- 1Division of Biochemistry, Faculty of Pharmacy, School of Pharmaceutical Sciences, Keio University, Tokyo, Japan
- 2Department of Microbiology and Immunology, School of Pharmaceutical Sciences, Wakayama Medical University, Wakayama, Japan
- 3Department of Immunology, Institute of Advanced Medicine, Wakayama Medical University, Wakayama, Japan
- 4Laboratory for Inflammatory Regulation, RIKEN Center for Integrative Medical Science (IMS-RCAI), Yokohama, Japan
- 5Precursory Research for Embryonic Science and Technology (PRESTO), Japan Science and Technology Agency, Saitama, Japan
- 6Institute of Fermentation Sciences (IFeS), Faculty of Food and Agricultural Sciences, Fukushima University, Fukushima, Japan
- 7International Research and Development Center for Mucosal Vaccines, The Institute of Medical Science, The University of Tokyo (IMSUT), Tokyo, Japan
Food allergy is a type I allergic reaction induced by mast cells and is mainly activated by allergen-specific immunoglobulin (Ig)E. Spi-B is an E26-transformation-specific (Ets) family transcription factor essential for the differentiation and functional maturation of several immune cell subsets, including mast cells. However, the possible involvement of Spi-B in food allergy remains unclear. In this study, we found that Spi-B-deficient mice were highly susceptible to food allergy to ovalbumin (OVA), as indicated by the exacerbation of diarrhea and elevation of serum IgE levels. These pathological changes were associated with enhanced mast cell infiltration into the intestinal lamina propria. Activation of mast cells in the intestinal mucosa was observed in Spib−/− mice, even under physiological conditions. Accordingly, Spi-B deficiency increased the translocation of fluorescently labeled dextran from the lumen to the serum, suggesting increased intestinal permeability in Spib−/− mice. Moreover, Spib−/− mice showed defects in oral tolerance induction to OVA. These data illustrate that Spi-B suppresses the development of food allergies by controlling the activation of intestinal mast cells and by inducing immune tolerance to food allergens.
Introduction
In food allergy, certain food ingredients trigger an abnormal immune response, including the induction of an antigen-specific T-helper-2 (Th2) response and impaired immune tolerance (1, 2). Food allergy symptoms can be systemic, including diarrhea, vomiting, skin rashes, and occasionally, shortness of breath (2). Immunoglobulin (Ig) E response mainly contributes to the pathogenesis of food allergies in sensitization and effector phases (3). During the sensitization phase, intimal exposure to a food allergen induces allergen-specific IgE, which binds to the high-affinity IgE receptor, FcεRI, on the surface of mast cells. Subsequent exposure to the same food allergen cross-links allergen-specific IgE to mast cells, eliciting an effector phase response. At the early stage of the effector phase, mast cells release chemical mediators, such as histamine, prostaglandins, and leukotrienes, to induce allergic symptoms. Furthermore, chemical mediators and cytokines (i.e., IL-4, IL-5, IL-13, and IL-33) produced by mast cells and basophils activate eosinophils and Th2 cells, which further amplify allergic reactions (4). Mast cell-deficient mice and mice with decreased mast cell activation show attenuated symptoms in food allergy models (5, 6), indicating that mast cells play a central role in food allergies.
The intestinal barrier system is well-developed to prevent the invasion of undesired antigens and harmful organisms. Intestinal epithelial cells form a physical barrier by tightly binding to each other through tight junctions. The thick mucus layers covering the intestinal epithelium act as physical barriers. Furthermore, secreted IgA functions as a humoral defense system to provide a first-line defense against microbes and allergens by inhibiting their attachment to the mucosal surfaces (7). Growing evidence suggests that intestinal barrier dysfunction contributes to food hypersensitivity through increased sensitization. For example, abnormal intestinal permeability has been observed in infants with food allergies (8). Furthermore, intestinal IL-9 overexpression predisposes oral antigen sensitization by increasing intestinal permeability through mast cell activation, eventually inducing intestinal anaphylaxis (9).
Spi-B is an E26-transformation-specific (Ets) family transcription factor expressed in various cells, including hematopoietic cells, plasmacytoid DCs (pDCs), microfold (M) cells, and tuft cells (10–14). This transcription factor has various functions, including the generation of lymphoid progenitor cells, B-cell proliferation and survival, and the development and functional maturation of pDCs (11, 13). Spi-B suppresses the differentiation of myeloid progenitor cells into mast cells by controlling the expression balance between PU.1 and GATA1 (15–17). Consequently, Spi-B deficiency enhances the number of mast cells. Interestingly, Spib−/− phenotype in mice was pronounced in the intestinal tract, as manifested by the prevention of helminthic infections in the intestinal tract by activated mast cells (18). We previously reported that Spi-B is critical for the differentiation of M cells that contribute to antigen-specific IgA response by transporting luminal antigens to mucosa-associated lymphoid tissue such as Peyer's patches (PPs) (12, 19). Therefore, Spi-B contributes to maintaining immune homeostasis, especially in the intestine; however, its influence on food allergies remains unknown.
In this study, we induced food allergies in Spib−/− mice to elucidate the association between Spi-B and food allergies. Spi-B deficiency caused severe allergic diarrhea and elevated antigen-specific IgE production. Mast cells were constitutively activated, and epithelial permeability was increased in Spib−/− mice. Furthermore, the delayed-type hypersensitivity (DTH) response was not sufficiently suppressed by the oral administration of antigens, suggesting that Spib−/− mice fail to develop oral tolerance. Our data illustrate the protective role of Spi-B in food allergies by securing intestinal barrier function and oral tolerance.
Materials and methods
Animals
BALB/cA mice were obtained from Japan Clea Co. (Tokyo, Japan). Spib−/− mice on a C57BL/6J background were backcrossed with BALB/cA mice at least eight times. All experiments were performed on age- and sex-matched mice (5–6 weeks old) and approved by the Keio University Animal Care Committee. The mice were housed under conventional conditions and received a standard diet (AIN-93G, Oriental Yeast, Osaka, Japan) and tap water.
Induction of food allergy
The food allergy (FA) model was created using a modified version of a previously reported method (20). Mice were intraperitoneally sensitized to 50 μg ovalbumin (OVA; purity ≥98%; Sigma Aldrich, St. Louis, MO, USA) with 50 µl Imject™ Alum (6 mg aluminum hydroxide and 6 mg magnesium hydroxide; Thermo Fisher Scientific, Rockford, IL, USA) once a week for a total of two times. One week after the last sensitization, the mice were orally administered OVA (1.0–1.3 mg/g body weight, purity ≥90%; Sigma Aldrich) four times every two days. Fecal consistency was visually examined within 1 h following each oral OVA administration to determine the fecal score as follows: 0, normal hard stool; 1, normal stool; 2, soft stool with shape; 3, soft stool out of shape; 4, diarrhea, liquid or without shape (21, 22).
Intestinal permeability
Mice were fasted for 4 h and then orally administered 4-kDa fluorescein isothiocyanate (FITC)-dextran (60 mg/100 g body weight; Sigma Aldrich). After 45 min, blood samples were collected via the facial vein and mixed with heparin (Mochida Pharmaceutical Co., Ltd., Tokyo, Japan). The blood samples were centrifuged at 2,000 × g for 10 min to collect plasma, which was then serially diluted from 1:1 to 1:4 in PBS (pH 7.4; Nacalai Tesque Inc., Kyoto, Japan) and 4-kDa FITC-dextran concentration was analyzed with a fluorescence spectrophotometer (Infinite 2,000; Tecan, Männedorf, Switzerland) at an excitation wavelength of 485 nm and an emission wavelength of 535 nm. Standard curves were obtained using the values of stepwise diluted 4-kDa FITC-dextran in PBS.
ELISA
The amounts of OVA-specific immunoglobulins in the serum and fecal samples were measured using ELISA. The 96-well ELISA plate (F96 Maxisorp Nunc-Immuno plate, Thermo Fisher Scientific) was coated with 50 μl OVA/PBS (1 mg/ml) per well for 16–18 h at 4 °C. Blood samples were collected from the facial vein (days 1, 6, and 13) and the heart (day 20). After 1 h incubation at room temperature (24–26 °C), the blood samples were centrifuged at 10,000 × g for 3 min, and sera were collected. Sera were further centrifuged at 10,000 × g for 3 min to avoid contamination with blood clots. The collected sera were diluted in 1% bovine serum albumin (BSA)/PBS for ELISA analysis and the amount of OVA-specific IgE was measured using an IgE ELISA kit (#432401; BioLegend, San Diego, CA, USA) according to the manufacturer's instructions. The amount of mucosal mast cell protease-1 (MMCP-1) in serum was measured using the ELISA Kit (#88-7503-88, Invitrogen, Waltham, USA).
To measure IgA levels, fecal samples were crushed and suspended in PBS containing a protease inhibitor cocktail (cOmplete EDTA-free®; Roche Diagnostics, Risch-Rotkreuz, Switzerland). The obtained fecal suspension was centrifuged at 2,000 × g for 10 min to collect the supernatants. To measure IgA levels, OVA-coated ELISA plates were washed three times with 0.1% Tween-20 (Nacalai Tesque) in PBS and then blocked with 2% BSA (Nacalai Tesque) in PBS for at least 1 h. Fecal supernatants were added to the wells and incubated for 2 h. After washing, IgA targeting HRP-conjugated antibodies (1:10000 dilution; Bethyl Laboratories, Montgomery, AL, USA) were added to the plate. After 1 h incubation and four washes, 100 μl TMB ELISA solution (Thermo Fisher Scientific) was added to each well and incubated in the dark. The enzymatic reaction was stopped by adding 100 μl of 1.2 M H2SO4. Optical density was measured at 450 nm and 570 nm using a microplate reader (Infinite 200; Tecan).
Flow cytometry
PPs, mesenteric lymph nodes (MLN), and spleen were isolated on day 20 (after 4th OVA challenge) and crushed using 1 ml syringe plungers and 100 μm cell strainers (Greiner Bio-One, Kremsmünster, Austria). Splenocytes were treated with RBC-Lysis buffer (BioLegend) to lyse the red blood cells. After filtering with 100 µm cell strainers, the single-cell suspensions were washed with HBSS (Nacalai Tesque) and resuspended with PBS containing 100 units/ml penicillin, 100 μg/ml streptomycin (#26253, Nacalai Tesque), 10 mM HEPES (pH 7.3, Nacalai Tesque), and 2% newborn calf serum (NBCS, Gibco, Thermo Fisher Scientific). The samples were incubated with anti-mouse CD16/32 antibody (BioLegend) to block the Fc receptor and then stained using antibodies listed in Supplementary Table S1. To label the dead cells, 7-AAD (BioLegend) was added to the cell suspension. The cells were analyzed using a flow cytometer (BD FACSCelesta or BD LSRII; BD Biosciences, NJ, USA). The leukocyte number was determined according to the manufacturer's protocol using precision count beads (BioLegend).
Immunostaining of mast cells
Jejunal tissues were dissected 5–6 cm from the pyloric region of the stomach. After fixation with 4% paraformaldehyde (PFA) (Nacalai Tesque) in PBS for 4 h, the tissues were placed in 30% sucrose (Nacalai Tesque) solution at 4 °C overnight. The tissues embedded in the OCT compound (Sakura Finetek, Tokyo, Japan) were quickly frozen in liquid nitrogen. Frozen blocks were sliced into 30 µm thin sections. Well-dried tissue sections on glass slides were treated with 0.3% Triton X-100 (Nacalai Tesque) in PBS for 15 min and then incubated with 10% donkey serum (Sigma Aldrich) for 30 min. Next, the sections were incubated with sheep anti-MMCP-1 antibody (1 μg/ml, clone #285008; R/D Systems, Inc., Minneapolis, MN, USA) and rat anti-E-cadherin (2.5 ng/ml, clone ECCD-2; Takara Bio Inc., Shiga, Japan) overnight, washed with PBS three times, and then treated with Cy3-labeled anti-sheep IgG (Jackson Immunoresearch, West Grove, PA, USA), Alexa Fluor® 488-labeled anti-rat IgG (Jackson Immunoresearch) and Hoechst 33342 (Molecular Probes, OR, USA) for 2 h. The stained sections were mounted using SlowFadeGold mounting medium (Thermo Fisher Scientific). The specimens were observed using an FV3000 confocal laser scanning microscope (Olympus, Tokyo, Japan).
Induction of oral tolerance
Oral tolerance was induced, as described in a previous study (23). Briefly, 25 mg OVA (purity >90%; Sigma Aldrich) in 0.25 ml PBS (Nacalai Tesque) or vehicle as a negative reference were orally administered to mice. The mice were subcutaneously immunized with 100 μg OVA and 100 μg complete Freund's adjuvant (CFA; Becton Dickinson, and Company Franklin Lakes, NJ, USA) 7 days after oral administration. After 14 days, serum OVA-specific IgG levels were analyzed. The DTH response was examined 7 days after subcutaneous sensitization. Ten micrograms OVA in 20 μl PBS or only PBS as a negative reference was administered to the right or left ear pinna, respectively. Ear swelling was measured using a micrometer (Mitutoyo, Kawasaki, Japan) 0 and 24 h after intradermal OVA administration. The DTH response was determined as the difference in thickness between the right and left ears in each mouse.
Statistical analysis
Values are expressed as mean ± standard error of the mean (SEM). Differences between the two groups were analyzed using an unpaired t-test. When variances were not homogeneous, data were analyzed using the Mann–Whitney U test. Differences between more than three groups were analyzed using the one-way analysis of variance (ANOVA) followed by Tukey's test. Non-parametric data were analyzed using the Kruskal–Wallis test, followed by Dunn's test. IgE and IgA levels and DTH response data were analyzed using two-way ANOVA, followed by Tukey's test. GraphPad Prism version 9 was used for all statistical analyses. Differences were considered statistically significant at p values less than 0.05.
Results
Spi-B deficiency exacerbates the symptoms of food allergy
We evaluated the effect of Spi-B deficiency on the development of food allergies. Since BALB/c mice are more susceptible to allergic diseases than C57BL/6 mice, we generated Spib−/− mice with a BALB/cA genetic background. Spib−/− and wild-type (WT) BALB/cA mice were sensitized by intraperitoneal injection with OVA and alum twice and challenged with OVA orally (Figure 1A). Fecal scores were measured as described in the Materials and Methods section. In WT mice, the incidence of diarrhea was 20% until the third challenge (day 18) and then increased up to 50% at the fourth challenge (day 20). Meanwhile, 60% of the Spib−/− mice developed diarrhea during the first challenge, with an incidence of 80% at the third challenge (Figure 1B). Likewise, the total stool scores for the four OVA challenges tended to increase in Spib−/− mice compared with sensitized WT control mice (Figure 1C). There was no significant difference between the stool scores of the non-sensitized Spib−/− and WT mice (Supplementary Figure S1). These observations imply that Spi-B deficiency aggravates the development of food allergies.
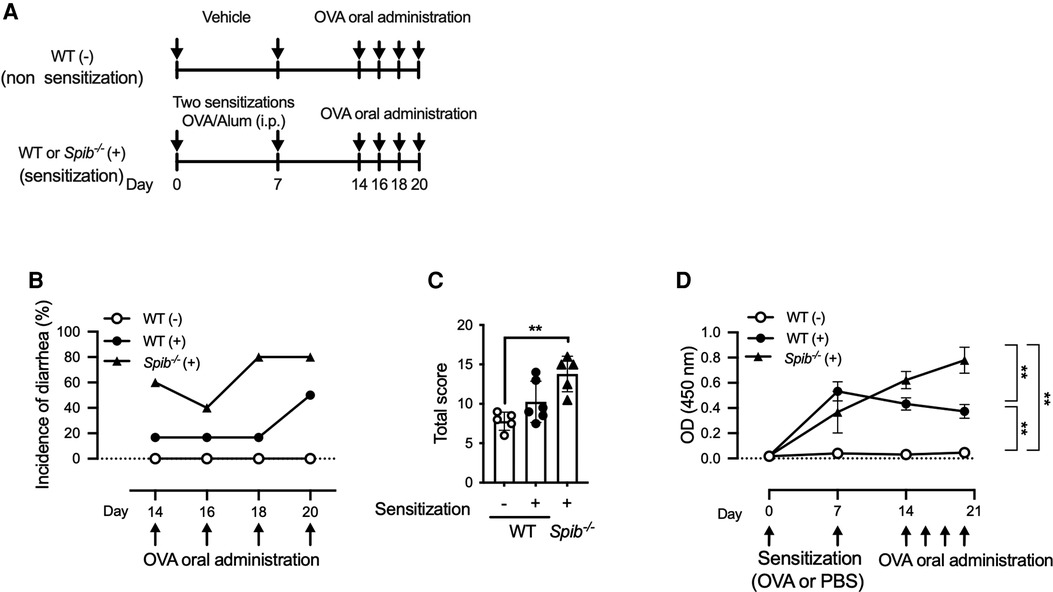
Figure 1. Spi-B deficiency exacerbates the symptoms of OVA-induced food allergy. (A) Experimental design for sensitization and oral administration of OVA to develop a food allergy model. (B,C) Fecal consistency was visually examined within 1 h of each oral OVA administration to determine the fecal score. The incidence of diarrhea was indicated by the fecal score of 4 (B) and total fecal scores during Day 14–20 (C). (D) Time course of OVA-specific IgE production after OVA sensitization. Serum samples were collected on the indicated days to measure OVA-specific IgE levels. Representative data from at least three independent experiments are shown. Data are expressed as the mean ± SEM (n = 5 or 6). (C,D) **p < 0.01, calculated using the Kruskal–Wallis test and two-way ANOVA.
Serum OVA-specific IgE increases in Spib−/− mice
IgE is a significant mediator of food allergic reactions. The serum levels of OVA-specific IgE in WT mice culminated on day 7 and slightly declined until day 20 (Figure 1D). In contrast, Spib−/− mice exhibited increased serum OVA-specific IgE levels after sensitization, which further elevated until day 20 (Figure 1D). Therefore, the serum levels of OVA-specific IgE on day 20 were significantly higher in Spib−/− mice than in WT mice (Figure 1D). Notably, OVA-specific IgE was undetectable in the serum of WT mice who were not sensitized (Figure 1D).
We performed flow cytometric analysis of lymphocytes from PPs, MLN, and the spleen to determine the number of IgE-producing B cells. Spi-B deficiency severely affected the total number of CD45+ leukocytes in the PPs of BALB/c background mice (Figures 2A,B), consistent with our previous report using Spib−/− C57BL/6J mice (19). Therefore, we mainly analyzed the MLN and spleen in subsequent analyses. In the spleen and MLN of WT mice, OVA sensitization increased the number of IgE+B220+ and IgE+B220− cells (Figures 2C,D). The numbers of both populations tended to decrease in Spib−/− mice, most likely because of the reduction in total lymphocytes (Figure 2B). In contrast, the number of IgE+B220− cells in the spleen was slightly increased in Spib−/− mice under both non-sensitized and sensitized conditions. However, the differences were not significant between the two groups (Figure 2D). These observations suggest that the spleen may be a significant site for OVA-specific IgE response in Spib−/− mice.
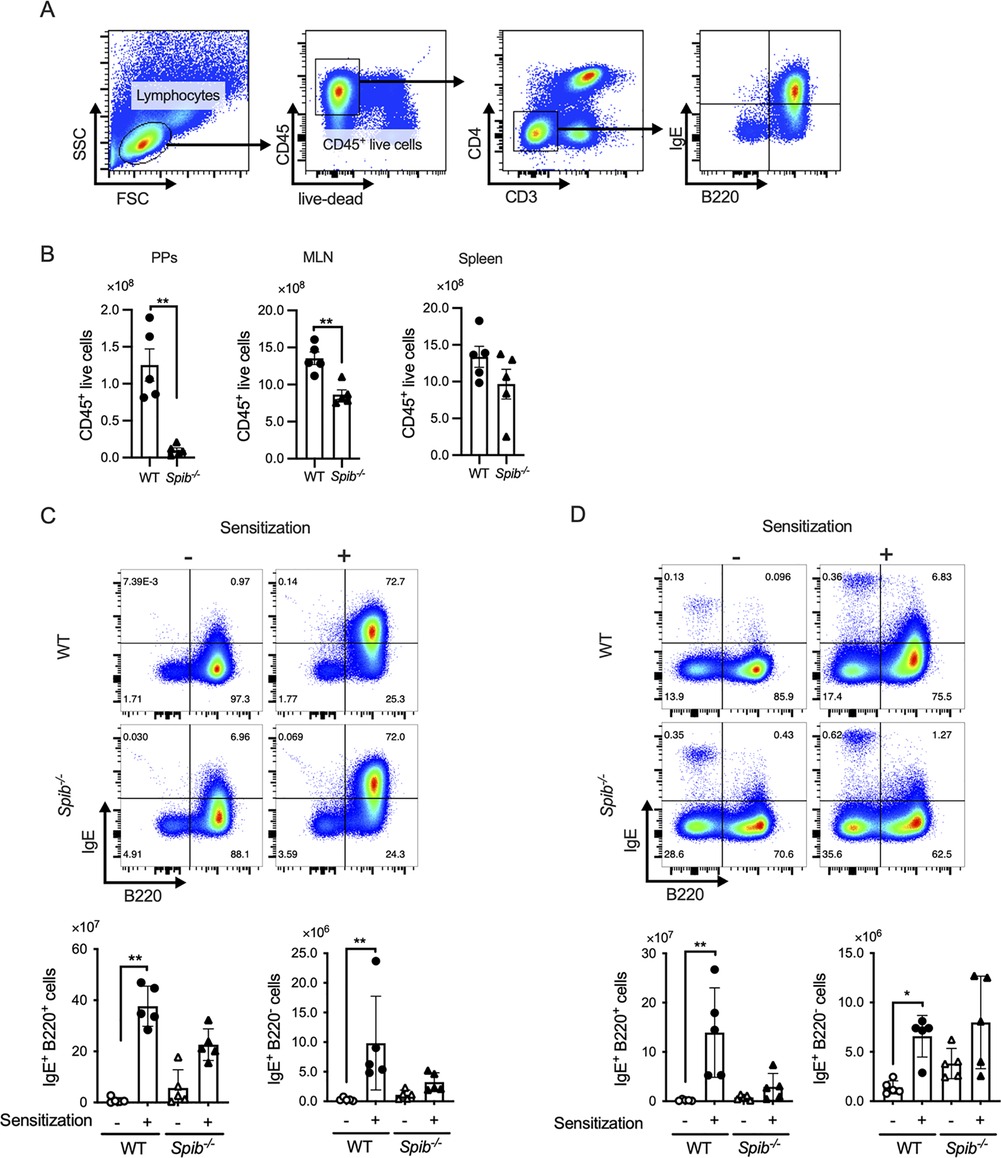
Figure 2. Splenic IgE+ cells are responsible for IgE production in Spib−/− mice. (A) Gating strategy of CD3− CD4− CD45+ live lymphocytes. (B) The numbers of CD45+ live lymphocytes of Peyer's patches (PPs), the mesenteric lymph node (MLN), and the spleen from OVA-sensitized mice (day 20). (C,D) The CD3− CD4− 7-AAD− CD45+ cells were further divided into IgE+ B220− and IgE+ B220− cells. The bar graphs show the number of IgE+ B220− and IgE+ B220− cells in the MLN (C) and the spleen (D). Representative data from at least three independent experiments are shown. Data are expressed as mean ± SEM (n = 5 or 6). *p < 0.05, **p < 0.01, calculated by unpaired t-test for B and Kruskal–Wallis test for C,D.
Spi-B deficiency activates mast cells in the intestinal mucosa
Mast cells are the major effectors in the development of IgE-dependent food allergy symptoms. Therefore, we analyzed the number and activation status of mast cells in the intestine after oral OVA challenge, with or without sensitization. MMCP-1 is a protease primarily expressed by mast cells in mucosal tissues. Immunofluorescence analysis of MMCP-1 demonstrated that OVA sensitization facilitated the accumulation of MMCP-1+ mast cells in the small intestinal lamina propria (siLP) of sensitized but not non-sensitized WT mice (Figure 3A). In contrast to non-sensitized WT mice, a substantial number of MMCP-1+ cells was observed in the siLP of Spib−/− mice, even under non-sensitized conditions (Figure 3A). Quantitative image analysis confirmed that the number of MMCP-1+ cells was significantly higher in Spib−/− mice than in WT mice under non-sensitized conditions (Figure 3B). MMCP-1 is stored in a granular form under a steady state and is rapidly secreted by mast cells upon their activation. Indeed, the serum level of MMCP-1 was significantly elevated after oral challenge with OVA in sensitized mice (Figure 3C). Serum MMCP-1 levels tended to be higher in Spib−/− mice than in WT mice. These results suggest that Spi-B deficiency accelerates the accumulation and activation of mast cells in the intestinal mucosa under allergic conditions (Figure 3C).
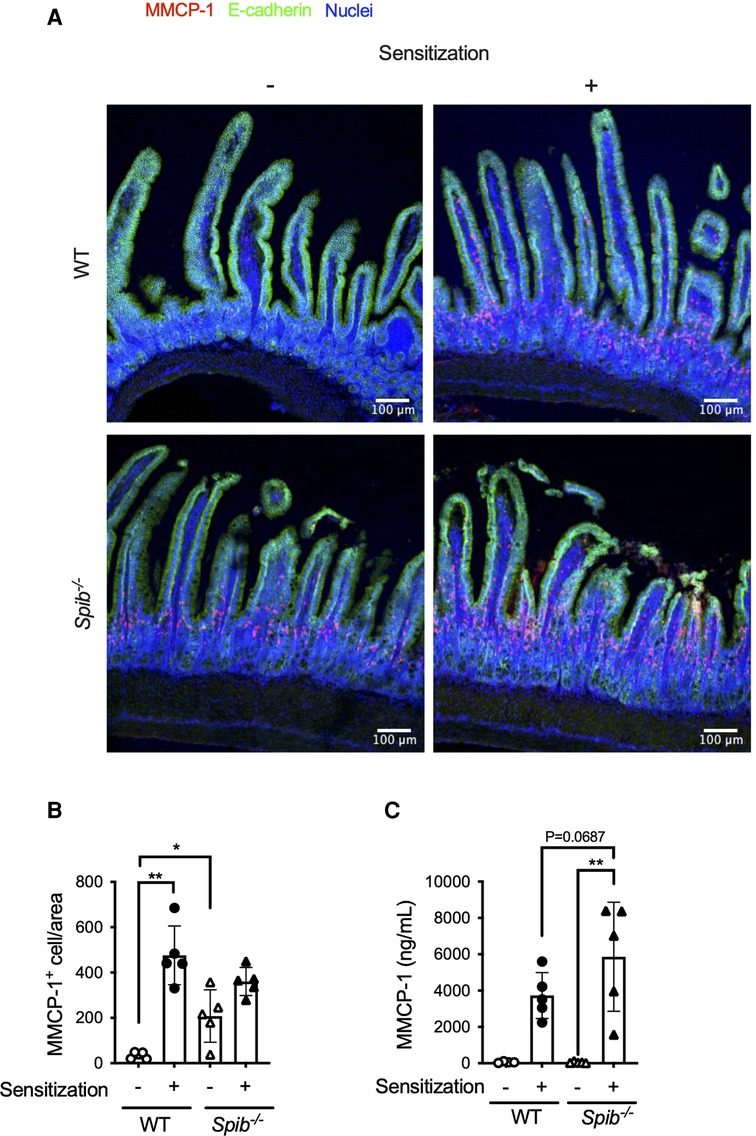
Figure 3. Spi-B deficiency increases intestinal mast cell activity. (A) Immunofluorescent images of the upper small intestine after OVA challenge (day 20). MMCP-1+ mast cells (red), E-cadherin (green), and nucleus (blue) are shown. Scale bars, 100 µm. (B,C) The number of MMCP-1+ mast cells in the intestine (B) and MMCP-1 level in the serum (C) were measured on day 20. Representative data from at least two independent experiments are shown. Data are expressed as mean ± SEM (n = 5 or 6). *p < 0.05, **p < 0.01, calculated by Kruskal–Wallis test for (B,C).
Spi-B deficiency impaired intestinal barrier functions
Given that mast cells infiltrated the siLP of non-sensitized Spib−/− mice (Figures 3A,B), we postulated that the absence of Spi-B may activate mast cells, even under physiological conditions. This hypothesis was supported by higher serum MMCP-1 levels in Spib−/− mice than in WT mice (Figure 4A). Since activated mast cells increase intestinal epithelial permeability (7, 24), we assessed this by measuring the translocation of FITC-labeled dextran from the intestinal lumen to the blood circulation. Spib−/− mice showed a significant increase in the amount of FITC-labeled dextran in the plasma, indicating increased intestinal permeability caused by Spi-B deficiency (Figure 4B).
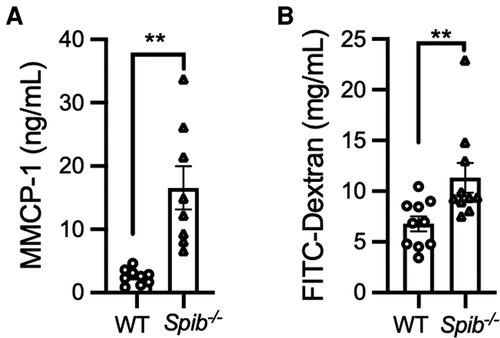
Figure 4. Degranulation of intestinal mast cells and increased intestinal permeability in Spib−/− mice at the steady state. (A) The steady-state serum level of MMCP-1 was measured by ELISA. (B) The fluorescent intensity of FITC-dextran was measured in the plasma at steady-state. Representative data from at least two independent experiments are shown. Data are expressed as mean ± SEM (n = 10 or 11). **p < 0.01, calculated by Mann–Whitney U test.
IgA secretion into the intestinal mucosa establishes a humoral immune barrier to prevent penetration of external antigens, including allergens, into the body. We measured fecal OVA-specific IgA levels at the same point as the measurement of serum IgE levels (Supplementary Figure S2). OVA-IgA was detected only after the fourth oral administration (day 20) and was markedly decreased in Spib−/− mice compared to WT mice.
Oral tolerance is disrupted in Spib−/− mice
Since the failure of oral tolerance predisposes individuals to food allergies, we investigated whether Spi-B deficiency affects oral tolerance. We first examined DTH response by measuring ear swelling on day 14 (Figure 5A). The DTH response to intradermal challenge with OVA was completely suppressed in OVA-fed WT mice but not in OVA-fed Spib−/− mice (Figure 5B).
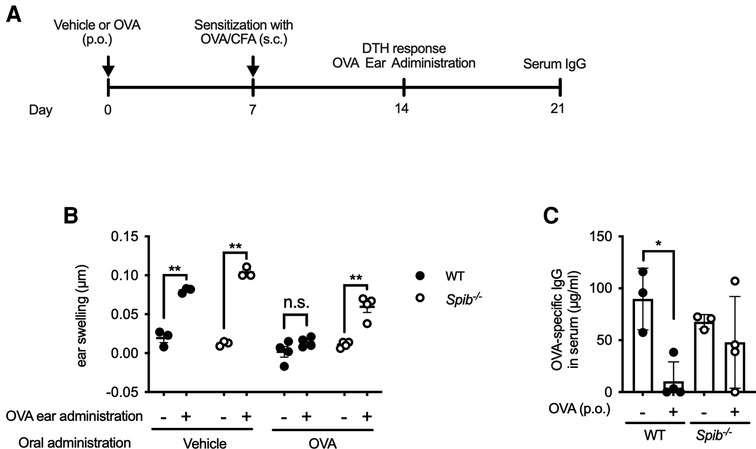
Figure 5. The oral tolerance is disrupted in Spib−/− mice. (A) The experimental design of investigating oral tolerance. (B) OVA/PBS or PBS were injected in the right or left ear, respectively. The ear swelling was measured to estimate DTH response. (C) OVA-specific IgG levels in the serum were measured by ELISA. Representative data from at least two independent experiments are shown. Data are expressed as mean ± SEM (n = 3 or 4). *p < 0.05, **p < 0.01, n.s.: not significant, calculated by two-way ANOVA for (B) and one-way ANOVA for (C).
Similarly, the induction of OVA-specific IgG was almost entirely suppressed in OVA-fed WT mice. In contrast, there was no significant difference in OVA-specific IgG levels between vehicle- and OVA-fed Spib−/− mice (Figure 5C). Therefore, we reasoned that Spi-B was essential for oral tolerance induction.
Discussion
The current study showed that Spi-B is a crucial regulator of food allergies in mice. Spi-B deficiency increases antigen-specific IgE levels and activates mast cells in the intestine. Consequently, the symptoms of diarrhea were exacerbated in Spib−/− mice. Interestingly, in Spib−/− mice, mast cells were activated even in the steady state and infiltrated into the siLP after oral administration of OVA without sensitization. Thus, mast cells were readily activated in the absence of Spi-B. Therefore, it is conceivable that excessive activation of mast cells contributes to the exacerbation of diarrhea symptoms in Spib−/− mice. An earlier study revealed that Spi-B regulates granulocyte differentiation by balancing GATA-1 and PU.1 that directly interact with and suppress each other (15–17). Hence, Spi-B deficiency expands the mast cell population to eliminate helminth infection by promoting the activation of ILC2 (18).
We also detected increased intestinal permeability in Spib−/− mice, which indicates an attenuated epithelial barrier. MMCP-1 release upon mast cell degranulation increases intestinal permeability in collaboration with other mast cell proteases, rMCP-II and MCPT-4, leading to allergic intestinal hypersensitivity (9, 24, 25). A recent study showed that Spi-B is essential for the proliferation of Tuft-2 cells, which sense bacterial metabolites to promote mucus secretion by goblet cells (14). Consistently, Spi-B deficiency causes thinning of the mucus layer by reducing mucus secretion from the goblet cells. Thus, the attenuated epithelial barrier in Spib−/− mice may have resulted from the activation of mast cells and the reduction of mucus secretion. Eventually, Spi-B deficiency may enhance the translocation of food antigens from the lumen to the intestinal tissue, leading to the early onset of diarrhea (Figure 1B). However, further investigations are necessary to verify this pathological model.
Spi-B deficiency causes the loss of functional M cells (12). Because M cells are responsible for luminal antigen uptake in mucosa-associated lymphoid tissue, Spi-B deficiency severely affects intestinal microbiota-specific IgA production and cellular immunity, such as the Th17 response (19). Consistent with the results of a previous study, we observed a reduction in OVA-specific IgA production in Spib−/− mice (19). Given that IgA suppresses attachment and invasion of antigens to the intestinal epithelium, the diminished IgA response in Spib−/− mice may further attenuate mucosal barrier function. Nevertheless, the diminished IgA response is unlikely to contribute to the exacerbation of food allergy symptoms in Spib−/− mice because OVA-specific fecal IgA was not detected until the late stage of food allergy (day 20) in WT and Spib−/− mice. In contrast, the exacerbation of diarrhea was already observed at the first OVA challenge (day 14) in Spib−/− mice.
In contrast to fecal IgA, serum levels of OVA-specific IgE were higher in Spib−/− mice than in wild-type mice. The number of IgE+B220− cells in the spleen under allergic conditions was sufficiently high and similar to that in the WT. The number of IgE+B220+ cells in the spleen was lower in Spib−/− mice than in WT mice. These observations raise the possibility that Spi-B deficiency may accelerate the differentiation of IgE+ B cells into plasmablasts/plasma cells in the spleen, elevating the serum IgE level in Spib−/− mice. Notably, the IgE+B220+ and IgE+B220− cell populations in the MLN were comparable between the two groups or slightly decreased in Spib−/− mice. While Spi-B is required for the antigen-specific IgA response and accumulation of immune cells in gut-associated lymphoid tissues, it may contribute to mitigating antigen-specific IgE responses in the systemic immune system.
Furthermore, OVA-specific IgE production in Spib−/− mice increased continuously throughout the oral challenge, although IgE production in WT mice tended to decrease slightly during repeated oral administration of OVA. This anomaly may be attributed to the impaired oral tolerance in Spib−/− mice. In support of this view, oral OVA administration to Spib−/− mice failed to suppress DTH and antigen-specific IgG responses. Compromised oral tolerance is a predisposing factor for food allergies (26, 27). Therefore, exacerbation of allergic symptoms in Spib−/− mice may at least partially result from impaired immune tolerance. Further studies are required to elucidate the mechanism by which Spi-B ensures oral tolerance. Notably, the locus of human SPIB is associated with primary biliary cirrhosis, an autoimmune disease (28). Thus, Spi-B may also play a role in the suppression of allergies and autoimmunity.
As the limitation study, we here employed systemic Spi-B KO mice. Because Spi-B is expressed in several types of cell lineages (e.g., myeloid progenitors, M cells, and pDCs), we were unable to identify the cell type responsible for exacerbating allergy. The generation of conditional knockout mice will elucidate the mechanisms of allergy exacerbation by Spi-B deficiency. Additionally, further investigations will be necessary to verify the pathological model that the elevation of mast cell-derived MMCP-1 due to Spi-B deficiency causes epithelial barrier dysfunction.
Within the limitation of this study, our study demonstrated the importance of Spi-B in ameliorating food allergies. Spi-B alleviates mast cell activation in the intestinal mucosa and secures intestinal barrier function and oral tolerance. Further analyses of the mucosal barrier and immunity mediated by Spi-B will improve our understanding of how immune homeostasis is maintained in the intestinal mucosa.
Data availability statement
The original contributions presented in the study are included in the article/Supplementary Materials, further inquiries can be directed to the corresponding author/s.
Ethics statement
The animal study was reviewed and approved by the Keio University Animal Care Committee.
Author contributions
NI: methodology, investigation, and writing of the original draft. KY, YN, SKo, and YF: Methodology and Investigation. TK: provision of critical materials. KH: conceptualization. SKi and KH: Supervision of funding acquisition and writing–review and editing. All authors contributed to the article and approved the submitted version.
Funding
This work was supported by JSPS KAKENHI grant numbers 19K07239 (SK), 20H05876, 20H00509, 22K19445 (KH), JST PRESTO grant number 19199152 (SK), JST Moonshot R/D grant number JPMJMS2025 (KH), AMED CREST grant numbers 21gm1010004h0106 and 21gm1310009h0002 (KH), Kobayashi Foundation Research Grant FY2017, and the Mitsubishi Foundation Research Grants in the Natural Science.
Acknowledgments
We thank Drs. Nobuhide Kobayashi, Motoyoshi Nagai, and Masayoshi Onuki for their helpful advice, and Ms. Aiko Saeki, Mr. Koya Hattori, Hiroaki Shiratori, and Yusuke Kinashi for their technical assistance. We would like to thank Editage (www.editage.com) for English language editing.
Conflict of interest
The authors declare that the research was conducted in the absence of any commercial or financial relationships that could be construed as a potential conflict of interest.
Publisher's note
All claims expressed in this article are solely those of the authors and do not necessarily represent those of their affiliated organizations, or those of the publisher, the editors and the reviewers. Any product that may be evaluated in this article, or claim that may be made by its manufacturer, is not guaranteed or endorsed by the publisher.
Supplementary material
The Supplementary Material for this article can be found online at: https://www.frontiersin.org/articles/10.3389/falgy.2022.996657/full#supplementary-material.
References
1. De Martinis M, Sirufo MM, Suppa M, Ginaldi L. New perspectives in food allergy. Int J Mol Sci. (2020) 21:1474. doi: 10.3390/ijms21041474
2. Turnbull JL, Adams HN, Gorard DA. Review article: the diagnosis and management of food allergy and food intolerances. Aliment Pharmacol Ther. (2015) 41:3–25. doi: 10.1111/apt.12984
3. Kumar S, Verma AK, Das M, Dwivedi PD. Molecular mechanisms of IgE mediated food allergy. Int Immunopharmacol. (2012) 13:432–9. doi: 10.1016/j.intimp.2012.05.018
4. Anvari S, Miller J, Yeh CY, Davis CM. IgE-mediated food allergy. Clin Rev Allergy Immunol. (2019) 57:244–60. doi: 10.1007/s12016-018-8710-3
5. Wang M, Takeda K, Shiraishi Y, Okamoto M, Dakhama A, Joetham A, et al. Peanut-induced intestinal allergy is mediated through a mast cell-IgE-FceRI-IL-13 pathway. J Allergy Clin Immunol. (2010) 126:306–316.e12. doi: 10.1016/j.jaci.2010.05.017
6. Reber LL, Marichal T, Mukai K, Kita Y, Tokuoka SM, Roers A, et al. Selective ablation of mast cells or basophils reduces peanut-induced anaphylaxis in mice. J Allergy Clin Immunol. (2013) 132:881–888.e11. doi: 10.1016/j.jaci.2013.06.008
7. Samadi N, Klems M, Untersmayr E. The role of gastrointestinal permeability in food allergy. Ann Allergy Asthma Immunol. (2018) 121:168–73. doi: 10.1016/j.anai.2018.05.010
8. Ventura MT, Polimeno L, Amoruso AC, Gatti F, Annoscia E, Marinaro M, et al. Intestinal permeability in patients with adverse reactions to food. Dig Liver Dis. (2006) 38:732–6. doi: 10.1016/j.dld.2006.06.012
9. Forbes EE, Groschwitz K, Abonia JP, Brandt EB, Cohen E, Blanchard C, et al. IL-9 and mast cell-mediated intestinal permeability predisposes to oral antigen hypersensitivity. J Exp Med. (2008) 205:897–913. doi: 10.1084/jem.20071046
10. Su GH, Ip HS, Cobb BS, Lu MM, Chen HM, Simon MC. The Ets protein Spi-B is expressed exclusively in B cells and T cells during development. J Exp Med. (1996) 184:203–14. doi: 10.1084/jem.184.1.203
11. Schotte R, Rissoan M, Bendriss-vermare N, Bridon J, Duhen T, Weijer K, et al. The transcription factor Spi-B is expressed in plasmacytoid DC precursors and inhibits T-, B-, and NK-cell development. Blood. (2003) 101:1015–23. doi: 10.1182/blood-2002-02-0438.Supported
12. Kanaya T, Hase K, Takahashi D, Fukuda S, Hoshino K, Sasaki I, et al. The Ets transcription factor Spi-B is essential for the differentiation of intestinal microfold cells. Nat Immunol. (2012) 13:729–36. doi: 10.1038/ni.2352
13. Sasaki I, Hoshino K, Sugiyama T, Yamazaki C, Yano T, Iizuka A, et al. Spi-B is critical for plasmacytoid dendritic cell function and development. Blood. (2012) 120:4733–43. doi: 10.1182/blood-2012-06-436527
14. Xiong Z, Zhu X, Geng J, Xu Y, Wu R, Li C, et al. Intestinal Tuft-2 cells exert antimicrobial immunity via sensing bacterial metabolite N-undecanoylglycine. Immunity. (2022) 55:686–700.e7. doi: 10.1016/j.immuni.2022.03.001
15. Rekhtman N, Radparvar F, Evans T, Skoultchi AI. Direct interaction of hematopoietic transcription factors PU.1 and GATA- 1: functional antagonism in erythroid cells. Genes Dev. (1999) 13:1398–411. doi: 10.1101/gad.13.11.1398
16. Zhang P, Behre G, Pan J, Iwama A, Wara-Aswapati N, Radomska HS, et al. Negative cross-talk between hematopoietic regulators: GATA proteins repress PU.1. Proc Natl Acad Sci. (1999) 96:8705–10. doi: 10.1073/pnas.96.15.8705
17. Nerlov C, Querfurth E, Kulessa H, Graf T. GATA-1 interacts with the myeloid PU.1 transcription factor and represses PU.1-dependent transcription. Blood. (2000) 95:2543–51. doi: 10.1182/blood.95.8.2543
18. Shimokawa C, Kanaya T, Hachisuka M, Ishiwata K, Hisaeda H, Kurashima Y, et al. Mast cells are crucial for induction of group 2 innate lymphoid cells and clearance of helminth infections. Immunity. (2017) 46:863–74.e4. doi: 10.1016/j.immuni.2017.04.017
19. Nakamura Y, Mimuro H, Kunisawa J, Furusawa Y, Takahashi D, Fujimura Y, et al. Microfold cell-dependent antigen transport alleviates infectious colitis by inducing antigen-specific cellular immunity. Mucosal Immunol. (2020) 13:679–90. doi: 10.1038/s41385-020-0263-0
20. Brandt EB, Strait RT, Hershko D, Wang Q, Muntel EE, Scribner TA, et al. Mast cells are required for experimental oral allergen-induced diarrhea. J Clin Invest. (2003) 112:1666–77. doi: 10.1172/JCI19785
21. Lianto P, Han S, Li X, Ogutu FO, Zhang Y, Fan Z, et al. Quail egg homogenate alleviates food allergy induced eosinophilic esophagitis like disease through modulating PAR-2 transduction pathway in peanut sensitized mice. Sci Rep. (2018) 8:1–15. doi: 10.1038/s41598-018-19309-x
22. Shin HS, Eom JE, Shin DU, Yeon SH, Lim S, Lee SY. Preventive effects of a probiotic mixture in an ovalbumin-induced food allergy model. J Microbiol Biotechnol. (2018) 28:65–76. doi: 10.4014/jmb.1708.08051
23. Fujihashi K, Dohi T, Rennert PD, Yamamoto M, Koga T, Kiyono H, et al. Peyer's patches are required for oral tolerance to proteins. Proc Natl Acad Sci. (2001) 98:3310–5. doi: 10.1073/pnas.061412598
24. Renga G, Moretti S, Oikonomou V, Borghi M, Zelante T, Paolicelli G, et al. IL-9 and mast cells are key players of candida albicans commensalism and pathogenesis in the gut. Cell Rep. (2018) 23:1767–78. doi: 10.1016/j.celrep.2018.04.034
25. Groschwitz KR, Ahrens R, Osterfeld H, Gurish MF, Han X, Åbrink M, et al. Mast cells regulate homeostatic intestinal epithelial migration and barrier function by a chymase/Mcpt4-dependent mechanism. Proc Natl Acad Sci. (2009) 106:22381–6. doi: 10.1073/pnas.0906372106
26. Burton OT, Rivas MN, Zhou JS, Logsdon SL, Darling AR, Koleoglou KJ, et al. Immunoglobulin E signal inhibition during allergen ingestion leads to reversal of established food allergy and induction of regulatory T cells. Immunity. (2014) 41:141–51. doi: 10.1016/j.immuni.2014.05.017
27. Rivas MN, Burton OT, Wise P, Charbonnier LM, Georgiev P, Oettgen HC, et al. Regulatory T cell reprogramming toward a Th2-Cezll-like lineage impairs oral tolerance and promotes food allergy. Immunity. (2015) 42:512–23. doi: 10.1016/j.immuni.2015.02.004
Keywords: Spi-B, food allergy, epithelial barrier, IgE, oral tolerance
Citation: Ishihara N, Nakamura Y, Yakabe K, Komiyama S, Fujimura Y, Kaisho T, Kimura S and Hase K (2022) Spi-B alleviates food allergy by securing mucosal barrier and immune tolerance in the intestine. Front. Allergy 3:996657. doi: 10.3389/falgy.2022.996657
Received: 18 July 2022; Accepted: 21 September 2022;
Published: 6 October 2022.
Edited by:
Yasutaka Mitamura, University of Zurich, SwitzerlandReviewed by:
Cristina Gomez-Casado, Heinrich Heine University of Düsseldorf, GermanyErin C. Steinbach, University of North Carolina at Chapel Hill, United States
© 2022 Ishihara, Nakamura, Yakabe, Komiyama, Fujimura, Kaisho, Kimura and Hase. This is an open-access article distributed under the terms of the Creative Commons Attribution License (CC BY). The use, distribution or reproduction in other forums is permitted, provided the original author(s) and the copyright owner(s) are credited and that the original publication in this journal is cited, in accordance with accepted academic practice. No use, distribution or reproduction is permitted which does not comply with these terms.
*Correspondence: Shunsuke Kimura a2ltdXJhLXNuQHBoYS5rZWlvLmFjLmpw Koji Hase aGFzZS1rakBwaGEua2Vpby5hYy5qcA==
Specialty Section: This article was submitted to Food Allergy, a section of the journal Frontiers in Allergy