- 1Department of Infection and Immunity, Luxembourg Institute of Health, Esch-sur-Alzette, Luxembourg
- 2Faculty of Science, Technology and Medicine, University of Luxembourg, Esch-sur-Alzette, Luxembourg
- 3Department of Allergology and Immunology, Centre Hospitalier de Luxembourg-Kanner Klinik, Luxembourg, Luxembourg
- 4Pediatric Allergy Department, Children’s Hospital, University of Nancy, Vandœuvre-lès-Nancy, France
- 5EA3450 DevAH, Faculty of Medecine, University of Lorraine, Vandoeuvre-lès-Nancy, France
Peanut allergy is a growing health concern that can cause mild to severe anaphylaxis as well as reduced quality of life in patients and their families. Oral immunotherapy is an important therapeutic intervention that aims to reshape the immune system toward a higher threshold dose reactivity and sustained unresponsiveness in some patients. From an immunological point of view, young patients, especially those under 3 years old, seem to have the best chance for therapy success. To date, surrogate markers for therapy duration and response are evasive. We provide a comprehensive overview of the current literature state regarding immune signatures evolving over the course of oral immunotherapy as well as baseline immune conditions prior to the initiation of treatment. Although research comparing clinical and immune traits in the first years of life vs. later stages across different age groups is limited, promising insights are available on immunological endotypes among peanut-allergic patients. The available data call for continued research to fill in gaps in knowledge, possibly in an integrated manner, to design novel precision health approaches for advanced therapeutic interventions in peanut allergy.
Introduction
Peanut allergy: a growing health concern
Food allergies can be IgE-mediated, non-IgE-mediated, or mixed types (1–3). IgE-mediated peanut allergy (PA) is one of the most common food allergies in both children and adults (4, 5). PA is also associated with severe reactions and a poor prognosis of outgrowth (10%–27% resolution at 4–12 years of age) (6, 7). PA appears to have the highest prevalence rate of 1%–2% in the Western population (4). In the United States, PAs increased 3.5-fold between the years 1997 and 2008 (8), which might vary in other regions (9). PA negatively affects the quality of life of patients and their caregivers, contributing to a significant financial and healthcare burden for society (4, 8, 10).
With the advent of immunotherapy options, monitoring therapy success by dissecting changes from a clinical and immunological point of view is strongly needed. Here, we review the present evidence and knowledge gaps for stratifying patients based on immune signatures before starting treatment in different age groups using oral immunotherapy (OIT).
Pathophysiology: disturbed epithelial barrier to Th2 immune skewing
PA results from an immune dysregulation to peanut proteins, with cupins (Ara h 1, Ara h 3) and 2S albumins (Ara h 2, Ara h 6) being highly potent allergens (11). Sensitization to such antigens occurs via damaged epithelial barriers (mainly the skin and gut), resulting in a release of epithelium-derived cytokines, interleukin (IL)-25, IL-33, and thymic stromal lymphopoietin (12). These “alarmins” activate dendritic cells (DCs), which captures allergens, inciting the expression of OX40L. OX40l then binds to OX40 on naïve T cells, skewing the immune system toward a T-helper type 2 (Th2) inflammatory response and producing IL-4, IL-9, and IL-13 (13–15). Apart from Th2 cells as a hallmark of allergic inflammation, innate lymphoid cells (ILC2) increase in number and secrete proinflammatory cytokines, such as IL-4, IL-5, and IL-13, which affect Th2 differentiation and activate B cells and effector cells [mast cells (MCs), basophils, and eosinophils] (16, 17). IL-4, secreted by antigen-specific Th2 cells, prompts class switching of B cells, resulting in the production of peanut-specific IgE (sIgE) antibodies. How Th2A cells, a Th2 subpopulation with low proliferation capacity, are involved in IgE production requires further clarification (18). T follicular helper (Tfh) cells producing IL-13 (Tfh 13) appear to further regulate the production of high-affinity sIgE. sIgE binds to the high-affinity IgE receptor (FcεRI) on the surface of effector cells such as MCs and basophils (3, 12, 19).
Upon re-exposure to peanuts, cross-linking of the sIgE bound to effector cells results in cell degranulation. This process induces the release of mediators such as histamine, prostaglandins, tryptase, and platelet-activating factors responsible for clinical manifestations of PA (20). Other immune processes further reinforce allergic inflammation. In a feedback loop mode, CD209+ monocyte-derived DCs increase the frequency of peanut-specific CD4+ T cells to enhance persisting PA responses (21).
Clinical assessment: understanding variable patterns of clinical reactivity
PA may present with mild symptoms to severe anaphylactic reactions. According to the Allergy Vigilance Network and European anaphylaxis registries, peanut-induced anaphylaxis is mainly common in children (15.4% in 2007–2018; 20% in 2002–2020) (22, 23). Intrinsic and extrinsic factors, such as the clinical history of asthma or previous reaction, sensitization to Ara h 2/Ara h 6, and the presence of cofactors (e.g., exercise and acute infections), may increase the risk of anaphylaxis (23, 24). Doses eliciting objective symptoms in 10% of PA patients appear low, ranging from 2.8 to 6.6 mg of peanut protein (25). However, threshold dose reactivity varies greatly among patients (26, 27). This variability might be due to multiple reasons, including the presence of cofactors, dietary composition, and digestion kinetics (28). A subgroup of patients experiences allergic cross-reactions to tree-nuts and botanically related legumes, such as lentil, lupine, and pea (17, 29–31). Typically, diagnosing PA is straightforward by combining the clinical history of the patient with tests involving peanut extract-sIgE and/or skin tests [95% positive predictive value (PPV): sIgE ≥ 35 kUA/L at 1 year of age, ≥2.1 kUA/L at 4 years; skin test wheal ≥8 mm] (32–34). Using single allergens for sIgE quantification further enhances the diagnostic accuracy (e.g., Ara h 2 ≥ 42.2 kUA/L in children between 2 and 7 years with PPV >95%) (35). Although often limited to research settings, the basophil activation test (BAT) can also be used in diagnosing PA; however, oral food challenges (OFCs) continue to be the gold standard (36, 37).
Immune signatures in OIT
Immunotherapy in clinical practice: treatment via the oral route
Managing PA based on strict avoidance is a strategy that leaves patients with a reduced quality of life and an increased risk of severe reactions to accidental exposures (38, 39). While therapeutic treatments with biologicals are under investigation in clinical trials (e.g., monoclonal anti-IgE antibody), OIT is presently the most commonly used treatment (40). Other alternative routes of immunotherapy (e.g., sublingual and epicutaneous) also show promising results in clinical studies (41, 42). Based on the extensive literature available on the efficacy of OIT, the Global Allergy and Asthma European Network (GA2LEN) recommends OIT for selected PA patients >4 years of age (43). The first OIT treatment using peanut powder was approved recently (44). Other OIT trials and real-life clinical practice often depend on commercially available food-grade products (e.g., peanut flour or snacks) (45–47). Overall, clinical outcomes can be highly variable. Through gradually increasing and continued intake of peanut doses, OIT increases the level of threshold dose reactivity as long as patients continue OIT (“desensitization”) (48, 49). Efficacy in achieving desensitization appears high (>60%–90%), as reported for children >3 years, adolescents, and adults (44, 45, 50). A state of remission, also often referred to as sustained unresponsiveness (SU), reported after a few months of OIT discontinuation, is seen only in a small subset of patients (51). Earlier trials on OIT used a higher maintenance dose of up to 4,000 mg of peanut protein daily, while recent studies have demonstrated similar efficacy with a lower maintenance dose of 300 mg (47, 50, 52–54). However, the exact duration and dose of OIT required to achieve long-term remission are still unclear (50–53). SU seems to develop more easily during OIT in children under 4 years. Both the IMPACT and DEVIL studies revealed a higher SU rate in younger age groups, 71% (<2 years) and 78% (<3 years of age), respectively (51, 54). From an immunological point of view, such a young patient group seems to be the ideal candidate to start OIT. However, because of safety concerns, the potential for spontaneous resolution, and logistic constraints, the GA2LEN recommends OIT at this age based on individual profiles (43). Thus, a careful patient-centered evaluation remains a crucial prerequisite to OIT (55–58). The availability of surrogate markers would be an important asset in refining patient selection for OIT in the future (Figure 1).
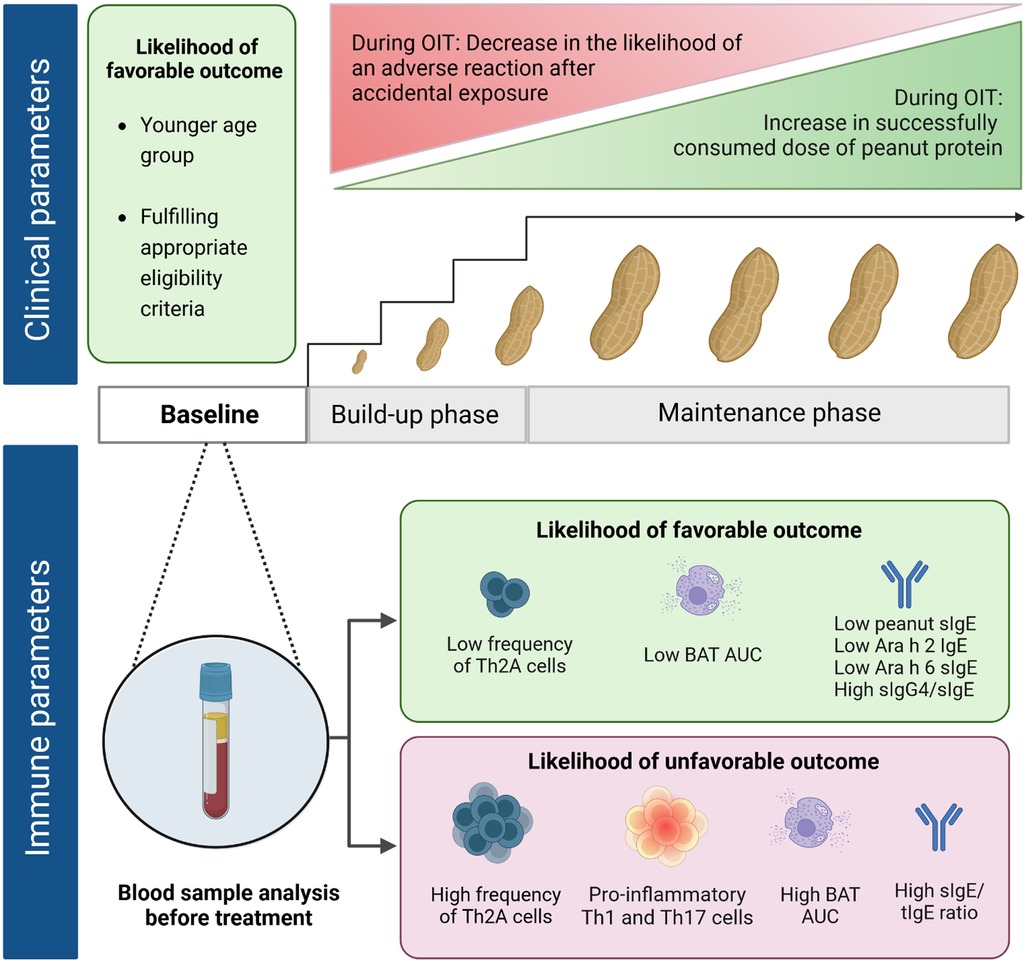
Figure 1. Clinical and immune parameters at baseline correlating with treatment outcomes of peanut oral immunotherapy. Favorable outcome, i.e., desensitization (ability to successfully complete the build-up phase without adverse reactions requiring epinephrine and reach a set maintenance dose OIT or able to successfully pass an exit OFC) and/or sustained unresponsiveness (ability to pass an exit OFC even after OIT discontinuation). Appropriate eligibility criteria, e.g., good compliance and absence of severe/uncontrolled asthma.
We refer to available reference literature for details on the immune mechanisms during OIT (16, 59, 60). Below, we outline key events of immune changes and integrate these with baseline conditions for different age groups (Table 1).
Immune changes occurring through OIT in children and adults
Th2 pathway
Early during OIT, CD63+ or CD203c+ basophil activation capacity decreases (62, 67). This rapid threshold increase in effector cell activation correlates with clinical desensitization and decreased skin test wheal diameter (59). Simultaneously, an initial peanut-specific IgE increase occurs during the build-up phase (51, 55). Expanding peanut-specific Th2 cells and memory B cells most likely contributes to the transient increase in peanut-sIgE (59, 68). During the maintenance phase, sIgE levels slowly decrease. This decline persists with ongoing treatment, as reflected by peanut-sIgE to total IgE (tIgE) ratios (69–71). As immunotherapy progresses, there is a reduction in the proliferative capacity of the pathogenic Th2 cells, along with a selective deletion of these cells and a decrease in the production of Th2 cytokines (IL-3, IL-5, and IL-9) (3). Such a downregulation appears to occur at both low (300 mg) and high (3,000 mg) maintenance doses (72). Indeed, this downregulation also correlates with clinical effects, as reported in the Probiotic Peanut Oral Immunotherapy (PPOIT) study based on reprogramming Th2 gene networks in the CD4+ T cell compartment (73). Expanding IL-10-producing regulatory T cells (Tregs) or B cells (Bregs) may be responsible for coordinating these events (59). IL-10 may also play a role in the induction of allergen-specific sIgG4, another hallmark of OIT (3, 44), as well as in other routes of allergen-specific immunotherapy (74–76).
Non-Th2 pathways
Manohar et al. showed that omalizumab-facilitated multi-OIT was able to not only decrease the frequency of peanut-reactive IL-4+CD4+ T cells but also modulate CD8+ T cells, γδ T cells, and patterns of homing marker expression. The expression of homing receptor G protein-coupled receptor 15 (GPR15) was downregulated on CD4+CD8+ and γδ T cells on treatment with omalizumab, which persisted when multi-OIT was continued. Skin homing markers such as C-C motif chemokine receptor type 4 (CCR4) and cutaneous leucocyte-associated antigen receptor (CLA) were upregulated on CD4+ and CD8+ T effector memory cells, respectively. The authors hypothesized that omalizumab-facilitated OIT could modulate effector T cell trafficking to target tissues (77).
Innate immune response
Zhou et al. demonstrated the role of natural killer (NK; CD56+, CD16+) cells in PA. Following OIT, fewer activated CD69+ NK cells were expressing both Th2 and Th1 cytokines upon peanut stimulation, which reduced significantly at the end of the maintenance phase compared with the baseline (66). In a small group of six PA children, OIT deviated the positive feedback loop between CD209+ monocyte-derived DCs and peanut-specific T cells, indicating the termination of PA responses (21).
Changes over OIT—infant/toddler vs. later in life
The detailed immune changes during OIT reported above were observed in patients older than 4 years. As infants and toddlers have a much better SU rate than older children, corresponding data are urgently needed to link their functionally distinct and uniquely regulated immune response to an improved OIT outcome (78).
Immune characteristics at OIT baseline linked to different age groups
Baseline immune cell profile
Heterogeneity of antigen-specific CD4+ T cells
Repertoires of peanut-reactive effector CD154+ T cells (pTeff) might vary among patients (79). Monian et al. (61) described the baseline characteristics of peanut-reactive CD4+ T cells and their correlation with clinical responses in PA patients aged 7 years and older. Six subtypes of highly clonal peanut-reactive pTeff were identified, namely, three Th2 phenotypes (Tfh2-like: high in costimulatory marker, CXCR5 and PDCD1 gene expression, resembling Tfh13 subsets; Th2reg-like: FOXP3 and TNFRSF9; and Th2A-like: GATA3, IL17RB, and PTGDR2), two Th1 phenotypes (Tfh1-like and Th1-conventional/conv), and one Th17 subtype. Lack of Th2A-like suppression during OIT positively correlated with poor treatment outcomes. In addition, a high degree of baseline inflammation seen by increased expression of inflammatory gene signatures on Th1 and Th17 cells (OX40, OX40L, STAT1, IL-17, and GPR15 genes) was associated with immunotherapy failure, indicating that an existing non-Th2 inflammation could limit successful OIT (61). In line with these findings, Calise et al. (18) confirmed pTeff baseline responses in the IMPACT trial, with PA children less than 4 years of age. Using ex vivo T cell profiling and flow cytometry, they described three main subtypes that were stable in the absence of OIT, namely, conventional Th2 (CRTH2−CXCR5−CXCR3−CCR6−CCR4+), Th2A (CRTH2 + CCR6−), and Th17-like (CRTH2−CCR6+).
Antigen-specific Th2 CD4+ T cell subsets
Based on previous findings, Berin et al. collectively described peanut-reactive CD4+ T cells as “Type 2 cells” encompassing Th2, Th2A, and Tfh13 subsets based on CD154 positivity and co-expression of IL-4 or IL-13 in PA patients from the Consortium for Food Allergy Research (CoFAR6 cohort) trial (80–82).
In PA patients aged 4 years and older, Bajzik et al. reported the role of Th2A pTeff in a subset of 42 patients from the PALISADE trial. Baseline conditions of patients with a low threshold dose reactivity (≤100 mg peanut protein) show a high frequency of pTeff cells predominantly of the Th2A phenotype (CRTH2+ pTeff). These Th2A cells also correlated with initial sIgE (44, 83). Luce et al. supported the selective suppression of those cells and showed similar findings under omalizumab-facilitated multi-OIT in 42 patients aged 5 years and older (here, the Th2A cell phenotype is CD4+CD45RO+CD45RB−CD27−CD49d+CD161+) (84). In PA children less than 4 years of age, the investigators of the IMPACT trial demonstrated that low baseline levels of Th2A pTeff cells were associated with SU after OIT completion (18). PA patients were classified as “Th2A-high immunotype” if Th2A subsets were >20% of total pTeff or “Th2A-low” if Th2A is <20% of pTeff. The Th2A-high immunotype is related to higher sIgE, sIgG4, and pTeff compared with the Th2A-low group. During active OIT, there was a selective decrease in the frequency of Th2A cells in all patients. Importantly, desensitized patients had a Th2A-high immunotype at baseline, while patients who achieved SU had a Th2A-low immunotype (desensitization refers to being able to tolerate 5 g of peanut protein by week 134 without severe symptoms; meanwhile, SU means remaining desensitized despite 26 weeks of OIT discontinuation).
CCR6+ peanut-reactive effector CD154+ T cell subset
In a subset of PA patients with low sIgE values at baseline, Th17-like CCR6+ pTeff appear to be the dominant immunotype, with inverse correlation to Th2A pTeff frequency (18, 81, 83). Bajzik et al. also showed that non-reactors [negative double-blind placebo-controlled food challenge (DBPCFC) at ≤100 mg peanut protein] had higher circulating CCR6+ pTeff at baseline compared with PA individuals with lower threshold dose reactivity. A transcriptomic analysis of these cells revealed the expression of Th1/Th17 and Treg-related genes (IFN-γ, RORγt, IL-17A, IL-17F, IL-22, IL-23R, CCL20, and FOXP3) (83). Reports on whether OIT affects this cell subtype are conflicting. In the PALISADE cohort, OIT did not modulate the frequency of this T cell subset, while in a multi-OIT study with omalizumab, the frequency of Th17 cells (CD4+CCR4+CCR6+) was reduced at week 30 (84).
TNF-α-producing dendritic cells
In PA children aged 5–12 years, OIT “responders” (defined as a successful build-up phase without adverse reactions requiring epinephrine and achieved maintenance dose) exhibited a decreased frequency of TNF-α-producing myeloid DC (mDC; defined as HLADR+CD11c+) (64). On the other hand, “non-responders” (defined as children with ≥1 adverse event requiring epinephrine and not reaching set maintenance doses) showed an increase in TNF-α-producing mDC. Moreover, responders had a transient reduced OX40L expression on mDCs at week 18 of OIT, contrary to non-responders. These findings suggested that a reduced proinflammatory DC phenotype in DCs could potentially contribute to predicting OIT outcomes.
Proportion of activated CD63+/CD203c+ basophils
The upregulation of degranulation markers such as CD63 and CD203c is commonly used to express basophil activation upon ex vivo stimulation utilizing the area under the curve (AUC) from dose–response curves. Basophil activation correlates with clinical outcomes during OFC and OIT (63, 85).
In 120 PA patients aged 5–55 years from the POISED study, participants were stratified as basophil “non/low,” “intermediate,” and “high” responders based on their baseline BAT (62). Briefly, patients were randomized to receive OIT or placebo. After 2 years of OIT, one group continued with a maintenance dose of 300 mg while the other discontinued for another year (50). Non-responders/low responders tolerated a higher cumulative dose at baseline OFC and experienced the most favorable OIT outcomes. Among the patients who discontinued OIT, 83% and 33% of “non-responders” passed the food challenge to 4 g of peanut protein at 13 and 52 weeks post-therapy, respectively (62). In another OIT study that included 30 patients aged 7–13 years, nine of the 22 patients who were desensitized achieved SU 4 weeks after OIT discontinuation (63). The baseline BAT did not distinguish between these two treatment outcome groups. However, a decrease in the BAT to the major peanut allergen Ara h 2 as early as 3 months of OIT correlated with SU.
OIT prediction—infant/toddler vs. later in life
The above-detailed baseline characteristics that might allow the prediction of OIT outcomes have been mainly reported in patients older than 4 years. The first data from the IMPACT trial, targeting infants/toddlers, revealed the involvement of Th2A-related immunotypes. Further studies are needed on this very young age group.
Baseline humoral profile
Considering the difficulty of stratifying patients based on their “immunotype” in a real-life setting, the utility of serological parameters as markers of treatment outcomes remains an area of interest. In 2017, the DEVIL study confirmed the safety and efficacy of peanut OIT in preschool children younger than 3 years of age. Baseline sIgE and Ara h 2 IgE values were negatively correlated with SU (54). To further evaluate the utility of these serological markers in the same cohort, Dreskin et al. (65) used cut-off values of 35 and 25 kUA/L for peanut-sIgE and Ara h 2-sIgE, respectively, to correlate those with the likelihood of achieving SU. To date, only two large randomized control studies have assessed SU to peanut OIT after a prolonged duration of peanut avoidance. Chinthrajah et al. (50) reported that patients over 4 years old who participated in the POISED study with a higher baseline peanut-sIgE/tIgE ratio and Ara h 1 and Ara h 2 IgE titer had a higher risk of treatment failure. Similarly, Jones et al., in a cohort of children from the IMPACT study (≤4 years of age), demonstrated that lower baseline sIgE levels were correlated with the likelihood of “remission” (51).
Table 1 highlights a brief summary of the baseline immune signatures that correlate with peanut OIT outcomes.
Conclusions
Peanut OIT has proven effective in desensitizing the majority of PA patients and achieving SU in a selected group, which is why it appears to be a promising approach in patient care. However, there are also significant challenges to implementing OIT in real life, where limitations apply to patients (e.g., risk and eligibility profiles to consider) and clinics (e.g., lack of expert centers for frequent visits and OFC). Furthermore, the optimal window of opportunity to start OIT, maintenance dose, frequency, and duration still need to be defined. The IMPACT and DEVIL trials, two recent landmark studies, suggested the optimal timing at the age of less than 3 years. Further studies are needed to compare clinical outcomes in different age groups and dissect underlying endotypes to discover novel surrogate biomarkers predictive of OIT response. In addition to the present findings (Figure 1), further insights might arise from new fields, such as CD8+ T cells and the gut microbiome in OIT or blood immune signatures over OFC, contributing to an integrated knowledge base on predictive OIT markers (86–88). This integrated approach will pave the way for precision medicine in food allergy treatment.
Author contributions
AK: Conceptualization, Supervision, Writing – Review & editing. NW: Conceptualization, Writing – Original draft. TB: Conceptualization, Writing – Original draft. FC-M: Writing – Review & editing. AD-C: Writing – Review & editing. IA: Writing – Review & editing. CH: Writing – Review & editing.
Funding
The authors declare that financial support was received for the research, authorship, and/or publication of this article.
The study was supported by the Ministry of Research, Luxembourg, and the PRIDE Program grant PRIDE19/14254520/i2TRON, PRIDE21/16749720/NEXTIMMUNE2 by Fonds National de la Recherche (FNR), Luxembourg, and the Marie Sklodowska-Curie Grant Agreement No. 101072377 by the European Union’s Horizon Europe Research and Innovation Program.
Conflict of interest
The authors declare that the research was conducted in the absence of any commercial or financial relationships that could be construed as a potential conflict of interest.
The authors declared that they were an editorial board member of Frontiers at the time of submission. This had no impact on the peer review process and the final decision.
Publisher's note
All claims expressed in this article are solely those of the authors and do not necessarily represent those of their affiliated organizations, or those of the publisher, the editors and the reviewers. Any product that may be evaluated in this article, or claim that may be made by its manufacturer, is not guaranteed or endorsed by the publisher.
References
1. Tordesillas L, Berin MC, Sampson HA. Immunology of food allergy. Immunity. (2017) 47(1):32–50. doi: 10.1016/j.immuni.2017.07.004
2. Sampath V, Abrams EM, Adlou B, Akdis C, Akdis M, Brough HA, et al. Food allergy across the globe. J Allergy Clin Immunol. (2021) 148(6):1347–64. doi: 10.1016/j.jaci.2021.10.018
3. Yu W, Freeland DMH, Nadeau KC. Food allergy: immune mechanisms, diagnosis and immunotherapy. Nat Rev Immunol. (2016) 16(12):751–65. doi: 10.1038/nri.2016.111
4. Lieberman JA, Gupta RS, Knibb RC, Haselkorn T, Tilles S, Mack DP, et al. The global burden of illness of peanut allergy: a comprehensive literature review. Allergy. (2021) 76(5):1367–84. doi: 10.1111/all.14666
5. Gupta RS, Warren CM, Smith BM, Jiang J, Blumenstock JA, Davis MM, et al. Prevalence and severity of food allergies among US adults. JAMA Netw Open. (2019) 2(1):e185630. doi: 10.1001/jamanetworkopen.2018.5630
6. Grabenhenrich LB, Dölle S, Moneret-Vautrin A, Köhli A, Lange L, Spindler T, et al. Anaphylaxis in children and adolescents: the European Anaphylaxis Registry. J Allergy Clin Immunol. (2016) 137(4):1128–37.e1. doi: 10.1016/j.jaci.2015.11.015
7. Mahr TA, Lieberman JA, Haselkorn T, Damle V, Ali Y, Chidambaram A, et al. Characteristics of peanut allergy diagnosis in a US health care claims database (2011–2017). J Allergy Clin Immunol Pract. (2021) 9(4):1683–94.e5. doi: 10.1016/j.jaip.2020.12.020
8. Lange L, Klimek L, Beyer K, Blümchen K, Novak N, Hamelmann E, et al. White paper on peanut allergy—part 1: epidemiology, burden of disease, health economic aspects. Allergo J Int. (2021) 30(8):261–9. doi: 10.1007/s40629-021-00189-z
9. Spolidoro GCI, Ali MM, Amera YT, Nyassi S, Lisik D, Ioannidou A, et al. Prevalence estimates of eight big food allergies in Europe: updated systematic review and meta-analysis. Allergy. (2023) 78:2361–417. doi: 10.1111/all.15801.
10. Nowak-Wegrzyn A, Hass SL, Donelson SM, Robison D, Cameron A, Etschmaier M, et al. The Peanut Allergy Burden Study: impact on the quality of life of patients and caregivers. World Allergy Organ J. (2021) 14(2):100512. doi: 10.1016/j.waojou.2021.100512
11. Dramburg S, Hilger C, Santos AF, de Las Vecillas L, Aalberse RC, Acevedo N, et al. EAACI molecular allergology user’s guide 2.0. Pediatr Allergy Immunol. (2023) 34(Suppl 28):e13854. doi: 10.1111/pai.13854
12. Sampath V, Nadeau KC. Newly identified T cell subsets in mechanistic studies of food immunotherapy. J Clin Invest. (2019) 129(4):1431–40. doi: 10.1172/JCI124605
13. Oyoshi MK, Larson RP, Ziegler SF, Geha RS. Mechanical injury polarizes skin dendritic cells to elicit a T(H)2 response by inducing cutaneous thymic stromal lymphopoietin expression. J Allergy Clin Immunol. (2010) 126(5):976–84; 84.e1–5. doi: 10.1016/j.jaci.2010.08.041
14. Han H, Thelen TD, Comeau MR, Ziegler SF. Thymic stromal lymphopoietin-mediated epicutaneous inflammation promotes acute diarrhea and anaphylaxis. J Clin Invest. (2014) 124(12):5442–52. doi: 10.1172/JCI77798
15. Lee JB, Chen CY, Liu B, Mugge L, Angkasekwinai P, Facchinetti V, et al. IL-25 and CD4(+) TH2 cells enhance type 2 innate lymphoid cell-derived IL-13 production, which promotes IgE-mediated experimental food allergy. J Allergy Clin Immunol. (2016) 137(4):1216–25.e5. doi: 10.1016/j.jaci.2015.09.019
16. Locke A, Hung L, Upton JEM, O'Mahony L, Hoang J, Eiwegger T. An update on recent developments and highlights in food allergy. Allergy. (2023) 78:2344–360. doi: 10.1111/all.15749
17. Sahiner UM, Layhadi JA, Golebski K, István Komlósi Z, Peng Y, Sekerel B, et al. Innate lymphoid cells: the missing part of a puzzle in food allergy. Allergy. (2021) 76(7):2002–16. doi: 10.1111/all.14776
18. Calise J, DeBerg H, Garabatos N, Khosa S, Bajzik V, Calderon LB, et al. Distinct trajectories distinguish antigen-specific T cells in peanut-allergic individuals undergoing oral immunotherapy. J Allergy Clin Immunol. (2023) 152:155–66. doi: 10.1016/j.jaci.2023.03.020
19. Sampson HA, O'Mahony L, Burks AW, Plaut M, Lack G, Akdis CA. Mechanisms of food allergy. J Allergy Clin Immunol. (2018) 141(1):11–9. doi: 10.1016/j.jaci.2017.11.005
20. Nguyen SMT, Rupprecht CP, Haque A, Pattanaik D, Yusin J, Krishnaswamy G. Mechanisms governing anaphylaxis: inflammatory cells, mediators, endothelial gap junctions and beyond. Int J Mol Sci. (2021) 22(15):7785. doi: 10.3390/ijms22157785
21. Zhou X, Yu W, Lyu SC, Macaubas C, Bunning B, He Z, et al. A positive feedback loop reinforces the allergic immune response in human peanut allergy. J Exp Med. (2021) 218(7):e20201793. doi: 10.1084/jem.20201793
22. Pouessel G, Alonzo S, Divaret-Chauveau A, Dumond P, Bradatan E, Liabeuf V, et al. Fatal and near-fatal anaphylaxis: the Allergy-Vigilance® Network data (2002-2020). Allergy. (2023) 78(6):1628–38. doi: 10.1111/all.15645
23. Maris I, Dölle-Bierke S, Renaudin JM, Lange L, Koehli A, Spindler T, et al. Peanut-induced anaphylaxis in children and adolescents: data from the European Anaphylaxis Registry. Allergy. (2021) 76(5):1517–27. doi: 10.1111/all.14683
24. Klemans RJ, Broekman HC, Knol EF, Bruijnzeel-Koomen CA, Otten HG, Pasmans SG, et al. Ara h 2 is the best predictor for peanut allergy in adults. J Allergy Clin Immunol Pract. (2013) 1(6):632–8.e1. doi: 10.1016/j.jaip.2013.07.014
25. Ballmer-Weber BK, Fernandez-Rivas M, Beyer K, Defernez M, Sperrin M, Mackie AR, et al. How much is too much? Threshold dose distributions for 5 food allergens. J Allergy Clin Immunol. (2015) 135(4):964–71. doi: 10.1016/j.jaci.2014.10.047
26. Purington N, Chinthrajah RS, Long A, Sindher S, Andorf S, O'Laughlin K, et al. Eliciting dose and safety outcomes from a large dataset of standardized multiple food challenges. Front Immunol. (2018) 9:2057. doi: 10.3389/fimmu.2018.02057
27. Santos AF, Du Toit G, O'Rourke C, Becares N, Couto-Francisco N, Radulovic S, et al. Biomarkers of severity and threshold of allergic reactions during oral peanut challenges. J Allergy Clin Immunol. (2020) 146(2):344–55. doi: 10.1016/j.jaci.2020.03.035
28. Luiten D, Biezeveld M, van Doorn O, Riady H, Yang M, Bergsma F, et al. Peanut thresholds in peanut-allergic children are related to dietary composition. Immun Inflamm Dis. (2023) 11(5):e841. doi: 10.1002/iid3.841
29. Juel-Berg N, Larsen LF, Küchen N, Norgil I, Hansen KS, Poulsen LK. Patterns of clinical reactivity in a Danish cohort of tree nut allergic children, adolescents, and young adults. Front Allergy. (2022) 3:824660. doi: 10.3389/falgy.2022.824660
30. Muller T, Luc A, Adam T, Jarlot-Chevaux S, Dumond P, Schweitzer C, et al. Relevance of sensitization to legumes in peanut-allergic children. Pediatr Allergy Immunol. (2022) 33(9):e13846. doi: 10.1111/pai.13846
31. Chentouh MM, Codreanu-Morel F, Boutebba A, Kler S, Revets D, Kuehn A, et al. Allergenic risk assessment of cowpea and its cross-reactivity with pea and peanut. Pediatr Allergy Immunol. (2022) 33(12):e13889. doi: 10.1111/pai.13889
32. Stiefel G, Anagnostou K, Boyle RJ, Brathwaite N, Ewan P, Fox AT, et al. BSACI guideline for the diagnosis and management of peanut and tree nut allergy. Clin Exp Allergy. (2017) 47(6):719–39. doi: 10.1111/cea.12957
33. Foong RX, Santos AF. Biomarkers of diagnosis and resolution of food allergy. Pediatr Allergy Immunol. (2021) 32(2):223–33. doi: 10.1111/pai.13389
34. Peters RL, Allen KJ, Dharmage SC, Koplin JJ, Dang T, Tilbrook KP, et al. Natural history of peanut allergy and predictors of resolution in the first 4 years of life: a population-based assessment. J Allergy Clin Immunol. (2015) 135(5):1257–66.e1–2. doi: 10.1016/j.jaci.2015.01.002
35. Beyer K, Grabenhenrich L, Härtl M, Beder A, Kalb B, Ziegert M, et al. Predictive values of component-specific IgE for the outcome of peanut and hazelnut food challenges in children. Allergy. (2015) 70(1):90–8. doi: 10.1111/all.12530
36. Duan L, Celik A, Hoang JA, Schmidthaler K, So D, Yin X, et al. Basophil activation test shows high accuracy in the diagnosis of peanut and tree nut allergy: the Markers of Nut Allergy Study. Allergy. (2021) 76(6):1800–12. doi: 10.1111/all.14695
37. Muraro A, Werfel T, Hoffmann-Sommergruber K, Roberts G, Beyer K, Bindslev-Jensen C, et al. EAACI food allergy and anaphylaxis guidelines: diagnosis and management of food allergy. Allergy. (2014) 69(8):1008–25. doi: 10.1111/all.12429
38. Polloni L, Muraro A. Anxiety and food allergy: a review of the last two decades. Clin Exp Allergy. (2020) 50(4):420–41. doi: 10.1111/cea.13548
39. Pouessel G, Turner PJ, Worm M, Cardona V, Deschildre A, Beaudouin E, et al. Food-induced fatal anaphylaxis: from epidemiological data to general prevention strategies. Clin Exp Allergy. (2018) 48(12):1584–93. doi: 10.1111/cea.13287
40. Dantzer JA, Wood RA. Anti-immunoglobulin E for food allergy. Ann Allergy Asthma Immunol. (2023) 131(1):11–22. doi: 10.1016/j.anai.2023.03.030
41. Kim EH, Keet CA, Virkud YV, Chin S, Ye P, et al. Open-label study of the efficacy, safety, and durability of peanut sublingual immunotherapy in peanut-allergic children. J Allergy Clin Immunol. (2023) 151(6):1558–65. doi: 10.1016/j.jaci.2023.01.036
42. Greenhawt M, Sindher SB, Wang J, O’Sullivan M, du Toit G, Kim EH, et al. Phase 3 trial of epicutaneous immunotherapy in toddlers with peanut allergy. N Engl J Med. (2023) 388(19):1755–66. doi: 10.1056/NEJMoa2212895
43. Muraro A, de Silva D, Halken S, Worm M, Khaleva E, Arasi S, et al. Managing food allergy: GA(2)LEN guideline 2022. World Allergy Organ J. (2022) 15(9):100687. doi: 10.1016/j.waojou.2022.100687
44. PALISADE Group of Clinical Investigators, Vickery BP, Vereda A, Casale TB, Beyer K, du Toit G, Hourihane JO, et al. AR101 oral immunotherapy for peanut allergy. N Engl J Med. (2018) 379(21):1991–2001. doi: 10.1056/NEJMoa1812856
45. Blumchen K, Trendelenburg V, Ahrens F, Gruebl A, Hamelmann E, Hansen G, et al. Efficacy, safety, and quality of life in a multicenter, randomized, placebo-controlled trial of low-dose peanut oral immunotherapy in children with peanut allergy. J Allergy Clin Immunol Pract. (2019) 7(2):479–91.e10. doi: 10.1016/j.jaip.2018.10.048
46. Vickery BP, Vereda A, Nilsson C, du Toit G, Shreffler WG, Burks AW, et al. Continuous and daily oral immunotherapy for peanut allergy: results from a 2-year open-label follow-on study. J Allergy Clin Immunol Pract. (2021) 9(5):1879–89.e13. doi: 10.1016/j.jaip.2020.12.029
47. Soller L, Abrams EM, Carr S, Kapur S, Rex GA, Leo S, et al. First real-world effectiveness analysis of preschool peanut oral immunotherapy. J Allergy Clin Immunol Pract. (2021) 9(3):1349–56.e1. doi: 10.1016/j.jaip.2020.10.045
48. Bégin P, Chan ES, Kim H, Wagner M, Cellier MS, Favron-Godbout C, et al. CSACI guidelines for the ethical, evidence-based and patient-oriented clinical practice of oral immunotherapy in IgE-mediated food allergy. Allergy Asthma Clin Immunol. (2020) 16:20. doi: 10.1186/s13223-020-0413-7
49. Pajno GB, Fernandez-Rivas M, Arasi S, Roberts G, Akdis CA, Alvaro-Lozano M, et al. EAACI guidelines on allergen immunotherapy: IgE-mediated food allergy. Allergy. (2018) 73(4):799–815. doi: 10.1111/all.13319
50. Chinthrajah RS, Purington N, Andorf S, Long A, O'Laughlin KL, Lyu SC, et al. Sustained outcomes in oral immunotherapy for peanut allergy (POISED study): a large, randomised, double-blind, placebo-controlled, phase 2 study. Lancet. (2019) 394(10207):1437–49. doi: 10.1016/S0140-6736(19)31793-3
51. Jones SM, Kim EH, Nadeau KC, Nowak-Wegrzyn A, Wood RA, Sampson HA, et al. Efficacy and safety of oral immunotherapy in children aged 1-3 years with peanut allergy (the Immune Tolerance Network IMPACT trial): a randomised placebo-controlled study. Lancet. (2022) 399(10322):359–71. doi: 10.1016/S0140-6736(21)02390-4
52. Nachshon L, Goldberg MR, Katz Y, Levy MB, Elizur A. Long-term outcome of peanut oral immunotherapy-real-life experience. Pediatr Allergy Immunol. (2018) 29(5):519–26. doi: 10.1111/pai.12914
53. Varshney P, Jones SM, Scurlock AM, Perry TT, Kemper A, Steele P, et al. A randomized controlled study of peanut oral immunotherapy: clinical desensitization and modulation of the allergic response. J Allergy Clin Immunol. (2011) 127(3):654–60. doi: 10.1016/j.jaci.2010.12.1111
54. Vickery BP, Berglund JP, Burk CM, Fine JP, Kim EH, Kim JI, et al. Early oral immunotherapy in peanut-allergic preschool children is safe and highly effective. J Allergy Clin Immunol. (2017) 139(1):173–81.e8. doi: 10.1016/j.jaci.2016.05.027
55. de Silva D, Rodríguez Del Río P, de Jong NW, Khaleva E, Singh C, Nowak-Wegrzyn A, et al. Allergen immunotherapy and/or biologicals for IgE-mediated food allergy: a systematic review and meta-analysis. Allergy. (2022) 77(6):1852–62. doi: 10.1111/all.15211
56. Chu DK, Wood RA, French S, Fiocchi A, Jordana M, Waserman S, et al. Oral immunotherapy for peanut allergy (PACE): a systematic review and meta-analysis of efficacy and safety. Lancet. (2019) 393(10187):2222–32. doi: 10.1016/S0140-6736(19)30420-9
57. Chinthrajah RS, Cao S, Dunham T, Sampath V, Chandra S, Chen M, et al. Oral immunotherapy for peanut allergy: the pro argument. World Allergy Organ J. (2020) 13(8):100455. doi: 10.1016/j.waojou.2020.100455
58. Tirumalasetty J, Barshow S, Kost L, Morales L, Sharma R, Lazarte C, et al. Peanut allergy: risk factors, immune mechanisms, and best practices for oral immunotherapy success. Expert Rev Clin Immunol. (2023) 19(7):785–95. doi: 10.1080/1744666X.2023.2209318
59. Barshow SM, Kulis MD, Burks AW, Kim EH. Mechanisms of oral immunotherapy. Clin Exp Allergy. (2021) 51(4):527–35. doi: 10.1111/cea.13824
60. Schoos AM, Bullens D, Chawes BL, Costa J, De Vlieger L, DunnGalvin A, et al. Immunological outcomes of allergen-specific immunotherapy in food allergy. Front Immunol. (2020) 11:568598. doi: 10.3389/fimmu.2020.568598
61. Monian B, Tu AA, Ruiter B, Morgan DM, Petrossian PM, Smith NP, et al. Peanut oral immunotherapy differentially suppresses clonally distinct subsets of T helper cells. J Clin Invest. (2022) 132(2):e150634. doi: 10.1172/JCI150634
62. Tsai M, Mukai K, Chinthrajah RS, Nadeau KC, Galli SJ. Sustained successful peanut oral immunotherapy associated with low basophil activation and peanut-specific IgE. J Allergy Clin Immunol. (2020) 145(3):885–96.e6. doi: 10.1016/j.jaci.2019.10.038
63. Patil SU, Steinbrecher J, Calatroni A, Smith N, Ma A, Ruiter B, et al. Early decrease in basophil sensitivity to Ara h 2 precedes sustained unresponsiveness after peanut oral immunotherapy. J Allergy Clin Immunol. (2019) 144(5):1310–9.e4. doi: 10.1016/j.jaci.2019.07.028
64. Anvari S, Watkin LB, Minard CG, Schuster K, Hassan O, Anagnostou A, et al. Reduced pro-inflammatory dendritic cell phenotypes are a potential indicator of successful peanut oral immunotherapy. PLoS One. (2022) 17(5):e0264674. doi: 10.1371/journal.pone.0264674
65. Dreskin SC, Germinaro M, Reinhold D, Chen X, Vickery BP, Kulis M, et al. IgE binding to linear epitopes of Ara h 2 in peanut allergic preschool children undergoing oral immunotherapy. Pediatr Allergy Immunol. (2019) 30(8):817–23. doi: 10.1111/pai.13117
66. Zhou X, Yu W, Dunham DM, Schuetz JP, Blish CA, DeKruyff RH, et al. Cytometric analysis reveals an association between allergen-responsive natural killer cells and human peanut allergy. J Clin Invest. (2022) 132(20):e157962. doi: 10.1172/JCI157962
67. Syed A, Garcia MA, Lyu SC, Bucayu R, Kohli A, Ishida S, et al. Peanut oral immunotherapy results in increased antigen-induced regulatory T-cell function and hypomethylation of forkhead box protein 3 (FOXP3). J Allergy Clin Immunol. (2014) 133(2):500–10. doi: 10.1016/j.jaci.2013.12.1037
68. Patil SU, Ogunniyi AO, Calatroni A, Tadigotla VR, Ruiter B, Ma A, et al. Peanut oral immunotherapy transiently expands circulating Ara h 2-specific B cells with a homologous repertoire in unrelated subjects. J Allergy Clin Immunol. (2015) 136(1):125–34.e12. doi: 10.1016/j.jaci.2015.03.026
69. Fernandez-Rivas M, Vereda A, Vickery BP, Sharma V, Nilsson C, Muraro A, et al. Open-label follow-on study evaluating the efficacy, safety, and quality of life with extended daily oral immunotherapy in children with peanut allergy. Allergy. (2022) 77(3):991–1003. doi: 10.1111/all.15027
70. Ponce M, Diesner SC, Szépfalusi Z, Eiwegger T. Markers of tolerance development to food allergens. Allergy. (2016) 71(10):1393–404. doi: 10.1111/all.12953
71. Wasserman RL, Hague AR, Pence DM, Sugerman RW, Silvers SK, Rolen JG, et al. Real-world experience with peanut oral immunotherapy: lessons learned from 270 patients. J Allergy Clin Immunol Pract. (2019) 7(2):418–26.e4. doi: 10.1016/j.jaip.2018.05.023
72. Kulis M, Yue X, Guo R, Zhang H, Orgel K, Ye P, et al. High- and low-dose oral immunotherapy similarly suppress pro-allergic cytokines and basophil activation in young children. Clin Exp Allergy. (2019) 49(2):180–9. doi: 10.1111/cea.13256
73. Ashley SE, Jones AC, Anderson D, Holt PG, Bosco A, Tang MLK. Remission of peanut allergy is associated with rewiring of allergen-driven T helper 2-related gene networks. Allergy. (2022) 77(10):3015–27. doi: 10.1111/all.15324
74. Fleischer DM, Shreffler WG, Campbell DE, Green TD, Anvari S, Assa'ad A, et al. Long-term, open-label extension study of the efficacy and safety of epicutaneous immunotherapy for peanut allergy in children: PEOPLE 3-year results. J Allergy Clin Immunol. (2020) 146(4):863–74. doi: 10.1016/j.jaci.2020.06.028
75. Scurlock AM, Burks AW, Sicherer SH, Leung DYM, Kim EH, Henning AK, et al. Epicutaneous immunotherapy for treatment of peanut allergy: follow-up from the Consortium for Food Allergy Research. J Allergy Clin Immunol. (2021) 147(3):992–1003.e5. doi: 10.1016/j.jaci.2020.11.027
76. Kim EH, Yang L, Ye P, Guo R, Li Q, Kulis MD, et al. Long-term sublingual immunotherapy for peanut allergy in children: clinical and immunologic evidence of desensitization. J Allergy Clin Immunol. (2019) 144(5):1320–6.e1. doi: 10.1016/j.jaci.2019.07.030
77. Manohar M, Dunham D, Gupta S, Yan Z, Zhang W, Minnicozzi S, et al. Immune changes beyond Th2 pathways during rapid multifood immunotherapy enabled with omalizumab. Allergy. (2021) 76(9):2809–26. doi: 10.1111/all.14833
78. Jalali S, Harpur CM, Piers AT, Auladell M, Perriman L, Li S, et al. A high-dimensional cytometry atlas of peripheral blood over the human life span. Immunol Cell Biol. (2022) 100(10):805–21. doi: 10.1111/imcb.12594
79. Chiang D, Chen X, Jones SM, Wood RA, Sicherer SH, Burks AW, et al. Single-cell profiling of peanut-responsive T cells in patients with peanut allergy reveals heterogeneous effector T(H)2 subsets. J Allergy Clin Immunol. (2018) 141(6):2107–20. doi: 10.1016/j.jaci.2017.11.060
80. Jones SM, Sicherer SH, Burks AW, Leung DY, Lindblad RW, Dawson P, et al. Epicutaneous immunotherapy for the treatment of peanut allergy in children and young adults. J Allergy Clin Immunol. (2017) 139(4):1242–52.e9. doi: 10.1016/j.jaci.2016.08.017
81. Berin MC, Agashe C, Burks AW, Chiang D, Davidson WF, Dawson P, et al. Allergen-specific T cells and clinical features of food allergy: lessons from CoFAR immunotherapy cohorts. J Allergy Clin Immunol. (2022) 149(4):1373–82.e12. doi: 10.1016/j.jaci.2021.09.029
82. Wambre E, Bajzik V, DeLong JH, O'Brien K, Nguyen QA, Speake C, et al. A phenotypically and functionally distinct human T(H)2 cell subpopulation is associated with allergic disorders. Sci Transl Med. (2017) 9(401):eaam9171. doi: 10.1126/scitranslmed.aam9171
83. Bajzik V, DeBerg HA, Garabatos N, Rust BJ, Obrien KK, Nguyen QA, et al. Oral desensitization therapy for peanut allergy induces dynamic changes in peanut-specific immune responses. Allergy. (2022) 77(8):2534–48. doi: 10.1111/all.15276
84. Luce S, Chinthrajah S, Lyu SC, Nadeau KC, Mascarell L. Th2A and Th17 cell frequencies and regulatory markers as follow-up biomarker candidates for successful multifood oral immunotherapy. Allergy. (2020) 75(6):1513–6. doi: 10.1111/all.14180
85. Santos AF, Du Toit G, Douiri A, Radulovic S, Stephens A, Turcanu V, et al. Distinct parameters of the basophil activation test reflect the severity and threshold of allergic reactions to peanut. J Allergy Clin Immunol. (2015) 135(1):179–86. doi: 10.1016/j.jaci.2014.09.001
86. Kaushik A, Dunham D, Han X, Do E, Andorf S, Gupta S, et al. CD8(+) T cell differentiation status correlates with the feasibility of sustained unresponsiveness following oral immunotherapy. Nat Commun. (2022) 13(1):6646. doi: 10.1038/s41467-022-34222-8
87. He Z, Vadali VG, Szabady RL, Zhang W, Norman JM, Roberts B, et al. Increased diversity of gut microbiota during active oral immunotherapy in peanut-allergic adults. Allergy. (2021) 76(3):927–30. doi: 10.1111/all.14540
Keywords: oral immunotherapy, peanut allergy, immune response, immunophenotyping, immunotherapy, food allergy
Citation: Wanniang N, Boehm T-M, Codreanu-Morel F, Divaret-Chauveau A, Assugeni I, Hilger C and Kuehn A (2023) Immune signatures predicting the clinical outcome of peanut oral immunotherapy: where we stand. Front. Allergy 4:1270344. doi: 10.3389/falgy.2023.1270344
Received: 31 July 2023; Accepted: 11 September 2023;
Published: 2 October 2023.
Edited by:
Enza D’Auria, Vittore Buzzi Children’s Hospital, ItalyReviewed by:
Jose Luis Subiza, Inmunotek SL, Spain© 2023 Wanniang, Boehm, Codreanu-Morel, Divaret-Chauveau, Assugeni, Hilger and Kuehn. This is an open-access article distributed under the terms of the Creative Commons Attribution License (CC BY). The use, distribution or reproduction in other forums is permitted, provided the original author(s) and the copyright owner(s) are credited and that the original publication in this journal is cited, in accordance with accepted academic practice. No use, distribution or reproduction is permitted which does not comply with these terms.
*Correspondence: Annette Kuehn annette.kuehn@lih.lu
†These authors have contributed equally to this work and share first authorship