- 1InBio, Charlottesville, VA, United States
- 2InBio, Cardiff, United Kingdom
- 3Department of Experimental Immunology, Amsterdam University Medical Center, Amsterdam, Netherlands
- 4Department of Biosciences and Medical Biology, University of Salzburg, Salzburg, Austria
- 5Department of Pathology, Microbiology and Immunology, Vanderbilt University Medical Center, Nashville, TN, United States
Introduction: Allergic reactions are mediated by human IgE antibodies that bind to and cross-link allergen molecules. The sites on allergens that are recognized by IgE antibodies have been difficult to investigate because of the paucity of IgE antibodies in a human serum. Here, we report the production of unique human IgE monoclonal antibodies to major inhaled allergens and food allergens that can be produced at scale in perpetuity.
Materials and methods: The IgE antibodies were derived from peripheral blood mononuclear cells of symptomatic allergic patients, mostly children aged 3–18 years, using hybridoma fusion technology. Total IgE and allergen-specific IgE was measured by ImmunoCAP. Their specificity was confirmed through ELISA and immunoblotting. Allergenic potency measurements were determined by ImmunoCAP inhibition. Biological activity was determined in vitro by comparing β-hexosaminidase release from a humanized rat basophilic cell line.
Results: Human IgE monoclonal antibodies (n = 33) were derived from 17 allergic patients with symptoms of allergic rhinitis, asthma, atopic dermatitis, food allergy, eosinophilic esophagitis, or red meat allergy. The antibodies were specific for five inhaled allergens, nine food allergens, and alpha-gal and had high levels of IgE (53,450–1,702,500 kU/L) with ratios of specific IgE to total IgE ranging from <0.01 to 1.39. Sigmoidal allergen binding curves were obtained through ELISA, with low limits of detection (<1 kU/L). Allergen specificity was confirmed through immunoblotting. Pairs of IgE monoclonal antibodies to Ara h 6 were identified that cross-linked after allergen stimulation and induced release of significant levels of β-hexosaminidase (35%–80%) from a humanized rat basophilic cell line.
Conclusions: Human IgE monoclonal antibodies are unique antibody molecules with potential applications in allergy diagnosis, allergen standardization, and identification of allergenic epitopes for the development of allergy therapeutics. The IgE antibody probes will enable the unequivocal localization and validation of allergenic epitopes.
Introduction
The discovery of IgE transformed the investigation of allergic diseases and led to the development of in vitro diagnostic tests which have become a routine part of patient care (1–4). Measurements of total IgE and allergen-specific IgE antibodies as markers of allergic responses have had a tremendous impact. They have fueled clinical trials of subcutaneous and oral immunotherapy and led to the introduction of new treatment modalities, such as monoclonal anti-IgE therapy and biologics for treatments of asthma and atopic dermatitis, and early introduction of foods for prevention of food allergy (5, 6). Analysis of IgE antibody responses has played a key role in investigating the mechanisms of anaphylaxis, the structural biology of allergens, and standardization of allergenic products (7). IgE sensitization has been a key indicator for epidemiologic and population-based studies of the etiology of allergic diseases (8–11).
One question that has eluded allergists since the discovery of IgE has been “What do IgE antibodies recognize and what constitutes an ‘allergenic’ epitope?” (12). Indeed, this question has been somewhat of a holy grail among the allergy community and has been difficult to resolve. Natural IgE antibody responses to environmental allergens are polyclonal, and the concentrations of the IgE antibody in the serum are very low, typically <100 or <250 ng/ml. These limitations exclude the use of natural IgE antibodies for direct structural studies of allergen–antibody interactions using x-ray crystallography and/or nuclear magnetic resonance (NMR) spectroscopy (13).
Alternatively, researchers have used indirect approaches to identify conformational IgE epitopes, namely, inhibition of IgE antibody binding to structurally modified allergens, using anti-peptide antibodies to inhibit IgE binding, or using hydrogen/deuterium exchange in NMR to generate variants with reduced IgE binding (13, 14). Linear IgE epitopes on food allergens have been defined using direct peptide-binding assays (15–17). Conformational epitopes may also be important for some food allergens (18).
The recent development of the hybridoma technology to produce allergen-specific human IgE monoclonal antibodies (hIgE mAb) provides a strategic pathway to investigate IgE responses (12, 19). The technology was first used to produce hIgE mAb to Aspergillus fumigatus from patients with allergic bronchopulmonary aspergillosis (19). An advantage of using the hybridoma technology is that milligram (mg) quantities of allergen-specific hIgE mAb can be produced with a natural pairing of heavy and light chains (12). Here, we describe the derivation of unique panels of hIgE mAb from children and adults with allergic rhinitis, asthma, atopic dermatitis, eosinophilic esophagitis, and adverse reactions to foods or red meat allergy. The hIgE mAb recognized the major inhaled allergens and food allergens of diagnostic and therapeutic importance, such as alpha-gal, the carbohydrate associated with red meat allergy (20).
Materials and methods
Generation of allergen-specific hIgE mAb
Peripheral blood mononuclear cells (PBMC) were obtained from 17 allergic subjects, aged 3–63 years, recruited from within the Vanderbilt University Medical Center (VUMC). The subjects diagnoses were based on clinical history, in vitro IgE testing, and/or skin prick tests to inhaled allergens or food allergens (Table 1). The protocol for recruiting and collecting blood samples from allergic subjects was approved by the VUMC Institutional Review Board (IRB 141330 and 142030).
Detailed cell culture methods for producing hIgE mAb from PBMC have recently been published (12, 19, 21, 22). Full technical details of these methods can be found at the following link: https://insight.jci.org/articles/view/123387/sd/1 (accessed 110923) and can be downloaded as a PDF file. Briefly, PBMC were cultured for 7 days with irradiated NIH3T3 cells expressing CD154, B-cell-activating factor, and IL-21 to selectively expand B cells. After screening for IgE through ELISA, IgE-producing B cells were fused with non-secreting myeloma cells and cloned using single-cell flow cytometry. The hIgE mAb generated using this B-cell culture system and hybridoma methodology were not created by artificial class switching. Screening for specificity only occurred after all IgE lines were fused. The hIgE mAb were screened for allergen specificity through ELISA by using microtiter plates coated with ∼1 µg/well of purified natural or recombinant allergens: nDer p 1, nDer p 2, nFel d 1, nCan f 1, rCan f 6, nAra h 1, nAra h 2, nAra h 3, nAra h 6, nBos d 5, nBos d 11, nGal d 2, nGal d 4, rAna o 3, and rJug r 1. The bound hIgE mAb was detected with peroxidase-labeled mouse anti-human IgE. The hIgE mAb were purified by affinity chromatography using immobilized monoclonal anti-IgE antibody omalizumab. The purity of the hIgE mAb was assessed by SDS-PAGE under reducing conditions.
Quantification and specificity of the hIgE mAb
The concentration of total IgE and allergen-specific IgE was determined through ImmunoCAP (Thermo Fisher Scientific, Kalamazoo, MI, USA, capacity) (4, 23). The specificity of the hIgE mAb was assessed through ELISA by using purified natural or recombinant allergens and immunoblotting. ImmunoCAP inhibition experiments were used to assess the ability of the hIgE mAb to inhibit polyclonal IgE antibody binding to allergen extracts.
The IgE concentration of the purified hIgE mAb was initially determined by total IgE ImmunoCAP using highly diluted samples (1/1,000 to >1/20,000). Once the concentration of each purified hIgE mAb stock was determined, samples were diluted to a concentration of ∼50,000 kU/L and re-assayed at 1/100–1/10,000 dilutions. The samples were assayed for the concentration of the allergen-specific IgE antibody by ImmunoCAP, and the ratio of total IgE to allergen-specific IgE was compared.
The specificity of the hIgE mAb was further assessed by ELISA using microtiter plates coated with 100 ng/well of purified allergen. Doubling dilutions of the hIgE mAb were prepared over a three-log range (0.1–100 kU/L) to form dose–response curves. The bound hIgE mAb was detected using peroxidase-labeled goat anti-human IgE (SeraCare, Milford, MA, USA).
For immunoblotting, purified natural or recombinant allergens, cetuximab, and extract samples were run on SDS-PAGE under reducing or non-reducing conditions. Allergens were transferred to PVDF membranes and incubated with a hIgE mAb at 1–4 µg/ml for 1 h. The membranes were washed and incubated for 30 min with alkaline phosphatase-conjugated mouse anti-human IgE Fc (SouthernBiotech, Birmingham, AL, USA) and Strep-Tactin (Bio-Rad, Hercules, CA, USA). They were developed with chemiluminescent substrate prior to chemiluminescence imaging.
ImmunoCAP inhibition
ImmunoCAP inhibition was performed according to the manufacturer's instructions. Pools of the hIgE mAb for Ara h 1 and Ara h 2 and for Der p 1 and Der p 2 were compared with human serum pools from patients allergic to peanuts and to house dust mites, respectively. Pools were diluted to ∼10 kU/L for peanut and house dust mite ImmunoCAP, respectively. The pool of hIgE mAb for peanuts comprised six Ara h 2 hIgE mAb (9H11, 13D9, 11F10, 38B7, 26C3, and 16A8) and a single Ara h 1 hIgE mAb (4G4). The pool of hIgE mAb for house dust mites used a single Der p 1 hIgE mAb (2L11) and three Der p 2 hIgE mAb (2G1, 1B8, and 2F10). Sera from patients allergic to peanuts were provided by Dr. Stephen Dreskin of the University of Colorado, Denver, CO, USA (24). Sera from patients allergic to dust mites were obtained from the CREATE project (25). Human serum samples were diluted to ∼10 kU/L against the allergen extract for use in ImmunoCAP inhibition. Allergen extracts from house dust mites or peanuts were diluted in serial 10-fold dilutions from 1,000 to 0.01 µg/ml and were used to inhibit pools of hIgE mAb or pools of allergic serum to the allergen ImmunoCAP.
Biological activity of the hIgE mAb
The functional activity of the hIgE mAb was assessed by using humanized rat basophilic leukemia (huRBL) cells transfected with human FcɛRI for passive sensitization with hIgE mAb (RBL-2H3 cell line) (26, 27). β-Hexosaminidase release was used as a marker of allergen-induced degranulation. Briefly, RBL-2H3 cells were sensitized with hIgE mAb pairs to Ara h 6 at 5–30 kU/L. After overnight sensitization with hIgE mAb, the cells were stimulated with 50 ng allergen. Untreated cells and cells sensitized with hIgE mAb but not stimulated with allergen were used as controls. For data analysis, the average background signal was subtracted from each value, the percentage of maximal lysis was calculated, and min–max normalized values were plotted. Results were observed from three independent experiments. An ordinary one-way ANOVA with a Tukey's mean multiple comparisons analysis was used to determine significant differences between the mediator release produced by all the combinations.
Results
Subject demographics and clinical information
The hIgE mAb to inhaled and food allergens were derived from 33 fusions from 17 selected donors who presented to the VUMC allergy clinic with clinical histories of allergic rhinitis, asthma, atopic dermatitis, eosinophilic esophagitis, or adverse reactions to foods. The donor population included 13 children (aged 3–18 years) and four adults (aged 27–63 years), with a preponderance of male (n = 10) to female (n = 7) donors. The research subjects had total IgE levels of 351 to >5,000 kU/L, together with high levels of allergen-specific IgE (65 to >100 kU/L) and/or strongly positive skin prick tests to allergen extracts (Table 1). More than 50% of the hIgE mAb were derived from three subjects with multiple sensitivities (P1–P3, Table 1), including a 3-year-old child allergic to food from whom the hIgE mAb to cashew, milk, and egg allergens were derived. The IgE B-cell frequency, as measured by the number of IgE-positive B-cell culture wells per 10 million PBMC used in culture, ranged from 2 to 12 per 107 PBMC, which is comparable to that reported in recent studies of IgE primary B-cell cultures from Aspergillus or parasitic worm-infected donors (19, 22).
Quantitative comparison of hIgE mAb reactivity
This paper describes the IgE-binding activity and biological activity of 33 hIgE mAb directed against five inhaled allergens (Der p 1, Der p 2, Fel d 1, Can f 1, and Can f 6) and against nine food allergens (Ara h 1, Ara h 2, Ara h 3, Ara h 6, Bos d 8, Gal d 2, Gal d 4, Ana o 3, and Jug r 1) and alpha-gal. Multiple hIgE mAb were generated against several allergens such as Der p 1, Der p 2, Fel d 1, Ara h 1, Ara h 2, Ara h 3, and Ara h 6 and alpha-gal. Following monoclonal anti-IgE affinity purification, yields of hIgE mAb were typically 2–30 mg, and purity was >90% under reducing conditions on SDS-PAGE (Figure 1). Most of the hIgE mAb (n = 29) showed homogeneous heavy- and light-chain bands of 75 kD and 25–30 kD, respectively. Two hIgE mAb, to alpha-gal (16D9) or Ara h 1 (3B10), showed truncated heavy chains at ∼50 kD either alone or in addition to the 75 kD heavy chain. The IgE mAb 16D9 bound to alpha-gal and to FcɛRI by ELISA. Despite the presence of only the truncated form in 3B10, this antibody did specifically recognize Ara h 1. However, it did not bind to FcɛRI (data not shown), which indicated that this band with lower MW than expected for a heavy chain possibly results from a truncated form that lost its capacity to bind the IgE high-affinity receptor, while keeping its capacity to bind the allergen.
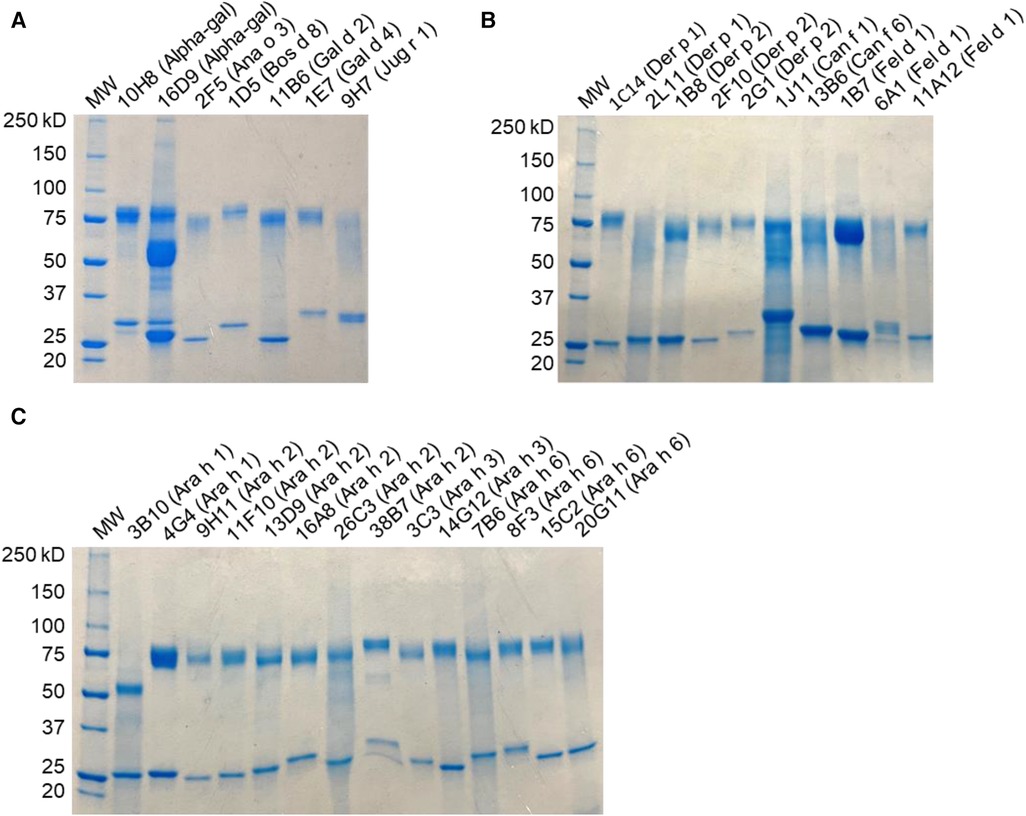
Figure 1. SDS-PAGE analysis of hIgE mAb. Samples were run under reducing conditions and stained with Coomassie blue, indicating heavy- and light-chain bands. (A) Food allergen panel. (B) Inhaled allergen panel. (C) Peanut allergen panel.
The total IgE measurements of purified hIgE mAb ranged from 53,450 to 1,702,500 kU/L (Table 2). To standardize IgE concentrations, the hIgE mAb were adjusted to a concentration of ∼50,000 kU/L and assayed for specific IgE using allergen extract or purified allergen ImmunoCAP. The ratio of total IgE to specific IgE varied by allergen and by individual hIgE mAb and ranged from <0.01 to 1.39 (Table 2). Low ratios (<0.2) were obtained for hIgE mAb to alpha-gal and to Ara h 6 (20M10), Can f 6, and Der p 1. Overall, a good agreement between the allergen-specific IgE values obtained with allergen extracts and purified allergens was reported.
Some hIgE mAb to peanut reacted strongly with allergen extract but displayed low IgE binding to the purified allergen, suggesting that the recombinant allergen(s) coupled to the cellulose solid phase of the ImmunoCAP may lack certain IgE epitope(s). This was investigated by comparing hIgE mAb binding to purified natural and recombinant allergens using the streptavidin CAP (28, 29). Purified nAra h 2, nAra h 3, and nAra h 6 were coupled to streptavidin CAPs and used to compare binding of four hIgE mAb. The hIgE mAb to Ara h 3 (14G12) and Ara h 6 (20M11) bound strongly to peanut extract but weakly (<10% reactivity) to the recombinant allergen ImmunoCAP. In contrast, the binding of these hIgE mAb to natural allergen on streptavidin CAP was 18–42-fold higher than the binding of hIgE mAb to the recombinant allergens and even exceeded its binding to peanut ImmunoCAP (Table 3).
Specificity of the hIgE mAb
The hIgE mAb showed a strong binding to purified allergens in ELISA with dose–response curves from <1 to 100 kU/L (Figure 2). In most cases, the dose–response curves were sigmoidal with limits of detection of <1 kU/L. The specificity of the selected hIgE mAb to alpha-gal, food allergens, and inhaled allergens was further evaluated by immunoblotting using purified allergens and allergen extracts under reducing and non-reducing conditions (Figure 3). The two hIgE mAb to alpha-gal (16D9 and 10H8) showed strong reactivity and similar dose–response curves in ELISA using a purified bovine thyroglobulin. They also showed a strong binding to bovine thyroglobulin and to cetuximab by immunoblotting, and no reactivity to a chicken meat extract control (Figure 3A). Anti-Ara h 1 hIgE mAb 3B10 reacted strongly with the 60 kD sub-unit of Ara h 1, and the hIgE mAb to Ara h 2 (26C3) bound to both 17 kD bands in the Ara h 2 doublet (Figure 3B,C). The hIgE mAb to Ana o 3 (2F5) and to Jug r 1 (9H7) bound strongly to each respective allergen under non-reducing and reducing conditions (Figure 3D,E). For inhaled allergens, the hIgE mAb to Der p 1 (2L11), Der p 2 (2F10), and Can f 1 (1J11) bound predominantly to single bands at their respective molecular weights (Figure 2F–H). The hIgE mAb to Fel d 1 (1B7) bound to the 14 kD Fel d 1 homodimer under non-reducing conditions and to the ∼6 kD homodimer fragment under reducing conditions (Figure 3I).
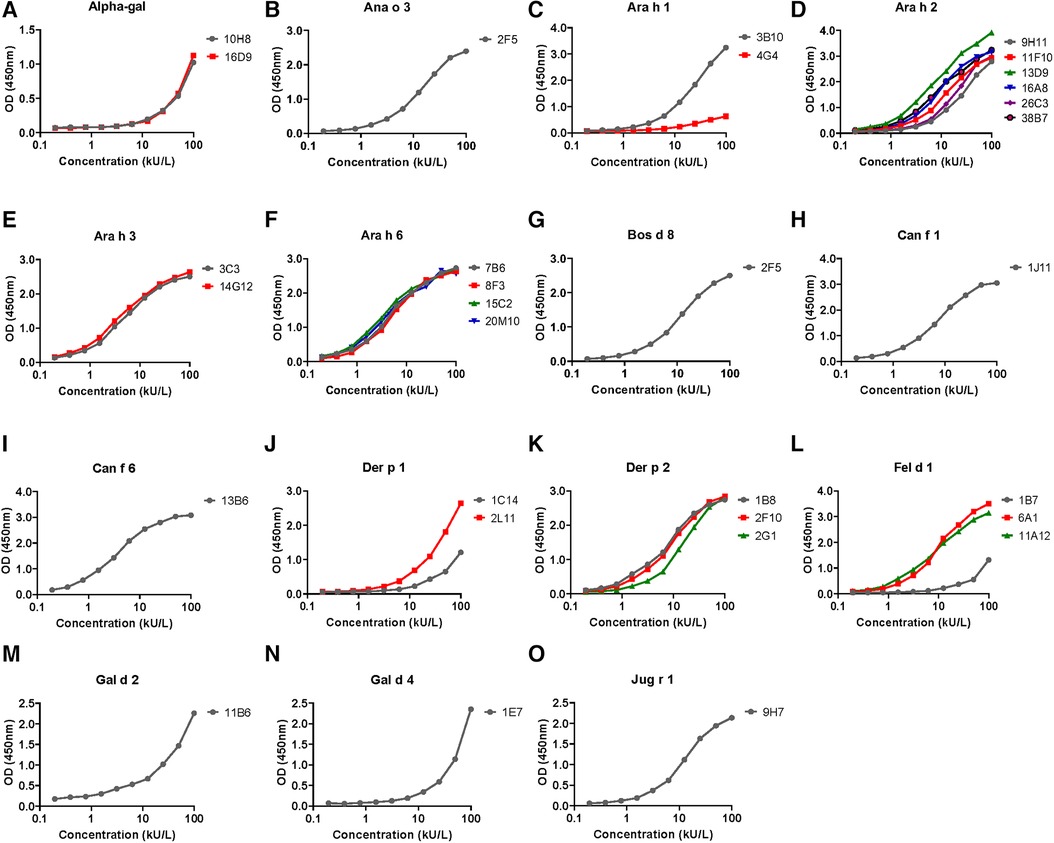
Figure 2. ELISA dose–response curves for hIgE mAb titered against purified natural or recombinant target allergen adsorbed onto microtiter plates (100 ng per well). Serial twofold dilutions of hIgE mAb were compared, starting at a concentration of 100 kU/L. The dose response curves for each of the 15 allergens tested are labeled (A) through (O), with insets denoting the allergen specificity e.g. A Alpha-gal, B Ana o 3, etc. The side bars indicate the individual hIgE mAb used for the control curves and are color coded to distinguish multiple antibodies. The ELISA curves are representative of two to three experiments performed for each allergen.
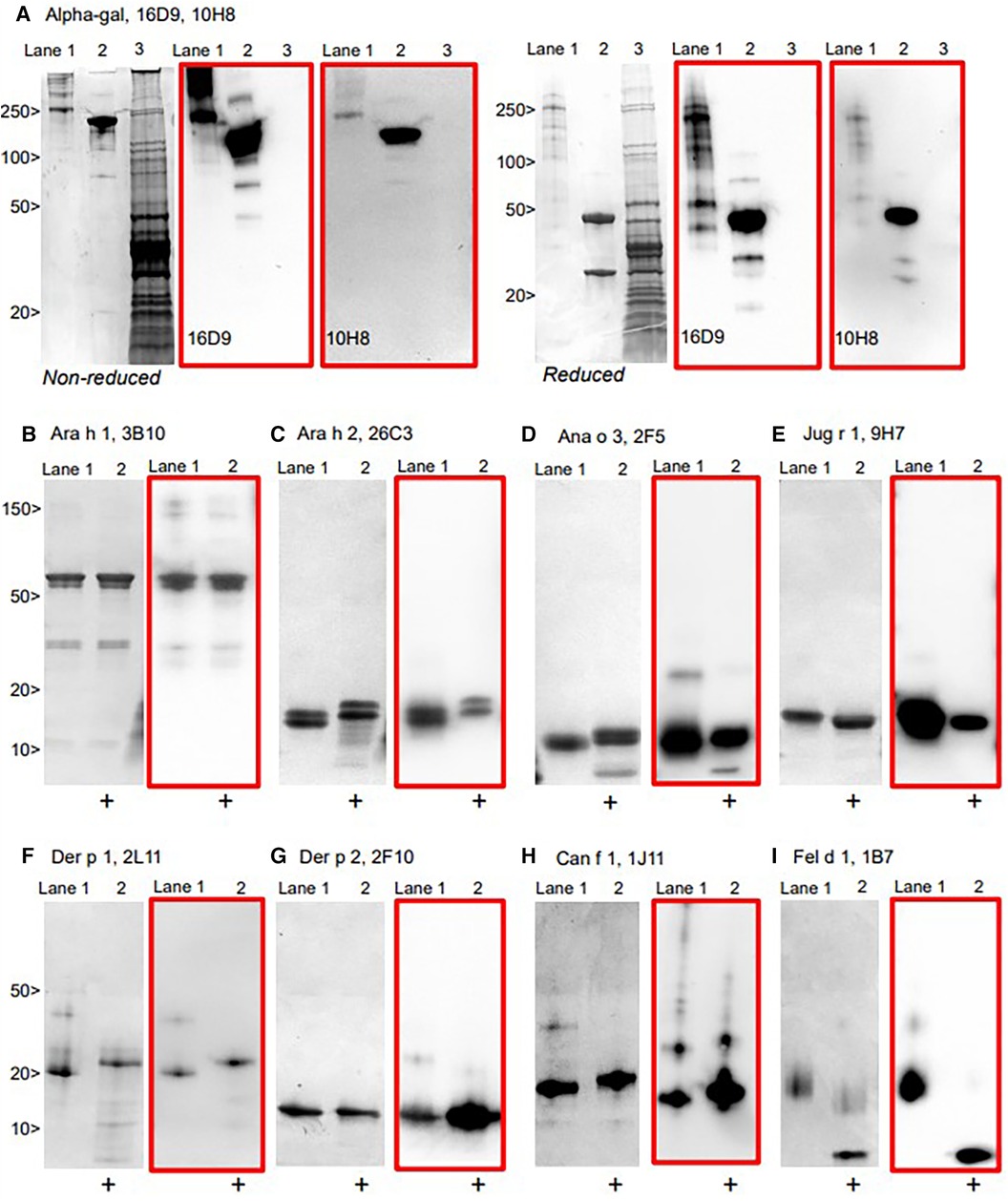
Figure 3. Immunoblotting of representative hIgE mAb using allergens run under non-reducing and reducing conditions on SDS-PAGE, with the matched immunoblot in red. (A) hIgE mAb to alpha-gal demonstrating reactivity to bovine thyroglobulin (Lane 1) and cetuximab (Lane 2), but not to chicken meat extract (Lane 3). (B–I) purified allergens non-reduced (Lane 1) and reduced [+] (Lane 2), with immunoblot (in red).
ImmunoCAP inhibition
To evaluate whether the pools of hIgE mAb could replace variable patient serum pools for potency assessment of immunotherapy extracts in standardization protocols, both were compared by ImmunoCAP inhibition targeting house dust mites and peanuts. Extracts of both allergen sources gave very similar inhibition curves using the pools of monoclonal antibodies and the pools of the human serum (Figure 4).
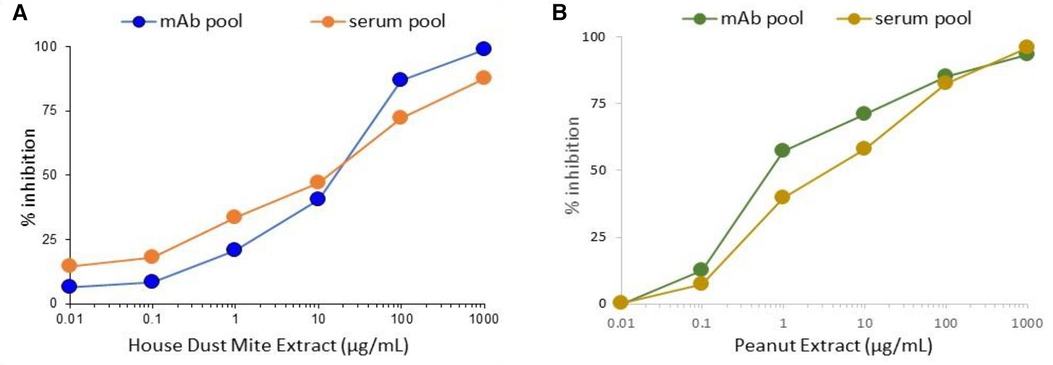
Figure 4. Comparison of inhibition of hIgE mAb or human serum pool IgE to house dust mite (A) or peanut extract (B).
Basophil mediator release by the hIgE mAb
The hIgE mAb to Der p 2, Fel d 1, or Ara h 2 were screened in pairs for their biological activity using a basophil mediator release assay by passively sensitizing huRBL cells. The aim was to determine (i) whether the hIgE mAb were directed against different epitopes and (ii) whether they could cross-link the high-affinity human IgE Fcε receptor that was transfected into the RBL cell line upon allergen stimulation to induce degranulation. The mediator release data for hIgE mAb pairs to Der p 2, Fel d 1, and Ara h 2 was recently published (27). For each of the selected allergens, antibody pairs induced 40%–80% β-hexosaminidase release, providing a strong evidence for multiple non-overlapping IgE epitopes.
In the present studies, five hIgE mAb to Ara h 6 (8F3, 20M10, 21C10, 20G11, and 7B6) were analyzed for basophil mediator release. Dose–response curves showed 30%–90% β-hexosaminidase release using several combinations of the hIgE mAb (Figure 5). Two of the hIgE mAb pairs (8F3 + 7B6 and 7B6 + 20G11) showed 50%–90% β-hexosaminidase release with Ara h 6 and with peanut extract, but no release with Ara h 2 was determined, indicating that they were specific for Ara h 6. The data strongly suggests that the hIgE mAb define multiple non-overlapping epitopes on Ara h 6.
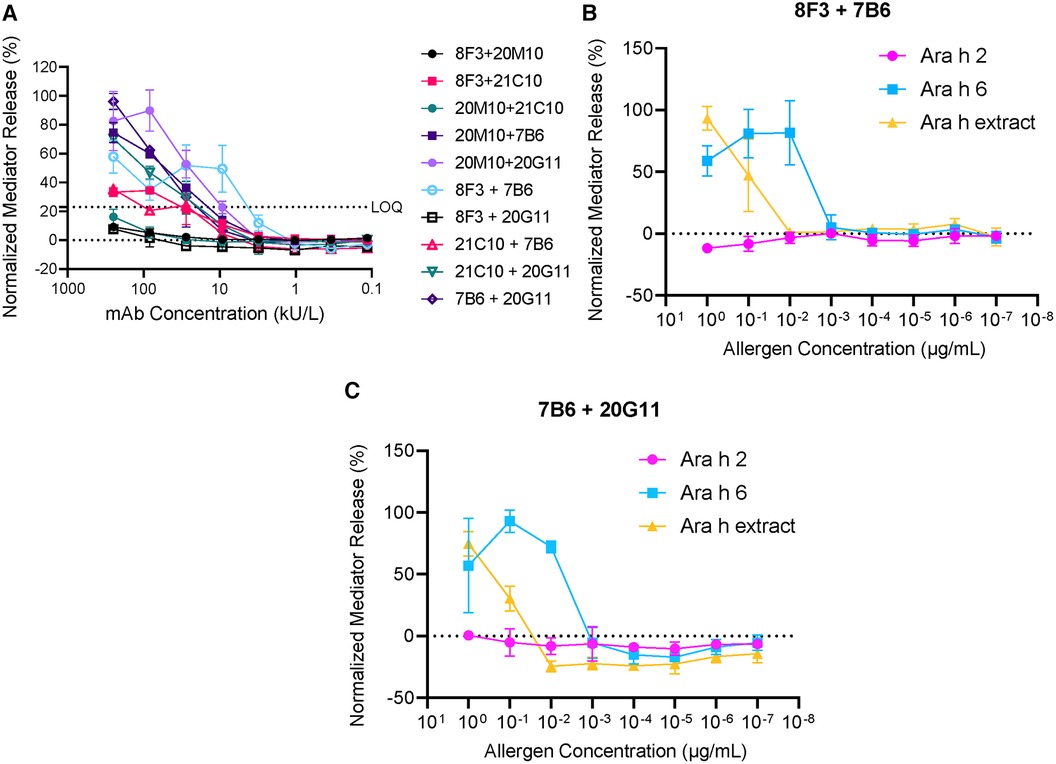
Figure 5. Mediator release induced by hIgE mAb to Ara h 6. (A) Dose–response curves using 10 different combinations of hIgE mAb. (B) Comparison of mediator release using Ara h 6, Ara h 2, and Ara h extract (8F3 and 7B6). (C) Comparison of mediator release using Ara h 6, Ara h 2, and Ara h extract (7B6 and 20G11). LOQ, limit of quantification.
Discussion
Previous approaches to study human IgE antibodies have involved the use of chimeric antibodies with allergen-specific murine Fab regions fused to human IgE Fc regions (30, 31). Phage display techniques were used to generate Fab resulting from random combinations of human IgE heavy- and light-chain variable domains (32). These approaches produced IgE constructs that were useful for in vitro studies, but the synthetic antibodies (not naturally occurring) either used murine variable regions or did not necessarily have the natural pairing of human heavy and light chains that formed the IgE response in allergic people (33). The novelty of the hIgE mAb is that they are derived from symptomatic allergic patients and represent part of the human IgE repertoire of these patients (12). The clones reported are very rare, as indicated in Table 1 by the low IgE B-cell frequency (average of six IgE-positive cells per 10 million PBMC). Plasmablasts are not amenable to this approach because they do not grow in culture. Memory B cells have been reported to co-exist in blood with plasmablasts, with both types of cells encoding IgE (34). They appear to be sufficient to generate the large panel of IgE mAb reported here, which are representative antibodies from the human repertoire of allergic individuals. The ability to derive a broad range of full length naturally occurring hIgE mAb to a diverse array of allergens enables systematic dissection of allergen-specific IgE responses.
The hIgE mAb donors had symptoms of asthma, atopic dermatitis, or adverse reactions to foods. The antibody specificities from these patients broadly correlated with their allergic symptoms. A surprising outcome, due to the low frequency of IgE encoding B cells which results in low absolute numbers in small blood draws, was that hIgE mAb were obtained from young children, aged 3–10 years, who comprised 53% of the patients. Unsurprisingly, most of the hIgE mAb to food allergens were mostly seen in children. For young children, hIgE mAb were derived from only 1 to 10 ml of peripheral blood. The success of this approach was attributed to the expansion of IgE encoding B cells using irradiated NIH3T3 fibroblasts expressing CD40 ligand and secreting B-cell-activating factor and IL-21 (12). The increase of B cells in culture allows for greater rates of successful myeloma fusion and hybridoma generation. This is essential given the small quantity of blood that can be obtained and the extreme rarity of IgE encoding B cells.
The total IgE data provided convincing evidence that the hIgE mAb could be produced at concentrations that were orders of magnitude higher than those found in allergic patients. Clinically, CAP class 6 or ∼100 kU/L is considered a very high level of allergen-specific IgE (4, 23). The hIgE mAb levels were three to four logs higher and were comparable with those seen in patients with hyper-IgE syndromes or IgE myelomas. To compare the relative IgE activity of the different antibodies, the hIgE mAb were standardized at a total IgE level of ∼50,000 kU/L and then compared for specific IgE reactivity with purified allergen or with allergen extract. The hIgE mAb were obtained from highly productive hybridomas and showed remarkable levels of allergen-specific IgE, generally 2,000 to >50,000 kU/L: values that are much higher than those found in sera from allergic patients. In most cases, a good agreement between the allergen-specific and extract specific IgE was found. Some discrepancies between the levels of total IgE and allergen-specific IgE were noted. For ∼50% of hIgE mAb, the specific IgE levels ranged between 50% and 136% of the total IgE values. However, seven of the hIgE mAb had specific IgE levels that were <20% of the total IgE, including the hIgE mAb to alpha-gal, Der p 1, and Can f 6. Even in these cases, the actual levels of allergen-specific IgE significantly exceeded those found in sera from allergic patients. The reasons for differences between total and specific IgE levels are unclear. In some cases, the allergen-specific IgE values exceed those of the total IgE. Taken together, these results could reflect differences in IgE binding to the monoclonal anti-IgE antibodies used in the total IgE ImmunoCAP and the recombinant allergens or other proteins that are coupled to the solid phase for specific IgE measurements. To some extent, this is supported by the streptavidin CAP data for the hIgE mAb to peanut allergens, which showed lower binding to recombinant allergens but strong binding to natural allergens on the streptavidin CAP.
For the first time, the availability of hIgE mAb to food and inhaled allergens provides molecular probes to determine the structures of allergenic epitopes recognized by allergic patients. This transformative capability has recently been applied to determine the structure of the conformational epitope recognized by the 2F10 hIgE mAb on Der p 2 by x-ray crystallography. The 2F10 hIgE mAb, in combination with other hIgE mAb to Der p 2, passively sensitized human FcεRIα-transgenic mice and caused anaphylaxis: a rigorous demonstration of biological relevance (35). In the present study, β-hexosaminidase release from passively sensitized huRBL cells was used as an alternative approach to assess its biological activity. We recently published detailed comparisons of hIgE mAb potency in the huRBL system (27). In those studies, the hIgE mAb pairs to Der p 2, Fel d 1, and Ara h 2 produced high levels of mediator release and were directed against non-overlapping epitopes on each allergen. The current study showed high levels of β-hexosaminidase release using hIgE mAb pairs to Ara h 6. Similar huRBL data for the hIgE mAb to Ara h 2 was recently reported, and together these data support the concept that the hIgE mAb to food allergens provide screening tools for food allergy therapeutics (36).
The only epitope that was defined prior to this study was alpha-gal (20). Previously, the only available monoclonal antibodies to alpha-gal were a commercial murine IgM antibody (M86) and a mouse IgG1 antibody (27H8) (36, 37). The two hIgE mAb recognize alpha-gal on both bovine thyroglobulin and cetuximab. The hIgE mAb were from a 63-year-old patient who had severe reactions on several occasions after consumption of red meat and had a high total IgE and specific IgE to alpha-gal (P4, Table 1). Interestingly, immediately prior to these systemic allergic reactions to red meat, the patient was reported to have severe skin rash resulting from innumerable lower extremity bites of larval stage ticks (seed ticks). The hIgE mAb to alpha-gal will enable better diagnostic tests for alpha-gal to be developed. Investigations of the immunopathogenesis of red meat allergy, including the presence of alpha-gal in the tick saliva and the role of alpha-gal in coronary artery disease, will also be facilitated (38–42).
Limitations of this study are that hIgE mAb from a given individual may not represent the complete repertoire of IgE responses among the allergic population or that the fusion process followed by the antibody screening may select for certain epitope(s). Patients were randomly enrolled in these studies based on history, symptoms, and positive skin test to given allergens, rather than being targeted cohorts of a given age range or allergic sensitivity. In the future, pediatric and adult populations could be targeted for certain allergens, e.g., foods (peanut, milk, egg) or inhaled allergens (mite, cat, dog, pollens), to obtain a broader spectrum of the hIgE mAb. This would enable linear or conformational epitopes on food allergens to be investigated (12).
The hIgE mAb have several applications in the investigation of allergic diseases: first, as potential replacements for human sera and, second, as reference material(s) in allergy diagnostics. Access to the panels of the sera from allergic patients presents a significant barrier to innovation for diagnostic test developers (43). The hIgE mAb can serve as probes to identify specific allergens, as positive controls for current IgE tests and for the next generation of microarrays, nanotechnology, and point-of-care tests that are in the pipeline. The hIgE mAb have similar applications in cellular tests, such as the basophil activation test (BAT). The need for standardized reagents and procedures to validate the BAT and improve quality control has recently been emphasized (44).
Since the discovery of IgE, standardization of serologic measurements of IgE has relied on the World Health Organization’s human serum references for measuring the IgE levels for clinical diagnosis. The IgE tests produced by different manufacturers show variability and are not interchangeable (4). The hIgE mAb could ultimately be used to produce purified IgE reference standards in lieu of serum-based references as a calibrator of IgE measurements. This would be analogous to the National Institutes of Standards and Technology NISTmAb Reference Materials for validation of the methods used for the manufacture of therapeutic antibodies (45).
The unique value of the hIgE mAb to define allergenic epitopes recognized by allergic patients, to answer the question about what constitutes an allergenic epitope, will have applications in allergy therapeutics. A recent clinical trial demonstrated the efficacy of allergen-specific monoclonal antibodies to Fel d 1 to treat mild asthma (46). Mutations in allergen sequences can reduce anaphylaxis in mouse models, as we have shown for Der p 2 (35). The hIgE mAb will also have applications in mechanistic studies of IgE cross-linking and basophil and mast cell activation, all of which could lead to development of novel therapeutics. Improvements in allergy diagnostics and therapeutics using the hIgE mAb have the potential to transform the allergy field for the benefit of allergic patients.
Data availability statement
The original contributions presented in the study are included in the article/Supplementary Material, further inquiries can be directed to the corresponding author.
Ethics statement
The studies involving humans were approved by Vanderbilt University Medical Center Institutional Review Board (IRB 141330 and 142030). The studies were conducted in accordance with the local legislation and institutional requirements. Written informed consent for participation in this study was provided by the participants’ legal guardians/next of kin.
Author contributions
MC: Conceptualization, Funding acquisition, Resources, Supervision, Validation, Writing – Original draft, Writing – Review and editing. BS: Investigation, Methodology, Project administration, Resources, Writing – Original draft. KR: Methodology, Project administration, Investigation, Resources, Visualization, Writing – Original draft. MB: Investigation, Methodology, Resources, Visualization, Writing – Review and editing. SA: Investigation, Methodology, Resources, Writing – Review and editing. JG: Investigation, Methodology, Resources, Writing – Review and editing. SV: Investigation, Methodology, Resources, Writing – Review and editing. RR: Data curation, Funding acquisition, Supervision, Writing – Review and editing. GP: Methodology, Writing – Review and editing. LA: Data curation, Funding acquisition, Resources, Supervision, Writing – Review and editing. SS: Conceptualization, Resources, Writing – Review and editing. AP: Funding acquisition, Resources, Supervision, Writing – Review and editing.
Funding
The author(s) declare financial support was received for the research, authorship, and/or publication of this article.
This research was supported by a Research Collaboration Agreement between the University of Salzburg and Amsterdam Medical Center, by the University of Salzburg priority program Allergy-Cancer-BioNano Research Centre, and by the Doctoral School Program Biomolecules of the University of Salzburg. LA received support from the Austrian Science Funds (projects P32189 and I 5312-B). This work was also funded in part by InBio and by the National Institute of Allergy and Infectious Diseases of the US National Institutes of Health (award numbers R01AI077653 to AP and MC as well as R21AI123307 and R01AI155668 to SAS). The content is solely the responsibility of the authors and does not necessarily represent the official views of the National Institutes of Health.
Conflict of interest
The research approach was developed by several authors who are employees of InBio (BS, KR, MB, SA, JG, AP, and MC). The hIgE mAb and some allergens used in this study were produced by InBio. AP is a contact principal investigator of the NIH R01 award that provided funding for the study. MC has a financial interest in InBio and is a co-investigator for the NIH R01 award. InBio has a license agreement with Vanderbilt University Medical Center for the commercialization of the hIgE mAb for research and diagnostic purposes. The hIgE mAb covered by this agreement are available from InBio (www.inbio.com). SS is an inventor on US patent 10908168-B2 for the generation of human IgE monoclonal antibodies, has received patent royalties, and has related patents pending. RR is a consultant for HAL Allergy BV, Citeq BV, Angany Inc., Reacta Healthcare Ltd., Mission MightyMe, and AB Enzymes; receives speaker fees from HAL Allergy BV, ALK, and Thermo Fisher Scientific; and has stock options with Angany Inc.
The remaining authors declare that the research was conducted in the absence of any commercial or financial relationships that could be construed as a potential conflict of interest.
The authors declared that they were an editorial board member of Frontiers, at the time of submission. This had no impact on the peer review process and the final decision.
Publisher's note
All claims expressed in this article are solely those of the authors and do not necessarily represent those of their affiliated organizations, or those of the publisher, the editors and the reviewers. Any product that may be evaluated in this article, or claim that may be made by its manufacturer, is not guaranteed or endorsed by the publisher.
References
1. Ishizaka K, Ishizaka T, Hornbrook MM. Physicochemical properties of reaginic antibody: V. Correlation of reaginic activity with gamma-E-globulin antibody. J Immunol. (1966) 97:840–53. doi: 10.4049/jimmunol.97.6.840
2. Johansson SG, Bennich H, Wide L. A new class of immunoglobulin in human serum. Immunology. (1968) 14:265–72.4170889
3. Wide L, Bennich H, Johansson SG. Diagnosis of allergy by an in-vitro test for allergen antibodies. Lancet. (1967) 2:1105–7. doi: 10.1016/S0140-6736(67)90615-0
4. Hamilton RG, Hemmer W, Nopp A, Kleine-Tebbe J. Advances in IgE testing for diagnosis of allergic disease. J Allergy Clin Immunol Pract. (2020) 8:2495–504. doi: 10.1016/j.jaip.2020.07.021
5. McGregor MC, Krings JG, Nair P, Castro M. Role of biologics in asthma. Am J Respir Crit Care Med. (2019) 199:433–45. doi: 10.1164/rccm.201810-1944CI
6. du TG, Sayre PH, Roberts G, Sever ML, Lawson K, Bahnson HT, et al. Effect of avoidance on peanut allergy after early peanut consumption. N Engl J Med. (2016) 374:1435–43. doi: 10.1056/NEJMoa1514209
7. Goodman RE, Chapman MD, Slater JE. The allergen: sources, extracts, and molecules for diagnosis of allergic disease. J Allergy Clin Immunol Pract. (2020) 8:2506–14. doi: 10.1016/j.jaip.2020.06.043
8. Rosenstreich DL, Eggleston P, Kattan M, Baker D, Slavin RG, Gergen P, et al. The role of cockroach allergy and exposure to cockroach allergen in causing morbidity among inner-city children with asthma. N Engl J Med. (1997) 336:1356–63. doi: 10.1056/NEJM199705083361904
9. Platts-Mills T, Vaughan J, Squillace S, Woodfolk J, Sporik R. Sensitisation, asthma, and a modified Th2 response in children exposed to cat allergen: a population-based cross-sectional study. Lancet. (2001) 357:752–6. doi: 10.1016/S0140-6736(00)04168-4
10. Custovic A, Soderstrom L, Ahlstedt S, Sly PD, Simpson A, Holt PG. Allergen-specific IgG antibody levels modify the relationship between allergen-specific IgE and wheezing in childhood. J Allergy Clin Immunol. (2011) 127:1480–5. doi: 10.1016/j.jaci.2011.03.014
11. Salo PM, Arbes SJ Jr., Jaramillo R, Calatroni A, Weir CH, Sever ML, et al. Prevalence of allergic sensitization in the United States: results from the National Health and Nutrition Examination Survey (NHANES) 2005-2006. J Allergy Clin Immunol. (2014) 134:350–9. doi: 10.1016/j.jaci.2013.12.1071
12. Smith SA, Chruszcz M, Chapman MD, Pomés A. Human monoclonal IgE antibodies—a major milestone in allergy. Curr Allergy Asthma Rep. (2023) 23:53–65. doi: 10.1007/s11882-022-01055-w
13. Pomés A, Chruszcz M, Gustchina A, Minor W, Mueller GA, Pedersen LC, et al. 100 years later: celebrating the contributions of x-ray crystallography to allergy and clinical immunology. J Allergy Clin Immunol. (2015) 136:29–37. doi: 10.1016/j.jaci.2015.05.016
14. Pomés A, Mueller GA, Chruszcz M. Structural aspects of the allergen-antibody interaction. Front Immunol. (2020) 11:2067. doi: 10.3389/fimmu.2020.02067
15. Cooke SK, Sampson HA. Allergenic properties of ovomucoid in man. J Immunol. (1997) 159:2026–32. doi: 10.4049/jimmunol.159.4.2026
16. Burks AW, Shin D, Cockrell G, Stanley JS, Helm RM, Bannon GA. Mapping and mutational analysis of the IgE-binding epitopes on Ara h 1, a legume vicilin protein and a major allergen in peanut hypersensitivity. Eur J Biochem. (1997) 245:334–9. doi: 10.1111/j.1432-1033.1997.t01-1-00334.x
17. Suárez-Fariñas M, Suprun M, Kearney P, Getts R, Grishina G, Hayward C, et al. Accurate and reproducible diagnosis of peanut allergy using epitope mapping. Allergy. (2021) 76:3789–97. doi: 10.1111/all.14905
18. Albrecht M, Kuhne Y, Ballmer-Weber BK, Becker WM, Holzhauser T, Lauer I, et al. Relevance of IgE binding to short peptides for the allergenic activity of food allergens. J Allergy Clin Immunol. (2009) 124:328–36. doi: 10.1016/j.jaci.2009.05.031
19. Wurth MA, Hadadianpour A, Horvath DJ, Daniel J, Bogdan O, Goleniewska K, et al. Human IgE mAbs define variability in commercial Aspergillus extract allergen composition. JCI Insight. (2018) 3(20):e123387. doi: 10.1172/jci.insight.123387
20. Chung CH, Mirakhur B, Chan E, Le QT, Berlin J, Morse M, et al. Cetuximab-induced anaphylaxis and IgE specific for galactose-alpha-1,3-galactose. N Engl J Med. (2008) 358:1109–17. doi: 10.1056/NEJMoa074943
21. Mueller GA, Glesner J, Daniel JL, Zhang J, Hyduke N, Richardson CM, et al. Mapping human monoclonal IgE epitopes on the major dust mite allergen der p 2. J Immunol. (2020) 205:1999–2007. doi: 10.4049/jimmunol.2000295
22. Hadadianpour A, Daniel J, Zhang J, Spiller BW, Makaraviciute A, DeWitt ÅM, et al. Human IgE mAbs identify major antigens of parasitic worm infection. J Allergy Clin Immunol. (2022) 150(6):1525–33. doi: 10.1016/j.jaci.2022.05.022
23. Karsonova A, Riabova K, Villazala-Merino S, Campana R, Niederberger V, Eckl-Dorna J, et al. Highly sensitive ELISA-based assay for quantification of allergen-specific IgE antibody levels. Allergy. (2020) 75:2668–70. doi: 10.1111/all.14325
24. Otsu K, Guo R, Dreskin SC. Epitope analysis of Ara h 2 and Ara h 6: characteristic patterns of IgE-binding fingerprints among individuals with similar clinical histories. Clin Exp Allergy. (2015) 45:471–84. doi: 10.1111/cea.12407
25. van Ree R, Chapman MD, Ferreira F, Vieths S, Bryan D, Cromwell O, et al. The CREATE project: development of certified reference materials for allergenic products and validation of methods for their quantification. Allergy. (2008) 63:310–26. doi: 10.1111/j.1398-9995.2007.01612.x
26. Wenger M, Bethanis A, Johnson L, Aglas L. Humanized mediator release assay as a read-out for allergen potency. J Vis Exp. (2021) (172). doi: 10.3791/62702
27. Pena-Castellanos G, Smith BRE, Pomés A, Smith SA, Stigler MA, Widauer HL, et al. Biological activity of human IgE monoclonal antibodies targeting Der p 2, Fel d 1, Ara h 2 in basophil mediator release assays. Front Immunol. (2023) 14:1155613. doi: 10.3389/fimmu.2023.1155613
28. Erwin EA, Custis NJ, Satinover SM, Perzanowski MS, Woodfolk JA, Crane J, et al. Quantitative measurement of IgE antibodies to purified allergens using streptavidin linked to a high-capacity solid phase. J Allergy Clin Immunol. (2005) 115:1029–35. doi: 10.1016/j.jaci.2004.12.1131
29. Satinover SM, Reefer AJ, Pomés A, Chapman MD, Platts-Mills TA, Woodfolk JA. Specific IgE and IgG antibody-binding patterns to recombinant cockroach allergens. J Allergy Clin Immunol. (2005) 115:803–9. doi: 10.1016/j.jaci.2005.01.018
30. Christensen LH, Holm J, Lund G, Riise E, Lund K. Several distinct properties of the IgE repertoire determine effector cell degranulation in response to allergen challenge. J Allergy Clin Immunol. (2008) 122:298–304. doi: 10.1016/j.jaci.2008.05.026
31. Schuurman J, Perdok GJ, Lourens TE, Parren PW, Chapman MD, Aalberse RC. Production of a mouse/human chimeric IgE monoclonal antibody to the house dust mite allergen Der p 2 and its use for the absolute quantification of allergen-specific IgE. J Allergy Clin Immunol. (1997) 99:545–50. doi: 10.1016/S0091-6749(97)70083-6
32. Gadermaier E, Levin M, Flicker S, Ohlin M. The human IgE repertoire. Int Arch Allergy Immunol. (2014) 163:77–91. doi: 10.1159/000355947
33. Steinberger P, Kraft D, Valenta R. Construction of a combinatorial IgE library from an allergic patient. Isolation and characterization of human IgE Fabs with specificity for the major timothy grass pollen allergen, Phl p 5. J Biol Chem. (1996) 271:10967–72. doi: 10.1074/jbc.271.18.10967
34. Croote D, Darmanis S, Nadeau KC, Quake SR. High-affinity allergen-specific human antibodies cloned from single IgE B cell transcriptomes. Science. (2018) 362:1306–9. doi: 10.1126/science.aau2599
35. Khatri K, Richardson CM, Glesner J, Kapingidza AB, Mueller GA, Zhang J, et al. Human IgE monoclonal antibody recognition of mite allergen Der p 2 defines structural basis of an epitope for IgE cross-linking and anaphylaxis in vivo. PNAS Nexus. (2022) 1:pgac054. doi: 10.1093/pnasnexus/pgac054
36. Suber J, Zhang Y, Ye P, Guo R, Burks AW, Kulis MD, et al. Novel peanut-specific human IgE monoclonal antibodies enable screens for inhibitors of the effector phase in food allergy. Front Immunol. (2022) 13:974374. doi: 10.3389/fimmu.2022.974374
37. Kreft L, Schepers A, Hils M, Swiontek K, Flatley A, Janowski R, et al. A novel monoclonal IgG1 antibody specific for galactose-alpha-1,3-galactose questions alpha-gal epitope expression by bacteria. Front Immunol. (2022) 13:958952. doi: 10.3389/fimmu.2022.958952
38. Crispell G, Commins SP, Archer-Hartman SA, Choudhary S, Dharmarajan G, Azadi P, et al. Discovery of alpha-gal-containing antigens in North American tick species believed to induce red meat allergy. Front Immunol. (2019) 10:1056. doi: 10.3389/fimmu.2019.01056
39. Villar M, Pacheco I, Mateos-Hernández L, Cabezas-Cruz A, Tabor AE, Rodríguez-Valle M, et al. Characterization of tick salivary gland and saliva alphagalactome reveals candidate alpha-gal syndrome disease biomarkers. Expert Rev Proteomics. (2021) 18:1099–116. doi: 10.1080/14789450.2021.2018305
40. Wilson JM, Nguyen AT, Schuyler AJ, Commins SP, Taylor AM, Platts-Mills TAE, et al. IgE to the mammalian oligosaccharide galactose-α-1,3-galactose is associated with increased atheroma volume and plaques with unstable characteristics-brief report. Arterioscler Thromb Vasc Biol. (2018) 38:1665–9. doi: 10.1161/ATVBAHA.118.311222
41. Pattarabanjird T, Wilson JM, Erickson LD, Workman LJ, Qiao H, Ghosheh Y, et al. Chemokine receptor activation enhances memory B cell class switching linked to IgE sensitization to alpha gal and cardiovascular disease. Front Cardiovasc Med. (2021) 8:791028. doi: 10.3389/fcvm.2021.791028
42. Kuravi KV, Sorrells LT, Nellis JR, Rahman F, Walters AH, Matheny RG, et al. Allergic response to medical products in patients with alpha-gal syndrome. J Thorac Cardiovasc Surg. (2022) 164:e411–24. doi: 10.1016/j.jtcvs.2021.03.100
43. Chapman MD, Wuenschmann S, King E, Pomés A. Technological innovations for high-throughput approaches to in vitro allergy diagnosis. Curr Allergy Asthma Rep. (2015) 15:36. doi: 10.1007/s11882-015-0539-8
44. Santos AF, Alpan O, Hoffmann HJ. Basophil activation test: mechanisms and considerations for use in clinical trials and clinical practice. Allergy. (2021) 76:2420–32. doi: 10.1111/all.14747
45. Schiel JE, Turner A, Mouchahoir T, Yandrofski K, Telikepalli S, King J, et al. The NISTmAb reference material 8671 value assignment, homogeneity, and stability. Anal Bioanal Chem. (2018) 410:2127–39. doi: 10.1007/s00216-017-0800-1
Keywords: IgE antibodies, allergy diagnostics, food allergy, alpha-gal, allergenic epitopes
Citation: Smith BRE, Reid Black K, Bermingham M, Agah S, Glesner J, Versteeg SA, van Ree R, Pena-Amelunxen G, Aglas L, Smith SA, Pomés A and Chapman MD (2023) Unique allergen-specific human IgE monoclonal antibodies derived from patients with allergic disease. Front. Allergy 4:1270326. doi: 10.3389/falgy.2023.1270326
Received: 31 July 2023; Accepted: 25 September 2023;
Published: 12 October 2023.
Edited by:
Rudolf Valenta, Medical University of Vienna, AustriaReviewed by:
Sabine Flicker, Medical University of Vienna, AustriaPeter Steinberger, Medical University of Vienna, Austria
Mats Ohlin, Lund University, Sweden
© 2023 Smith, Reid Black, Bermingham, Agah, Glesner, Versteeg, van Ree, Pena-Amelunxen, Aglas, Smith, Pomés and Chapman. This is an open-access article distributed under the terms of the Creative Commons Attribution License (CC BY). The use, distribution or reproduction in other forums is permitted, provided the original author(s) and the copyright owner(s) are credited and that the original publication in this journal is cited, in accordance with accepted academic practice. No use, distribution or reproduction is permitted which does not comply with these terms.
*Correspondence: Martin D. Chapman mdc@inbio.com
†These authors have contributed equally to this work and share first authorship