated the reactions of nitrating agents with the two allergens. Here, we summarize relevant findings on the chemical modification of these allergens by TNM, peroxynitrite, polluted air, O/NO gas mixtures, and their effects on innate and adaptive immune responses and discuss future research perspectives.
2. Tyrosine nitration
The proportion of nitrated tyrosine residues in a protein can be represented by the tyrosine nitration degree (ND) and provides information about the extent of nitrosative and oxidative damage in a protein. It is defined as the ratio of nitrated tyrosine residues to the total number of tyrosine residues of a protein molecule. The ND of a protein can be determined by a variety of chromatographic, immunochemical, and mass spectrometry-based methods (28, 48–53).
For Bet v 1, the reaction with TNM yielded nitration degrees of up to 70% compared to peroxynitrite with up to 50% and to O/NO achieving up to 22% nitration (29). The reaction of Bet v 1 with polluted air resulted in lower nitration degrees of 10% but shows that the allergen can be nitrated under environmental conditions (21). In contrast to Bet v 1, the grass pollen allergen Phl p 5 has received less attention with respect to tyrosine nitration. Only recently, Backes et al. (30) reported Phl p 5 nitration degrees of up to 40 % for the reaction with ONOO and up to 10% for O/NO exposure.
The determination of the total nitration degree is particularly useful for kinetic investigations of tyrosine nitration, but not all tyrosine residues of a protein are nitrated with the same efficiency (52). Factors that determine the selectivity of tyrosine for the nitration reaction are the protein structure and the position of the tyrosine residues, the nitrating agent and the reaction conditions (10, 52, 54). Exposure of the aromatic ring to the surface of the protein, the location of the tyrosine within a loop structure, and its proximity to an adjacent negative charge favor nitration (55).
Determining the preferred nitration sites is particularly important for understanding the impact of nitration on protein functionality and interactions with the immune system. The total ND can be misleading when specific, biologically relevant tyrosine residues are highly nitrated while others remain unmodified as a single highly nitrated tyrosine, even in the background of relatively low total ND, can have a significant impact on protein structure and function.
Figure 1 shows the positions and the relative solvent accessibility (RSA) of the tyrosine residues for both allergens based on protein databank entries PDB 4A88 (Bet v 1) and PDB 2M64 (Phl p 5) (56, 57). Bet v 1 consists of 159 amino acids and contains seven tyrosine residues (Y5, Y66, Y81, Y83, Y120, Y150, Y158) as potential nitration sites. Based on the RSA values, all tyrosine residues from Bet v 1 are well accessible for modification, with the exception of Y120. This is in agreement with Karle et al. (9), who predicted for another databank entry of Bet v 1 (PDB 1BV1) and based on accessibility and electrostatics that Y5, Y81, Y83, Y150, and Y158 are preferentially nitrated, while the positively charged environment of Y66 disfavors nitration, and Y120 is inaccessible due to its shielded position. Gusenkov et al. (59) characterized ONOO-modified Bet v 1 (at ONOO to tyrosine molar ratios of 1:1 and 5:1) and could distinguish up to 12 variants with one to sixfold nitration. The three- to sixfold nitrated variants were detected only in samples modified with higher amounts of ONOO (molar ratio 5:1). Their results indicate the occurrence of Bet v 1 variants with identical nitration degrees but site-specific nitration.
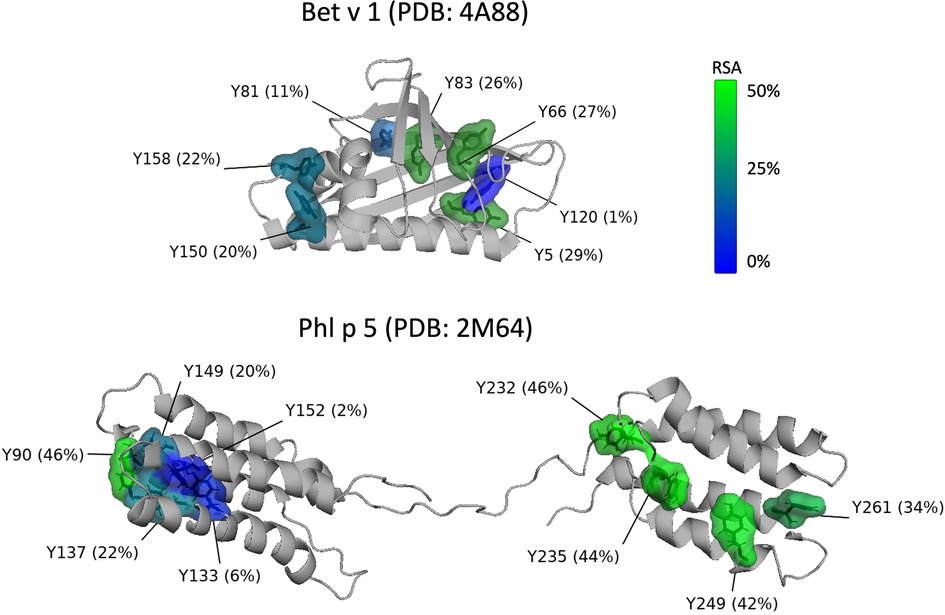
Figure 1. Position and relative solvent accessibility (RSA) of the tyrosine residues in the 3D-structure (ribbon view) of Bet v 1.0101 (PDB accession code: 4A88) (56) and Phl p 5.0101 (PDB accession code: 2M64, assembly 1) (57) created with the PyMOL Molecular Graphics System, Version 2.5.2 Schrödinger, LLC (58). Tyrosine residues are displayed as molecular surface and numbered according to the amino acid sequence of full-length Bet v 1 and Phl p 5. Coloring of the tyrosine residues is according to their RSA from blue (0%, buried) to green (50%, exposed).
Reinmuth-Selzle et al. (29) found that the preferred reaction sites of Bet v 1 vary depending on the nitrating agent. Residues Y81 and Y83 were the preferred nitration sites for TNM, Y83 and Y158 for O/NO, and Y150 for ONOO. These tyrosine residues have high solvent accessibility and are located in hydrophobic protein environments. Residues Y150 and Y158 are located in the C-terminal helix and Y81 and Y83 in the hydrophobic cavity, both key positions for the binding of specific IgE as well as ligands such as fatty acids, cytokines, and flavonoids (29). Low levels of nitration were achieved for Y81 (O/NO), Y150 (TNM, O/NO), and Y158 (ONOO). Y5 and Y120 nitration could not be detected and Y66 nitration was only found for ONOO-modified Bet v 1, but could not be quantified. Gusenkov and Stutz (60) later found that the reaction with ONOO leads to nitration of six tyrosine residues (Y5, Y66, Y81, Y83, Y150, Y158) with preferential nitration of the two surface-exposed tyrosine residues Y5 and Y66.
Site-specific nitration and different variants can also be expected for Phl p 5, but so far, to our knowledge, the preferred nitration sites have not been determined for the grass pollen allergen. After cleavage of the 25 amino acid signal peptide, the mature Phl p 5 consists of 287 amino acids including 12 tyrosine residues: Y30, Y42, Y90, Y133, Y137, Y149, Y152, Y232, Y235, Y249, Y261, and Y310. The 3-D ribbon structure of Phl p 5 is shown in Figure 1. Because the available structure covers only the amino acid residues 55 to 285 only nine tyrosine residues (Y90-Y261) are included. The protein consists of two domains that are flexibly connected by a central linker region. Each domain is formed by a 4-helix bundle stabilized by a hydrophobic core (57).
Applying the commonly used RSA threshold of 20% to define buried and exposed residues, two out of the nine tyrosine residues (Y133, Y152) can be classified as buried, while the other seven residues (Y90, Y137, Y149, Y232, Y235, Y249, Y261) are surface exposed and can be expected to be more susceptible to nitration (55, 61, 62). Further studies are needed to determine the preferred nitration sites in the grass pollen allergen.
3. Dityrosine cross-linking
Dityrosine cross-linking can lead to protein oligomerization and the formation of insoluble protein. In contrast to tyrosine nitration, less is known about the oligomerization via dityrosine cross-linking for the two allergens as the initial studies focused on tyrosine nitration. However, dimer and trimer formation was observed but not quantified for the reaction of Bet v 1 with TNM (9, 35). The reaction of Bet v 1 with ONOO yielded up to 42% protein dimers and higher oligomers (63). For Bet v 1, the tyrosine residues, Y5, Y66 and Y150 were suggested to be involved in the dityrosine cross-linking due to their solvent-exposed position (35, 64).
For Phl p 5, exposure to O/NO and ONOO lead to the formation of protein dimers and higher oligomers for up to 50% of the protein mass (30, 63). For dimerization and further oligomerization, the effect of steric hindrance, however, is likely more important than for the nitration reaction (22). Thus, for Phl p 5, the tyrosine residues Y90, Y232, Y235, Y249, and Y261 could potentially be involved in dityrosine formation due to their high solvent exposure (Figure 1). For both allergens, further studies are needed to determine the preferred reaction sites for dityrosine cross-linking and to investigate the extent of cross-linking under different reaction conditions. Since different structural dimers may have different biological effects, a site-selective characterization is also required for dityrosine cross-links, similar to the concept of the tyrosine-specific degree of nitration.
4. Influence of the reaction conditions on tyrosine nitration and dityrosine formation
Several studies have investigated the influence of experimental conditions on the extent of tyrosine nitration and dityrosine cross-linking. For example, for the reaction with TNM, the ND of Bet v 1 strongly depends on the molar ratio of TNM to tyrosine residues (29), whereas the reaction time seems to be less important (51). Similarly, also for the reaction with ONOO, the ND depends on the molar ratio of ONOO to tyrosine residues (29, 30, 63). In contrast to the reaction with TNM, the ND for the reaction of Bet v 1 with ONOO depends on the reaction time. Shorter reaction times (15 min vs. 100 min) lead to higher NDs and, in addition, low temperatures (4C vs. RT) and the addition of a chelator diethylenetriamine pentaacetic acid (DTPA), which prevents the reaction of ONOO with metal ions, such as iron or copper, favor nitration (29). Reaction time and temperature affect the generally rapid degradation of ONOO and the degree of protein degradation, which increases with increasing reaction time and temperature.
The reaction with O/NO is strongly influenced by changes in the O concentration and rather insensitive to changes in the NO concentration (30, 65). For the grass pollen allergen Phl p 5, higher nitration and oligomerization was observed at higher gas concentrations and longer reaction times, but the highest oligomerization was achieved for the reaction with O alone (30). This can be explained by a competitive reaction of the tyrosyl radical formed in a first reaction step with O, which either reacts with an NO molecule, leading to the formation of 3-nitrotyrosine, or with another tyrosyl radical, forming a dityrosine cross-link (22, 66). Without the addition of NO, there is no competitive reaction resulting in higher oligomerization of the protein. Higher temperatures can increase the rate of tyrosyl radical formation by ozonolysis, contributing to the formation of nitrated and cross-linked protein species, especially under tropical and summer smog conditions (21, 65, 67). In this context, also the lifetime of an allergen in the air becomes important as a longer atmospheric residence time increases the chances for chemical modification by O and NO.
Under atmospheric conditions, the reaction rates also depend on the phase state of the proteins which, in turn, depends on temperature and relative humidity. At high temperature and high humidity, the phase state of atmospheric particles is liquid, changing to viscous, semi-solid, or even glassy at low temperature and low humidity (66, 68, 69). In the liquid state, O and NO diffuse faster into proteins and can therefore react faster than with solid or semi-solid proteins (70). For reactions of Bet v 1 with atmospherically relevant concentrations of O/NO in the aqueous phase, the nitration rate was one order of magnitude higher (ND 20% per day) than for solid and semi-solid proteins on filter samples (ND 2% per day), indicating an increased relevance of these processes under humid summer smog conditions (29). For solid or semi-solid proteins, such as protein films on the surface of aerosol particles at low relative humidity, the tyrosine residues near the surface are expected to be nitrated more efficiently since the nitration of tyrosine residues in the bulk of the protein film is kinetically limited by the diffusivity of O and NO (22, 71).
5. Nitration and dityrosine cross-linking increase allergenicity of Bet v 1 and Phl p 5
For TNM-modified Bet v 1, several effects on the immune response have been reported. These include increased proliferation of Bet v 1-specific T cells, greater proteolytic resistance, enhanced antigen presentation, altered cytokine profiles, increased IgE binding, and mediator release (8, 9, 35). Bet v 1 is a small protein with several IgE binding sites clustered in a small area (64, 72–75). Protein dimers and oligomers may play an important role in the reported changes in allergenicity because they display repetitive epitopes. Repetitive epitope presentation is required for the cross-linking of B-cell receptors and activation of B-cells and IgE production, and also facilitates cross-linking of the effector cell-bound IgE, a key event in the initiation of the allergic response (76–80). Aggregation and adsorption to natural or anthropogenic particles can also lead to the display of multiple epitopes (10). In addition to repetitive epitope presentation, nitration and dityrosine cross-linking can lead to epitope modification or shielding, or neoepitope formation (8, 10, 78, 81, 82).
Figure 2 illustrates how the native (monomeric) and modified Bet v 1 variants may increase or decrease cross-linking of mast cell-bound IgE. Binding of monomeric Bet v 1 does not induce cross-linking of IgE binding to the same epitope. Bet v 1 dimers formed by chemical modification can provide two epitope sites that facilitate IgE cross-linking. Binding of monomers may also lead to mast cell degranulation at high allergen concentrations if two monomers bind in close proximity to each other and dimerize on the mast cell surface (76). Bet v 1 monomers could also cross-link IgE if the mast cell carries IgEs, whose binding sites on the monomer do not overlap. This would be more likely if structural changes or nitration lead to the formation of neoepitopes, such as haptenic nitroaromatic groups, which are particularly small and highly immunogenic (83).
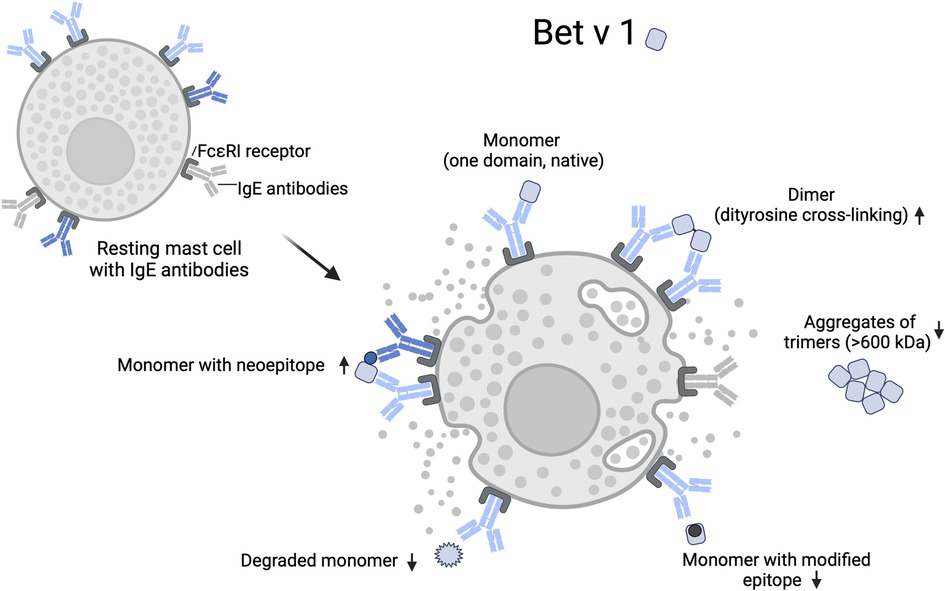
Figure 2. Mast cell degranulation by native and modified Bet v 1. IgE cross-linking on effector cells requires binding to at least two epitopes. Chemical modification can lead to an increase or decrease of accessible epitopes on an allergen through nitration, intra- or intermolecular dityrosine cross-linking, conformational changes, and protein degradation. Arrows indicate a relative increase or decrease of IgE cross-linking by individual allergen variants. Modification of epitope sites and shielding of epitopes by aggregation reduces IgE cross-linking, while the formation of neoepitopes can increase the IgE cross-linking if specific antibodies are bound to the mast cell. Created with BioRender.com.
In sera of allergic patients, Gruijthuijsen et al. (8) found higher levels of IgE specific for the TNM-modified Bet v 1 than for the native Bet v 1. This suggests that some patients may have been exposed and sensitized to different variants of Bet v 1, as their sera contained IgE specific for the neoepitopes of Bet v 1. Chemical modification of epitope sites can theoretically also lead to reduced IgE cross-linking if the IgE are specific for the unmodified epitope or if oligomerization leads to altered epitope presentation or shielding by steric hindrance and/or altered charge interactions (84, 85). For example, reduced basophil activation and mediator release has been reported for trimeric Bet v 1, which tends to form high molecular weight aggregates in solution likely reducing the number of displayed epitopes (86).
Recognition of allergens by airway epithelial receptors such as the Toll-like receptor 4 (TLR4) and other direct allergen-airway epithelial interactions are the first events following allergen inhalation. TLR4 activation has been found for the grass pollen allergen Phl p 5 but not for the birch pollen allergen Bet v 1 (63). TLR4 activation leads to the release of cytokines and other danger signals that initiate the presentation of the allergen to immune cells and the production of allergen-specific IgE, which is crucial for the allergic response phase (63, 87–92). Peroxynitrite modification enhanced TLR4 activation of Phl p 5 by factors of up to 2.1 (63). This may promote sensitization to the grass pollen allergen under conditions of oxidative stress through positive feedback loops (31, 63, 93).
6. Future research
Understanding how environmental risk factors such as air pollution affect the allergenic potential of proteins, either directly or indirectly via oxidative stress, is crucial for the protection of public health (10, 69, 94). The existing literature shows that the reaction of Bet v 1 and Phl p 5 with O/NO or ONOO can result in various mixtures of nitrated and cross-linked variants with altered allergenicity. Potential real-world exposure and variations in the allergenic potential among the nitrated and cross-linked variants remain unclear. Future studies should investigate the extent of allergen modification, both within pollen and as free allergens under environmental conditions. Because allergen content and release from pollen vary, airborne allergens and their variants should be monitored (95–98). In vitro and in vivo studies are needed to assess the allergenic potential of the individual variants and their mixtures in the development and response phases of allergic diseases. In addition, research is needed to investigate the importance of photo-oxidation and the reaction of allergenic proteins with the air pollutant peroxyacetyl nitrate on tyrosine nitration and dityrosine cross-linking (23, 24, 27).
Author contributions
J-FN: Writing – original draft, Writing – review & editing, Visualization; NB: Writing – review & editing, Visualization; ATB: Writing – review & editing; MGW: Writing – review & editing; UP: Writing – review & editing.
Funding
The author(s) declare financial support was received for the research, authorship, and/or publication of this article.
The work was funded by the Max Planck Society.
Conflict of interest
The authors declare that the research was conducted in the absence of any commercial or financial relationships that could be construed as a potential conflict of interest.
Publisher's note
All claims expressed in this article are solely those of the authors and do not necessarily represent those of their affiliated organizations, or those of the publisher, the editors and the reviewers. Any product that may be evaluated in this article, or claim that may be made by its manufacturer, is not guaranteed or endorsed by the publisher.
References
1. Thomson L, Tenopoulou M, Lightfoot R, Tsika E, Parastatidis I, Martinez M, et al., Immunoglobulins against tyrosine-nitrated epitopes in coronary artery disease. Circulation. (2012) 126:2392–401. doi: 10.1161/CIRCULATIONAHA.112.103796
2. Khan F, Siddiqui AA. Prevalence of anti-3-nitrotyrosine antibodies in the joint synovial fluid of patients with rheumatoid arthritis, osteoarthritis, systemic lupus erythematosus. Clin Chim Acta. (2006) 370:100–7. doi: 10.1016/j.cca.2006.01.020
3. Maina MB, Al-Hilaly YK, Serpell LC. Dityrosine cross-linking, its potential roles in Alzheimer’s disease. Front Neurosci. (2023) 17:1132670. doi: 10.3389/fnins.2023.1132670
4. Bandookwala M, Sengupta P. 3-Nitrotyrosine: a versatile oxidative stress biomarker for major neurodegenerative diseases. Int J Neurosci. (2020) 130:1047–62. doi: 10.1080/00207454.2020.1713776
5. Carmen Baez MD, La Paz Scribano MD, Tarán M, Fonseca I, Balceda A, Blencio S, et al., Nitrotyrosine as a biomarker: recovery of histopathological atherogenic lesions in rats treated with ascorbic acid. Trends Med. (2018) 18:1–5. doi: 10.15761/TiM.1000152
6. DiMarco T, Giulivi C. Current analytical methods for the detection of dityrosine, a biomarker of oxidative stress, in biological samples. Mass Spectrom Rev. (2007) 26:108–20. doi: 10.1002/mas.20109
7. Untersmayr E, Diesner SC, Oostingh GJ, Selzle K, Pfaller T, Schultz C, et al., Nitration of the egg-allergen ovalbumin enhances protein allergenicity but reduces the risk for oral sensitization in a murine model of food allergy. PLoS One. (2010) 5:e14210. doi: 10.1371/journal.pone.0014210
8. Gruijthuijsen Y, Grieshuber I, Stöcklinger A, Tischler U, Fehrenbach T, Weller M, et al., Nitration enhances the allergenic potential of proteins. Int Arch Allergy Immunol. (2006) 141:265–75. doi: 10.1159/000095296
9. Karle AC, Oostingh GJ, Mutschlechner S, Ferreira F, Lackner P, Bohle B, et al., Nitration of the pollen allergen Bet v 1.0101 enhances the presentation of Bet v 1-derived peptides by HLA-DR on human dendritic cells. PLoS One. (2012) 7:e31483. doi: 10.1371/journal.pone.0031483
10. Reinmuth-Selzle K, Kampf CJ, Lucas K, Lang-Yona N, Fröhlich-Nowoisky J, Shiraiwa M, et al., Air pollution, climate change effects on allergies in the anthropocene: abundance, interaction, and modification of allergens and adjuvants. Environ Sci Technol. (2017) 51:4119–41. doi: 10.1021/acs.est.6b04908
11. Bowatte G, Lodge CJ, Knibbs LD, Lowe AJ, Erbas B, Dennekamp M, et al., Traffic-related air pollution exposure is associated with allergic sensitization, asthma, and poor lung function in middle age. J Allergy Clin Immunol. (2017) 139:122–129.e1. doi: 10.1016/j.jaci.2016.05.008
12. Guarnieri M, Balmes JR. Outdoor air pollution and asthma. Lancet. (2014) 383:1581–92. doi: 10.1016/S0140-6736(14)60617-6
13. Taylor PE, Flagan RC, Miguel AG, Valenta R, Glovsky MM. Birch pollen rupture and the release of aerosols of respirable allergens. Clin Exp Allergy. (2004) 34:1591–6. doi: 10.1111/j.1365-2222.2004.02078.x
14. Bacsi A, Choudhury B, Dharajiya N, Sur S, Boldogh I. Subpollen particles: carriers of allergenic proteins and oxidases. J Allergy Clin Immunol. (2006) 118:844–50. doi: 10.1016/j.jaci.2006.07.006
15. Bryce M, Drews O, Schenk M, Menzel A, Estrella N, Weichenmeier I, et al., Impact of urbanization on the proteome of birch pollen and its chemotactic activity on human granulocytes. Int Arch Allergy Immunol. (2010) 151:46–55. doi: 10.1159/000232570
16. Motta A, Marliere M, Peltre G, Sterenberg P, Lacroix G. Traffic-related air pollutants induce the release of allergen-containing cytoplasmic granules from grass pollen. Int Arch Allergy Immunol. (2006) 139:294–8. doi: 10.1159/000091600
17. Visez N, Chassard G, Azarkan N, Naas O, Sénéchal H, Sutra JP, et al., Wind-induced mechanical rupture of birch pollen: potential implications for allergen dispersal. J Aerosol Sci. (2015) 89:77–84. doi: 10.1016/j.jaerosci.2015.07.005
18. Ouyang Y, Xu Z, Fan E, Li Y, Zhang L. Effect of nitrogen dioxide and sulfur dioxide on viability and morphology of oak pollen: air pollution increase pollen allergens. Int Forum Allergy Rhinol. (2016) 6:95–100. doi: 10.1002/alr.21632
19. Sedghy F, Varasteh AR, Sankian M, Moghadam M. Interaction between air pollutants and pollen grains: the role on the rising trend in allergy. Rep Biochem Mol Biol. (2018) 6:219–24. 29766006; PMCID: PMC5941124.29766006
20. Maya-Manzano JM, Oteros J, Rojo J, Traidl-Hoffmann C, Schmidt-Weber C, Buters J. Drivers of the release of the allergens Bet v 1, Phl p 5 from birch, grass pollen. Environ Res. (2022) 214:113987. doi: 10.1016/j.envres.2022.113987
21. Franze T, Weller MG, Niessner R, Pöschl U. Protein nitration by polluted air. Environ Sci Technol. (2005) 39:1673–8. doi: 10.1021/es0488737
22. Kampf CJ, Liu F, Reinmuth-Selzle K, Berkemeier T, Meusel H, Shiraiwa M, et al., Protein cross-linking and oligomerization through dityrosine formation upon exposure to ozone. Environ Sci Technol. (2015) 49:10859–66. doi: 10.1021/acs.est.5b02902
23. Pattison DI, Rahmanto AS, Davies MJ. Photo-oxidation of proteins. Photochem Photobiol Sci. (2012) 11:38–53. doi: 10.1039/c1pp05164d
24. Meusel H, Elshorbany Y, Kuhn U, Bartels-Rausch T, Reinmuth-Selzle K, Kampf CJ, et al., Light-induced protein nitration and degradation with HONO emission. Atmos Chem Phys. (2017) 17:11819–33. doi: 10.5194/acp-2017-277
25. Marussi G, Vione D. Secondary formation of aromatic nitroderivatives of environmental concern: photonitration processes triggered by the photolysis of nitrate and nitrite ions in aqueous solution. Molecules. (2021) 26:2550. doi: 10.3390/molecules26092550
26. Mariano A, Bigioni I, Scotto d’Abusco A, Baseggio Conrado A, Maina S, Francioso A, et al., Pheomelanin effect on UVB radiation-induced oxidation/nitration of l-tyrosine. Int J Mol Sci. (2021) 23:267. doi: 10.3390/ijms23010267
27. Lin JK, Chen KJ, Liu GY, Chu YR, Lin-Shiau SY. Nitration and hydoxylation of aromatic amino acid and guanine by the air pollutant peroxyacetyl nitrate. Chem Biol Interact. (2000) 127:219–36. doi: 10.1016/S0009-2797(00)00181-2
28. Franze T, Weller MG, Niessner R, Pöschl U. Enzyme immunoassays for the investigation of protein nitration by air pollutants. Analyst. (2003) 128:824–31. doi: 10.1039/B303132B
29. Reinmuth-Selzle K, Ackaert C, Kampf CJ, Samonig M, Shiraiwa M, Kofler S, et al., Nitration of the birch pollen allergen Bet v 1.0101: efficiency and site-selectivity of liquid and gaseous nitrating agents. J Proteome Res. (2014) 13:1570–7. doi: 10.1021/pr401078h
30. Backes AT, Reinmuth-Selzle K, Leifke AL, Ziegler K, Krevert CS, Tscheuschner G. Reaction products, kinetics, and health effects. Int J Mol Sci. (2021) 22:7616. doi: 10.3390/ijms22147616
31. Ziegler K, Kunert AT, Reinmuth-Selzle K, Leifke AL, Widera D, Weller MG, et al., Chemical modification of pro-inflammatory proteins by peroxynitrite increases activation of TLR4, NF-B: implications for the health effects of air pollution and oxidative stress. Redox Biol. (2020) 37:101581. doi: 10.1016/j.redox.2020.101581
32. Ischiropoulos H. Protein tyrosine nitration—an update. Arch Biochem Biophys. (2009) 484:117–21. doi: 10.1016/j.abb.2008.10.034
33. Beckman JS, Freeman BA. Apparent hydroxyl radical production by peroxynitrite: implications for endothelial injury from nitric oxide and superoxide. Med Sci. (1990) 87:1620–4. doi: 10.1073/pnas.87.4.1620
34. Ischiropoulos H, Zhu L, Chen J, Tsai M, Martin JC, Smith CD, et al., Peroxynitrite-mediated tyrosine nitration catalyzed by superoxide dismutase. Arch Biochem Biophys. (1992) 298:431–7. doi: 10.1016/0003-9861(92)90431-U
35. Ackaert C, Kofler S, Horejs-Hoeck J, Zulehner N, Asam C, Von Grafenstein S, et al., The impact of nitration on the structure and immunogenicity of the major birch pollen allergen Bet v 1.0101. PLoS One. (2014) 9:e104520. doi: 10.1371/journal.pone.0104520
36. Abello N, Kerstjens HAM, Postma DS, Bischoff R. Protein tyrosine nitration: selectivity, physicochemical and biological consequences, denitration, and proteomics methods for the identification of tyrosine-nitrated proteins. J Proteome Res. (2009) 8:3222–38. doi: 10.1021/pr900039c
37. Campolo N, Issoglio F, Estrin D, Bartesaghi S, Radi R. 3-Nitrotyrosine, related derivatives in proteins: precursors, radical intermediates and impact in function. Essays Biochem. (2020) 64:111–33. doi: 10.1042/EBC20190052
38. Houée-Lévin C, Bobrowski K, Horakova L, Karademir B, Schöneich C, Davies MJ, et al., Exploring oxidative modifications of tyrosine: an update on mechanisms of formation, advances in analysis and biological consequences. Free Radic Res. (2015) 49:347–73. doi: 10.3109/10715762.2015.1007968
39. Nuriel T, Hansler A, Gross SS. Protein nitrotryptophan: formation, significance and identification. J Proteomics. (2011) 74:2300–12. doi: 10.1016/j.jprot.2011.05.032
40. Lee SJ, Lee JR, Kim YH, Park YS, Park SI, Park HS, et al., Investigation of tyrosine nitration and nitrosylation of angiotensin II and bovine serum albumin with electrospray ionization mass spectrometry. Rapid Commun Mass Spectrom. (2007) 21:2797–804. doi: 10.1002/rcm.3145
41. Andersson K, Lidholm J. Characteristics and immunobiology of grass pollen allergens. Int Arch Allergy Immunol. (2003) 130:87–107. doi: 10.1159/000069013
42. Hrabina M, Peltre G, Van Ree R, Moingeon P. Grass pollen allergens. Clin Exp Allergy Rev. (2008) 8:7–11. doi: 10.1111/j.1472-9733.2008.00126.x
43. Hauser M, Asam C, Himly M, Palazzo P, Voltolini S, Montanari C, et al., Bet v 1-like pollen allergens of multiple Fagales species can sensitize atopic individuals. Clin Exp Allergy. (2011) 41:1804–14. doi: 10.1111/j.1365-2222.2011.03866.x
44. Dramburg S, Hilger C, Santos AF, De Las Vecillas L, Aalberse RC, Acevedo N, et al., EAACI molecular allergology user’s guide 2.0. Pediatr Allergy Immunol. (2023) 34:e13854. doi: 10.1111/pai.13854
45. Pablos I, Wildner S, Asam C, Wallner M, Gadermaier G. Pollen allergens for molecular diagnosis. Curr Allergy Asthma Rep. (2016) 16:31. doi: 10.1007/s11882-016-0603-z
46. Mothes N, Horak F, Valenta R. Transition from a botanical to a molecular classification in tree pollen allergy: implications for diagnosis and therapy. Int Arch Allergy Immunol. (2004) 135:357–73. doi: 10.1159/000082332
47. Popescu FD. Molecular biomarkers for grass pollen immunotherapy. World J Methodol. (2014) 4:26. doi: 10.5662/wjm.v4.i1.26
48. Walcher W, Franze T, Weller MG, Pöschl U, Huber CG. Liquid- and gas-phase nitration of bovine serum albumin studied by LC-MS and LC-MS/MS using monolithic columns. J Proteome Res. (2003) 2:534–42. doi: 10.1021/pr034034s
49. Franze T, Weller MG, Niessner R, Pöschl U. Comparison of nitrotyrosine antibodies and development of immunoassays for the detection of nitrated proteins. Analyst. (2004) 129:589–96. doi: 10.1039/B402624A
50. Teixeira D, Fernandes R, Prudêncio C, Vieira M. 3-Nitrotyrosine quantification methods: current concepts and future challenges. Biochimie. (2016) 125:1–11. doi: 10.1016/j.biochi.2016.02.011
51. Yang H, Zhang Y, Pöschl U. Quantification of nitrotyrosine in nitrated proteins. Anal Bioanal Chem. (2010) 397:879–86. doi: 10.1007/s00216-010-3557-3
52. Zhang Y, Yang H, Pöschl U. Analysis of nitrated proteins and tryptic peptides by HPLC-chip-MS/MS: site-specific quantification, nitration degree, and reactivity of tyrosine residues. Anal Bioanal Chem. (2011) 399:459–71. doi: 10.1007/s00216-010-4280-9
53. Selzle K, Ackaert C, Kampf CJ, Kunert AT, Duschl A, Oostingh GJ, et al., Determination of nitration degrees for the birch pollen allergen Bet v 1. Anal Bioanal Chem. (2013) 405:8945–9. doi: 10.1007/s00216-013-7324-0
54. Bartesaghi S, Ferrer-Sueta G, Peluffo G, Valez V, Zhang H, Kalyanaraman B, et al., Protein tyrosine nitration in hydrophilic and hydrophobic environments. Amino Acids. (2007) 32:501–15. doi: 10.1007/s00726-006-0425-8
55. Souza JM, Daikhin E, Yudkoff M, Raman C, Ischiropoulos H. Factors determining the selectivity of protein tyrosine nitration. Arch Biochem Biophys. (1999) 371:169–78. doi: 10.1006/abbi.1999.1480
56. Kofler S, Asam C, Eckhard U, Wallner M, Ferreira F, Brandstetter H. Crystallographically mapped ligand binding differs in high and low IgE binding isoforms of birch pollen allergen Bet v 1. J Mol Biol. (2012) 422:109–23. doi: 10.1016/j.jmb.2012.05.016
57. Göbl C, Focke-Tejkl M, Najafi N, Schrank E, Madl T, Kosol S, et al., Flexible IgE epitope-containing domains of Phl p 5 cause high allergenic activity. J Allergy Clin Immunol. (2017) 140:1187–91. doi: 10.1016/j.jaci.2017.05.005
59. Gusenkov S, Ackaert C, Stutz H. Separation and characterization of nitrated variants of the major birch pollen allergen by CZE-ESI-TOF MS: CE and CEC. Electrophoresis. (2013) 34:2695–704. doi: 10.1002/elps.201300151
60. Gusenkov S, Stutz H. Top-down and bottom-up characterization of nitrated birch pollen allergen Bet v 1a with CZE hyphenated to an Orbitrap mass spectrometer. Electrophoresis. (2018) 39:1190–200. doi: 10.1002/elps.201700413
61. Ischiropoulos H. Biological selectivity and functional aspects of protein tyrosine nitration. Biochem Biophys Res Commun. (2003) 305:776–83. doi: 10.1016/S0006-291X(03)00814-3
62. Savojardo C, Manfredi M, Martelli PL, Casadio R. Solvent accessibility of residues undergoing pathogenic variations in humans: from protein structures to protein sequences. Front Mol Biosci. (2021) 7:626363. doi: 10.3389/fmolb.2020.626363
63. Reinmuth-Selzle K, Bellinghausen I, Leifke AL, Backes AT, Bothen N, Ziegler K, et al., Chemical modification by peroxynitrite enhances TLR4 activation of the grass pollen allergen Phl p 5. Front Allergy. (2023) 4:1066392. doi: 10.3389/falgy.2023.1066392
64. Kofler S, Ackaert C, Samonig M, Asam C, Briza P, Horejs-Hoeck J, et al., Stabilization of the dimeric birch pollen allergen Bet v 1 impacts its immunological properties. J Biol Chem. (2014) 289:540–51. doi: 10.1074/jbc.M113.518795
65. Liu F, Lakey PSJ, Berkemeier T, Tong H, Kunert AT, Meusel H, et al., Atmospheric protein chemistry influenced by anthropogenic air pollutants: nitration and oligomerization upon exposure to ozone and nitrogen dioxide. Faraday Discuss. (2017) 200:413–27. doi: 10.1039/C7FD00005G
66. Shiraiwa M, Ammann M, Koop T, Pöschl U. Gas uptake and chemical aging of semisolid organic aerosol particles. Proc Natl Acad Sci. (2011) 108:11003–8. doi: 10.1073/pnas.1103045108
67. Sandhiya L, Kolandaivel P, Senthilkumar K. Oxidation and nitration of tyrosine by ozone and nitrogen dioxide: reaction mechanisms and biological and atmospheric implications. J Phys Chem B. (2014) 118:3479–90. doi: 10.1021/jp4106037
68. Koop T, Bookhold J, Shiraiwa M, Pöschl U. Glass transition and phase state of organic compounds: dependency on molecular properties and implications for secondary organic aerosols in the atmosphere. Phys Chem Chem Phys. (2011) 13:19238. doi: 10.1039/c1cp22617g
69. Shiraiwa M, Ueda K, Pozzer A, Lammel G, Kampf CJ, Fushimi A, et al., Aerosol health effects from molecular to global scales. Environ Sci Technol. (2017) 51:13545–67. doi: 10.1021/acs.est.7b04417
70. Berkemeier T, Steimer SS, Krieger UK, Peter T, Pöschl U, Ammann M, et al., Ozone uptake on glassy, semi-solid and liquid organic matter and the role of reactive oxygen intermediates in atmospheric aerosol chemistry. Phys Chem Chem Phys. (2016) 18:12662–74. doi: 10.1039/C6CP00634E
71. Shiraiwa M, Selzle K, Yang H, Sosedova Y, Ammann M, Pöschl U. Multiphase chemical kinetics of the nitration of aerosolized protein by ozone and nitrogen dioxide. Environ Sci Technol. (2012) 46:6672–80. doi: 10.1021/es300871b
72. Ganglberger E, Grunberger K, Guntaka RV, Sponer B, Breiteneder H, Boltz-Nitulescu G, et al., Allergen mimotopes for 3-dimensional epitope search and induction of antibodies inhibiting human IgE. FASEB J. (2000) 14:2177–84. doi: 10.1096/fj.99-1000com
73. Uehara M, Sato K, Abe Y, Katagiri M. Sequential IgE epitope analysis of a birch pollen allergen (Bet v1) and an apple allergen (Mal d1). Allergol Int. (2001) 50:57–62. doi: 10.1046/j.1440-1592.2001.00201.x
74. Gieras A, Cejka P, Blatt K, Focke-Tejkl M, Linhart B, Flicker S, et al., Mapping of conformational IgE epitopes with peptide-specific monoclonal antibodies reveals simultaneous binding of different IgE antibodies to a surface patch on the major birch pollen allergen, Bet v 1. J Immunol. (2011) 186:5333–44. doi: 10.4049/jimmunol.1000804
75. Hecker J, Diethers A, Schulz D, Sabri A, Plum M, Michel Y, et al., An IgE epitope of Bet v 1 and fagales PR10 proteins as defined by a human monoclonal IgE. Allergy. (2012) 67:1530–7. doi: 10.1111/all.12045
76. Niemi MH, Rytkönen-Nissinen M, Miettinen I, Jänis J, Virtanen T, Rouvinen J. Dimerization of lipocalin allergens. Sci Rep. (2015) 5:13841. doi: 10.1038/srep13841
77. Schöll I, Kalkura N, Shedziankova Y, Bergmann A, Verdino P, Knittelfelder R, et al., Dimerization of the major birch pollen allergen Bet v 1 is important for its in vivo IgE-cross-linking potential in mice. J Immunol. (2005) 175:6645–50. doi: 10.4049/jimmunol.175.10.6645
78. Jensen-Jarolim E, Mechtcheriakova D. Allergen-associated and tumor-associated molecular patterns. In: Penichet ML, Jensen-Jarolim E, editors. Cancer and IgE. Totowa, NJ: Humana Press (2010). p. 231–54. Available from: https://doi.org/10.1007/978-1-60761-451-7˙10
79. Rouvinen J, Jänis J, Laukkanen ML, Jylhä S, Niemi M, Päivinen T, et al., Transient dimers of allergens. PLoS One. (2010) 5:e9037. doi: 10.1371/journal.pone.0009037
80. Pali-Schöll I, Jensen-Jarolim E. The concept of allergen-associated molecular patterns (AAMP). Curr Opin Immunol. (2016) 42:113–8. doi: 10.1016/j.coi.2016.08.004
81. Hasan-Abad AM, Mohammadi M, Mirzaei H, Mehrabi M, Motedayyen H, Arefnezhad R. Impact of oligomerization on the allergenicity of allergens. Clin Mol Allergy. (2022) 20:5. doi: 10.1186/s12948-022-00172-1
82. Ahmad P. Peroxynitrite induced structural changes result in the generation of neo-epitopes on human serum albumin. Int J Biol Macromol. (2013) 59:349–56. doi: 10.1016/j.ijbiomac.2013.04.068
83. Nisonoff A, Shaw AR, Pressman D. The nitro group as a determinant of immunologic specificity. J Am Chem Soc. (1959) 81:1418–23. doi: 10.1021/ja01515a033
84. Tscheppe A, Breiteneder H. Recombinant allergens in structural biology, diagnosis, and immunotherapy. Int Arch Allergy Immunol. (2017) 172:187–202. doi: 10.1159/000464104
85. Najafi N, Hofer G, Gattinger P, Smiljkovic D, Blatt K, Selb R, et al., Fusion proteins consisting of Bet v 1 and Phl p 5 form IgE-reactive aggregates with reduced allergenic activity. Sci Rep. (2019) 9:4006. doi: 10.1038/s41598-019-39798-8
86. Campana R, Vrtala S, Maderegger B, Dall’Antonia Y, Zafred D, Blatt K. A model for hypoallergenic activity revealed for Bet v 1 trimer. Mol Immunol. (2011) 48:431–41. doi: 10.1016/j.molimm.2010.09.016
87. Gandhi VD, Vliagoftis H. Airway epithelium interactions with aeroallergens: role of secreted cytokines and chemokines in innate immunity. Front Immunol. (2015) 6:147. doi: 10.3389/fimmu.2015.00147
88. Lambrecht BN, Hammad H. Allergens and the airway epithelium response: gateway to allergic sensitization. J Allergy Clin Immunol. (2014) 134:499–507. doi: 10.1016/j.jaci.2014.06.036
89. Li DQ, Zhang L, Pflugfelder SC, De Paiva CS, Zhang X, Zhao G, et al., Short ragweed pollen triggers allergic inflammation through Toll-like receptor 4-dependent thymic stromal lymphopoietin/OX40 ligand/OX40 signaling pathways. J Allergy Clin Immunol. (2011) 128:1318–1325.e2. doi: 10.1016/j.jaci.2011.06.041
90. Hosoki K, Aguilera-Aguirre L, Brasier AR, Kurosky A, Boldogh I, Sur S. Facilitation of allergic sensitization and allergic airway inflammation by pollen-induced innate neutrophil recruitment. Am J Respir Cell Mol Biol. (2016) 54:81–90. doi: 10.1165/rcmb.2015-0044OC
91. Li J, Zhang L, Chen X, Chen D, Hua X, Bian F, et al., Pollen/TLR4 innate immunity signaling initiates IL-33/ST2/Th2 pathways in allergic inflammation. Sci Rep. (2016) 6:36150. doi: 10.1038/srep36150
92. Salazar F, Ghaemmaghami AM. Allergen recognition by innate immune cells: critical role of dendritic and epithelial cells. Front Immunol. (2013) 4:356. doi: 10.3389/fimmu.2013.00356
93. Lucas K, Maes M. Role of the toll like receptor (TLR) radical cycle in chronic inflammation: possible treatments targeting the TLR4 pathway. Mol Neurobiol. (2013) 48:190–204. doi: 10.1007/s12035-013-8425-7
94. Pöschl U. Air pollution, oxidative stress, and public health in the anthropocene. In: Al-Delaimy WK, Ramanathan V, Sánchez Sorondo M, editors. Health of people, health of planet and our responsibility. Cham: Springer International Publishing (2020). p. 79–92. Available from: https://doi.org/10.1007/978-3-030-31125-4\_7
95. Buters JT, Kasche A, Weichenmeier I, Schober W, Klaus S, Traidl-Hoffmann C. Evidence for geographical differences between west and south Germany. Int Arch Allergy Immunol. (2008) 145:122–30. doi: 10.1159/000108137
96. Buters JT, Thibaudon M, Smith M, Kennedy R, Rantio-Lehtimäki A, Albertini R, et al., Release of Bet v 1 from birch pollen from 5 European countries. Results from the HIALINE study. Atmos Environ. (2012) 55:496–505. doi: 10.1016/j.atmosenv.2012.01.054
97. Buters J, Prank M, Sofiev M, Pusch G, Albertini R, Annesi-Maesano I, et al., Variation of the group 5 grass pollen allergen content of airborne pollen in relation to geographic location and time in season. J Allergy Clin Immunol. (2015) 136:87–95. doi: 10.1016/j.jaci.2015.01.049
Keywords: allergy, Bet v 1, Phl p 5, nitration, dimers, oligomers, peroxynitrite, air pollution
Citation: Fröhlich-Nowoisky J, Bothen N, Backes AT, Weller MG and Pöschl U (2023) Oligomerization and tyrosine nitration enhance the allergenic potential of the birch and grass pollen allergens Bet v 1 and Phl p 5. Front. Allergy 4:1303943. doi: 10.3389/falgy.2023.1303943
Received: 28 September 2023; Accepted: 20 November 2023;
Published: 5 December 2023.
Edited by:
João Cavaleiro Rufo, University Porto, PortugalReviewed by:
Victoria Rodinkova, National Pirogov Memorial Medical University, Ukraine© 2023 Fröhlich-Nowoisky, Bothen, Backes, Weller and Pöschl. This is an open-access article distributed under the terms of the Creative Commons Attribution License (CC BY). The use, distribution or reproduction in other forums is permitted, provided the original author(s) and the copyright owner(s) are credited and that the original publication in this journal is cited, in accordance with accepted academic practice. No use, distribution or reproduction is permitted which does not comply with these terms.
*Correspondence: Janine Fröhlich-Nowoisky ai5mcm9obGljaEBtcGljLmRl