- School of Science and Technology, Nottingham Trent University, Nottingham, United Kingdom
Introduction: Exercise-induced bronchoconstriction (EIB) is a prevalent condition in athletes. EIB screening studies identify many athletes with undiagnosed EIB. Moreover, there is a poor relationship between EIB and dyspnea symptoms recalled from memory.
Purpose: This study investigated: (I) the prevalence of EIB in British university field hockey athletes; (II) the effect of sex and diagnostic criteria on EIB prevalence; and (III) the association between EIB and contemporaneous dyspnea symptoms.
Methods: 52 field hockey athletes (age: 20 ± 2 years; height: 173 ± 9 cm; body mass: 72 ± 10 kg; male = 31; female = 22) completed a eucapnic voluntary hyperpnea (EVH) test with multi-dimensional dyspnea scores measured 3–10 mins post-EVH. A test was deemed positive (EIB+) if a fall index (FI) ≥10% in FEV1 occurred at two consecutive time points post-test (FIATS). Two further criteria were used to assess the effect of diagnostic criteria on prevalence: FI≥10%, determined by a pre-to-post-EVH fall in FEV1 of ≥10% at any single time-point; and FI≥10%−NORM calculated as FI≥10% but with the fall in FEV1 normalized to the mean ventilation achieved during EVH.
Results: EIB prevalence was 19% and greater in males (30%) than females (5%). In EIB+ athletes, 66% did not have a previous diagnosis of EIB or asthma and were untreated. Prevalence was significantly influenced by diagnostic criteria (P = 0.002) ranging from 19% (FIATS) to 38% (FI≥10%−NORM). Dyspnea symptoms were higher in EIB+ athletes (P ≤ 0.031), produced significant area under the curve for receive operator characteristics (AUC ≥ 0.778, P ≤ 0.011) and had high negative prediction values (≥96%).
Conclusion: Overall, 19% of university field hockey athletes had EIB, and most were previously undiagnosed and untreated. EVH test diagnostic criteria significantly influences prevalence rates, thus future studies should adopt the ATS criteria (FIATS). Contemporaneous dyspnea symptoms were associated with bronchoconstriction and had high negative prediction values. Therefore, contemporaneous dyspnea scores may provide a useful tool in excluding a diagnosis of EIB.
Introduction
Exercise-induced bronchoconstriction (EIB) is characterized by a transient narrowing of the airways following exercise. In susceptible individuals EIB is triggered by increased airway surface osmolality and reduced airway temperature caused by exercise hyperpnea, and is characterized by airway smooth muscle contraction, inflammation, and hyperaemia (1). These processes reduce airway caliber and lung function which may limit athletic performance, particularly in endurance sports (2). Screening athletes for EIB is therefore important to understand the scale of the issue and ensure athletes who require treatment are identified.
The prevalence of EIB in athletes competing in individual (e.g. badminton, rowing, speed skating, athletics) and team sports (e.g. soccer, rugby, hockey, volleyball, lacrosse) is relatively high (18%–62%) (3–5), although it may vary depending on the type of sport and the associated ventilatory demand, and the environmental conditions in which the sport is performed. A lower prevalence of EIB (13%) has been reported in non-athletes, although this may be an underestimate because this cohort only included individuals without a prior diagnosis of asthma (6). Unfortunately, there is an increasing realization that self-reported respiratory symptoms yield a high number of both false-positive and false negative EIB diagnoses (3–5, 7). Indeed, previous reports on the poor diagnostic accuracy of respiratory symptoms may be undermined by diagnosis methodology. For instance, respiratory symptoms were assessed retrospectively through memory recall with participants at rest and asymptomatic (3–5), rather than shortly after a bronchial provocation test when EIB and symptoms are present. This is problematic because the reduction in forced expiratory volume in 1 s (FEV1), a hallmark characteristic of EIB, may relate poorly to respiratory symptoms that are assessed retrospectively and reliant on memory (8). Conversely, momentary conscious respiratory symptoms are more likely to relate to the severity and underpinning pathophysiology of EIB if assessed soon after a bronchial provocation test (8).
An EIB diagnosis is typically made by measuring changes in lung function shortly after a bronchoprovocation test such as exercise, EVH or inhaled mannitol. However, previous studies have used different diagnostic criteria which may affect EIB prevalence (3–6, 9). Furthermore, sex differences in the prevalence of EIB in athletes is rarely addressed. Given that EIB may compromise health and exercise performance it is important to establish the extent of the issue EIB presents in athletes and understand the influence of diagnostic criteria and sex on prevalence. Moreover, there is a need to understand the interplay between EIB and dyspnea symptoms that are assessed soon after a bronchoprovocation test.
Therefore, the aims of the present study were to investigate: (I) the prevalence of EIB in university field hockey athletes; (II) the effect of sex and diagnostic criteria on EIB prevalence; and (III) the relationship between EIB and contemporaneous dyspnea symptoms.
Material and methods
Participants
Fifty-three British university field hockey athletes (age: 20 ± 2 years; height: 173 ± 9 cm; body mass: 72 ± 10 kg; male = 31; female = 22), training and competing 8 ± 3 h per week and competing in British University Championships fixtures, provided written informed consent to participate in the study. The study was approved by the Nottingham Trent University Human Ethics Committee (approval number: 582) and all procedures conformed to the standard set by the Declaration of Helsinki.
Experimental design
Testing took place across four consecutive months (November to February). Participants attended the laboratory on one occasion to perform an EVH test with spirometry measured before and after to evaluate the presence and severity of EIB. Ten minutes after the EVH test, participants completed a multidimensional dyspnea profile (MDP) (10). Participants refrained from exercise and caffeine ingestion on the day of testing. Participants with prescribed asthma medication (n = 4) were instructed to cease taking their medication prior to the EVH test (short-acting β2-agonist: ≥8 h; short-acting muscarinic antagonist: ≥4-h; long-acting bronchodilators (≥24-h; inhaled corticosteroids: ≥12-h; combined long-acting bronchodilators + inhaled corticosteroids: ≥24-h) (1, 11, 12).
Spirometry and EVH test
Baseline spirometry was assessed according to ATS/ERS guidelines (13) using a pneumotachograph (Pneumotach; Vitalograph, Buckingham, UK) calibrated with a 3-L syringe. EIB was assessed using an EVH test comprising 6-minutes of breathing at a target minute ventilation () of 85% of predicted maximal voluntary ventilation (MVV) (baseline FEV1 × 30) (12, 15). The EVH test was set up as previously described (12) and was considered valid if the mean was ≥60% of predicted MVV (baseline FEV1 × 21), or a participant averaged <60% of predicted MVV whilst having a positive EVH test response. If a participant did not meet the criteria for a valid EVH test they were invited back for a second attempt. was recorded from the volume of air passed through a dry gas meter every minute. Spirometry was assessed in duplicate 3, 6, 10, 15, and 20-min after the EVH test, with the highest values used for subsequent analysis. The difference between pre and post EVH test spirometry was termed the fall index (FI).
EIB diagnostic criteria
EIB was diagnosed when FEV1 fell, relative to baseline, by ≥10% at two consecutive time points after EVH, which conforms to ATS guidelines (hereafter termed FIATS) (1, 14). Participants with a positive or negative FIATS EVH test response were classified as either EIB positive (EIB+) or EIB negative (EIB−). To evaluate the effect of diagnostic criteria on the prevalence of EIB, two additional criteria were used: (1) a pre-to-post-EVH fall in FEV1 of ≥10% at any single time-point (hereafter termed FI≥10%) (15); and (2) calculated the same as FI≥10% but with the FI normalized to the mean achieved during EVH (hereafter termed FI≥10%−NORM) (16) using the following calculation:
Multi-dyspnea profile
Between 3 and 10 mins after the EVH test [which typically captures the peak fall in FEV1 in EIB+ individuals (3)] participants completed a MDP(10). The MDP consists of 11 items evaluating sensory and affective dimensions of dyspnea, although affective dimensions were not assessed in the present study. The first item (A1) assesses the unpleasantness or discomfort of breathing on a scale ranging from 0 (“neutral”) to 10 (“unbearable”). The subsequent five items assess the intensity of sensory dimensions on a scale ranging from 0 (“none”) to 10 (“as intense as I can imagine”). The five items include, S1: my breathing requires muscle work or effort; S2: I am not getting enough air; S3: my chest and lungs feel tight or constricted; S4: my breathing requires mental effort or concentration; and S5: I am breathing a lot. Items were scored individually and as an “immediate perception domain score” (IPDS) calculated as the sum of A1 and S1–S5.
Statistical analysis
Participants were grouped according to EVH test response (EIB+ or EIB−) and sex. Independent samples t-tests assessed between-group (EIB+ vs. EIB−; male vs. female) differences in baseline spirometry, average percentage of MVV achieved during EVH, peak fall in FEV1, and MDP items. Receiver operator characteristic (ROC) curves were determined for MDP items that differed between EIB+ and EIB− groups. Stepwise multiple regression was performed using the peak fall in FEV1 as the dependent variable and MDP items as candidate predictors. Mixed model repeated measures ANOVA assessed the effects of time (baseline and 3, 10, 15, and 20-min post-EVH test) on FEV1, with a between-subjects factor of EIB diagnosis (EIB+ vs. EIB−). Significant main and interaction effects were followed by independent samples t-tests at each measurement point. Within-group changes in FEV1 were assessed using one-way repeated measures ANOVA followed by Tukey's post-hoc test. A Cochran Q test followed by a McNemar post-hoc test assessed differences in the number of EIB+ and EIB− diagnoses based on each FI criteria. Pearson's correlation coefficient was used to determine the relationship between selected variables. Statistical significance was set at P < 0.05, except for the McNemar test, which was set at P < 0.0083. For significant differences, 95% confidence intervals are presented. Effect sizes are presented as Cohen's d. Data were analyzed using IBM SPSS Statistics V26.0 and presented as mean ± SD unless indicated otherwise.
Results
One participant had a baseline FEV1 < 70% of predicted and therefore could not perform an EVH test. Five participants did not achieve an average MVV and not experience a ≥10% fall in FEV1 from pre-to-post-EVH test. One of these five participants accepted the invitation to repeat the EVH test on a separate day. During their second EVH test, this participant was again unable to achieve an average MVV and did not experience a ≥10% fall in FEV1 from pre-to-post-EVH test. Accordingly, all five participants were excluded from further analysis. Therefore, 47 participants (male = 27; female = 20) completed a valid EVH test (Figure 1). Of this cohort, 4 (9%) had a current GP diagnosis of asthma and none had a current EIB diagnosis.
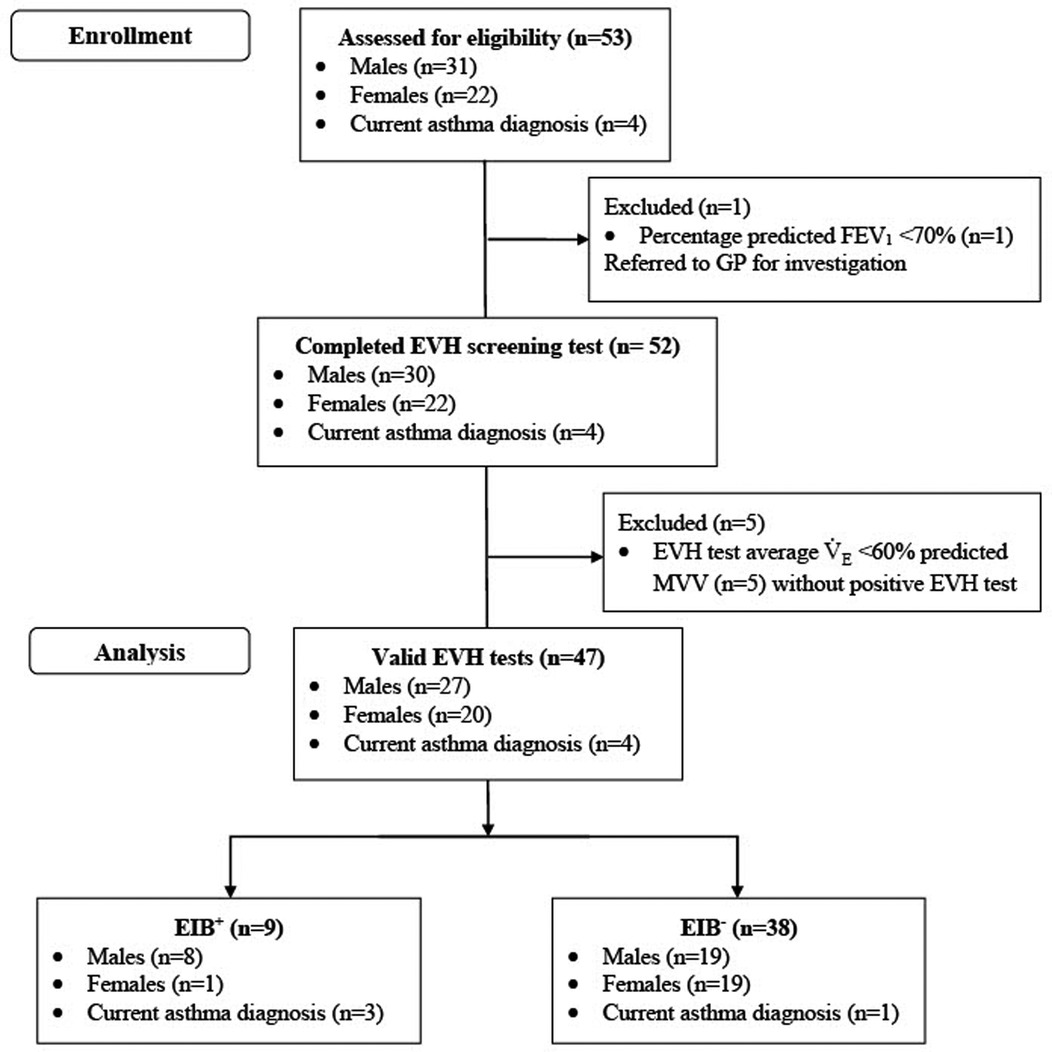
Figure 1. Participant flow diagram. FEV1, forced expiratory volume in 1 s; EVH, eucapnic voluntary hyperpnea; MVV, maximal voluntary ventilation.
Baseline spirometry
Baseline spirometry (Table 1) was not different between EIB+ and EIB− groups (P = 0.273–0.816; d = 0.09–0.41). FEV1 (% predicted) was lower in males (91 ± 10%) than females (97 ± 9%) [P = 0.032; 95% CI (−12, −0.6%); d = 0.63]. FVC (% predicted) was lower in males (94 ± 10%) than females (100 ± 10%) [P = 0.050; 95% CI (−11, −0.0004%); d = 0.58].
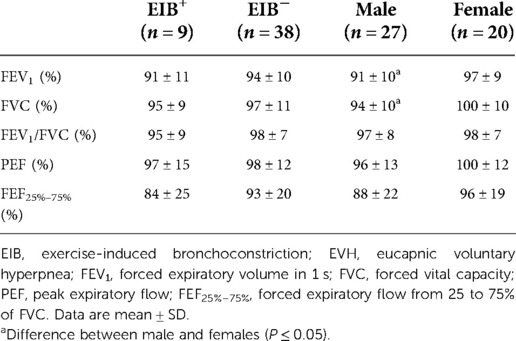
Table 1. Baseline pulmonary function for EIB+ and EIB− groups, and male and female groups irrespective of EIB diagnosis. Values represent percentage of the predicted value.
Spirometry after the EVH test
For the percentage fall in FEV1, there was a main effect of time and a group × time interaction (P ≤ 0.001). The fall in FEV1 was greater in the EIB+ group than the EIB− group at all-time points after EVH (P ≤ 0.001; d = 1.2–2.1). In the EIB+ group, FEV1 was below baseline throughout recovery (P ≤ 0.006; d = 1.35–1.88). In the EIB+ group, peak falls in FEV1 were observed at 3-min (n = 4), 6-min (n = 2), 10-min (n = 2), and 15-min (n = 1) after EVH (Figure 2). By design, the peak fall in FEV1 was greater in the EIB+ (−18 ± 3%) than EIB− (−7 ± 3%) group [P < 0.001; 95% CI (−14, −10%); d = 2.23]. The prevalence of EIB+ for all participants, males, and females, was 19%, 30%, and 5% respectively. Of the EIB+ participants 6/9 (66%) did not have a previous diagnosis of EIB or asthma. Three of the four athletes (75%) with a prior diagnosis of asthma were EIB+.
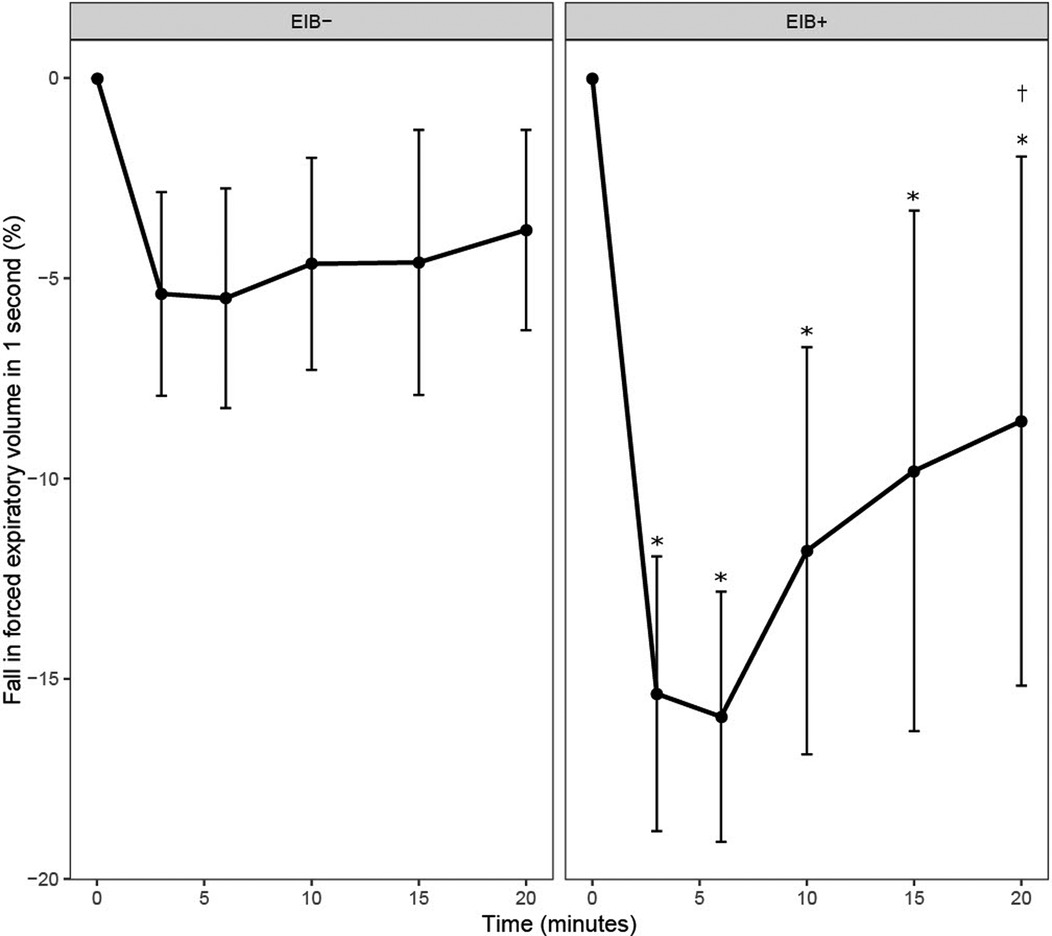
Figure 2. Fall in forced expiratory volume in 1 s (FEV1) from baseline (0) following the eucapnic voluntary hyperpnea test in EIB+ and EIB− groups. *Different from baseline in EIB+ group (P ≤ 0.045). †Different from 3- and 6-min post-EVH in the EIB+ group (P ≤ 0.002).
Average minute ventilation during the EVH test
Of the 52 participants completing an EVH test, six achieved less than 60% of predicted MVV, one of which was EIB+. Average during the EVH test ranged from 53%–93% of predicted MVV in the 47 participants that completed a valid EVH test. Average was not different between EIB+ (69 ± 10% MVV) and EIB− (72 ± 7% MVV) groups (P = 0.246; d = 0.43), or between males (72 ± 8% MVV) and females (71 ± 7% MVV) (P = 0.492; d = 0.19). The average during the EVH test was not related to the peak fall in FEV1 (r = 0.22, P = 0.147) (Figure 3).
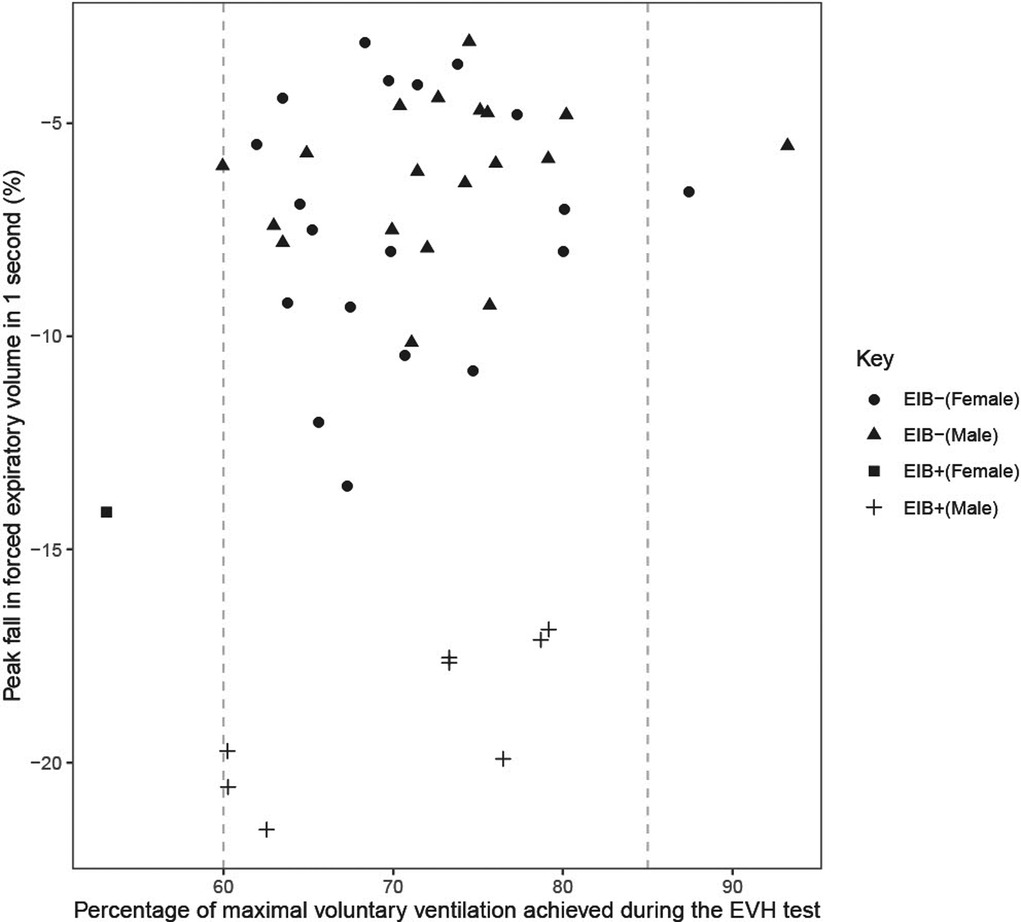
Figure 3. Peak fall in forced expiratory volume in 1 s (FEV1) from pre to post eucapnic voluntary hypernea (EVH) test in relation to the percentage of maximal voluntary ventilation (MVV) achieved during the EVH test. Vertical dashed line indicates the 60% and 85% of predicted MVV targets for participants during the EVH test.
Effect of diagnostic criteria on EIB prevalence
The prevalence of EIB+ differed between FI criteria [χ2 (3) = 14.818, P = 0.002] (Table 2). Prevalence was greater under FI10%−NORM than FIATS (P = 0.004), but no differences were found between FIATS and FI≥10%, or FI≥10% and FI10%−Norm (P ≥ 0.063).
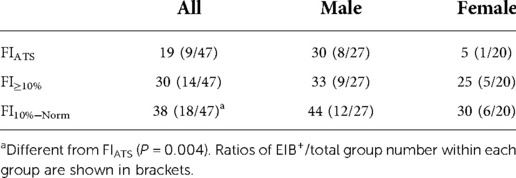
Table 2. Prevalence (%) of EIB+ based on three different fall index (FI) criteria for all athletes (n = 47), males (n = 27), and females (n = 20).
Dyspnea symptoms after the EVH test
Of the 47 participants completing a valid EVH test, 43 successfully completed the MDP. Of these, 9 were in the EIB+ group. The IPDS (sum of all questions) was higher in the EIB+ (15 ± 9) than the EIB− (6 ± 8) group [P = 0.031; 95% CI (2, 15); d = 1.1]. A1 (unpleasantness or discomfort of breathing) was higher in the EIB+ (5 ± 2) than the EIB− (1 ± 2) group [P = 0.001; 95% CI (1, 4); d = 0.72]. Similarly, S3 (my chest and lungs feel tight or constricted) was higher in the EIB+ (3 ± 3) than EIB− (1 ± 2) group [P = 0.014; 95% CI (0, 4); d = 1.4]. S1, S2, S4 and S5 items were not different between groups (P = 0.060–0.824; d = 0.09–0.92). ROC analyses produced significant area under the curves for A1 [AUC = 0.843; P ≤ 0.001; 95% CI (0.727, 0.959)], IPDS [AUC = 0.778; P = 0.011; 95% CI (0.635, 0.92)] scores but not S3 scores [AUC = 0.706; P = 0.055; 95% CI (0.496, 0.916)] (Figure 4). The maximal combined sensitivity and specificity cut-off for detecting EIB corresponded to scores of A1 = 3 (sensitivity = 1; specificity = 0.63; positive prediction value = 43%; negative prediction value = 100%), IPDS = 7 (sensitivity = 0.89; specificity = 0.66; positive prediction value = 42%; negative prediction value = 96%), and S3 = 3 (sensitivity = 0.67; specificity = 0.77; positive prediction value = 43%; negative prediction value = 90%). The peak fall in FEV1 after EVH correlated negatively with IPDS (r = −0.31; P = 0.044) and A1 (r = −0.45; P = 0.003) scores but not S3 scores (r = −0.28; P = 0.065). Multiple regression analysis of the peak fall in FEV1 revealed item A1 to be the only contributing factor.
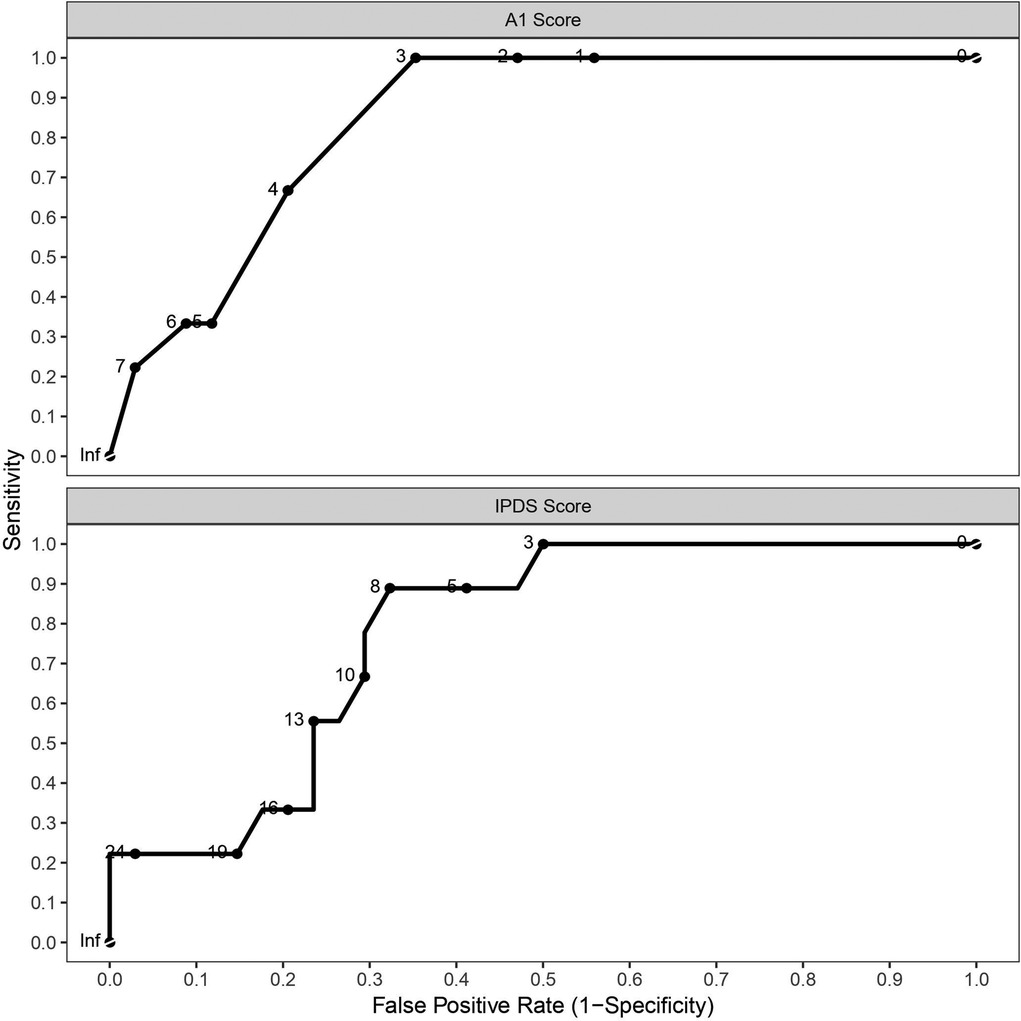
Figure 4. Receiver operating characteristics curves for multi-dyspnea profile items A1 (unpleasantness or discomfort of breathing) and IPDS (sum of all questions).
Discussion
Main findings
The main findings of the present study were fourfold: (I) prevalence of EIB in British university field hockey athletes was 19% and greater in males (30%) than females (5%); (II) out of the nine participants identified as having EIB, six (66%) did not have a previous diagnosis of EIB or asthma and were untreated despite having a mean peak fall in FEV1 of 18%; (III) prevalence of EIB ranged from 19%–38% depending on the diagnostic FI criteria used; and (IIII) contemporaneous dyspnea symptoms (measured shortly after the EVH test) were higher in EIB+ participants and were sensitive in detecting an EIB diagnosis.
Prevalence of EIB
The present study comprised, to date, the largest cohort of field hockey athletes screened for EIB. The prevalence of EIB in our cohort of field hockey athletes (19%) supports that EIB is prevalent in competitive athletes (3–5). Interestingly, none of the nine EIB+ athletes had a formal diagnosis of EIB. Three had a current GP diagnosis of asthma along with prescribed asthma maintenance and reliever therapy. Between 40%–90% of individuals with a physician diagnosis of asthma are estimated to have EIB (17). Similarly, we found 75% of participants with a previous diagnosis of asthma to be EIB+. Conversely, six of nine EIB+ athletes (66%) had no previous diagnosis of asthma and EIB and were untreated. These findings support previous screening studies in elite athletes which uncover a significant amount of EIB+ athletes with no prior diagnosis (3). These findings highlight the importance of screening athletes for EIB to ensure they receive appropriate treatment. Adequate treatment is important to facilitate athletic performance and reduce the risk of life-threatening bronchoconstriction.
EIB prevalence in the present study was lower than reported in other mixed sex university athletic populations (39%–43%) (4, 5). This is due, in part, to previous studies using FI≥10% to diagnose EIB, which is less conservative than the FIATS criteria and therefore results in higher prevalence rates (9). Indeed, using FI≥10% in the current study yielded a 30% prevalence rate which is more comparable to previous reports (3–5, 18). Previous studies (4, 5) also screened for EIB in athletes from different sports, which affects prevalence rates. Compared to the present study, a higher prevalence of EIB (38%), based on FIATS, has been reported in 21 elite hockey athletes (sex not reported) (3). Higher prevalence may be explained, in part, by higher training volumes performed by elite hockey athletes, which exacerbates stress on the airways and thereby increase the risk of developing EIB (19). Compared with the present cohort, the elite hockey athletes also achieved a higher % of predicted MVV during EVH (79% vs. 71% in the present study); however, a relationship between the % of predicted MVV achieved during EVH and the subsequent fall in FEV1 was not observed in the present study nor in the study of elite hockey athletes (3).
Of the 52 athletes completing an EVH test, six athletes (12%) were unable to achieve the ventilation requirements for a valid EVH test (≥60% predicted MVV) (12), including one EIB+ athlete. This was despite the investigators providing extensive encouragement and coaching for participants to achieve their target during EVH tests. Previous reports have shown 4% of elite athletes and 21% of recreationally active non-athletes are unable to achieve ≥60% predicted MVV during the EVH test (3, 6), suggesting that valid EVH tests are more likely in elite athletes compared to non-elite athletes and non-athletes. In the present study, only two participants were able to equal or exceed their EVH test target (≥85% predicted MVV) during the EVH test. This target during EVH is often prescribed for testing in athletes (12), however this may not be attainable by most non-elite athletes. Due to issues with some participants achieving their target during an EVH test, classifying individuals with borderline responses can be problematic. For example, in the present study, five athletes had ≥10% drop in FEV1 at only one time point post-EVH, which classifies them as EIB− under the ATS guidelines. Borderline responses, particularly those with a of predicted MVV, should thus be interpreted with care and additional EVH testing may be necessary to facilitate an accurate diagnosis (20). Moreover, further research is needed to establish: (1) whether borderline responses have clinical relevance or implications for exercise performance, and (2) the extent to which borderline responses reflect true EIB, which could be explored by examining the response to a bronchodilator after the EVH test.
Sex differences in EIB prevalence
In the present study, the prevalence of EIB in field hockey athletes was lower in females (5%) than males (30%). Similar EIB prevalence rates (based on FI≥10%) between male (42%) and female (38%) university athletes have been reported previously (4), although the cohort of 22 sports did not include field hockey, which might partly explain this discrepancy. It might also be explained, in part, by differences in EIB diagnostic criteria. In support, if the diagnosis of EIB in the present study is based on FI≥10%, the prevalence in males (33%) and females (25%) is more comparable. In contrast to the present study, the prevalence of EIB was higher in female (26%) than male (18%) elite winter sport athletes (21). An explanation for this discrepancy is unclear but might be related to sport-specific differences such as environmental conditions and/or the type of challenge (exercise vs. EVH). It is also noteworthy that EIB screening in females is confounded by fluctuations in sex hormones during the menstrual cycle, with peak falls in FEV1 following exercise challenge tests being 4.5% greater during the mid-luteal phase of the menstrual cycle in comparison with the mid-follicular phase (22). Although it remains to be empirically tested/confirmed, fluctuations in female reproductive hormones would be expected to have similar effects on EVH test responses. Therefore, a limitation of the present study, and previous studies investigating EIB prevalence in females, is that menstrual cycle phase and contraceptive therapies were not documented / controlled. Nevertheless, our results suggest that the prevalence of EIB, when diagnosed using FIATS, is lower in female compared to male field hockey athletes.
Dyspnea and EIB
Dyspnea symptoms, namely the “unpleasantness or discomfort of breathing” (A1), “my chest and lungs feel tight or constricted” (S3), and the sum of A1 and scores relating to the intensity of sensory dimensions (IPDS), were higher in EIB+ than EIB− field hockey athletes. Interestingly, A1 and IPDS scores had a sensitivity and specificy for detecting EIB comparable to a methacholine challenge (23). Our findings contrast previous studies reporting that only ∼50% of EIB+ individuals report dyspnea symptoms and that such symptoms are not associated with EIB (3–5, 7, 18). However, these studies administered questionnaires with participants at rest and asymptomatic, rather than soon after an exercise or EVH challenge when EIB and symptoms are present. This is a limitation for two reasons: (I) symptom recall from previous habitual exercise would lack validity if the stimulus was insufficient to induce bronchoconstriction (e.g., exercise MVV); and (II) retrospective self-report measures that rely on memory are poorly tied to momentary biological processes and their fluctuation (8). Therefore, a strength of the present study is that the MDP was administered 3–10 mins after the EVH test and was therefore more likely to connect momentary conscious dyspnea symptoms with the underpinning pathophysiology of EIB (8). This may explain why the MDP items IPDS and A1 were both associated with the post-EVH fall in FEV1 and were good predictors of EIB classification in the present cohort. These results therefore suggest that contemporaneous dyspnea symptoms are associated with EIB diagnosis. Interestingly, an A1 score of 3 had a 100% negative prediction value to the post-EVH fall in FEV1 and, therefore, from a practical perspective this offers a useful tool for coaches / practitioners and athletes to rule out the likelihood that EIB is/was present.
Conclusion
In conclusion, the prevalence of EIB in university field hockey athletes was 19% and greater in males than females. Many of the athletes identified as having EIB had no previous diagnosis of EIB or asthma despite having significant reductions in FEV1 and scoring highly for dyspnea symptoms. The prevalence of EIB depends on the FI criteria employed; thus, previous studies that did not use FIATS may have overestimated the prevalence of EIB. Finally, contemporaneous dyspnea symptoms were associated with EIB, had high negative prediction value, and may therefore offer a useful tool in ruling out the presence of EIB.
Data availability statement
The raw data supporting the conclusions of this article will be made available by the authors, without undue reservation.
Ethics statement
The studies involving human participants were reviewed and approved by Nottingham Trent University Human Invasive Ethical Review Committee. The patients/participants provided their written informed consent to participate in this study.
Author contributions
RN, GS, NW, PL, / MJ all contributed to trial design, preparation and analysis, manuscript preparation and review. RN, GS, / PL all contributed to data collection. All authors contributed to the article and approved the submitted version.
Funding
This study was part funded by a Physiological Society Research Grant to NCW supporting lab consumable purchases for EVH testing.
Conflict of interest
The authors declare that the research was conducted in the absence of any commercial or financial relationships that could be construed as a potential conflict of interest.
Publisher's note
All claims expressed in this article are solely those of the authors and do not necessarily represent those of their affiliated organizations, or those of the publisher, the editors and the reviewers. Any product that may be evaluated in this article, or claim that may be made by its manufacturer, is not guaranteed or endorsed by the publisher.
References
1. Weiler JM, Brannan JD, Randolph CC, Hallstrand TS, Parsons J, Silvers W, et al. Exercise-induced bronchoconstriction update—2016. J Allergy Clin Immunol. (2016) 138(5):1292–5. doi: 10.1016/j.jaci.2016.05.029
2. Price OJ, Hull JH, Backer V, Hostrup M, Ansley L. The impact of exercise-induced bronchoconstriction on athletic performance: a systematic review. Sports Med. (2014) 44(12):1749–61. doi: 10.1007/s40279-014-0238-y
3. Dickinson J, McConnell A, Whyte G. Diagnosis of exercise-induced bronchoconstriction: eucapnic voluntary hyperpnoea challenges identify previously undiagnosed elite athletes with exercise-induced bronchoconstriction. Br J Sports Med. (2011) 45(14):1126–31. doi: 10.1136/bjsm.2010.072520
4. Parsons JP, Kaeding C, Phillips G, Jarjoura D, Wadley G, Mastronarde JG. Prevalence of exercise-induced bronchospasm in a cohort of varsity college athletes. Med Sci Sports Exerc. (2007) 39(9):1487–92. doi: 10.1249/mss.0b013e3180986e45
5. Burnett DM, Burns S, Merritt S, Wick J, Sharpe M. Prevalence of exercise-induced bronchoconstriction measured by standardized testing in healthy college athletes. Respir Care. (2016) 61(5):571–6. doi: 10.4187/respcare.04493
6. Molphy J, Dickinson J, Hu J, Chester N, Whyte G. Prevalence of bronchoconstriction induced by eucapnic voluntary hyperpnoea in recreationally active individuals. J Asthma. (2014) 51(1):44–50. doi: 10.3109/02770903.2013.838256
7. Ansley L, Kippelen P, Dickinson J, Hull JH. Misdiagnosis of exercise-induced bronchoconstriction in professional soccer players. Allergy. (2012) 67(3):390–5. doi: 10.1111/j.1398-9995.2011.02762.x
8. Conner TS, Barrett LF. Trends in ambulatory self-report: the role of momentary experience in psychosomatic medicine. Psychosom Med. (2012) 74(4):327. doi: 10.1097/PSY.0b013e3182546f18
9. Koch S, Sinden SM, Koehle MS. Inconsistent calculation methodology for the eucapnic voluntary hyperpnoea test affects the diagnosis of exercise-induced bronchoconstriction. BMJ Open Respir Res. (2018) 5(1):e000358. doi: 10.1136/bmjresp-2018-000358
10. Banzett RB, O'Donnell CR, Guilfoyle TE, Parshall MB, Schwartzstein RM, Meek PM, et al. Multidimensional Dyspnea Profile: an instrument for clinical and laboratory research. Eur Respir J. (2015) 45(6):1681–91. doi: 10.1183/09031936.00038914
11. Anderson SD, Kippelen P. Assessment of EIB: what you need to know to optimize test results. Immunol. Allergy Clin.. (2013) 33(3):363–80. doi: 10.1016/j.iac.2013.02.006
12. Hull JH, Ansley L, Price OJ, Dickinson JW, Bonini M. Eucapnic voluntary hyperpnea: gold standard for diagnosing exercise-induced bronchoconstriction in athletes? Sports Med. (2016) 46(8):1083–93. doi: 10.1007/s40279-016-0491-3
13. Miller MR. ATS/ERS task force: standardisation of spirometry. Eur Respir J. (2005) 26:319–38. doi: 10.1183/09031936.05.00034805
14. Parsons JP, Hallstrand TS, Mastronarde JG, Kaminsky DA, Rundell KW, Hull JH, et al. An official American Thoracic Society clinical practice guideline: exercise-induced bronchoconstriction. Am J Respir Crit Care Med. (2013) 187(9):1016–27. doi: 10.1164/rccm.201303-0437ST
15. Anderson SD, Argyros GJ, Magnussen H, Holzer K. Provocation by eucapnic voluntary hyperpnoea to identify exercise induced bronchoconstriction. Br J Sports Med. (2001) 35(5):344–7. doi: 10.1136/bjsm.35.5.344
16. Hurwitz KM, Argyros GJ, Roach JM, Eliasson AH, Phillips YY. Interpretation of eucapnic voluntary hyperventilation in the diagnosis of asthma. Chest. (1995) 108(5):1240–5. doi: 10.1378/chest.108.5.1240
17. McFadden ER Jr, Gilbert IA. Exercise-induced asthma. N Engl J Med. (1994) 330(19):1362–7. doi: 10.1056/NEJM199405123301907
18. Jackson AR, Hull JH, Hopker JG, Dickinson JW. Impact of detecting and treating exercise-induced bronchoconstriction in elite footballers. ERJ Open Res. (2018) 4(2):00122-2017. doi: 10.1183/23120541.00122-2017
19. Kippelen P, Anderson SD. Pathogenesis of exercise-induced bronchoconstriction. Immunol Allergy Clin. (2013) 33(3):299–312. doi: 10.1016/j.iac.2013.02.002
20. Price OJ, Ansley L, Hull JH. Diagnosing exercise-induced bronchoconstriction with eucapnic voluntary hyperpnea: is one test enough? J Allergy Clin Immunol. (2015) 3(2):243–9. doi: 10.1016/j.jaip.2014.10.012
21. Wilber RL, Rundell KW, Szmedra L, Jenkinson DM, Im J, Drake SD. Incidence of exercise-induced bronchospasm in Olympic winter sport athletes. Med Sci Sports Exercise. (2000) 32(4):732–7. doi: 10.1097/00005768-200004000-00003
22. Stanford KI, Mickleborough TD, Ray S, Lindley MR, Koceja DM, Stager JM. Influence of menstrual cycle phase on pulmonary function in asthmatic athletes. Eur J Appl Physiol. (2006) 96(6):703–10. doi: 10.1007/s00421-005-0067-7
Keywords: exercise-induced bronchoconstriction, exercise-induced asthma, dyspnea, athletes, eucapnic voluntary hyperpnea, prevalence
Citation: Needham RS, Sharpe GR, Williams NC, Lester PA and Johnson MA (2022) Exercise-induced bronchoconstriction in university field hockey athletes: Prevalence, sex differences, and associations with dyspnea symptoms. Front. Allergy 3:994947. doi: 10.3389/falgy.2022.994947
Received: 15 July 2022; Accepted: 12 September 2022;
Published: 30 September 2022.
Edited by:
Andrew Simpson, University of Hull, United KingdomReviewed by:
John Molphy, Liverpool John Moores University, United KingdomAnnalisa Cogo, Istituto Pio XII, Italy
© 2022 Needham, Sharpe, Williams, Lester and Johnson. This is an open-access article distributed under the terms of the Creative Commons Attribution License (CC BY). The use, distribution or reproduction in other forums is permitted, provided the original author(s) and the copyright owner(s) are credited and that the original publication in this journal is cited, in accordance with accepted academic practice. No use, distribution or reproduction is permitted which does not comply with these terms.
*Correspondence: Neil C. Williams bmVpbC53aWxsaWFtc0BudHUuYWMudWs=
Specialty Section: This article was submitted to Asthma, a section of the journal Frontiers in Allergy